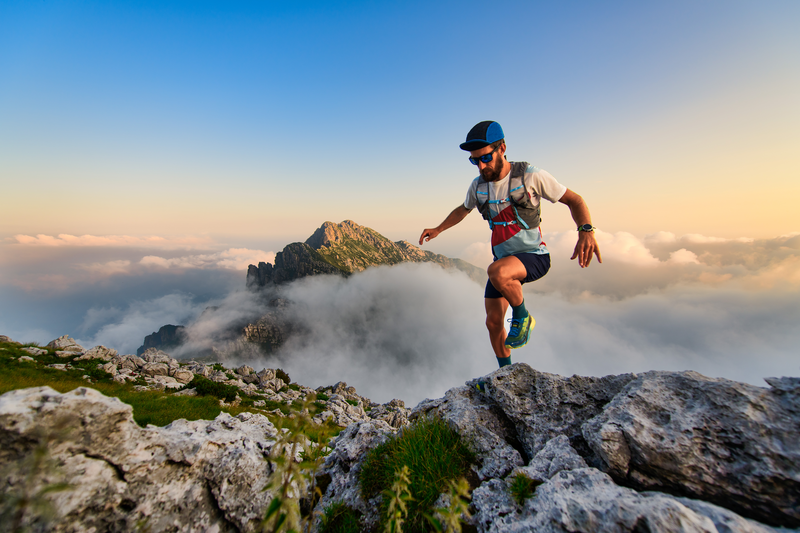
95% of researchers rate our articles as excellent or good
Learn more about the work of our research integrity team to safeguard the quality of each article we publish.
Find out more
EDITORIAL article
Front. Cell. Neurosci. , 09 August 2024
Sec. Non-Neuronal Cells
Volume 18 - 2024 | https://doi.org/10.3389/fncel.2024.1463579
This article is part of the Research Topic 15 Years of Frontiers in Cellular Neuroscience: Myelination and Remyelination Processes View all 5 articles
Editorial on the Research Topic
15 years of frontiers in cellular neuroscience: myelination and remyelination processes
Myelin, the insulating sheath around nerve axons, plays a critical role in the conduction of electrical signals within the nervous system (Nave, 2010). Comprising nearly half of the brain's white matter and even more in peripheral myelinated axons, myelin is essential for proper nervous system development and maintenance. Myelin sheaths are lipid-rich substances produced by oligodendrocytes in the central nervous system (CNS) and Schwann cells (SCs) in the peripheral nervous system (PNS) (Figure 1) (Balakrishnan et al., 2020; Yu et al., 2023). One oligodendrocyte in the CNS can insulate several axons, unlike SCs in the PNS, which wrap around only a single axon. Decades of research, propelled by cutting-edge technologies, have significantly advanced our knowledge of myelin's structure, functions, and the dynamic processes of myelination and remyelination. Myelination, regulated by a precise genetic program, is essential for developmental neurobiology and ongoing neuronal function and integrity (Sock and Wegner, 2019). Disruptions in myelination, seen in conditions like multiple sclerosis, Guillain-Barre syndrome, and Charcot-Marie-Tooth disease, can result in severe neurological deficits (Mehndiratta and Gulati, 2014). However, the nervous system can repair itself through remyelination, a regenerative process where damaged myelin sheaths are repaired or replaced. This process is carried out by glial progenitor cells in the CNS and residual SCs in the PNS (Momenzadeh and Jami, 2021). Understanding these mechanisms is key to developing treatments for a wide array of neurological disorders, highlighting the importance of myelination and remyelination in neurobiology. This editorial synthesizes findings from two reviews and two research articles, shedding light on the latest advancements in neuroimaging and cellular biology that enhance our understanding of myelin dynamics in both healthy and diseased states of the nervous system.
Figure 1. Illustrates the processes of myelination and remyelination in both the CNS and PNS. (A) In the CNS, the process starts with oligodendrocyte progenitor cells and progresses through several stages to produce myelinating oligodendrocytes. Following demyelinating injuries, these progenitor cells migrate, proliferate, and differentiate at the injury site, leading to remyelination by forming new myelin sheaths. (B) In the PNS, Schwann cells evolve from neural crest cells through several developmental stages, culminating in mature myelinating or non-myelinating Schwann cells. These mature cells are pivotal in forming the myelin sheath, which is essential for insulating axons, thereby enhancing the speed and efficiency of electrical signal transmission across nerve fibers. Damage to these cells can lead to demyelination, with adjacent Schwann cells undergoing dedifferentiation and redifferentiation to support nerve regeneration. Created with BioRender.com.
Modern neuroimaging techniques provide crucial insights into myelin dynamics in the human brain, both under normal and pathological conditions (Laule et al., 2007). The review by Kujawa et al. presents new magnetic resonance imaging (MRI) techniques and biophysical models to map myelin in vivo, highlighting the potential of physical exercise to influence myelination and remyelination in the human brain. It details current research, including four cross-sectional studies, four longitudinal studies, and a case report, showcasing the beneficial effects of an active lifestyle on myelin content across all ages. The findings suggest that intensive aerobic exercise can induce myelin expansion, underscoring the importance of exercise in managing demyelination in aging and neurodegenerative conditions. This review advocates for further research to determine the most beneficial exercise intensities for neurological health, making it an invaluable asset for clinical and research applications.
The remarkable adaptability of SCs is crucial after nerve damage or in cases of demyelinating neuropathies, where they first dedifferentiate and then redifferentiate to aid in nerve regeneration and recovery (Boerboom et al., 2017). The review article by Zhang et al. discusses the critical issue of delayed peripheral nerve injury (PNI) repair in elderly patients, focusing on the role of aging SCs. As the primary facilitators of nerve repair, SCs orchestrate various reparative functions, including demyelination, secretion of neurotrophic factors, and axon remyelination. This review highlights how structural and functional changes in aged SCs contribute to diminished nerve repair capabilities that result in chronic pain, muscle atrophy, and severe disability. Exploring these age-related alterations emphasizes the urgent need for further research into SC biology to potentially enhance therapeutic strategies for PNI in the elderly.
The specific localization of proteins within the nervous system's various cells is crucial for their functionality, impacting nerve development and maintenance (Sock and Wegner, 2019). The study by Fazal et al. focuses on the distribution and functionality of SARM1 in myelinating glia cells, addressing whether its dysfunction could impact neuropathology or interfere with myelination therapies. The study reveals that while SARM1 mRNA and protein are present in oligodendrocytes, with activation leading to cell death, it is notably absent or non-functional in peripheral glia such as SCs and satellite glia. This suggests that therapeutic strategies targeting SARM1 to preserve axons in nervous system diseases are unlikely to affect myelination negatively. The findings also underscore that SARM1 is not necessary for the initiation or maintenance of myelination in both the central and peripheral nervous systems. This research crucially informs the development of SARM1 inhibitors as potential treatments for neurological disorders, providing a clearer path for targeting this protein without harming myelin integrity.
Demyelinating diseases cause severe long-term neurological damage. Promoting remyelination can restore nerve function and prevent further neuronal loss and clinical disability, driving research into drugs that could enhance remyelination for therapeutic use (Harlow et al., 2015). Cisneros-Mejorado et al. explore the potential of β-carbolines to enhance remyelination in a rat model of demyelination in the inferior cerebellar peduncle (DRICP model). Employing the DRICP model, the authors induced demyelination using ethidium bromide, confirming the damage histologically and assessing it with diffusion-weighted MRI. The study evaluated the remyelinating effects of three β-carbolines that modulate the GABAA receptor in oligodendrocytes. Notably, the N-butyl-β-carboline-3-carboxylate (β-CCB) and ethyl 9H-pyrido [3,4-b]indole-3-carboxylate (β-CCE) demonstrated significant efficacy in promoting remyelination as evidenced by improved dMRI metrics and increased myelin content histologically. These findings suggest that specific β-carbolines could be promising in therapeutic strategies targeting white matter recovery.
The Research Topic of articles reviewed herein not only enrich our understanding of myelin dynamics in both normal and pathological states but spotlight the intrinsic capability of the nervous system to adapt and repair itself. From enhancing our grasp of neuroimaging techniques to unraveling the molecular intricacies of myelination and remyelination processes, these studies contribute to neuroscientific progress; in addition, they emphasize the potential of targeted physical and pharmacological interventions to mitigate or reverse the effects of demyelinating conditions. As research continues to evolve, these insights hold the promise of improving diagnostic, therapeutic, and preventive strategies in neurology, thereby bettering patient outcomes in the face of debilitating neurological diseases. This underscores the critical importance of ongoing research in myelin biology as a cornerstone for advancing our approach to neurological health and disease management.
QQ: Methodology, Software, Writing – original draft, Writing – review & editing. BH: Conceptualization, Software, Supervision, Writing – original draft, Writing – review & editing.
The author(s) declare that financial support was received for the research, authorship, and/or publication of this article. QQ was supported by a Veteran Affair RR&D Career Development Award (1IK1RX003985-01A1). BH was supported by a National Institute of Neurological Disorders and Stroke grant (R01NS124813).
The authors declare that the research was conducted in the absence of any commercial or financial relationships that could be construed as a potential conflict of interest.
All claims expressed in this article are solely those of the authors and do not necessarily represent those of their affiliated organizations, or those of the publisher, the editors and the reviewers. Any product that may be evaluated in this article, or claim that may be made by its manufacturer, is not guaranteed or endorsed by the publisher.
Balakrishnan, A., Belfiore, L., Chu, T. H., Fleming, T., Midha, R., Biernaskie, J., et al. (2020). Insights into the role and potential of Schwann cells for peripheral nerve repair from studies of development and injury. Front. Mol. Neurosci. 13:608442. doi: 10.3389/fnmol.2020.608442
Boerboom, A., Dion, V., Chariot, A., and Franzen, R. (2017). Molecular mechanisms involved in schwann cell plasticity. Front. Mol. Neurosci. 10:38. doi: 10.3389/fnmol.2017.00038
Harlow, D. E., Honce, J. M., and Miravalle, A. A. (2015). Remyelination therapy in multiple sclerosis. Front. Neurol. 6:257. doi: 10.3389/fneur.2015.00257
Laule, C., Vavasour, I. M., Kolind, S. H., Li, D. K., Traboulsee, T. L., Moore, G. R., et al. (2007). Magnetic resonance imaging of myelin. Neurotherapeutics. 4, 460–484. doi: 10.1016/j.nurt.2007.05.004
Mehndiratta, M. M., and Gulati, N. S. (2014). Central and peripheral demyelination. J. Neurosci. Rural Pract. 5, 84–86. doi: 10.4103/0976-3147.127887
Momenzadeh, S., and Jami, M. S. (2021). Remyelination in PNS and CNS: current and upcoming cellular and molecular strategies to treat disabling neuropathies. Mol. Biol. Rep. 48, 8097–8110. doi: 10.1007/s11033-021-06755-6
Nave, K. A. (2010). Myelination and support of axonal integrity by glia. Nature. 468, 244–252. doi: 10.1038/nature09614
Sock, E., and Wegner, M. (2019). Transcriptional control of myelination and remyelination. Glia. 67, 2153–2165. doi: 10.1002/glia.23636
Keywords: myelination, remyelination, demyelination, glial cells, oligodendrocytes, Schwann cells, neuron, axon
Citation: Qiu Q and Hu B (2024) Editorial: 15 years of frontiers in cellular neuroscience: myelination and remyelination processes. Front. Cell. Neurosci. 18:1463579. doi: 10.3389/fncel.2024.1463579
Received: 12 July 2024; Accepted: 26 July 2024;
Published: 09 August 2024.
Edited and reviewed by: Marie-Ève Tremblay, University of Victoria, Canada
Copyright © 2024 Qiu and Hu. This is an open-access article distributed under the terms of the Creative Commons Attribution License (CC BY). The use, distribution or reproduction in other forums is permitted, provided the original author(s) and the copyright owner(s) are credited and that the original publication in this journal is cited, in accordance with accepted academic practice. No use, distribution or reproduction is permitted which does not comply with these terms.
*Correspondence: Bo Hu, Ymh1QGhvdXN0b25tZXRob2Rpc3Qub3Jn
Disclaimer: All claims expressed in this article are solely those of the authors and do not necessarily represent those of their affiliated organizations, or those of the publisher, the editors and the reviewers. Any product that may be evaluated in this article or claim that may be made by its manufacturer is not guaranteed or endorsed by the publisher.
Research integrity at Frontiers
Learn more about the work of our research integrity team to safeguard the quality of each article we publish.