- 1Department of Neurology, West China Hospital, Sichuan University, Chengdu, China
- 2Department of Neurology, The Affiliated Hospital of Southwest Medical University, Luzhou, China
Background: The association between cytokines in peripheral blood and clinical symptoms of multiple system atrophy (MSA) has been explored in only a few studies with small sample size, and the results were obviously controversial. Otherwise, no studies have explored the diagnostic value of serum cytokines in MSA.
Methods: Serum cytokines, including interleukin-6 (IL-6), interleukin-8 (IL-8), and tumor necrosis factor alpha (TNF-α), were measured in 125 MSA patients and 98 healthy controls (HCs). Correlations of these serum cytokines with clinical variables were analyzed in MSA patients. Diagnostic value of cytokines for MSA was plotted by receiver operating curves.
Results: No significant differences were found in sex and age between the MSA group and the HCs. TNF-α in MSA patients were significantly higher than those in HCs (area under the curve (AUC) 0.768), while IL-6 and IL-8 were not. Only Hamilton Anxiety Scale (HAMA) has a positive correlation between with TNF-α in MSA patients with age and age at onset as covariates. Serum IL-6 was associated with HAMA, Hamilton Depression Scale (HAMD), the Unified MSA Rating Scale I (UMSARS I) scores, the UMSARS IV and the Instrumental Activity of Daily Living scores. However, IL-8 was not associated with all clinical variables in MSA patients. Regression analysis showed that HAMA and age at onset were significantly associated with TNF-α, and only HAMA was mild related with IL-6 levels in MSA patients.
Conclusion: Serum TNF-α and IL-6 levels in MSA patients may be associated with anxiety symptom; however, only TNF-α was shown to be a useful tool in distinguishing between MSA and HCs.
1 Introduction
Multiple system atrophy (MSA) is a rare, rapidly progressing and fatal neurodegenerative disease, characterized by a variable combination of parkinsonism, cerebellar atrophy, and autonomic dysfunction of unknown etiology (Fanciulli and Wenning, 2015). Besides these core symptoms, many other non-motor manifestations also have been observed in MSA, including sleep dysfunction, constipation, depression, and anxiety (Zhang et al., 2018; Benrud-Larson et al., 2005; Lin et al., 2020). The clinical diagnosis requires the presence of autonomic dysfunction in combination with parkinsonian-like symptoms, poorly responsive to levodopa and/or cerebellar ataxia (Poewe et al., 2022). The neuropathological feature of MSA is the glial inclusion body composed of misfolded alpha-synaptic nucleoproteins (α-syn) (Fanciulli et al., 2019). The manifestation of MSA overlap with Parkinson’s disease (PD), other types of atypical parkinsonism, and other cerebellar disorders (Poewe et al., 2022; Gilman et al., 2000). In addition, MSA also presents with numerous non-motor symptoms, including sleep disturbances, constipation, depression, and anxiety. Extensive research indicates that mood disorders are common, particularly depression and anxiety in MSA patients (Zhang et al., 2018; Benrud-Larson et al., 2005; Ghorayeb et al., 2002). These symptoms can appear even before the onset of motor symptoms, posing challenges for early diagnosis of the disease (Zhang et al., 2018). Currently, there is still no effective treatment for MSA, and no objective markers for reference either. Therefore, identifying readily accessible and objective indicators to aid in the early diagnosis of MSA is significant. Furthermore, understanding the underlying mechanisms of these indicators can provide a theoretical foundation and research direction for the development of novel targeted therapies.
Although the etiology is unclear, many studies have shown that chronic neuroinflammation may be an important pathogenesis in MSA (Vieira et al., 2015; Jellinger, 2014; Ishizawa et al., 2008). Cytokines play a crucial role in neuroinflammation, particularly in the pathogenesis of MSA. Neuroinflammation typically accompanies neuronal damage and immune responses within the nervous system, leading to the release of inflammatory cells and immune molecules. Cytokines serve as signal transducers and regulators of immune responses in this process (Tang and Le, 2016). Dysregulation of neuroinflammation feedback in MSA would trigger microglial activation into effector phenotypes, namely M1 and M2. Once the M1 phenotype is activated, it will produce pro-inflammatory and cytotoxic molecules, including tumor necrosis factor alpha (TNF-α), interleukin-6 (IL-6), interleukin-1β (IL-1β), reactive oxygen species (ROS) and excitatory amino acids, which would induce more neuronal damage and cell dysfunction (Tang and Le, 2016). However, clinical studies focusing on inflammation in MSA are still relatively lacking. Starhof et al. found that cerebrospinal fluid (CSF) C-reactive protein (CRP), TNF-α, IL-6 and IL-1β levels in MSA patients were significantly higher than those in PD patients, and these cytokines have good diagnostic value in distinguishing MSA from PD (AUC 0.77, p = 0.007, 95% CI 0.660–0.867) (Starhof et al., 2018). However, this study did not investigate the association between inflammatory factors and clinical symptoms of MSA. A few studies examined the role of blood cytokines in MSA, but the sample sizes of these studies were relatively small and the results were conflicting (Jellinger, 2014; Kim et al., 2019; Brodacki et al., 2008; Kaufman et al., 2013).
Therefore, this cross-sectional study is aiming to: (1) examine the cytokine levels in the peripheral blood of MSA patients and health controls (HCs) with a large sample size, (2) verify the reliability of these serum cytokines as biomarkers in MSA diagnosis, (3) explore the correlations of these cytokines with clinical manifestations.
2 Materials and methods
2.1 Subjects
A total of 104 patients (58 male and 46 female) with MSA were recruited from the Department of Neurology in West China Hospital (WCH), the largest hospital in southwestern China, 21 MSA patients (10 male and 11 female) from the Affiliated Hospital of Southwest Medical University were also registered in our study, based on the diagnostic consensus for MSA published in 2008 (Gilman et al., 2008), and patients with “probable MSA” were included. Notably, our patients were followed up every 6 months, and diagnoses were confirmed at the follow-up visits. Specimens were collected when patients were identified as having “probable MSA.” Exclusion criteria were as follows: (1) PD, vascular parkinsonism, Progressive Supranuclear Palsy (PSP), Cortico-Basal Degeneration (CBD), and Dementia with Lewy Bodies (DLB); (2) having a family history of autosomal dominance; (3) acute or chronic inflammatory system or immune system diseases complicated with various potential infections; (4) patients taking non-steroidal anti-inflammatory drugs or glucocorticoids orally; (5) malignant tumors, or other life-threatening diseases; (6) recent history of trauma or head surgery; (7) education period less than 3 years.
We recruited 98 gender- and age-matched HCs from outpatient settings in WCH. HCs were primarily recruited from the family members of MSA patients to ensure alignment of their lifestyle factors. Clinical neurologists assessed the current health status of the HCs and the subject with a personal or family history of neurological degeneration was excluded.
The Research Ethics Review Committee of West China Hospital of Sichuan University approved the research protocol. All participants signed an informed written consent form for the use of their clinical data for scientific purposes after adequate explanation.
2.2 Clinical assessment
Trained researchers, verified for consistency, were responsible for conducting face-to-face interviews to collect detailed demographic information and assess relevant clinical presentations. In addition, the scale evaluators and the statistical data analysts were belonging to different group to avoid subjectivity. The Unified MSA Rating Scale (UMSARS) is a commonly used semi-quantitative rating scale to assess motor symptoms in patients with MSA (Krismer et al., 2022). The total score of UMSARS scale for all items in Part I and Part II were recorded. The Part III was autonomic nerve examination, which was achieved by neurogenic orthostatic hypotension (NOH) examination. We had the patients empty the bladder, lie flat for more than 10 min, and measured blood pressure in a quiet environment. Then, patients were instructed to stand immediately, and blood pressure was measured quickly at the 1st, 3rd, 5th, and 10th minutes after standing (Pavy-Le Traon et al., 2016). NOH was diagnosed if one or more of the following blood pressure changes were registered: systolic blood pressure (SBP) drop ≥20 mmHg from baseline, diastolic blood pressure (DBP) drop ≥10 mmHg from baseline, or SBP ≤90 mmHg regardless of how much blood pressure drops (Freeman et al., 2011; Brignole et al., 2018). The Part IV of the UMSARS quantitatively assesses the degree of incapacity on a scale of 0, 1, 2, 3, and 4 (0, patients completely able to take care of themselves; 1, patients need little help in daily life; 2, patients spend most time of the day in self-care and household tasks; 3, patients need a lot of help in daily life; 4, patients who are completely relying on caregivers). The Frontal Assessment Battery (FAB) and the Montreal Cognitive Assessment (MoCA) were used to examine the cognitive function (Royall, 2001; Nasreddine et al., 2005). The Hamilton Anxiety Scale (HAMD) and the Hamilton Depression Scale (HAMA) were used to assess patients’ emotional state (Ballesteros et al., 2007; Maier et al., 1988). The Epworth sleepiness scale (ESS) as a tool to assess daytime sleepiness, with a score of 10 indicating significant daytime sleepiness (Johns, 1991). The Parkinson’s Disease Sleep Scale (PDSS-2) and the Rapid Eye Movement Sleep Behavior Disorder Screening Questionnaire (RBDSQ) were used for sleep assessment including nocturnal disturbances and REM-sleep behaviors disorder (RBD) (Trenkwalder et al., 2011; Stiasny-Kolster et al., 2007). Fatigue was assessed in the MSA patients with Fatigue Severity Scale (FSS) (Friedman et al., 2010). The Non-Motor Symptom Scale (NMSS) was used to assess the overall aspect of a patient’s non-motor symptoms (Martinez-Martin et al., 2009). The Parkinson’s Quality of Living Questionnaire (PDQ39), Activity of Daily Living (ADL), Instrumental Activity of Daily Living (IADL) were used to assess quality of life of patients with MSA from different perspectives (Zhao et al., 2021; Pashmdarfard and Azad, 2020). We asked patients to rate their satisfaction for current life (0 for dissatisfaction, 100 for very satisfied) and recorded the scores as Subjective Life Satisfaction (SLS).
2.3 Measurements of peripheral blood cytokines
The peripheral blood samples from each MSA patient and HC were collected around 7:00 am, and all samples were sent to the laboratory center within two hours for testing. This study measured serum TNF-α, IL-6 and interleukin-8 (IL-8) levels, and enzyme-linked immunosorbent assay (ELISA) assay was used according to the kit-specific manufacturer’s instructions, and all data were recorded in pg./ml.
2.4 Statistical analysis
Demographic data were compared between MSA patients and HCs by applying Student’s t test for continuous variables and chi-square tests for categorical variables. IL-6, IL-8, and TNF-α levels were non-normally distributed in both the MSA group and the HCs in Kolmogorov–Smirnov one-sample test, even after the logarithmic transformation. Therefore, we used the Mann–Whitney U test to compare these cytokine levels in MSA patients and the HCs. Spearman correlation coefficient was used to evaluate the linear relationship between the clinical variables and cytokine levels. Spearman partial correlation analysis was used to adjust the potential influencing factors. Bonferroni correction was applied to optimize for multiple tests. A stepwise forward regression model was used, with IL-6 or TNF-α as dependent variable. Clinical variables, which had a p-value <0.15 in the correlation analysis, and other factors that were found to influence cytokine levels, were included as independent variables. The variance inflation factor (VIF) was used to assessed multicollinearity among independent variables.
A binary logistic regression model was used to further explore the association between cytokines and MSA. The presence or absence of MSA was set as the dependent variable, while age, TNF-α and IL-6 were set as covariates. The area under the curve (AUC) for TNF-α in MSA diagnosis were calculated with corresponding sensitivity, specificity, and 95% confidence intervals (CI). The data of the skewed distribution was described by the median and interquartile range (IQR), and the data with the normal distribution was described by mean and standard deviation (SD). SPSS 27.0 software and GraphPad Prism 9.0 were used in statistical analyses and statistical mapping, and a two-tailed signal difference at the p ≤ 0.05 level was applied.
3 Results
Clinical/demographic characteristics of MSA patients and HCs were shown in Table 1. It showed that there was no significant difference in the sex ratio (χ2 = 2.928, p = 0.087, Table 1 and Figure 1C) and age between MSA patients and the HCs (59.82 ± 8.42 vs. 59.54 ± 6.53, p = 0.784, Table 1 and Figure 1B). The Mann–Whitney U test showed that the serum TNF-α (median 6.36, IQR 5.13–7.72) levels in MSA patients were significantly higher than those in HCs (median 4.27, IQR 0.99–5.76, p < 0.001, Table 1 and Figure 1A). The levels of IL-6 (median 1.95, IQR 1.50–2.73) were higher in MSA patients than those in HCs (median 1.84, IQR 1.25–2.79, Table 1 and Figure 1A), but the difference was not statistically significant (p = 0.406). Serum IL-8 levels in MSA patients (median 7.00, IQR 5.03–10.78) were not statistically different from those in HCs (median 6.75, IQR 5.07–10.80, p = 0.664, Table 1 and Figure 1A). All these results were verified through Bonferroni multiple correction.
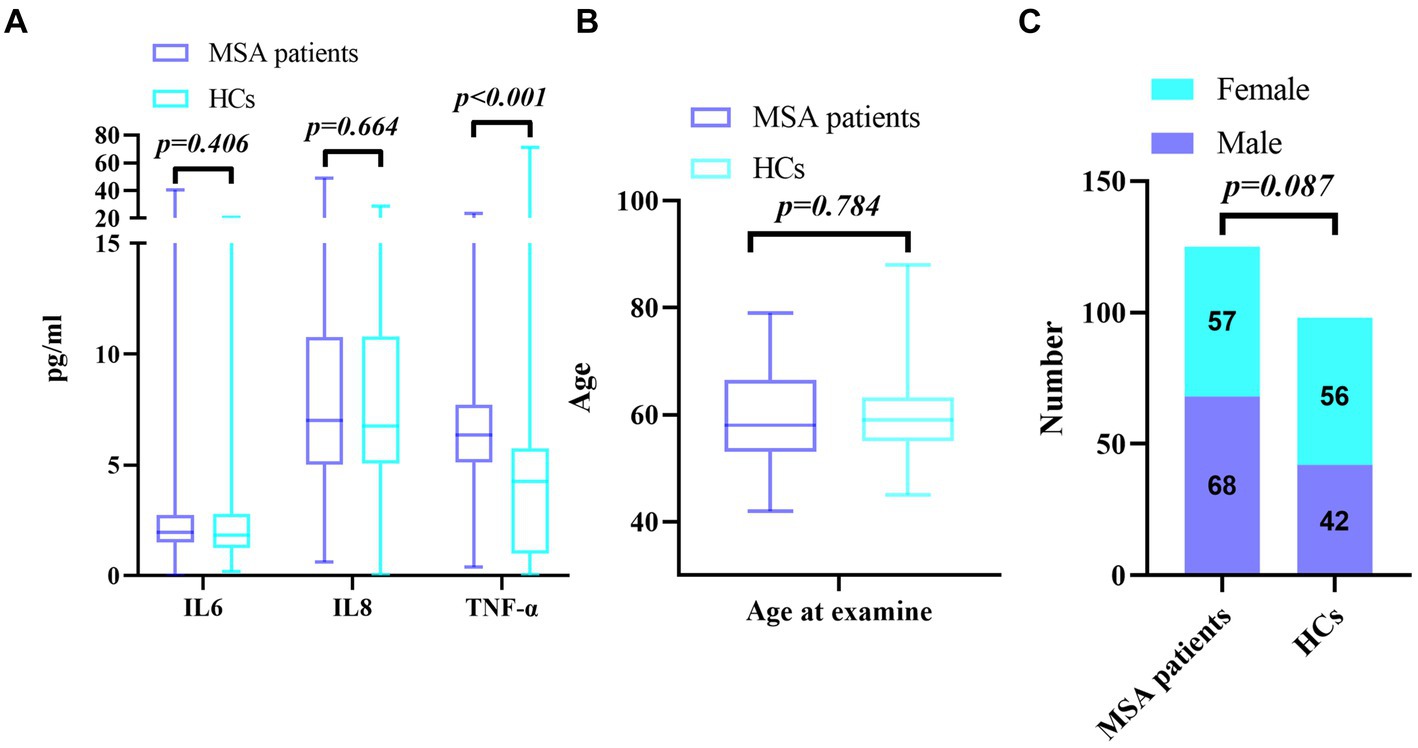
Figure 1. (A) Comparison of serum cytokine levels between MSA and HCs; (B) Comparison of age between MSA and HCs; (C) Comparison of gender distribution between MSA and HCs.
Spearman correlation analysis showed no associations between serum IL-8 levels and clinical/demographic variables (Table 2). Several clinical/demographic variables in MSA patients were found to be correlated with serum IL-6 levels, including HAMA (r = 0.257, p = 0.004), HAMD (r = 0.220, p = 0.014), UMSARS I (r = 0.211, p = 0.018), UMSARS IV (r = 0.280, p = 0.002) and IADL scores (r = −0.230, p = 0.010), but not with other remaining variables. Serum TNF-α levels were correlated with HAMA scores (r = 0.217, p = 0.023), Fatigue (r = 0.176, p = 0.049), NOH (r = −0.194, p = 0.030), age (r = 0.259, p = 0.004), and age at onset (r = 0.270, p = 0.002), but were not related to other clinical variables, including MoCA, FAB, NMSS, UMSARS I, UMSARS II, UMSARS IV, HAMD, PDQ-39, PDSS-2, ESS, RBD, ADL, IADL, and SLS (Table 2). After adjusting for age and age at onset, Spearman partial analysis showed that serum TNF-α levels were only significantly associated with HAMA (r = 0.340, p < 0.001, Table 2).
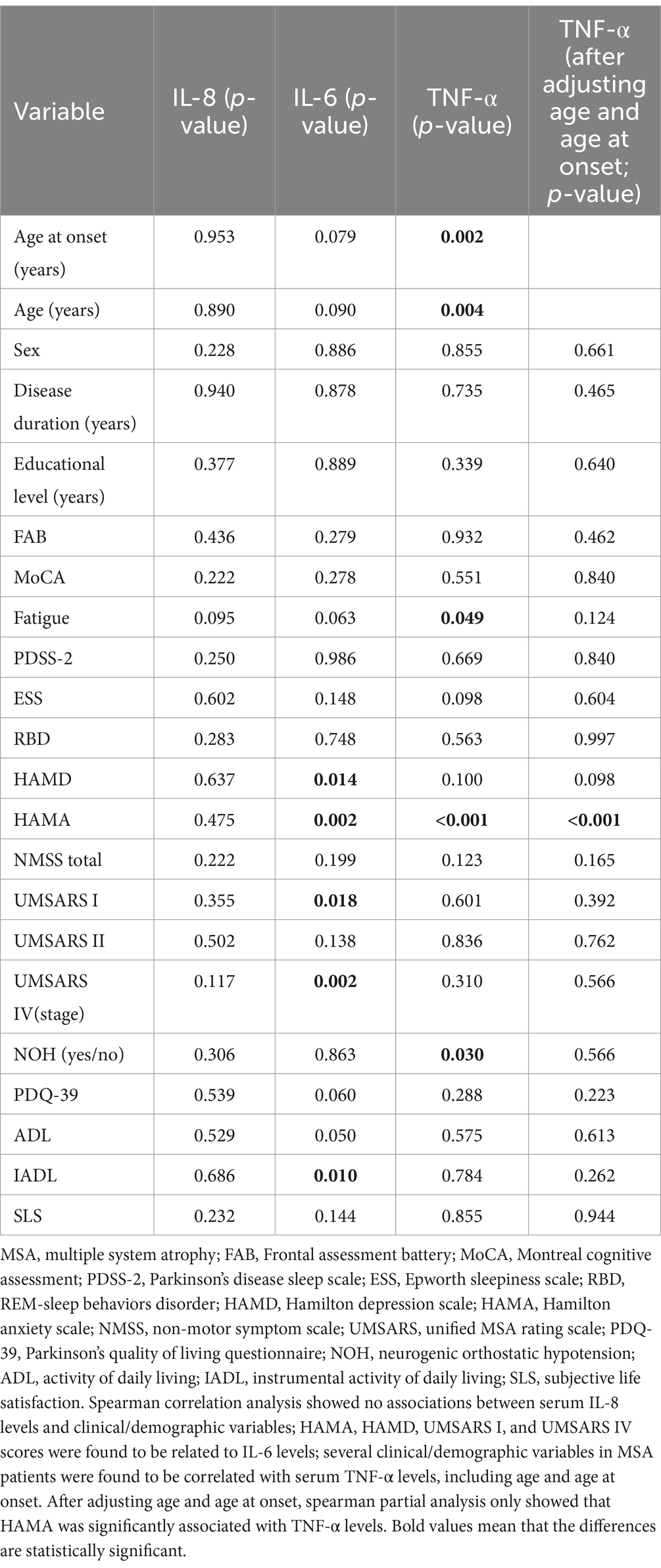
Table 2. Clinical/demographic variables of MSA patients and the associations with inflammatory factor levels.
In the stepwise forward regression analysis, the age, age at onset, UMSARS I, UMSARS II, UMSARS IV, PDQ-39, Fatigue, ESS, HAMD, HAMA, ADL, IADL and SLS were used as independent variables, and IL-6 was set as dependent variables; the results showed that only HAMA was related to serum IL-6 levels ([F = 8.687, p = 0.004, R2 = 0.066, Adjusted R2 = 0.058]). With age, age at onset, Fatigue, HAMA, HAMD, ESS, NMSS and NOH as independent variables, we found that TNF-α was associated with HAMA and age at onset (F = 13.535, p < 0.001, R2 = 0.182, Adjusted R2 = 0.168). There was no multicollinearity among all included independent variables (all VIF < 1.0).
The binary logistic regression model showed that only TNF-α was positively associated with the presence or absence of MSA (OR 1.237, p < 0.001). The Receiver operating characteristic curve (ROC) curve of TNF-α for the diagnosis of MSA showed AUC was 0.768 (p < 0.01, 95% CI 0.705–0.832). The critical value of TNF-α for the diagnosis of MSA was 5.125 pg./mL, with a diagnostic sensitivity of 75.2% and a specificity of 68.4% (Figure 2).
4 Discussion
In the present study, we found a pronounced increase in the serum levels of TNF-α in MSA patients compared with HCs. Furthermore, the TNF-α serum levels in MSA patients were found to be associated with HAMA. In addition, ROC curve analysis indicated that TNF-α could discriminate MSA patients from HCs, as a screening diagnosis biomarker. To the best of our knowledge, this is the first study to investigate the diagnostic value of serum cytokines in MSA patients, with a large sample size.
4.1 Serum levels of TNF-α was elevated in MSA patients
TNF-α, as a main pro-inflammatory cytokine, is one of the key regulators of the inflammatory response and is mainly synthesized by activated microglia in the central nervous system (CNS) during neuroinflammatory (Lieberman et al., 1989). Studies have found that TNF-α released by microglia plays a major role in angiotensin induced dopaminergic cell and oligodendrocyte death through multiple mechanisms (Probst-Cousin et al., 1998; Borrajo et al., 2014; Ndayisaba et al., 2019). A previous genetic study showed that TNF-α may have a toxic role in the pathophysiological process of MSA (Nishimura et al., 2005). Lenalidomide can reduce the proliferation of astrocyte, microglia and the level of α-syn by inhibiting the production of TNF-α in MSA mice, and the effect of lenalidomide can partially improve the motor dysfunction of MSA mice (Valera et al., 2017). These findings support TNF-α as an important biomarker in MSA. Our results showed that MSA patients had higher TNF-α than HCs, which is consistent with the results of two previous studies (Brodacki et al., 2008; Kaufman et al., 2013). One previous study also found that the higher TNF-α levels were associated with mild motor symptoms and early stage of disease (Kaufman et al., 2013). However, another study found no association between TNF-α and MSA, probably due to the long disease duration (3.4 ± 1.7 years) in the recruited MSA patients (Kim et al., 2019). Studies have shown that neuroinflammation could be an early event in the pathogenic mechanism of MSA, and in the early stage of neurodegeneration, increased cytokine levels may reflect a compensatory physiological response to neuronal injury with potential neuroprotective purposes, whereas in the middle or late stage of the disease, this protective mechanism may be markedly attenuated (Müller et al., 1998; Ahmed et al., 2012; Stefanova et al., 2007; Yamasaki et al., 2017). In the present study, the disease duration was 1.74 ± 1.31 years, indicating that our patients were mostly in the early stage of MSA. We found that the TNF-α levels were significantly higher than those in HCs, and this result can be partially explained by the relatively short disease duration in our MSA patients. Furthermore, in studies using CSF samples, TNF-α were also found to be significantly increased in MSA patients compared to PD or normal controls, and CSF TNF-α was even considered an important cytokine in MSA (Starhof et al., 2018; Compta et al., 2019; Fellner and Wenning, 2019). Although this study used serum samples, it was found that serum TNF-α can directly cause blood–brain barrier dysfunction by reducing extracellular resistance and cell polarity to induce or aggravate neurodegenerative diseases (Sweeney et al., 2019; Yang et al., 2022; O’Carroll et al., 2015; Murdaca et al., 2022). Therefore, our study reported that elevated TNF-α levels in serum may have potential as a biomarker in MSA, especially in the early stage of disease.
In this study, we did not find any significant differences in IL-6 levels between MSA patients and HCs, and this finding was not consistent with other studies which found higher IL-6 levels in MSA (Brodacki et al., 2008; Kaufman et al., 2013; Hall et al., 2018). However, these previous studies also had found that IL-6 levels were also increased in PD patients compared to HCs, while TNF-α levels were not increased, so the author emphasized that the observed increase of IL-6 levels may not be specific for MSA (Kaufman et al., 2013; Hall et al., 2018). Different from the above-mentioned studies, we collected serum samples after an overnight fast to avoid the influence of some pro-/anti-inflammatory diets on circulating cytokines (Emerson et al., 2017; Sun et al., 2022). In addition, patients treated with NSAIDs were included in one previous study, but we excluded this interference in our study (Brodacki et al., 2008). Notably, that the sample size in these two studies were relatively small, with no more than 20 cases. We did not find any differences in serum IL-8 levels between MSA patients and HCs. Till now, there were no studies on IL-8 levels in serum of MSA patients. In CSF, one study found that the CSF IL-8 levels in MSA patients were higher than those in the HCs, but another study reported that the CSF IL-8 levels in MSA patients were significantly lower than those in the control group (Yamasaki et al., 2017; Hall et al., 2018). These findings suggested that CSF IL-8 levels may fluctuate dramatically in MSA patients. In addition, the different inclusion and exclusion criteria for these studies may partially explain the inconsistence. In summary, our findings indicated that serum IL-6 and IL-8 may have low value in diagnosis of MSA.
4.2 Diagnostic value of serum TNF-α for MSA
To our knowledge, this is the first study to investigate the diagnostic value of serum cytokines for MSA. As confirmed by ROC curves, TNF-α levels exhibited high values of AUC, and the sensitivities and specificities of TNF-α were also significant, indicating that TNF-α may be a reliable biomarker for the diagnosis of MSA. MSA patients typically exhibit signs of immune system activation and neuroinflammation. Elevated levels of TNF-α may reflect this inflammatory state (Stefanova et al., 2007). Moreover, TNF-α not only plays a crucial role in inflammatory responses but may also impact neuroprotective mechanisms and neuronal function, thereby also relating to the clinical symptoms of MSA (Stefanova and Wenning, 2016). Thus, TNF-α is involved in the pathogenesis of MSA. It is worth noting that studies have shown that CSF levels of TNF-α in patients with MSA were significantly higher than those in PD or HCs, regardless of the duration and subtype (MSA-P or MSA-C) (Starhof et al., 2018; Compta et al., 2019). CRP combined with a cytokine set (including TNF-α) in CSF was also found to have the best diagnostic value for discriminating PD (AUC = 0.77, p = 0.007, 95% CI 0.660–0.867) (Starhof et al., 2018). Therefore, in the future studies, combination of serum TNF-α with other inflammation factors may have higher diagnostic value in MSA.
4.3 Serum levels of TNF-α and Il-6 were associated with the HAMA scores in MSA patients
Anxiety, as one of the most common emotional disorders in MSA patients, usually leads to multiple hospital admissions because of excessive worry about their disease, which increases the disease burden, occupies the medical resources, and affects the quality of life of patients (Schrag et al., 2010). Our previous studies found that anxiety in MSA patients was related to uncontrollable factors, including gender (female), longer disease duration and disease severity (Zhang et al., 2018). The present study found that elevated TNF-α levels was a risk factor for anxiety mood in MSA patients even after adjusting age and age at onset (r = 0.340, p < 0.001). Stepwise forward regression analysis also confirmed a positive association of TNF-α with HAMA score and age at onset. These findings illustrate the possible involvement of TNF-α in the progression of anxiety symptoms in MSA patients. A previous study did not found any significant correlations between cytokines and non-motor symptoms in MSA patients, but this study only included 14 MSA patients (Kaufman et al., 2013). In addition, anxiety has been found to be significantly positively correlated with TNF-α levels in related studies focusing on PD patients (Wang et al., 2016; Lindqvist et al., 2012; Menza et al., 2010). Furthermore, severe anxiety and depression can also induce immune activation, leading to elevated levels of cytokines, including TNF-α, and the use of immunosuppressants such as interferon-α can alleviate these emotional disorders (Anisman, 2009). Another study included 70 patients with generalized anxiety disorder (GAD), and found that GAD patients had significantly elevated serum cytokines, including TNF-α, but serum TNF-α levels were significantly reduced after successful treatment of anxiety disorders (Hoge et al., 2018). These findings suggest that chronic inflammation may affect the anxiety mood in MSA patients. Our study also found a positive correlation between serum TNF-α levels and age at onset in MSA patients, which is also consistent with previous findings that serum TNF-α levels were related to aging and significantly higher in older adults than in younger adults (Nozu et al., 2018; Chen et al., 2023; Wyczalkowska-Tomasik et al., 2016).
Although correlation analysis found that IL-6 was linearly associated with multiple clinical variables, stepwise forward regression analysis found only HAMA had positive association with IL-6 level, which suggested that IL-6 had some effect on anxiety in MSA patients. Previous studies also have found that IL-6 was also associated with many non-motor symptoms in PD patients including anxiety and depression (Selikhova et al., 2002; Veselý et al., 2018). Some studies have found that serum IL-6 level may be related to anxiety symptoms in other diseases, and the use of IL-6 antagonist can significantly improve these anxiety symptoms (Tiosano et al., 2020; Zou et al., 2020; Yang et al., 2017). These studies also seem to illustrate that IL-6 may be involved in the pathogenesis of anxiety symptoms. From a mechanistic perspective, TNF-α and IL-6 are primary inflammatory mediators in the immune system (Connolly et al., 2021). Elevated levels of TNF-α and IL-6 may inhibit the synthesis and release of neurotransmitters such as serotonin and dopamine, thereby leading to mood dysregulation disorders like anxiety. Inflammatory responses can alter the expression and signaling of neurotransmitter receptors such as NMDA receptors and γ-aminobutyric acid (GABA) receptors, influencing mood regulation and cognitive function. TNF-α and IL-6 may contribute to the onset of anxiety symptoms in MSA patients through these mechanisms (Khandaker et al., 2015; Pariante, 2017). Therefore, suitable clinical intervention on the anxiety may have potential benefits in MSA by monitoring the serum TNF-α and IL-6 levels.
5 Limitations
There are several limitations in this study. (1) Due to the cross-sectional design of this study, we were unable to further reveal the causal relationship between TNF-α and IL-6 levels and anxiety symptoms in MSA patients. (2) All diagnoses were based on clinical diagnosis. Although we ensured the diagnosis of MSA by following the patients for at least six months, the possibility of misdiagnosis still existed due to the lack of neuropathological basis. (3) The participants were only recruited from two tertiary hospitals in China, and further results of a multicenter study will be needed in the future.
6 Conclusion
In summary, this study found that serum TNF-α levels was increased in MSA patients compared to those in HCs. Serum TNF-α may serve as a biomarker in diagnosis of MSA. In addition, the elevated TNF-α and IL-6 levels were associated with anxiety symptoms in MSA patients, and further studies with longitudinal and prospective designs from multiple centers and ethnic populations are required to verify these preliminary findings.
Data availability statement
The raw data supporting the conclusions of this article will be made available by the authors, without undue reservation.
Ethics statement
The studies involving humans were approved by the Research Ethics Review Committee of West China Hospital of Sichuan University approved the research protocol. The studies were conducted in accordance with the local legislation and institutional requirements. The participants provided their written informed consent to participate in this study.
Author contributions
XC: Data curation, Methodology, Writing – original draft. SC: Data curation, Writing – original draft. XL: Data curation, Methodology, Writing – original draft. JF: Data curation, Methodology, Writing – original draft. JY: Data curation, Methodology, Writing – original draft. RO: Data curation, Methodology, Writing – original draft. LZ: Methodology, Writing – original draft. QW: Data curation, Writing – original draft. XG: Methodology, Writing – original draft. HS: Conceptualization, Resources, Supervision, Writing – review & editing.
Funding
The author(s) declare that financial support was received for the research, authorship, and/or publication of this article. This study was supported by the Clinical Research Incubation Project of West China Hospital of Sichuan University (Grant No. 20HXFH035), the grant from the Cadres Health Care Project in Sichuan Province (Grant No. 2023-111), and grant from the Science and Technology Bureau Fund of Sichuan Province of China (No. 2023YFQ0098).
Acknowledgments
We are greatly indebted to the all subjects for their participation in the study.
Conflict of interest
The authors declare that the research was conducted in the absence of any commercial or financial relationships that could be construed as a potential conflict of interest.
Publisher’s note
All claims expressed in this article are solely those of the authors and do not necessarily represent those of their affiliated organizations, or those of the publisher, the editors and the reviewers. Any product that may be evaluated in this article, or claim that may be made by its manufacturer, is not guaranteed or endorsed by the publisher.
Supplementary material
The Supplementary material for this article can be found online at: https://www.frontiersin.org/articles/10.3389/fncel.2024.1459884/full#supplementary-material
References
Ahmed, Z., Asi, Y. T., Sailer, A., Lees, A. J., Houlden, H., Revesz, T., et al. (2012). The neuropathology, pathophysiology and genetics of multiple system atrophy. Neuropathol. Appl. Neurobiol. 38, 4–24. doi: 10.1111/j.1365-2990.2011.01234.x
Anisman, H. (2009). Cascading effects of stressors and inflammatory immune system activation: implications for major depressive disorder. J. Psychiatry Neurosci. 34, 4–20
Ballesteros, J., Bobes, J., Bulbena, A., Luque, A., Dal-Ré, R., Ibarra, N., et al. (2007). Sensitivity to change, discriminative performance, and cutoff criteria to define remission for embedded short scales of the Hamilton depression rating scale (HAMD). J. Affect. Disord. 102, 93–99. doi: 10.1016/j.jad.2006.12.015
Benrud-Larson, L. M., Sandroni, P., Schrag, A., and Low, P. A. (2005). Depressive symptoms and life satisfaction in patients with multiple system atrophy. Mov. Disord. 20, 951–957. doi: 10.1002/mds.20450
Borrajo, A., Rodriguez-Perez, A. I., Diaz-Ruiz, C., Guerra, M. J., and Labandeira-Garcia, J. L. (2014). Microglial TNF-α mediates enhancement of dopaminergic degeneration by brain angiotensin. Glia 62, 145–157. doi: 10.1002/glia.22595
Brignole, M., Moya, A., de Lange, F. J., Deharo, J. C., Elliott, P. M., Fanciulli, A., et al. (2018). 2018 ESC guidelines for the diagnosis and management of syncope. Eur. Heart J. 39, 1883–1948. doi: 10.1093/eurheartj/ehy037
Brodacki, B., Staszewski, J., Toczyłowska, B., Kozłowska, E., Drela, N., Chalimoniuk, M., et al. (2008). Serum interleukin (IL-2, IL-10, IL-6, IL-4), TNFalpha, and INFgamma concentrations are elevated in patients with atypical and idiopathic parkinsonism. Neurosci. Lett. 441, 158–162. doi: 10.1016/j.neulet.2008.06.040
Chen, P., Chen, F., Lei, J., and Zhou, B. (2023). Pomegranate polyphenol punicalagin improves learning memory deficits, redox homeostasis, and neuroinflammation in aging mice. Phytother. Res. 37, 3655–3674. doi: 10.1002/ptr.7848
Compta, Y., Dias, S. P., Giraldo, D. M., Pérez-Soriano, A., Muñoz, E., Saura, J., et al. (2019). Cerebrospinal fluid cytokines in multiple system atrophy: a cross-sectional Catalan MSA registry study. Parkinsonism Relat. Disord. 65, 3–12. doi: 10.1016/j.parkreldis.2019.05.040
Connolly, M. G., Potter, O. V., Sexton, A. R., and Kohman, R. A. (2021). Effects of toll-like receptor 4 inhibition on spatial memory and cell proliferation in male and female adult and aged mice. Brain Behav. Immun. 97, 383–393. doi: 10.1016/j.bbi.2021.06.008
Emerson, S. R., Kurti, S. P., Harms, C. A., Haub, M. D., Melgarejo, T., Logan, C., et al. (2017). Magnitude and timing of the postprandial inflammatory response to a high-fat meal in healthy adults: a systematic review. Adv. Nutr. 8, 213–225. doi: 10.3945/an.116.014431
Fanciulli, A., Stankovic, I., Krismer, F., Seppi, K., Levin, J., and Wenning, G. K. (2019). Multiple system atrophy. Int. Rev. Neurobiol. 149, 137–192. doi: 10.1016/bs.irn.2019.10.004
Fanciulli, A., and Wenning, G. K. (2015). Multiple-system atrophy. N. Engl. J. Med. 372, 249–263. doi: 10.1056/NEJMra1311488
Fellner, L., and Wenning, G. K. (2019). Multiple system atrophy - are cerebrospinal fluid cytokines reliable potential diagnostic marker? Parkinsonism Relat. Disord. 65, 1–2. doi: 10.1016/j.parkreldis.2019.09.019
Freeman, R., Wieling, W., Axelrod, F. B., Benditt, D. G., Benarroch, E., Biaggioni, I., et al. (2011). Consensus statement on the definition of orthostatic hypotension, neurally mediated syncope and the postural tachycardia syndrome. Clin. Auton. Res. 21, 69–72. doi: 10.1007/s10286-011-0119-5
Friedman, J. H., Alves, G., Hagell, P., Marinus, J., Marsh, L., Martinez-Martin, P., et al. (2010). Fatigue rating scales critique and recommendations by the movement disorders society task force on rating scales for Parkinson's disease. Mov. Disord. 25, 805–822. doi: 10.1002/mds.22989
Ghorayeb, I., Yekhlef, F., Chrysostome, V., Balestre, E., Bioulac, B., and Tison, F. (2002). Sleep disorders and their determinants in multiple system atrophy. J. Neurol. Neurosurg. Psychiatry 72, 798–800. doi: 10.1136/jnnp.72.6.798
Gilman, S., Little, R., Johanns, J., Heumann, M., Kluin, K. J., Junck, L., et al. (2000). Evolution of sporadic olivopontocerebellar atrophy into multiple system atrophy. Neurology 55, 527–532. doi: 10.1212/WNL.55.4.527
Gilman, S., Wenning, G. K., Low, P. A., Brooks, D. J., Mathias, C. J., Trojanowski, J. Q., et al. (2008). Second consensus statement on the diagnosis of multiple system atrophy. Neurology 71, 670–676. doi: 10.1212/01.wnl.0000324625.00404.15
Hall, S., Janelidze, S., Surova, Y., Widner, H., Zetterberg, H., and Hansson, O. (2018). Cerebrospinal fluid concentrations of inflammatory markers in Parkinson's disease and atypical parkinsonian disorders. Sci. Rep. 8:13276. doi: 10.1038/s41598-018-31517-z
Hoge, E. A., Bui, E., Palitz, S. A., Schwarz, N. R., Owens, M. E., Johnston, J. M., et al. (2018). The effect of mindfulness meditation training on biological acute stress responses in generalized anxiety disorder. Psychiatry Res. 262, 328–332. doi: 10.1016/j.psychres.2017.01.006
Ishizawa, K., Komori, T., Arai, N., Mizutani, T., and Hirose, T. (2008). Glial cytoplasmic inclusions and tissue injury in multiple system atrophy: a quantitative study in white matter (olivopontocerebellar system) and gray matter (nigrostriatal system). Neuropathology 28, 249–257. doi: 10.1111/j.1440-1789.2007.00855.x
Jellinger, K. A. (2014). Neuropathology of multiple system atrophy: new thoughts about pathogenesis. Mov. Disord. 29, 1720–1741. doi: 10.1002/mds.26052
Johns, M. W. (1991). A new method for measuring daytime sleepiness: the Epworth sleepiness scale. Sleep 14, 540–545. doi: 10.1093/sleep/14.6.540
Kaufman, E., Hall, S., Surova, Y., Widner, H., Hansson, O., and Lindqvist, D. (2013). Proinflammatory cytokines are elevated in serum of patients with multiple system atrophy. PLoS One 8:e62354. doi: 10.1371/journal.pone.0062354
Khandaker, G. M., Cousins, L., Deakin, J., Lennox, B. R., Yolken, R., and Jones, P. B. (2015). Inflammation and immunity in schizophrenia: implications for pathophysiology and treatment. Lancet Psychiatry 2, 258–270. doi: 10.1016/S2215-0366(14)00122-9
Kim, R., Kim, H. J., Kim, A., Jang, M., Kim, A., Kim, Y., et al. (2019). Does peripheral inflammation contribute to multiple system atrophy? Parkinsonism Relat. Disord. 64, 340–341. doi: 10.1016/j.parkreldis.2019.03.020
Krismer, F., Seppi, K., Jönsson, L., Åström, D. O., Berger, A. K., Simonsen, J., et al. (2022). Sensitivity to change and patient-centricity of the unified multiple system atrophy rating scale items: a data-driven analysis. Mov. Disord. 37, 1425–1431. doi: 10.1002/mds.28993
Lieberman, A. P., Pitha, P. M., Shin, H. S., and Shin, M. L. (1989). Production of tumor necrosis factor and other cytokines by astrocytes stimulated with lipopolysaccharide or a neurotropic virus. Proc. Natl. Acad. Sci. USA 86, 6348–6352. doi: 10.1073/pnas.86.16.6348
Lin, J. Y., Zhang, L. Y., Cao, B., Wei, Q. Q., Ou, R. W., Hou, Y. B., et al. (2020). Sleep-related symptoms in multiple system atrophy: determinants and impact on disease severity. Chin. Med. J. 134, 690–698. doi: 10.1097/CM9.0000000000001211
Lindqvist, D., Kaufman, E., Brundin, L., Hall, S., Surova, Y., and Hansson, O. (2012). Non-motor symptoms in patients with Parkinson's disease - correlations with inflammatory cytokines in serum. PLoS One 7:e47387. doi: 10.1371/journal.pone.0047387
Maier, W., Buller, R., Philipp, M., and Heuser, I. (1988). The Hamilton anxiety scale: reliability, validity and sensitivity to change in anxiety and depressive disorders. J. Affect. Disord. 14, 61–68. doi: 10.1016/0165-0327(88)90072-9
Martinez-Martin, P., Rodriguez-Blazquez, C., Abe, K., Bhattacharyya, K. B., Bloem, B. R., Carod-Artal, F. J., et al. (2009). International study on the psychometric attributes of the non-motor symptoms scale in Parkinson disease. Neurology 73, 1584–1591. doi: 10.1212/WNL.0b013e3181c0d416
Menza, M., Dobkin, R. D., Marin, H., Mark, M. H., Gara, M., Bienfait, K., et al. (2010). The role of inflammatory cytokines in cognition and other non-motor symptoms of Parkinson's disease. Psychosomatics 51, 474–479. doi: 10.1176/appi.psy.51.6.474
Müller, T., Blum-Degen, D., Przuntek, H., and Kuhn, W. (1998). Interleukin-6 levels in cerebrospinal fluid inversely correlate to severity of Parkinson's disease. Acta Neurol. Scand. 98, 142–144. doi: 10.1111/j.1600-0404.1998.tb01736.x
Murdaca, G., Paladin, F., Casciaro, M., Vicario, C. M., Gangemi, S., and Martino, G. (2022). Neuro-Inflammaging and psychopathological distress. Biomedicines 10:92133. doi: 10.3390/biomedicines10092133
Nasreddine, Z. S., Phillips, N. A., Bédirian, V., Charbonneau, S., Whitehead, V., Collin, I., et al. (2005). The Montreal cognitive assessment, MoCA: a brief screening tool for mild cognitive impairment. J. Am. Geriatr. Soc. 53, 695–699. doi: 10.1111/j.1532-5415.2005.53221.x
Ndayisaba, A., Jellinger, K., Berger, T., and Wenning, G. K. (2019). TNFα inhibitors as targets for protective therapies in MSA: a viewpoint. J. Neuroinflammation 16:80. doi: 10.1186/s12974-019-1477-5
Nishimura, M., Kuno, S., Kaji, R., and Kawakami, H. (2005). Influence of a tumor necrosis factor gene polymorphism in Japanese patients with multiple system atrophy. Neurosci. Lett. 374, 218–221. doi: 10.1016/j.neulet.2004.10.056
Nozu, A., Hamano, S., Tomokiyo, A., Hasegawa, D., Sugii, H., Yoshida, S., et al. (2018). Senescence and odontoblastic differentiation of dental pulp cells. J. Cell. Physiol. 234, 849–859. doi: 10.1002/jcp.26905
O’Carroll, S. J., Kho, D. T., Wiltshire, R., Nelson, V., Rotimi, O., Johnson, R., et al. (2015). Pro-inflammatory TNFα and IL-1β differentially regulate the inflammatory phenotype of brain microvascular endothelial cells. J. Neuroinflammation 12:131. doi: 10.1186/s12974-015-0346-0
Pariante, C. M. (2017). Why are depressed patients inflamed? A reflection on 20 years of research on depression, glucocorticoid resistance and inflammation. Eur. Neuropsychopharmacol. 27, 554–559. doi: 10.1016/j.euroneuro.2017.04.001
Pashmdarfard, M., and Azad, A. (2020). Assessment tools to evaluate activities of daily living (ADL) and instrumental activities of daily living (IADL) in older adults: a systematic review. Med. J. Islam Repub. Iran 34:33.
Pavy-Le Traon, A., Piedvache, A., Perez-Lloret, S., Calandra-Buonaura, G., Cochen-De Cock, V., Colosimo, C., et al. (2016). New insights into orthostatic hypotension in multiple system atrophy: a European multicentre cohort study. J. Neurol. Neurosurg. Psychiatry 87, 554–561. doi: 10.1136/jnnp-2014-309999
Poewe, W., Stankovic, I., Halliday, G., Meissner, W. G., Wenning, G. K., Pellecchia, M. T., et al. (2022). Multiple system atrophy. Nat. Rev. Dis. Primers 8:56. doi: 10.1038/s41572-022-00382-6
Probst-Cousin, S., Rickert, C. H., Schmid, K. W., and Gullota, F. (1998). Cell death mechanisms in multiple system atrophy. J. Neuropathol. Exp. Neurol. 57, 814–821. doi: 10.1097/00005072-199809000-00002
Royall, D. R. (2001). The FAB: a frontal assessment battery at bedside. Neurology 57:565. doi: 10.1212/WNL.57.3.565
Schrag, A., Sheikh, S., Quinn, N. P., Lees, A. J., Selai, C., Mathias, C., et al. (2010). A comparison of depression, anxiety, and health status in patients with progressive supranuclear palsy and multiple system atrophy. Mov. Disord. 25, 1077–1081. doi: 10.1002/mds.22794
Selikhova, M. V., Kushlinskii, N. E., Lyubimova, N. V., and Gusev, E. I. (2002). Impaired production of plasma interleukin-6 in patients with Parkinson's disease. Bull. Exp. Biol. Med. 133, 81–83. doi: 10.1023/A:1015120930920
Starhof, C., Winge, K., Heegaard, N. H. H., Skogstrand, K., Friis, S., and Hejl, A. (2018). Cerebrospinal fluid pro-inflammatory cytokines differentiate parkinsonian syndromes. J. Neuroinflammation 15:305. doi: 10.1186/s12974-018-1339-6
Stefanova, N., Reindl, M., Neumann, M., Kahle, P. J., Poewe, W., and Wenning, G. K. (2007). Microglial activation mediates neurodegeneration related to oligodendroglial alpha-synucleinopathy: implications for multiple system atrophy. Mov. Disord. 22, 2196–2203. doi: 10.1002/mds.21671
Stefanova, N., and Wenning, G. K. (2016). Review: multiple system atrophy: emerging targets for interventional therapies. Neuropathol. Appl. Neurobiol. 42, 20–32. doi: 10.1111/nan.12304
Stiasny-Kolster, K., Mayer, G., Schäfer, S., Möller, J. C., Heinzel-Gutenbrunner, M., and Oertel, W. H. (2007). The REM sleep behavior disorder screening questionnaire—a new diagnostic instrument. Mov. Disord. 22, 2386–2393. doi: 10.1002/mds.21740
Sun, M., Wang, L., Guo, Y., Yan, S., Li, J., Wang, X., et al. (2022). The association among inflammatory diet, glycohemoglobin, and cognitive function impairment in the elderly: based on the NHANES 2011-2014. J. Alzheimers Dis. 87, 1713–1723. doi: 10.3233/JAD-215688
Sweeney, M. D., Zhao, Z., Montagne, A., Nelson, A. R., and Zlokovic, B. V. (2019). Blood-brain barrier: from physiology to disease and back. Physiol. Rev. 99, 21–78. doi: 10.1152/physrev.00050.2017
Tang, Y., and Le, W. (2016). Differential roles of M1 and M2 microglia in neurodegenerative diseases. Mol. Neurobiol. 53, 1181–1194. doi: 10.1007/s12035-014-9070-5
Tiosano, S., Yavne, Y., Watad, A., Langevitz, P., Lidar, M., Feld, J., et al. (2020). The impact of tocilizumab on anxiety and depression in patients with rheumatoid arthritis. Eur. J. Clin. Investig. 50:e13268. doi: 10.1111/eci.13268
Trenkwalder, C., Kohnen, R., Högl, B., Metta, V., Sixel-Döring, F., Frauscher, B., et al. (2011). Parkinson's disease sleep scale—validation of the revised version PDSS‐2. Mov. Disord. 26, 644–652. doi: 10.1002/mds.23476
Valera, E., Spencer, B., Fields, J. A., Trinh, I., Adame, A., Mante, M., et al. (2017). Combination of alpha-synuclein immunotherapy with anti-inflammatory treatment in a transgenic mouse model of multiple system atrophy. Acta Neuropathol. Commun. 5:2. doi: 10.1186/s40478-016-0409-1
Veselý, B., Dufek, M., Thon, V., Brozman, M., Királová, S., Halászová, T., et al. (2018). Interleukin 6 and complement serum level study in Parkinson's disease. J. Neural Transm 125, 875–881. doi: 10.1007/s00702-018-1857-5
Vieira, B. D., de Felicio, R., LFG, A., Costa, J. H., Ortega, R., Vieira, B. D., et al. (2015). Neuroinflammation in multiple system atrophy: response to and cause of α-Synuclein aggregation. Front. Cell. Neurosci. 9:437. doi: 10.3389/fncel.2015.00437
Wang, X. M., Zhang, Y. G., Li, A. L., Long, Z. H., Wang, D., Li, X. X., et al. (2016). Relationship between levels of inflammatory cytokines in the peripheral blood and the severity of depression and anxiety in patients with Parkinson's disease. Eur. Rev. Med. Pharmacol. Sci. 20, 3853–3856
Wyczalkowska-Tomasik, A., Czarkowska-Paczek, B., Zielenkiewicz, M., and Paczek, L. (2016). Inflammatory markers change with age, but do not fall beyond reported Normal ranges. Arch. Immunol. Ther. Exp. 64, 249–254. doi: 10.1007/s00005-015-0357-7
Yamasaki, R., Yamaguchi, H., Matsushita, T., Fujii, T., Hiwatashi, A., and Kira, J. I. (2017). Early strong intrathecal inflammation in cerebellar type multiple system atrophy by cerebrospinal fluid cytokine/chemokine profiles: a case control study. J. Neuroinflammation 14:89. doi: 10.1186/s12974-017-0863-0
Yang, C. J., Liu, D., Xu, Z. S., Shi, S. X., and du, Y. J. (2017). The pro-inflammatory cytokines, salivary cortisol and alpha-amylase are associated with generalized anxiety disorder (GAD) in patients with asthma. Neurosci. Lett. 656, 15–21. doi: 10.1016/j.neulet.2017.07.021
Yang, J., Ran, M., Li, H., Lin, Y., Ma, K., Yang, Y., et al. (2022). New insight into neurological degeneration: inflammatory cytokines and blood-brain barrier. Front. Mol. Neurosci. 15:1013933. doi: 10.3389/fnmol.2022.1013933
Zhang, L. Y., Cao, B., Zou, Y. T., Wei, Q. Q., Ou, R. W., Zhao, B., et al. (2018). Depression and anxiety in multiple system atrophy. Acta Neurol. Scand. 137, 33–37. doi: 10.1111/ane.12804
Zhao, N., Yang, Y., Zhang, L., Zhang, Q., Balbuena, L., Ungvari, G. S., et al. (2021). Quality of life in Parkinson's disease: a systematic review and meta-analysis of comparative studies. CNS Neurosci. Ther. 27, 270–279. doi: 10.1111/cns.13549
Keywords: multiple system atrophy, TNF-α, IL-6, diagnostic value, association analysis
Citation: Chen X, Chen S, Lai X, Fu J, Yang J, Ou R, Zhang L, Wei Q, Guo X and Shang H (2024) Diagnostic value and correlation analysis of serum cytokine levels in patients with multiple system atrophy. Front. Cell. Neurosci. 18:1459884. doi: 10.3389/fncel.2024.1459884
Edited by:
Junhui Wang, University of Toronto, CanadaReviewed by:
Zhangyu Zou, Fujian Medical University Union Hospital, ChinaJiming Kong, University of Manitoba, Canada
Anastasia Bougea, National and Kapodistrian University of Athens, Greece
Copyright © 2024 Chen, Chen, Lai, Fu, Yang, Ou, Zhang, Wei, Guo and Shang. This is an open-access article distributed under the terms of the Creative Commons Attribution License (CC BY). The use, distribution or reproduction in other forums is permitted, provided the original author(s) and the copyright owner(s) are credited and that the original publication in this journal is cited, in accordance with accepted academic practice. No use, distribution or reproduction is permitted which does not comply with these terms.
*Correspondence: Huifang Shang, aGZzaGFuZzIwMDJAMTI2LmNvbQ==