- 1Department of Anatomy, Biomedical Center, Faculty of Medicine, University of Iceland, Reykjavik, Iceland
- 2ULB Neuroscience Institute (UNI), Université Libre de Bruxelles (ULB), Brussels, Belgium
Mast cells (MCs) are located in the meninges of the central nervous system (CNS), where they play key roles in the immune response. MC-deficient mice are advantageous in delineating the role of MCs in the immune response in vivo. In this study, we illustrate that a mutation in microphthalmia-associated transcription factor (Mitf) affects meningeal MC number in a dosage-dependent manner. C57BL/6J Mitf null mice lack meningeal MCs completely, whereas heterozygous mice have on average 25% fewer MCs. Mitf heterozygous mice might be a valuable tool to study the role of MCs in the meninges.
1 Introduction
Mast cells (MCs) are tissue-resident immune cells found throughout the body, especially at the interface between the body and the external environment, where they take part in the earliest immune responses. The immune responses of the central nervous system (CNS) are restricted in comparison to the rest of the body due to the presence of the blood–brain barrier (BBB), which serves as an internal boundary separating the CNS from the body. MCs are located at the BBB (Khalil et al., 2007) but also in the protective tissues surrounding the CNS, the meninges. The meninges are divided into three layers: dura, arachnoid, and pia. Arteries lie in the subarachnoid space, and MCs are located primarily in the dura in linear arrays alongside the arteries (Dimlich et al., 1991) and in proximity to neurons, including nociceptors (Rozniecki et al., 1999). MCs likely play a role in the normal physiological function of the meninges and are important for maintaining the BBB (Sayed et al., 2010). When activated, MCs degranulate a multitude of bioactive substances (including biogenic amines and MC-specific proteases), which affect other cells. MCs have different secretive phenotypes depending on the tissue microenvironment (Gurish and Austen, 2012). They are best known for their role in allergies as first responders to potentially harmful substances, but their role in the immune response following infections is still not fully understood (Valent et al., 2020; Dahlin et al., 2022). Meningeal MCs have also been shown to play roles in neurogenic inflammation, i.e., inflammation without infection, a central mechanism in migraines (Levy et al., 2006, 2019). Additionally, meningeal MCs are implicated in the outcome of stroke and other CNS pathologies (Arac et al., 2014; Russi et al., 2018). Understanding the role of MCs in the meninges is therefore of high importance.
1.1 The role of meningeal mast cell and mouse models
Inflammation is a central mechanism in CNS pathology with both short- and long-term effects. Inflammation can occur without infection, become chronic, or increase with age (inflammaging). Understanding how inflammatory processes can be influenced negatively or positively is instrumental in alleviating challenging pathological conditions found in many neurological diseases. During pathological conditions, crosstalk between different cell types is complex, especially in the CNS, and it is therefore difficult to model cell–cell interactions, e.g., in cell cultures. More complex models are needed to understand the role of MCs in the immune response, and mouse models that lack meningeal MCs have been central in delineating their role in the CNS. Many of these studies are based on mutations in Kit/Kitl—genes encoding for a growth factor and its receptor important for MC progenitor proliferation and differentiation (Grimbaldeston et al., 2005). Another mouse model is mutations in the microphthalmia-associated transcription factor (Mitf), which results in a stark reduction in MC number or absence depending on tissue (Kitamura et al., 2002; Ingason et al., 2019). There are other models in which MCs are targeted for apoptosis or depleted (Reber et al., 2012; Feyerabend et al., 2016; Sasaki et al., 2021; Dahlin et al., 2022).
1.2 The Mitf gene is required for mast cell generation and function
MITF is a basic helix–loop–helix leucine zipper transcription factor that plays a critical role in the development of many cell types (Hemesath et al., 1994; Steingrimsson et al., 2004), including melanocytes, retinal pigment cells, osteoclasts, and a subpopulation of CNS neurons (Nakayama et al., 1998; Steingrimsson et al., 2002; Gudjohnsen et al., 2015; Atacho et al., 2020). The Mitf gene has been shown to be both important for the generation (Kitamura et al., 2002; Morii et al., 2004; Sasaki et al., 2016; Ingason et al., 2019) and function of MCs (Morii et al., 1996, 1997; Nechushtan and Razin, 2002; Morii and Oboki, 2004; Paruchuru et al., 2022). There are connections between Mitf and Kit signaling (Tsujimura et al., 1996; Lee et al., 2011), and Mitf plays a key role in the binary lineage choice between MCs and basophils during development. The bipotent basophil/MC progenitors (BMCPs) develop into basophils or MCs depending on the relative ratio of C/EBPα to MITF, which are negatively correlated with each other (Qi et al., 2013; Sasaki et al., 2016). Mitf mutations cause these progenitors to develop into basophil-like cells. In Mitf null mutant mice, MCs are absent from the peritoneum (Morii and Oboki, 2004) and the heart (Ingason et al., 2019) and are reduced in number in the skin (Kim et al., 1999; Morii and Oboki, 2004). To date, the effect of the loss of Mitf on meningeal MCs has not been reported.
2 Methods
2.1 Animals
Animals of both sexes were used from the following mouse strains: C57BL/6 J (wild type) and Mitf mutant mice: heterozygote C57BL/6 J-Mi-mi-vga9/+ and homozygote C57BL/6 J-Mitf-mi-vga9/−mi-vga9. All animals were kept at the animal facility of the University of Iceland (VRIII) in a controlled environment at 21–22°C. Food and water were provided ad libitum. Sacrifice was performed by cervical dislocation. Animal procedures were approved by the Committee on Experimental Animals, according to Regulation 460/2017 and European Union Directive 2010/63 (license number 2013-03-01).
2.2 Avidin staining
Following euthanasia, the calvarium was removed from the skull, and the meninges adherent to the calvarium were fixed in 4% PFA for 30 min and rinsed with cold PBS. The whole meninges were removed, permeabilized, and blocked with blocking buffer (0.1% Triton 100x and 5% normal goat serum in PBS) for 1 h at room temperature. The samples were incubated with avidin AF488 conjugated (Catalog number A21370, Invitrogen) diluted 1/1000 and DAPI in a blocking buffer for 1 h at room temperature (Tharp et al., 1985). Finally, the samples were washed three times with 0.1% Triton X-100 in PBS for 20 min and mounted on slides using Fluoromount. Images of the whole meninges were acquired using an Olympus FV10-MCPSU Confocal Microscope.
2.3 Toluidine blue staining
The meninges were stained attached to the calvarium. The samples were submerged in a toluidine blue staining solution (1 g toluidine blue/100 mL of 70% EtOH in dH2O) for 5 min at room temperature. Subsequently, the meninges were washed three times in distilled H2O for 5 min, rinsed five times in 90% EtOH, and five times in 96% EtOH. Finally, the meninges were dissected from the calvarium and mounted on slides using solidifying mounting media. A Leica DM LB microscope (Leica Microsystems) at 10x magnification was used for image acquisition.
2.4 Statistical methods
The results obtained from MC quantification were analyzed by unpaired Student’s t-tests using GraphPad Prism 9. Numerical results represent the mean amount of MC/mm2 and the standard error of mean (SEM).
3 Results
3.1 Dosage dependence of Mitf on meningeal mast cells/results
To determine the degree to which meningeal MCs are affected by the lack of Mitf, the dura was collected from wild-type, heterozygous, and mutant adult mice, 2–3 months old. MCs were stained with avidin, a glycoprotein that binds to the granules of MCs (Tharp et al., 1985; Figure 1). There was a clear absence of MCs in the dura of the mutant animals and a reduction in MC number in heterozygotes. To quantify the number of MCs, toluidine blue, a dye that stains specifically acidic tissue components, and MC granules in purple were used to identify MCs in meninges. Toluidine staining of dura MCs confirmed the reduction of MC number in the heterozygotes (41.04 /mm2 ± 2.23 n = 14, wild type 49.9/mm2 ± 2.23 n = 17), while in the mutant no MCs (n = 15) were detected (Figure 2). No difference was observed between the sexes (N = 10, heterozygotes p = 0.288, wild type p = 0.642). The reduction in the heterozygotes was on average 25%, and not all heterozygotes showed a reduction. Some variation in the difference was observed between different experiments (difference between wild-type and heterozygotes: 10–28%).
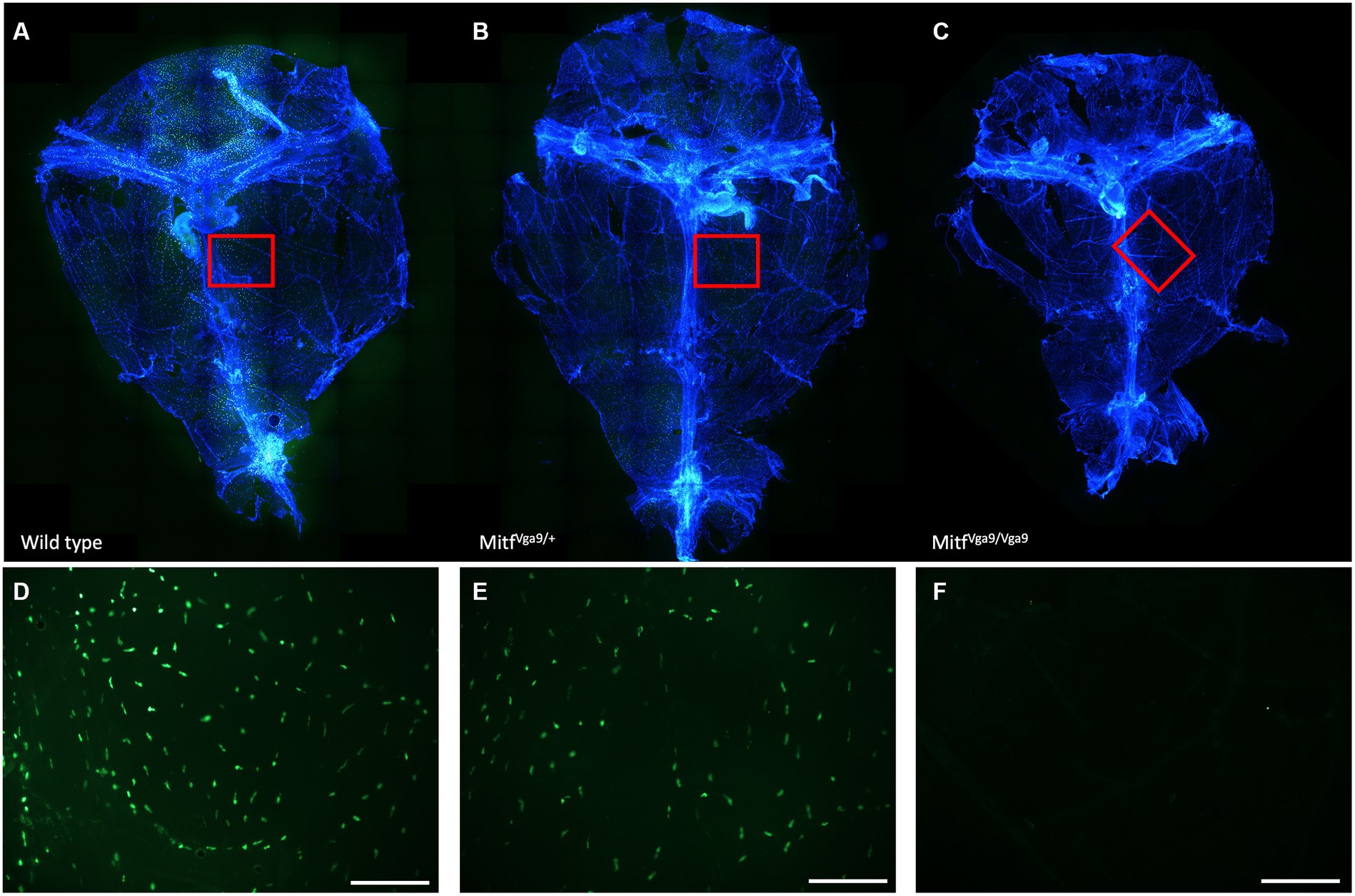
Figure 1. Distribution of MCs within meninges. Avidin staining of whole meninges of wild-type (A), Mitf heterozygote [(B) Mitfmi-vga9/+], and Mitf homozygote [(C) Mitfmi-vga9/mi-vga9] mice. Below (D–F) is a close-up of the meningeal MC distribution.
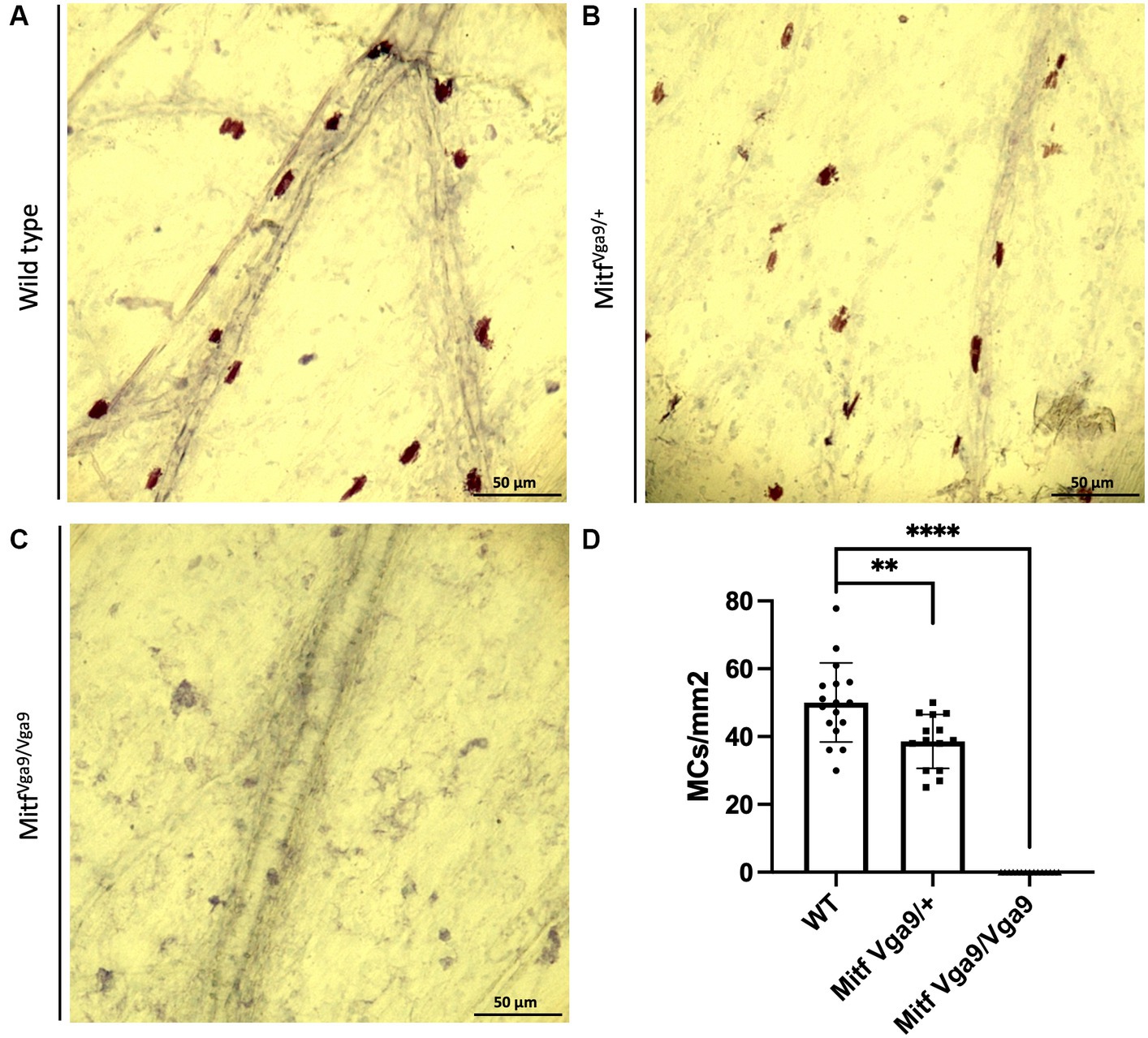
Figure 2. Loss of Mitf leads to a reduction in meningeal MC number. Toluidine blue staining of MCs from wild-type (A), Mitf heterozygote [(B) Mitfmi-vga9/+], and Mitf homozygote [(C) Mitfmi-vga9/mi-vga9] mouse meninges. (D) MC number in different genotypes. Wild-type mice n = 17, heterozygote C57BL/6 J-Mitfmi-vga9/+ n = 14, C57BL/6 J-Mitfmi-vga9/mi-vga9 n = 15. Asterisks indicate statistical significance [p < 0.01 (**) and p < 0.0001 (****)]. Scale bar represents 50 μm.
4 Discussion
MCs play a central role in the meninges and have diverse connections to CNS pathology, especially inflammation but also stroke, migraine, and neurological diseases. Mouse models of MC deficiency are crucial to the study of MC biology, which is often difficult to address in ex vivo models (human material or cell culture) due to cell–cell interactions. Of these models, the Mitf mutant mouse is promising due to the reduction of MCs throughout the body (Morii et al., 2004; Ingason et al., 2019). We show here that Mitf null mice lack meningeal MCs and can therefore be used to study the role of MCs in the immune responses defending the CNS. However, it is important to note that studies using constitutive Mitf mutant mice must also consider the lack of meningeal melanocytes (Gudjohnsen et al., 2015) and that any hypotheses of Mitf function in the CNS must take the lack of both of these cell types into account.
Importantly, Mitf heterozygous mice, which are phenotypically identical to wild-type mice, show a reduction in meningeal MC number as previously reported for cardiac MCs (Ingason et al., 2019). In the heart, the reduction of MCs in the heterozygote is approximately 50% (Ingason et al., 2019) and in the meninges, it is approximately 25%. While two copies of Mitf are required for a normal number of MCs, this suggests a tissue-dependent Mitf dosage effect or defects in maintaining MCs in heterozygotes. This is in accordance with known haploinsufficiency in Mitf mutant mice (Harris et al., 2018; Atacho et al., 2020; Han et al., 2020). Increased inflammation is central to CNS pathologies. Aging affects MCs (Gunin et al., 2011; Chatterjee and Gashev, 2012; Kutukova et al., 2016) and has been reported to be proinflammatory (Elbasiony et al., 2023) during aging. As Mitf is also required for MC function, Mitf heterozygous mice might be more applicable for studies of meningeal MCs during aging in comparison to other MC models, which are limited due to the absence or reduced number of MCs. Further studies are needed to examine the extent of this effect and the possibility of using Mitf heterozygotes to further explore the compromised function of meningeal MCs during aging.
Data availability statement
The raw data supporting the conclusions of this article will be made available by the authors, without undue reservation.
Ethics statement
The animal study was approved by the Icelandic Committee on Experimental Animals. The study was conducted in accordance with the local legislation and institutional requirements.
Author contributions
AS: Data curation, Investigation, Writing – review & editing. PP: Conceptualization, Investigation, Methodology, Project administration, Writing – original draft, Writing – review & editing.
Funding
The author(s) declare financial support was received for the research, authorship, and/or publication of this article. This research was funded by the University of Iceland.
Acknowledgments
The authors would like to thank Signe Skadborg, Eiríkur Steingrímsson, and Sana Gadiwalla for their assistance and input.
Conflict of interest
The authors declare that the research was conducted in the absence of any commercial or financial relationships that could be construed as a potential conflict of interest.
Publisher’s note
All claims expressed in this article are solely those of the authors and do not necessarily represent those of their affiliated organizations, or those of the publisher, the editors and the reviewers. Any product that may be evaluated in this article, or claim that may be made by its manufacturer, is not guaranteed or endorsed by the publisher.
References
Arac, A., Grimbaldeston, M. A., Nepomuceno, A. R. B., Olayiwola, O., Pereira, M. P., Nishiyama, Y., et al. (2014). Evidence that meningeal mast cells can worsen stroke pathology in mice. Am. J. Pathol. 184, 2493–2504. doi: 10.1016/j.ajpath.2014.06.003
Atacho, D. A. M., Reynisson, H., Petursdottir, A. T., Eysteinsson, T., Steingrimsson, E., and Petersen, P. H. (2020). Mitf links neuronal activity and long-term homeostatic intrinsic plasticity. eNeuro 7, ENEURO.0412–ENEU19.2020. doi: 10.1523/ENEURO.0412-19.2020
Chatterjee, V., and Gashev, A. A. (2012). Aging-associated shifts in functional status of mast cells located by adult and aged mesenteric lymphatic vessels. Am. J. Physiol. Heart Circ. Physiol. 303, H693–H702. doi: 10.1152/ajpheart.00378.2012
Dahlin, J. S., Maurer, M., Metcalfe, D. D., Pejler, G., Sagi-Eisenberg, R., and Nilsson, G. (2022). The ingenious mast cell: contemporary insights into mast cell behavior and function. Allergy 77, 83–99. doi: 10.1111/all.14881
Dimlich, R. V., Keller, J. T., Strauss, T. A., and Fritts, M. J. (1991). Linear arrays of homogeneous mast cells in the dura mater of the rat. J. Neurocytol. 20, 485–503. doi: 10.1007/BF01252276
Elbasiony, E., Cho, W. J., Singh, A., Mittal, S. K., Zoukhri, D., and Chauhan, S. K. (2023). Increased activity of lacrimal gland mast cells are associated with corneal epitheliopathy in aged mice. NPJ Aging 9:2. doi: 10.1038/s41514-023-00099-0
Feyerabend, T. B., Gutierrez, D. A., and Rodewald, H. R. (2016). Of mouse models of mast cell deficiency and metabolic syndrome. Cell Metab. 24, 1–2. doi: 10.1016/j.cmet.2016.06.019
Grimbaldeston, M. A., Chen, C. C., Piliponsky, A. M., Tsai, M., Tam, S. Y., and Galli, S. J. (2005). Mast cell-deficient W-sash c-kit mutant kit (W-sh/W-sh) mice as a model for investigating mast cell biology in vivo. Am. J. Pathol. 167, 835–848. doi: 10.1016/S0002-9440(10)62055-X
Gudjohnsen, S. A., Atacho, D. A., Gesbert, F., Raposo, G., Hurbain, I., Larue, L., et al. (2015). Meningeal melanocytes in the mouse: distribution and dependence on Mitf. Front. Neuroanat. 9:149. doi: 10.3389/fnana.2015.00149
Gunin, A. G., Kornilova, N. K., Vasilieva, O. V., and Petrov, V. V. (2011). Age-related changes in proliferation, the numbers of mast cells, eosinophils, and cd45-positive cells in human dermis. J. Gerontol. A Biol. Sci. Med. Sci. 66, 385–392. doi: 10.1093/gerona/glq205
Gurish, M. F., and Austen, K. F. (2012). Developmental origin and functional specialization of mast cell subsets. Immunity 37, 25–33. doi: 10.1016/j.immuni.2012.07.003
Han, S., Chen, J., Hua, J., Hu, X., Jian, S., Zheng, G., et al. (2020). MITF protects against oxidative damage-induced retinal degeneration by regulating the NRF2 pathway in the retinal pigment epithelium. Redox Biol. 34:101537. doi: 10.1016/j.redox.2020.101537
Harris, M. L., Fufa, T. D., Palmer, J. W., Joshi, S. S., Larson, D. M., Incao, A., et al. (2018). A direct link between MITF, innate immunity, and hair graying. PLoS Biol. 16:e2003648. doi: 10.1371/journal.pbio.2003648
Hemesath, T. J., Steingrimsson, E., McGill, G., Hansen, M. J., Vaught, J., Hodgkinson, C. A., et al. (1994). Microphthalmia, a critical factor in melanocyte development, defines a discrete transcription factor family. Genes Dev. 8, 2770–2780. doi: 10.1101/gad.8.22.2770
Ingason, A. B., Mechmet, F., Atacho, D. A. M., Steingrimsson, E., and Petersen, P. H. (2019). Distribution of mast cells within the mouse heart and its dependency on Mitf. Mol. Immunol. 105, 9–15. doi: 10.1016/j.molimm.2018.11.009
Khalil, M., Ronda, J., Weintraub, M., Jain, K., Silver, R., and Silverman, A. J. (2007). Brain mast cell relationship to neurovasculature during development. Brain Res. 1171, 18–29. doi: 10.1016/j.brainres.2007.07.034
Kim, D. K., Morii, E., Ogihara, H., Lee, Y. M., Jippo, T., Adachi, S., et al. (1999). Different effect of various mutant MITF encoded by mi, mi(or), or mi(wh) allele on phenotype of murine mast cells. Blood 93, 4179–4186. doi: 10.1182/blood.V93.12.4179
Kitamura, Y., Morii, E., Jippo, T., and Ito, A. (2002). Regulation of mast cell phenotype by MITF. Int. Arch. Allergy Immunol. 127, 106–109. doi: 10.1159/000048178
Kutukova, N. A., Nazarov, P. G., Kudryavtseva, G. V., and Shishkin, V. I. (2016). Mast cells and aging. Adv. Gerontol. 29, 586–593.
Lee, Y. N., Brandal, S., Noel, P., Wentzel, E., Mendell, J. T., McDevitt, M. A., et al. (2011). KIT signaling regulates MITF expression through miRNAs in normal and malignant mast cell proliferation. Blood 117, 3629–3640. doi: 10.1182/blood-2010-07-293548
Levy, D., Burstein, R., and Strassman, A. M. (2006). Mast cell involvement in the pathophysiology of migraine headache: a hypothesis. Headache 46, S13–S18. doi: 10.1111/j.1526-4610.2006.00485.x
Levy, D., Labastida-Ramirez, A., and MaassenVanDenBrink, A. (2019). Current understanding of meningeal and cerebral vascular function underlying migraine headache. Cephalalgia 39, 1606–1622. doi: 10.1177/0333102418771350
Morii, E., Ito, A., Jippo, T., Koma, Y., Oboki, K., Wakayama, T., et al. (2004). Number of mast cells in the peritoneal cavity of mice: influence of microphthalmia transcription factor through transcription of newly found mast cell adhesion molecule, spermatogenic immunoglobulin superfamily. Am. J. Pathol. 165, 491–499. doi: 10.1016/S0002-9440(10)63314-7
Morii, E., Jippo, T., Tsujimura, T., Hashimoto, K., Kim, D. K., Lee, Y. M., et al. (1997). Abnormal expression of mouse mast cell protease 5 gene in cultured mast cells derived from mutant mi/mi mice. Blood 90, 3057–3066. doi: 10.1182/blood.V90.8.3057
Morii, E., and Oboki, K. (2004). MITF is necessary for generation of prostaglandin D2 in mouse mast cells. J. Biol. Chem. 279, 48923–48929. doi: 10.1074/jbc.M407026200
Morii, E., Tsujimura, T., Jippo, T., Hashimoto, K., Takebayashi, K., Tsujino, K., et al. (1996). Regulation of mouse mast cell protease 6 gene expression by transcription factor encoded by the mi locus. Blood 88, 2488–2494. doi: 10.1182/blood.V88.7.2488.bloodjournal8872488
Nakayama, A., Nguyen, M. T., Chen, C. C., Opdecamp, K., Hodgkinson, C. A., and Arnheiter, H. (1998). Mutations in microphthalmia, the mouse homolog of the human deafness gene MITF, affect neuroepithelial and neural crest-derived melanocytes differently. Mech. Dev. 70, 155–166. doi: 10.1016/S0925-4773(97)00188-3
Nechushtan, H., and Razin, E. (2002). The function of MITF and associated proteins in mast cells. Mol. Immunol. 38, 1177–1180. doi: 10.1016/S0161-5890(02)00059-7
Paruchuru, L. B., Govindaraj, S., and Razin, E. (2022). The critical role played by mitochondrial MITF serine 73 phosphorylation in immunologically activated mast cells. Cell 11:589. doi: 10.3390/cells11030589
Qi, X., Hong, J., Chaves, L., Zhuang, Y., Chen, Y., Wang, D., et al. (2013). Antagonistic regulation by the transcription factors C/EBPalpha and MITF specifies basophil and mast cell fates. Immunity 39, 97–110. doi: 10.1016/j.immuni.2013.06.012
Reber, L. L., Marichal, T., and Galli, S. J. (2012). New models for analyzing mast cell functions in vivo. Trends Immunol. 33, 613–625. doi: 10.1016/j.it.2012.09.008
Rozniecki, J. J., Dimitriadou, V., Lambracht-Hall, M., Pang, X., and Theoharides, T. C. (1999). Morphological and functional demonstration of rat dura mater mast cell-neuron interactions in vitro and in vivo. Brain Res. 849, 1–15. doi: 10.1016/S0006-8993(99)01855-7
Russi, A. E., Walker-Caulfield, M. E., and Brown, M. A. (2018). Mast cell inflammasome activity in the meninges regulates EAE disease severity. Clin. Immunol. 189, 14–22. doi: 10.1016/j.clim.2016.04.009
Sasaki, H., Imanishi, M., Fujikura, D., Sugiyama, M., Tanimoto, K., Mochiji, Y., et al. (2021). New inducible mast cell-deficient mouse model (Mcpt5/Cma1(DTR)). Biochem. Biophys. Res. Commun. 551, 127–132. doi: 10.1016/j.bbrc.2021.03.025
Sasaki, H., Kurotaki, D., and Tamura, T. (2016). Regulation of basophil and mast cell development by transcription factors. Allergol. Int. 65, 127–134. doi: 10.1016/j.alit.2016.01.006
Sayed, B. A., Christy, A. L., Walker, M. E., and Brown, M. A. (2010). Meningeal mast cells affect early T cell central nervous system infiltration and blood-brain barrier integrity through TNF: a role for neutrophil recruitment? J. Immunol. 184, 6891–6900. doi: 10.4049/jimmunol.1000126
Steingrimsson, E., Copeland, N. G., and Jenkins, N. A. (2004). Melanocytes and the microphthalmia transcription factor network. Annu. Rev. Genet. 38, 365–411. doi: 10.1146/annurev.genet.38.072902.092717
Steingrimsson, E., Tessarollo, L., Pathak, B., Hou, L., Arnheiter, H., Copeland, N. G., et al. (2002). Mitf and Tfe3, two members of the Mitf-Tfe family of bHLH-zip transcription factors, have important but functionally redundant roles in osteoclast development. Proc. Natl. Acad. Sci. USA 99, 4477–4482. doi: 10.1073/pnas.072071099
Tharp, M. D., Seelig, L. L. Jr., Tigelaar, R. E., and Bergstresser, P. R. (1985). Conjugated avidin binds to mast cell granules. J. Histochem. Cytochem. 33, 27–32. doi: 10.1177/33.1.2578142
Tsujimura, T., Morii, E., Nozaki, M., Hashimoto, K., Moriyama, Y., Takebayashi, K., et al. (1996). Involvement of transcription factor encoded by the mi locus in the expression of c-kit receptor tyrosine kinase in cultured mast cells of mice. Blood 88, 1225–1233. doi: 10.1182/blood.V88.4.1225.bloodjournal8841225
Keywords: microphthalmia-associated transcription factor, mast cells, meninges, inflammation, aging
Citation: Sabaté San José A and Petersen PH (2024) Absence of meningeal mast cells in the Mitf mutant mouse. Front. Cell. Neurosci. 18:1337621. doi: 10.3389/fncel.2024.1337621
Edited by:
Antonietta Bernardo, National Institute of Health (ISS), ItalyReviewed by:
Shweta Pradip Jadhav, Consultant, Carlsbad, CA, United StatesEnrico Radaelli, University of Pennsylvania, United States
Copyright © 2024 Sabaté San José and Petersen. This is an open-access article distributed under the terms of the Creative Commons Attribution License (CC BY). The use, distribution or reproduction in other forums is permitted, provided the original author(s) and the copyright owner(s) are credited and that the original publication in this journal is cited, in accordance with accepted academic practice. No use, distribution or reproduction is permitted which does not comply with these terms.
*Correspondence: Petur Henry Petersen, cGhlbnJ5QGhpLmlz