- Department of Pathology, Rady Faculty of Health Sciences, University of Manitoba, Winnipeg, MB, Canada
The history of research concerning ependymal cells is reviewed. Cilia were identified along the surface of the cerebral ventricles c1835. Numerous anatomical and histopathological studies in the late 1800’s showed irregularities in the ependymal surface that were thought to be indicative of specific pathologies such as syphilis; this was subsequently disproven. The evolution of thoughts about functions of cilia, the possible role of ependyma in the brain-cerebrospinal fluid barrier, and the relationship of ependyma to the subventricular zone germinal cells is discussed. How advances in light and electron microscopy and cell culture contributed to our understanding of the ependyma is described. Discoveries of the supraependymal serotoninergic axon network and supraependymal macrophages are recounted. Finally, the consequences of loss of ependymal cells from different regions of the central nervous system are considered.
Introduction
The typical medical school curriculum does not transmit much information about the ependyma. There are perhaps two slides in an introductory neurocytology lecture and passing mention in lectures concerning neurodevelopment and cerebrospinal fluid (CSF) physiology. I started thinking about ependymal cells in 1983 when I began my PhD studies, investigating the pathogenesis of hydrocephalus and shunt obstruction. My mentor was Dr. J. Edward Bruni, a neuroanatomist and electron microscopist who had been studying tanycytes and their role in brain physiology (Bruni, 1974). Leap forward 40 years, in the course of studying over 6,000 human brains in detail as a neuropathologist, I see ependymal cells almost every day. And I still ponder this seemingly simple epithelium.
In the past almost two centuries, much has been learned about the structure and function of ependymal cells, as well as the associated cell populations on top of and below the ependymal surface. Herein, I review the history of research concerning the ependyma and offer some perspective from the point of view of an experienced neuropathologist.
Earliest anatomical and pathological descriptions of ependyma
The presence of fluid-filled spaces within the brain (i.e., the ventricles) has been recognized since antiquity (Mortazavi et al., 2014). Wenzel and Wenzel (1806) wrote that the ventricles are “lined by an extremely fine membrane, which covers what little medullary substance the walls of the cavity contain, and which is sometimes easily removed from the whole inner surface when much water has accumulated in the cavity” (translated from German). In 1833, the Czech anatomist Jan Purkyně (Purkinje) and his student Gabriel Valentin were dissecting fertilized eggs from a rabbit uterus using a microscope. They observed that the surface of the oviduct was moving vigorously. Curious about it, they examined the surfaces and lumens of various organs in several species at magnifications of 300–400×. They initially stated that the “Flimmerbewegungen” (flickering movements, which were later determined to be caused by cilia) were present only on the inner surfaces of the female reproductive tract and the respiratory tract, but not on the arachnoid (Purkinje and Valentin, 1834). Two years later, Purkinje studied sheep and pig fetuses and reported that he had “finally succeeded in discovering the cilia and their movements in the entire cerebral cavities of mammals” (translated from German), albeit not on the choroid plexus epithelial cells (Purkinje and Valentin, 1835; Purkinje, 1836). He referred to these as the “Flimmermembranen” (ciliated membranes). Valentin, having moved away to establish his own laboratory, published the first illustration of ependymal cells in 1836 (Figure 1; Valentin, 1836).
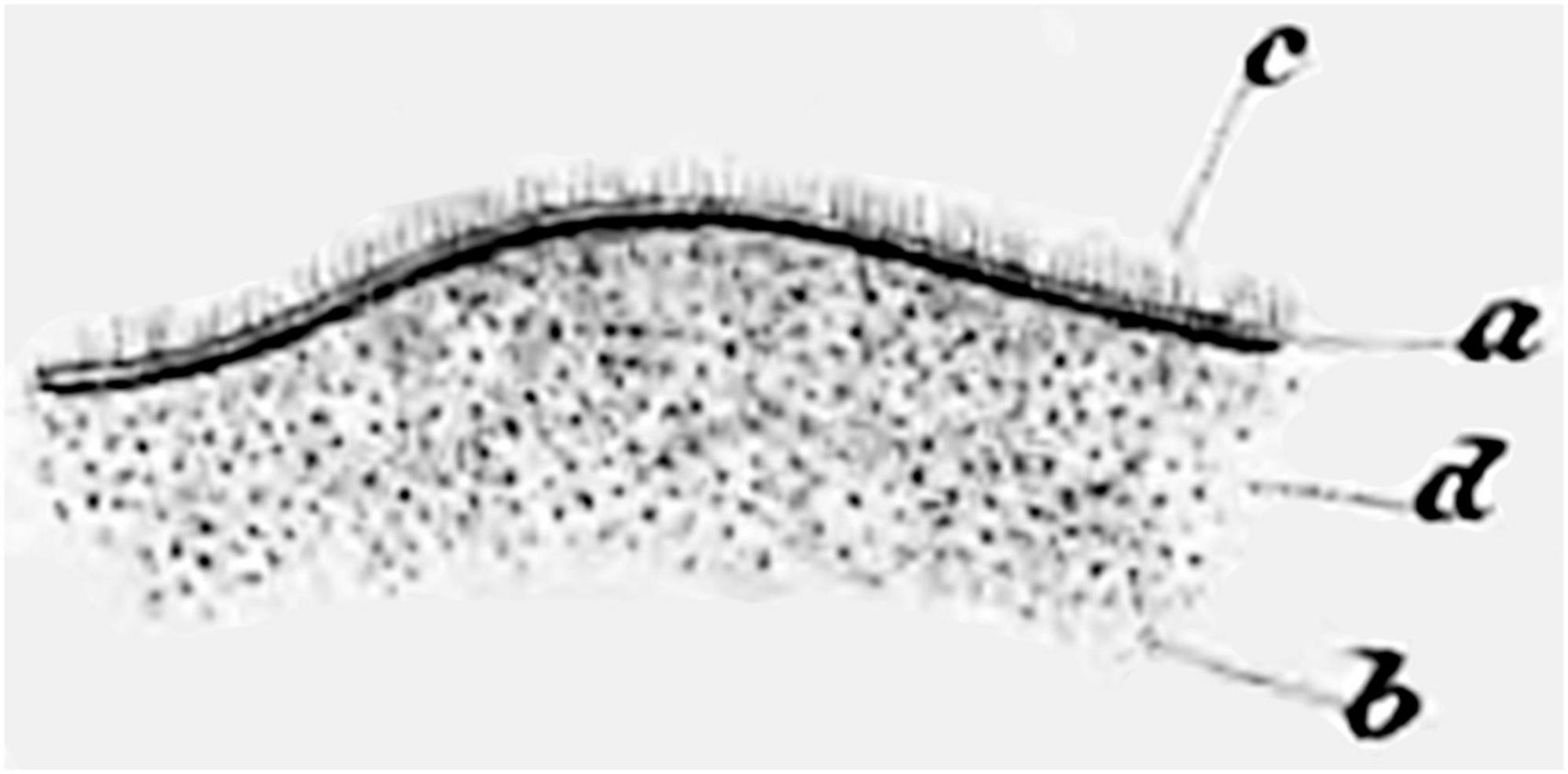
Figure 1. Hand-drawn image from Valentin (1836), the first to illustrate cilia on the ventricle surface. Figure 27 shows “Depiction of the fibrillation movement on the surface of the lateral ventricle of the adult human, as it is still evident during the undisturbed vibrations. a. The ciliated epithelium. b. The hairs visible as individual dots when viewed from the surface and from above. c. The upright hairs visible from the side on the folded edge. d. The balls of nerve substance that faintly shine through the ciliated epithelium” (Valentin, 1836) (illustration in public domain).
The term “ependyma” (from the Greek word meaning “outer garment” or “investment”) in reference to the surface of the ventricles (the “ependyma ventriculorum”) was likely first used by the German anatomist Carl Ernst Bock a few years after Purkinje’s discovery c1839 (Šimon, 2016). Bock clearly described it as “an extremely delicate, transparent, cellular membrane, which is so intimately fused with the surface of the parts that protrude from the walls of the cerebral cavity that it can only be distinguished from them in connection with a thin, inner surface adhering layer of nerve substance” (translated from German) (Bock, 1840). The term ependyma became widely used in the anatomical literature soon after (Kolliker, 1854; Peaslee, 1957). Detailed descriptions of choroid plexus appeared during the same era (Haeckel, 1859). Some authors later advocated, albeit unsuccessfully, for use of alternate terminology such as “ependymium” (similar to “epithelium”) (Altschul, 1941).
In the mid-1800’s, the German pathologist Rudolf Virchow described brain tissues adjacent to the ependyma and introduced the term “neuro-glia” (“nerve glue”) (Virchow, 1853, 1863; Lyons, 1854). In his textbooks, Virchow suggested that the term ependyma should include the surface epithelium along with the underlying “connective tissue” (Virchow, 1863; Figure 2). It is not clear that this collective definition was widely adopted; regardless, it remains important for writers of the current literature to define what they mean by “ependyma.”
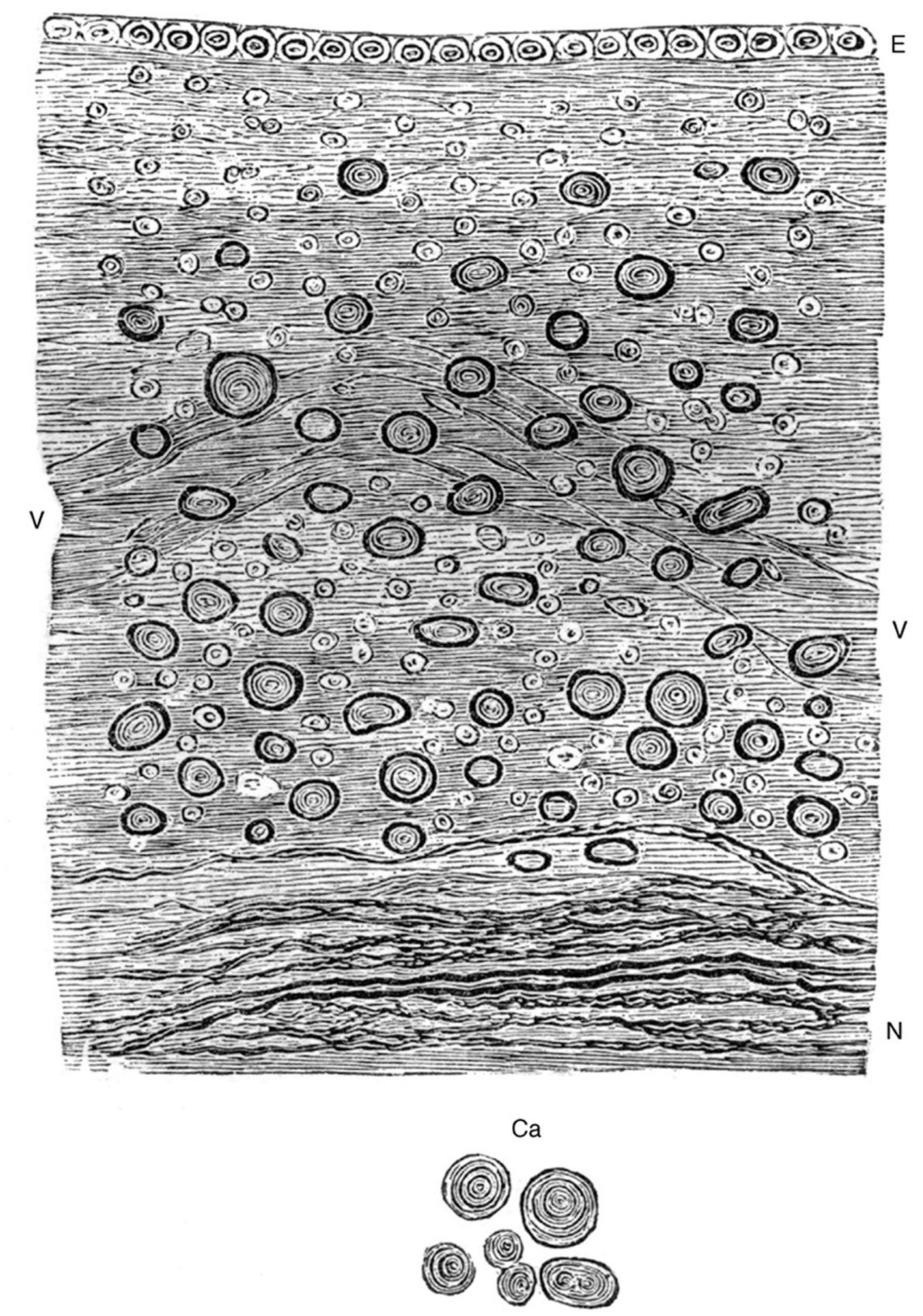
Figure 2. Hand-drawn image from Virchow (1858) (Figure 94) showing the relationship between the ependymal epithelium at the ventricle surface and the underlying neuroglial tissue. “Ependyma ventriculorum and neuro-glia from the floor of the fourth cerebral ventricle. E, Epithelium, N, nerve-fibers. Between them the free portion of the neuro-glia with numerous connective-tissue-corpuscles and nuclei, at V a vessel. In addition, numerous corpora amylacea, which are moreover represented separately at Ca. 300 diameters” (Virchow, 1858, 1863) (illustration in public domain).
Many early German pathology textbooks including (Rokitansky, 1856), and others all described granular changes along the ependymal surface in the context of hydrocephalus and infections (Walther, 1897). Friedreich (1858) described thickening and hardening of the ependymal lining following some cases of brain infection. Of particular historical interest was the perceived association between ependymal granulations (“granular ependymitis”) and insanity, syphilis, etc. (Addison, 1862; Beadles, 1895; Figure 3). Baroncini concluded in 1,888 that granulations were not specifically related to disease (Baroncini, 1888), but the concept persisted for decades (Blachford, 1903; Baird, 1910).
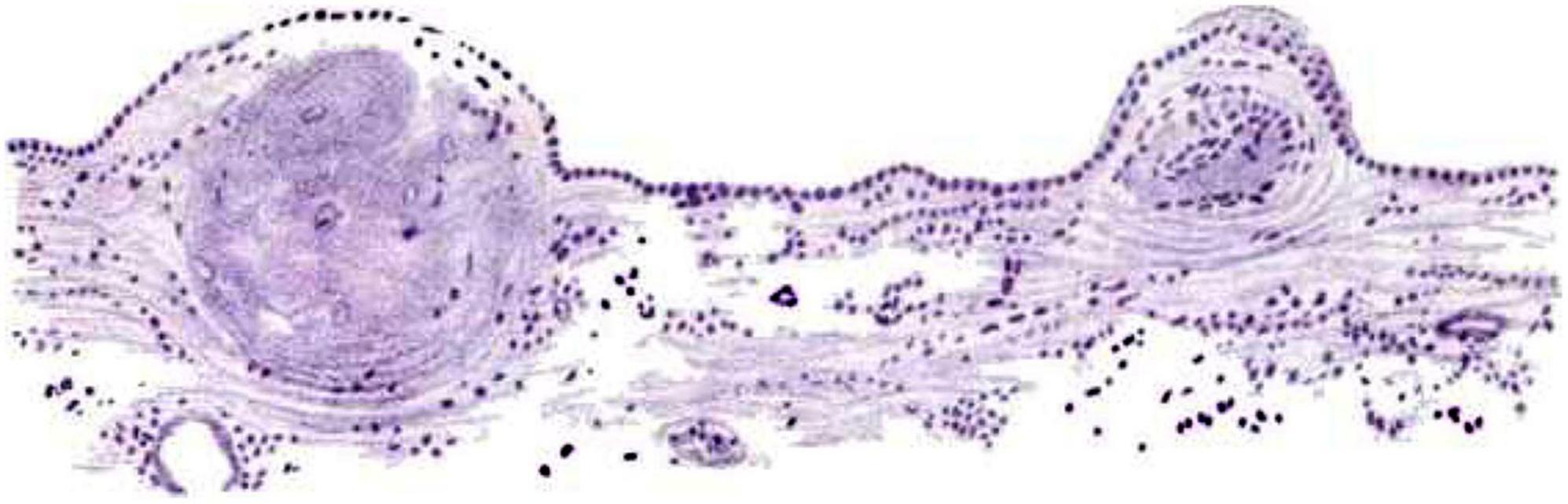
Figure 3. Hand-painted image from Beadles (1895) (Figure 3) showing “Ependyma of lateral ventricle from a G.P. (general paresis case) Two granulations in an early stage. The membrane is only slightly thickened, except where the nodules are appearing, and is evenly covered by a single layer of columnar-shaped epithelium. Beneath the thickenings are groups of deeply-staining cells resembling that of glandular epithelium. Logwood (stain; Haematoxylum campechianum)” (Beadles, 1895) (illustration in public domain).
Early hypotheses about the functions of the ependyma
In the mid-1800’s, Friedreich postulated that fluid resorption occurred at the ependymal surface, with cilia facilitating this function (Friedreich, 1858). By the late 1800’s there were many hypotheses about the role(s) of ependymal cells. In 1879, Deecke published a rambling summary of suggested interactions between ependymal cells and the underlying tissues, similarities with ciliated epithelia in other organs, and possible differences between higher and lower animals. He mentioned protective, absorptive, and sensory roles (Deecke, 1879). In an 1890 study of cat brains using improved microscopy methods, Fish distinguished the ciliated nature of mural ependymal cells from the (relatively) non-ciliated choroid plexus epithelial cells. He also identified anchoring basal processes of ependymal cells in some regions of brain (Figure 4; Fish, 1891). In 1897, Eurich focused attention on the basal processes of ependymal cells and their relationship to germinal cells, which were then known as astroblasts and spongioblasts. He was one of the first to highlight the possibility that ependyma might serve a germinal function, although he was skeptical of the idea that “each individual neuroglia cell is directly derived from an ependyma cell” (Eurich, 1897).
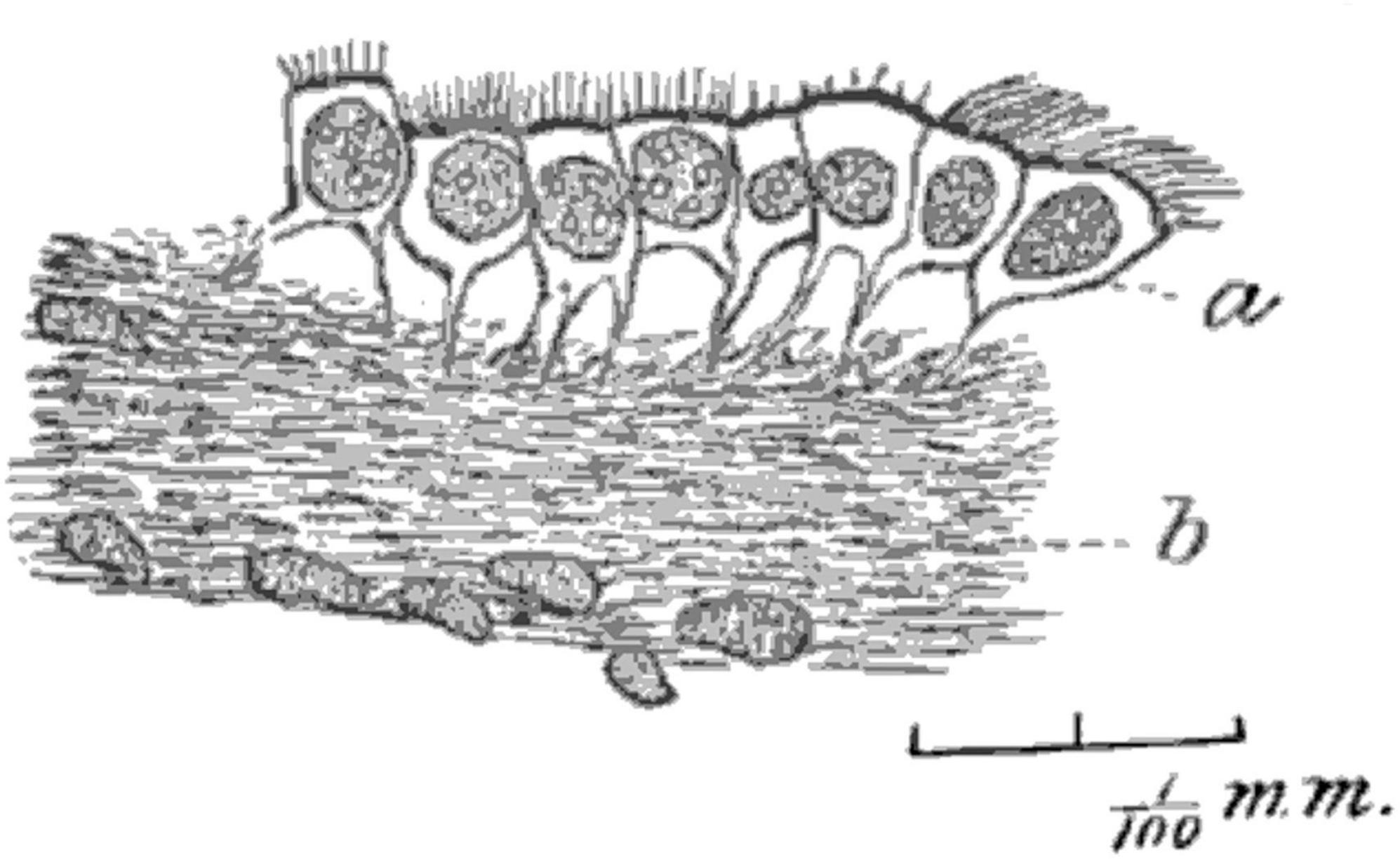
Figure 4. Hand-drawn image from Fish (1891) (Figure 2) used a Zeiss 8 mm objective and Abbe camera lucida. Tissue from human mesencephalon was fixed with Gaule’s method, embedded in colloidin, and sectioned. The image shows “the layer of (ependymal) cells is partially detached from the neuroglia, showing their root-like processes. Magnified about 700 diameters” (Fish, 1891) (illustration in public domain).
Improved understanding of ependymal morphology and pathology in the early 1900’s
During the 1800s, the vast majority of ependyma illustrations were hand drawn versions of microscopic observations. Likely the earliest photomicrographs of ependymal cells appeared in the 1896 thesis by Thomas Adair at Edinburgh. He published an organized review of ependyma form and function along with a detailed account of ependymal histology in a variety of species. This included the fourth ventricles of 56 humans with a range of neurological conditions including mania, melancholia (depression), dementia, syphilis, epilepsy, and hydrocephalus (Adair, 1896; Figure 5). He clearly illustrated foci of ependymal discontinuity, ependymal granulations, and buried islands of ependymal cells with and without microscopic lumens. He considered most of these to be degenerative, the result of “previous active congestions of the brain” or “acute or subacute inflammatory processes.” He also mentioned tumors “as large as a pea, or larger”; some of these likely represent subependymomas (see section titled “Neoplasms of the ependyma”).
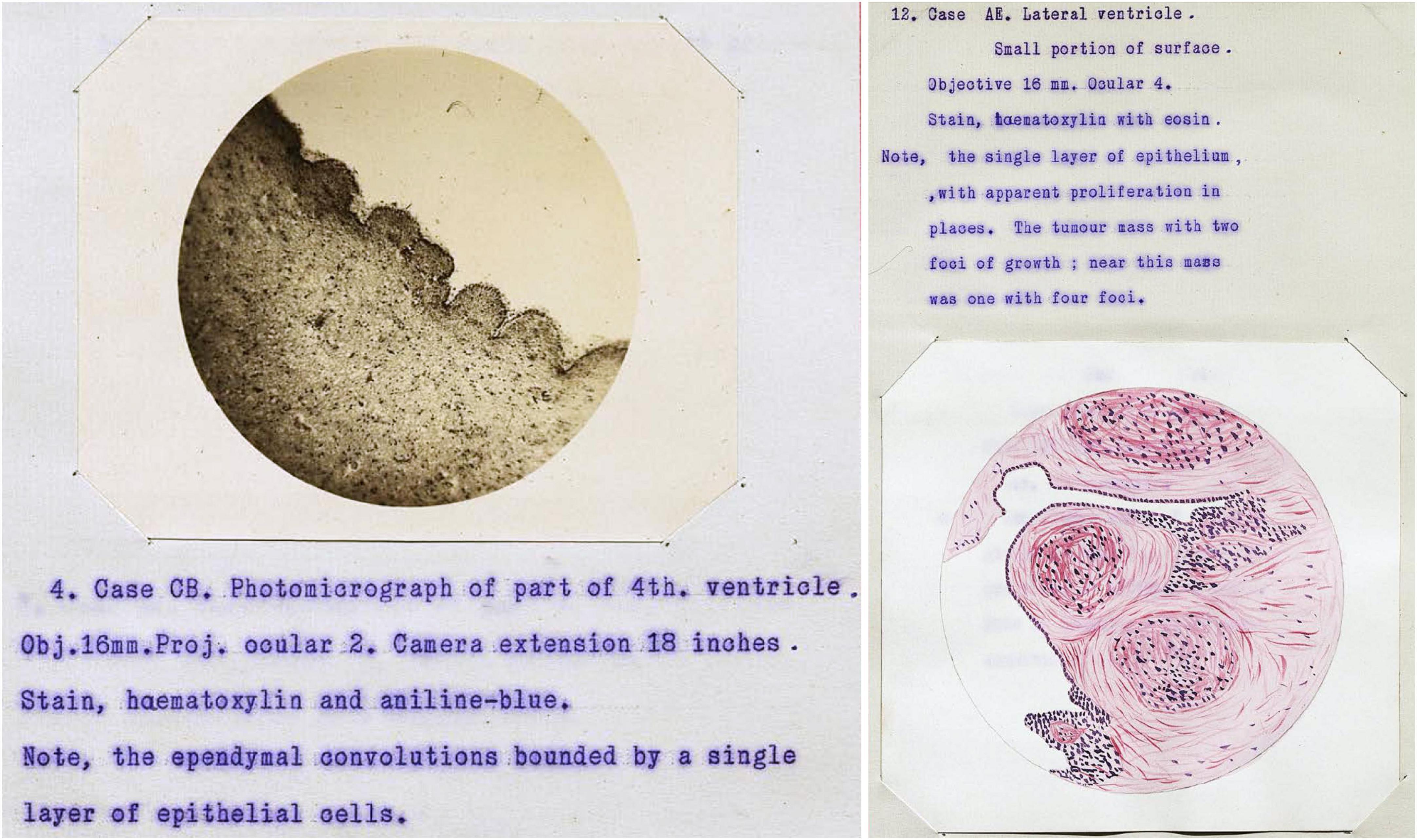
Figure 5. Case illustrations from Adair (1896) thesis showing the first published photomicrographs of ependymal cells (illustration in public domain).
In 1900, František Studnička from Prague published a beautifully detailed account (with drawings only) summarizing prior work and describing regional anatomical differences in the ependyma of species ranging from lower vertebrates to humans. He wrote “the ependyma is present everywhere on the inner surface of the cerebrospinal tubes” and he described situations of secondary loss with transformation into neuroglial cells. He demonstrated at the base of each cilium small “Basalkörperchen” (basal bodies) or “Blepharoplasten” (blepharoplasts) (Figure 6; Studnička, 1900).
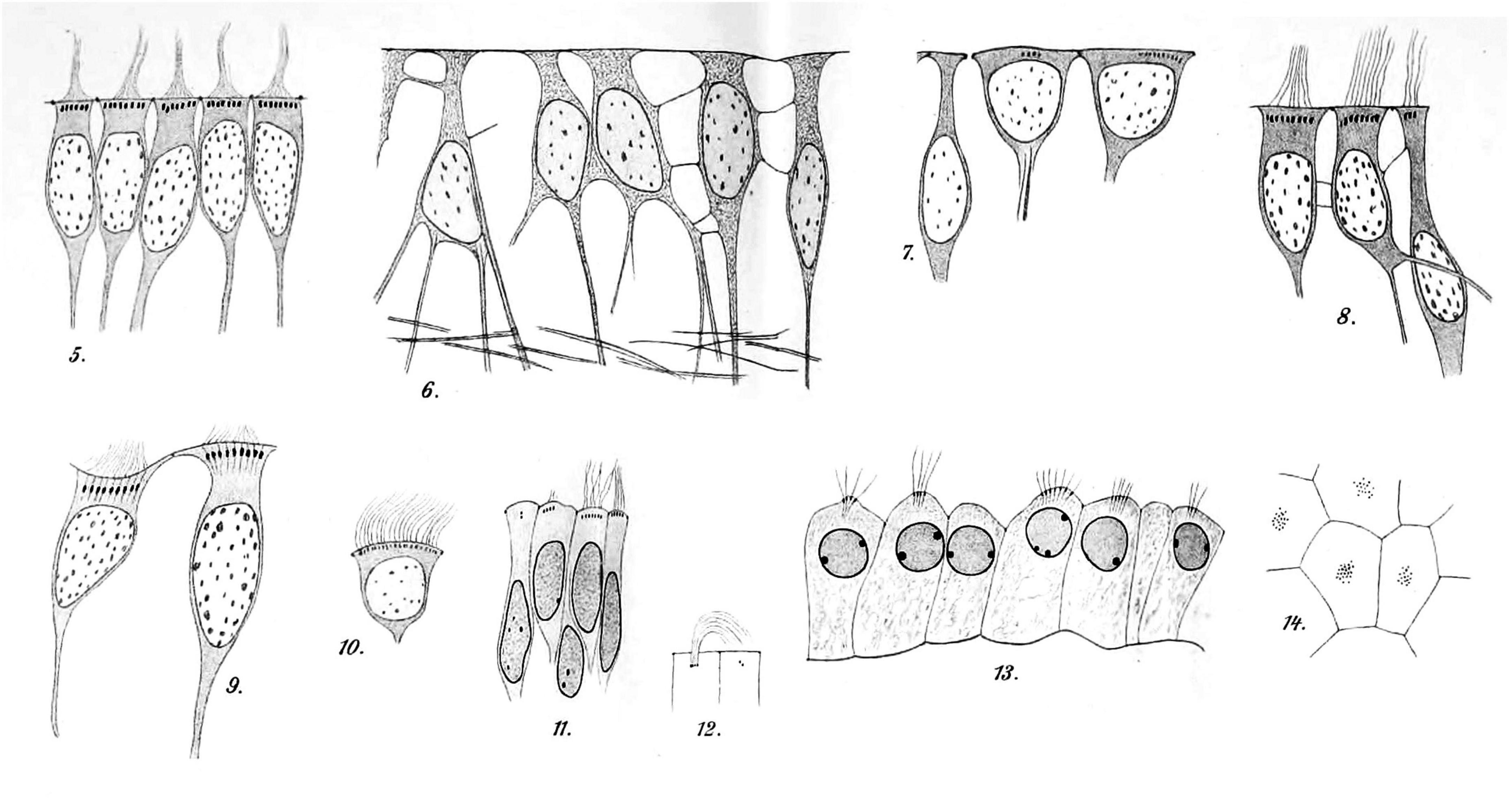
Figure 6. Hand-drawn images of ependymal cells from human brains [reproduced and re-organized from Studnička (1900) Tafel XXXII/XXXIII]. Images 5–10 are ependymal cells of the fourth ventricle of an adult. Images 11 and 12 are ependymal cells from the lateral ventricle of a fetal brain. The dark spots at the base of the cilia are the basal bodies. Images 13 (sectioned) and 14 (en face) show epithelial cells of the lateral ventricle choroid plexus from a fetal brain. Tissues had been processed with Muller’s fixative (potassium dichromate), sectioned, and stained with iron hematoxylin (Studnička, 1900) (illustration in public domain).
During the decades soon after, there was little progress. Anatomists and pathologists continued to describe areas of ependyma loss in varied circumstances and the associated proliferation of glial tissue along the ventricle walls (“ependymal gliomatosis”) (Margulis, 1913). Serial sectioning of tissue showed that some clusters of ependymal cells below the ventricular surface were truly isolated “rosettes” while others represented parts of narrow diverticulae. Incorrectly, some of these were believed to be the result of ependymal cell hyperplasia (Altschul, 1941).
Increasingly in the 1930’s, publications concerning ependymal biology and pathology were written in English rather than German. It was becoming clear that some infectious agents (e.g., syphilis and tuberculosis) could damage the ependymal lining of the ventricles thereby scarring the CSF pathways and causing hydrocephalus (Arnold, 1934). Several investigators evaluated acute ependymal changes following blunt head trauma. Rand and Courville (1931) reported vacuolization of ependymal and choroid plexus epithelium in association with brain edema. Gierlich (1936) documented “hemorrhages under intact and defective ependyma” in many autopsy cases. Most textbooks of anatomy had fairly superficial contemplations of ependymal cells, considering them to be a terminally differentiated cell population (Ranson, 1935). However, in one textbook of neuropathology, the author suggested many possible roles of ependymal cells (a) in support of subjacent cells, (b) as a possible source of fluid secretion including CSF and specialized protein secretions such as from the subcommissural organ (to produce Reissner’s fiber), (c) as an organ for sensing CSF composition especially via associated nerve fibers, and (d) as an organ of generation and regeneration. These were being considered particularly likely in lower vertebrates but conceivably also in man (Agduhr, 1932). Around the same time, physiologic studies on living animals were used to study CSF production by choroid plexus (Putnam and Ask-Upmark, 1934).
Central canal of the spinal cord
From the earliest descriptions, ependymal cells similar to those in the ventricles were known to line the central canal of the spinal cord (Virchow, 1854; Deecke, 1879). Generally, they were considered as a continuum, but some differences were noted. Proximity to a proteinaceous structure within the central canal was observed in the mid 1800s (Reissner, 1860; Sanders, 1878). This came to be known as the Reissner fiber, which originates as a secretion from the subcommissural organ at the cranial end of the cerebral aqueduct (Aboitiz and Montiel, 2021). Around 1900, interest grew in the proliferative capacity of spinal cord ependymal cells and/or closely related germinal cell populations. Hamilton described mitoses among ciliated ependymal cells in the lumbar cord of postnatal rats (Hamilton, 1901). Hooker (1915) speculated that ependymal cells play a role in frog spinal cord regeneration. However, it was not until the 1950s that interest and experimentation on this relationship in fish, amphibians, and reptiles expanded (Piatt, 1955; Anderson and Waxman, 1983). The extent to which ependymal/progenitor cells can contribute to recovery from spinal cord damage in mammals is the subject of recent research and is beyond the scope of this article (Marichal et al., 2017).
Streeter described regression of the ependymal cell layer during embryonic development of the human filum terminale (Streeter, 1919). Schultze (1882) provided histopathologic descriptions of syringomyelia (enlargement and disruption of the central canal) and obliteration of the central canal by ependymal cells. The latter situation was eventually recognized as a near unanimous state in adult humans (see section titled “Ependymal cell loss”).
Study of ependymal cells by electron microscopy
Owing to the limits of photon wavelengths, spatial resolution of light microscopy in the 20th century (up to 1,000× magnification) could not reveal much beyond the epithelial and ciliated nature of ependymal cells. Electron microscopy opened new avenues of research. Transmission electron microscopy (TEM), which uses electrons rather than photons, allows > 100,000× magnification. However, the techniques for preparation of biological samples were crude in the early 1950’s. TEM was initially used to demonstrate the “brush border” (microvilli) on mouse choroid plexus epithelial cells, which were easy to isolate. Microvilli were thought to be an absorptive organelle, so the investigators did not understand their role on secretory cells (Dalton et al., 1950, 1951). By the mid-1950’s, TEM methods had improved considerably and were increasingly used by numerous research groups for detailed studies of choroid plexus epithelial cells (Maxwell and Pease, 1956) and the demonstrations of microvilli, intercellular junctions, and basal lamina on ependymal cells (Luse, 1956; Pease, 1956; Brightman and Palay, 1963; Klinkerfuss, 1964; Malinsky, 1968; Leonhardt, 1972; Figure 7). Injection of ferritin particles into the CSF compartment showed that the ependymal layer serves at least a partial barrier to entry of molecules into brain parenchyma (Brightman, 1965).
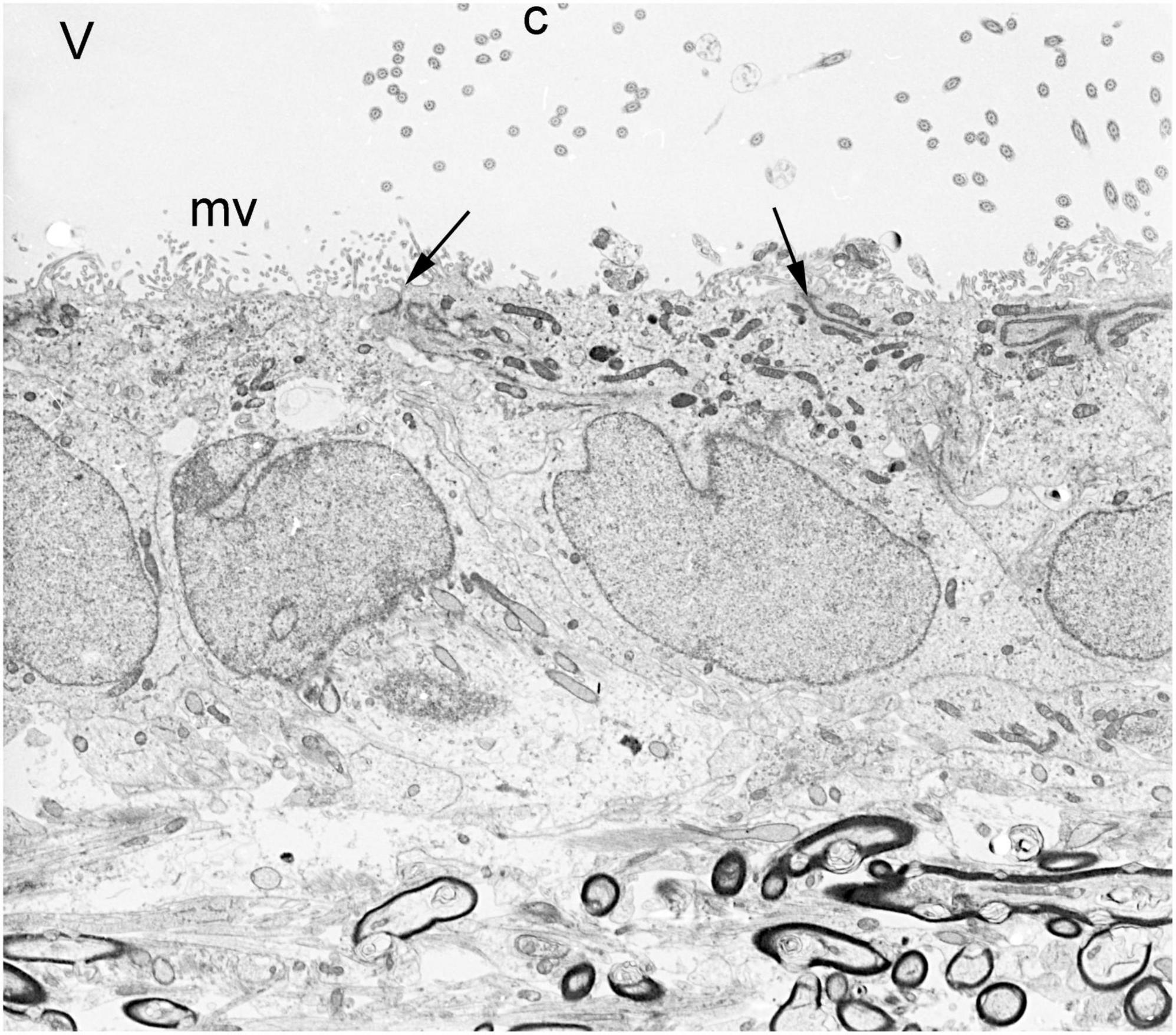
Figure 7. Transmission electron micrograph of normal ependymal cells lining the lateral ventricle of an adult rabbit brain. V = ventricle, c = cilia, mv = microvilli, arrows = apical junctional complexes, dark structures at bottom = myelinated axons of corpus callosum. Magnification 4,300×.
Transmission electron microscopy was typically used to study ependyma in a plane perpendicular to the ventricle surface, clearly showing how ependymal cells are organized with respect to their neighbors in the underlying glial layer. However, understanding the overall organization of the ventricle surface required some guess work. Uncertainty was mitigated by scanning electron microscopy (SEM), which conveys information about surface topology. Initially in 1965 SEM was applied to human lung tissue samples with suboptimal results (Jaques et al., 1965), but techniques improved rapidly. By the early to mid 1970’s SEM was used for elegant studies of the inner brain surfaces of large animals including humans (Bruni et al., 1972; Clementi and Marini, 1972; Noack et al., 1972; Scott et al., 1972; Weindl and Joynt, 1972; Allen and Low, 1973). Detailed studies of cilia distribution in normal and pathological conditions (especially hydrocephalus wherein the ventricles are enlarged and their surfaces stretched) were conducted (Borit and Sidman, 1972; Nielsen and Gauger, 1974; Page, 1975). The combined approaches of TEM and SEM allowed detailed investigations of the specialized circumventricular organs (Weindl, 1965), especially around the third and fourth ventricles (Fleischhauer, 1961; Bruni, 1974). Modern publications substantiate the utility of electron microscopy in the discrimination of subtle regional differences in these ependymal populations (Lorencova et al., 2020). Furthermore, SEM revealed the full extent of supraependymal axons and macrophages (Mitchell and Ham, 1998; Figure 8) (see below). SEM was frequently used for characterization of ventricular surfaces for about three decades, but since 2000 it has been used in only a few publications per year for very focused applications.
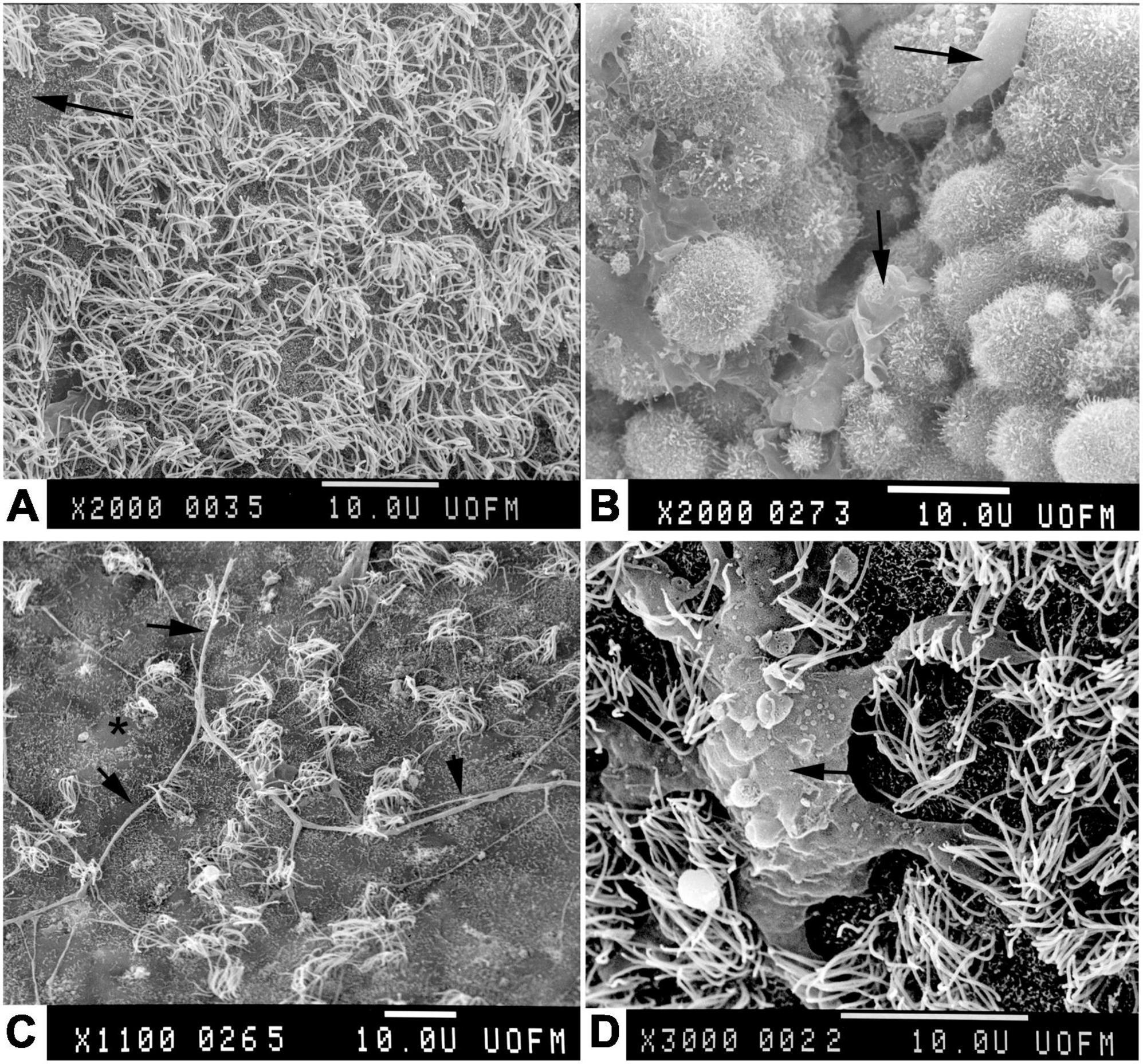
Figure 8. Scanning electron micrographs of adult rabbit brains. (A) Normal ventricle wall with normal density of apical cilia on ependyma. Where cilia are sparse, microvilli can be seen (arrow). (B) Normal choroid plexus epithelial cells with apical macrophages (Kolmer cells, arrows). Note the paucity of cilia in comparison to ependymal cells. (C) Ventricle wall of rabbit that had been hydrocephalic for 4 weeks. Note the sparsity of ependymal cilia, focal loss of microvilli (*), and the long supraependymal axon processes (arrows) (Del Bigio, 1987). (D) Supraependymal macrophage (arrow) with blunt processes on the ependymal surface of a rabbit that had an intraventricular implant for 3 days (Del Bigio and Bruni, 1986).
Ependymal cells in vitro
Hogue (1947) cultured explants of cerebellum from a 90 mm human fetus (approximately 15 weeks gestation) and observed that ependymal cilia remained active for at least 90 days in vitro. A decade later in separate labs, Friede and Hild grew ependymal cells in monolayers thereby allowing direct observation of cilia activity under a range of physiologic and pharmacologic conditions (Friede, 1955; Hild, 1957). However, it was not until the 1960’s onward that in vitro experiments became more frequently used for interrogation of ependymal cell properties including the developmental biology, permeability, and mechanics of cilia motion (Hild et al., 1965; Singer and Goodman, 1966; Dalen et al., 1971; Abney et al., 1981; Laabich et al., 1989; Delgehyr et al., 2015). Co-culture experiments showed that an underlying layer of astrocytes was important for differentiation of ependymal cells (Araki et al., 1983).
Role of ependymal cilia
As described in detail above, cilia were identified as the distinguishing feature of ependymal cells lining the ventricles of the brain. Speculation about their function began immediately. Purkinje and others assumed that ependymal cilia serve to move fluid or debris, much as in the airway (Purkinje, 1836). However, it was a century before direct hypothesis testing became possible. Work in the 1960’s showed coordinated cilia activity on explanted brain fragments indicating that they might create currents in CSF at least in proximity to the brain surface (Worthington and Cathcart, 1963; Dalen et al., 1971). In live frogs and rats, ependymal cilia were shown to move in the general anatomical direction of CSF flow and could rapidly move cell debris (Cathcart and Worthington, 1964; Worthington and Cathcart, 1966; Nelson and Wright, 1974). However, direct comparison with airway tissue showed that ependymal cilia beat more rapidly (about 30 cycles per second) are less efficient particle carriers (Roth et al., 1985). Recent work confirms the cilia beat gradients described decades earlier, but show that they establish local flow networks to preferentially distribute small molecules and that they do not contribute to bulk flow of CSF (Faubel et al., 2016). TEM was the mainstay for studying cilia structure for decades (Roy et al., 1974), but immunohistochemistry and immunofluorescence are now equally or more valuable through their ability to localize specific protein components.
Even in the absence of overt pathology, almost all ependymal cells are immunoreactive for ubiquitin (Kawanishi et al., 2003), which is a protein involved in degradation and recycling of proteins through the proteasome. This was initially thought to indicate that ependymal cells are subject to proteotoxic stresses not encountered by other brain cells (Kandel et al., 2023). However, the more likely explanation is that motile cilia proteins along with Foxj1 transcription factor have high turnover rates that require ubiquitin for recycling (Stephens, 1999; Long et al., 2015; Abdi et al., 2018; Hao et al., 2021).
Axons and nerves associated with the ependymal layer
Silver impregnation was used to label axons running alongside subependymal blood vessels of the human ventricular system in the 1930’s. Such axons have scattered projections into the ependymal layer (Deery, 1935; Frey and Stoll, 1947). These CSF-contacting neurons were studied extensively by Vigh and Vigh-Teichmann (1971, 1998) beginning in the 1960’s. Most are situated in the spinal cord, fourth ventricle, and third ventricle where, current data show, they serve as chemoreceptors (Wyart et al., 2023).
An apparently distinct set of intraventricular (supraependymal) axons runs on top of the ependymal layer (Figure 8). Beginning in the mid 1960’s Leonhardt and Lindner (1967) and Leonhardt and Lindemann (1973) as well as others conducted extensive morphological studies of these axons in experimental mammals (Alvarez-Morujo et al., 1992). During the 1970’s in rat experiments, they were shown by autoradiography and later by immunohistochemistry to originate in the raphe nucleus of the brainstem. These axons were postulated to release serotonin directly into the CSF (Aghajanian and Gallager, 1975; Chan-Palay, 1976; Tramu et al., 1983), but in 1979 synaptic contacts between serotoninergic axons and ependymal cells of the rat subcommissural organ were demonstrated (Mollgard and Wiklund, 1979). Soon after, supraependymal axons were demonstrated in the human brain (Richards et al., 1980). Interest in the direct effects of serotonin on ependymal cells began in the 1960s (Svanidze and Bregvadze, 1968; Jorge, 1977). Lorez (1976) hypothesized that serotonin regulates cilia activity; however, direct experimentation on the exposed rat fourth ventricle failed to prove so. Although previously shown in other cell types, it was not until 25 years later in 2001 that serotonergic stimulation of ependymal cilia beating frequency was shown directly in cell culture (Nguyen et al., 2001) (see section titled “Role of ependymal cilia”). Recent work suggests that supraependymal axons might also contribute to regulation of the subependymal stem cell niche (Tong et al., 2014).
Supraependymal macrophages
Microglial cells, which were first described c1920 by del Río-Hortega (1919, 1920), are the resident macrophage/immune cell population of the central nervous system. Distinct populations have been identified diffusely within the brain parenchyma, in perivascular compartments, and in the meninges. A discrete subependymal population was identified in macaque monkeys in 1937 (Dewulf, 1937) and described in great detail in rabbits in 1965 (Cammermeyer, 1965). SEM studies in the 1970’s showed a widespread population of cells adherent to the ventricle surface (Coates, 1973). Structural and functional studies showed that these too are a resident monocyte/macrophage population (Albrecht and Bleier, 1979) similar to (Kolmer) epiplexus cells that are located on the surfaces of choroid plexus epithelial cells (Allen, 1975; Schwarze, 1975; Figure 8).
In the 1980’s, experimental injections of peptides or induced inflammation (injection of bacillus Calmette-Guerin into cisterna magna) showed that the supraependymal cells originated from the periventricular blood vessels and could increase in quantity by enhanced extravasation or by local proliferation (Merchant and Merchant, 1980a,b; Saland et al., 1983, 1984). As elsewhere, the main role of these cells appears to be phagocytosis in response to periventricular cell injury, infection, or hemorrhage.
Ependymal cell loss
“Accidental” loss of ependymal cells was recognized as a common feature of human brains in the 1930’s (Agduhr, 1932). Johnson and Johnson (1972) reported that 65/100 adult brains had focal loss of ependyma from the lateral ventricle walls; in association there was “increased accumulation of glial fibers or loosening of glial fibers, or both.” There was no difference between young adults and the elderly “suggesting that the lesion dated from preadult life” (Johnson and Johnson, 1972). Dooling et al. (1977) studied the ependymal lining of 111 human fetuses. They showed that focal loss of ependyma becomes apparent in occipital horns of the lateral ventricles as early as 23 weeks gestation, several weeks after the germinal matrix involutes and ciliated ependymal cells first appear. Patchy loss of ependyma along the corpus callosum and in the temporal horn over the hippocampus appears by 25 weeks and progresses with advancing gestation (Dooling et al., 1977). My personal experience with 1000’s of brains is that gaps in the ependyma are ubiquitous (while perhaps not “normal”) along the human callosum and occipital horns and that they tend to become more widespread in the lateral ventricles with increasing age (Figure 9). Accompanying sites of ependyma loss are increases in the thickness of the periventricular astroglial layer. Experimental studies in rats showed that subependymal germinal cells proliferate and differentiate into astrocytes in this situation (Willis et al., 1976). In his excellent 1995 review paper, Sarnat addressed the matter of subependymal or subventricular gliosis extensively (Sarnat, 1995).
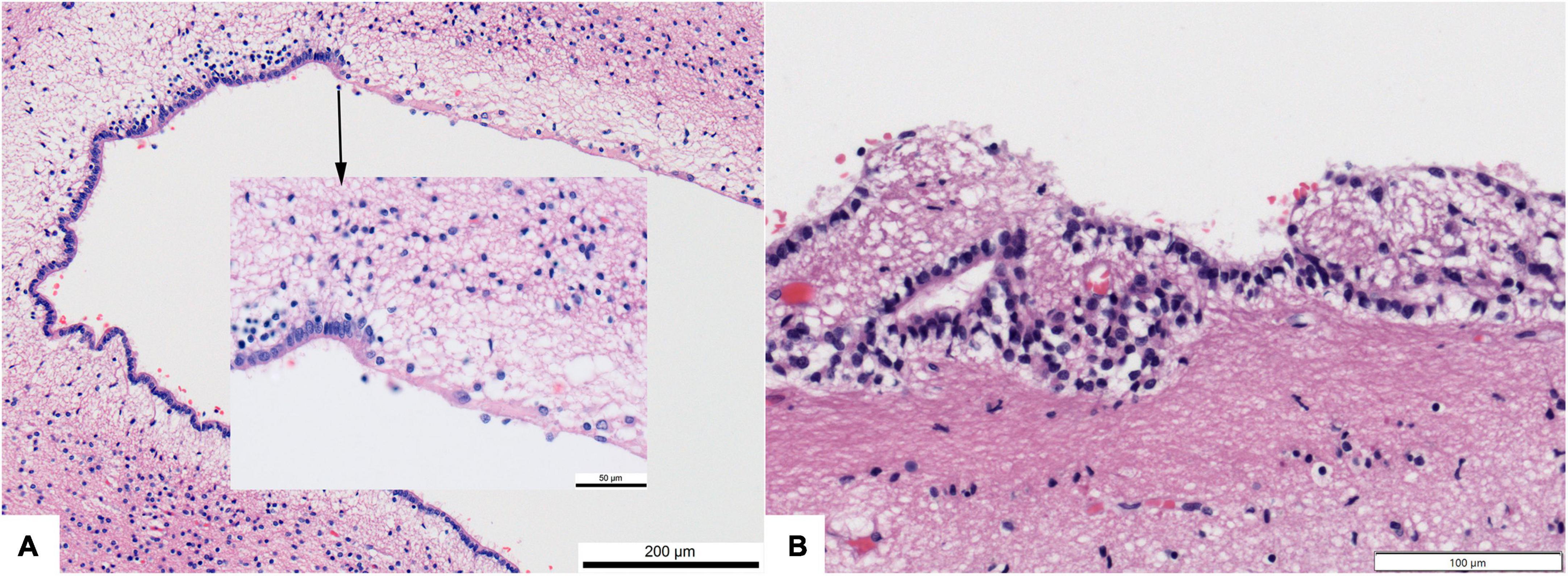
Figure 9. Photomicrographs showing regional loss of ependyma in otherwise normal human brains. (A) Dorsolateral angle of frontal horn of lateral ventricle in a 4 month infant (200× original magnification). Inset–transition to ependyma loss (600×). (B) Focal loss and buried islands of ependymal cells along the third ventricle of a 68 year old. All hematoxylin and eosin stain.
In the lateral ventricles of humans, patchy ependyma loss seems to be a benign phenomenon. Less often in association with developmental anomalies, regional adhesion of lateral ventricle walls occurs where ependymal cells are absent (Davidoff, 1946). In rodents, the apical surfaces of otherwise intact ependymal cells can become entangled such that the ventricular surfaces fuse (Sturrock, 1979).
Ependymal disruption and disorganization in the spinal cord is accompanied by obliteration of the central canal in almost all humans by the third decade (Agduhr, 1932; Kasantikul et al., 1979; Milhorat et al., 1994; Torrillas de la Cal et al., 2021).
Of greatest clinical significance is ependymal disorganization along the cerebral aqueduct. The aqueduct has a continuous ependymal lining in essentially all normal human brains, although there may be small diverticulae (Emery and Staschak, 1972; Alvarez et al., 1987). So-called “stenosis” of the cerebral aqueduct, which is potentially associated with severe enlargement of the lateral ventricles (hydrocephalus), was known to be associated with a disrupted ependymal cell layer by 1900 (Del Bigio, 1993). Integrity of the ependymal layer is necessary for maintenance of aqueduct patency in rodents (Paez et al., 2007) and ependyma loss followed by wall adhesion and glial “scarring” may be the cause of aqueduct narrowing (Del Bigio, 2014).
The cause of ependymal cell loss is not known with certainty in individual human cases, but compelling epidemiological and experimental evidence indicates that otherwise minor viral infections might be responsible. Beginning in the 1940’s, inoculation of experimental chicks and mice with influenza virus was shown to infect and destroy ependymal cells (Henle and Henle, 1946). Ependymal cells are susceptible to several other common viruses including mumps (Hamburger and Habel, 1947; Johnson et al., 1967) and parainfluenza (Mims and Murphy, 1973; Tardieu and Weiner, 1982). These viruses do not have a direct impact on other glial cell populations or neurons. Experimental reovirus infection in hamsters causes necrotizing ependymitis and obliteration of the spinal central canal (Milhorat and Kotzen, 1994). Since c1900 it has been known that degenerative ependymal changes also occur as a consequence of hydrocephalus during which stretched ependymal cells become flattened and cilia regress (Del Bigio, 1993). If ventriculomegaly occurs in the fetus, loss of ependyma coverage appears to compromise the underlying subventricular zone (Dominguez-Pinos et al., 2005) (see section titled “The relationship of ependymal cells to the brain’s germinal cell population”).
Gaps in the ependyma likely persist because mature ciliated cells do not readily proliferate following injury, as has been shown in rat brains (Bruni et al., 1983) and human spinal cord (Paniagua-Torija et al., 2018).
Barrier function
The concept of blood-brain, brain-CSF, and external meningeal physiologic barriers arose early in the twentieth century. Decades later in the 1960’s it became apparent from ventricular infusion studies that an intact ependymal layer could serve as a partial barrier capable of blocking passive movement of large molecules from the CSF into the brain parenchyma (Pollay and Davson, 1963; Pollay, 1967; Rall, 1968; Del Bigio, 1995b). Small molecules, ions (e.g., K+), and water move freely across the ependyma (Cserr, 1965). Robust barrier function would not be expected because ependymal cells are attached to each other by zonula adherens type junctions and not tight junctions (Brightman and Reese, 1969). It should be noted that the inter-ependymal junctional complexes are more complex in immature sheep brains when germinal matrix cells are still proliferating (Mollgard et al., 1987).
Many experiments with large animals in the 1970’s indicated that ventricle wall permeability to tracers is increased in association with hydrocephalus (Milhorat et al., 1970). The ependymal layer is attenuated and disrupted when the ventricles enlarge (Del Bigio, 1993). Considering that ventricular outflow of CSF is reduced, it is not clear if tracer flux changes indicate a true increase in permeability or more simply an altered fluid equilibrium.
Relationship of ependymal cells to the brain’s germinal cell population
It has been known for decades that ciliated ependymal cells differentiate at the ventricular surface as proliferation in the adjacent subependymal zone declines (Del Bigio, 2011). Studies of immature animals in the 1960’s showed that the periventricular germinal tissue begins as a poorly differentiated stratified cell layer and matures into a simple ciliated epithelium (Tennyson and Pappas, 1962). The biology of this transformation is understood only through very recent work (i.e., <20 years) and readers are referred to other sources (cited here). Briefly, several signaling mechanisms drive terminal differentiation of ependymal cells (Spassky et al., 2005; Kyrousi et al., 2017; Redmond et al., 2019; Stratton et al., 2019). Other writers address the complex debate about whether ciliated ependymal cells are themselves progenitors or if they act only in a supporting or protective role for the subependymal cell progenitor population (Stensaas and Gilson, 1972; Ohata and Alvarez-Buylla, 2016; Coletti et al., 2018; Shah et al., 2018).
Neoplasms of the ependyma (ependymoma and subependymoma)
The term “ependymoma” appeared c1898 (Benda, 1898) although periventricular brain tumors had been previously described (Mallory, 1902). Bailey cautioned in 1924 that most tumors called “ependymal gliomas” likely did not originate from ependymal cells and merely were located near the cerebral ventricles. He stated that “true ependymomas” should be “tumors originating from ependymal cells and more or less closely reproducing their structure” (Bailey, 1924). The classic ependymoma recapitulates some of the features of ependymal cells including intercellular junctions and cilia projecting into microlumens. Myxopapillary ependymoma, a morphologically distinct variant that arises from the filum terminale of the spinal cord, was identified in 1933 (Kernohan et al., 1933). A benign variant of periventricular ependymoma, the subependymoma, was recognized in 1945 (Scheinker, 1945). Dorothy Russell, one of the doyens of tumor neuropathology, called it a “dubious addition” (Russell, 1950) but it remains a legitimate entity in the classification of brain tumors (Louis et al., 2021). Knowledge of the genetics of ependymoma and possible origins from radial glial began to emerge in the mid 2000s (de Bont et al., 2008; Mack and Taylor, 2017) and have advanced rapidly since (Junger et al., 2021; Santi et al., 2022).
Summary and comments regarding relevance to human neuropathology
Research during the past two centuries had shed considerable understanding on the role of the ependyma, a simple and often ignored cell population in the brain. Neuropathologists and neurobiologists have published numerous good reviews concerning the ependymal structure and function in past decades (Bargmann et al., 1982; Bruni et al., 1985; Sarnat, 1992, 1995, 1998; Del Bigio, 1995a,b, 2010; Bruni, 1998; Moore, 2016; Nelles and Hazrati, 2022; Deng et al., 2023). Other reviews appear as chapters in books whose major subject is hydrocephalus (Vidovic et al., 2016). Readers are referred to these papers for more in depth considerations of some of the intermediate history.
In my work as a neuropathologist who examines human brains across the full age spectrum, what have I learned about the ependyma in normal brains? Usually it is present. Some regions, for example over the corpus callosum or in the occipital horns of the lateral ventricles, exhibit foci of ependymal attenuation or loss. These foci often have minor, focal chronic astroglial hyperplasia or buried islands of ependymal cells. In the circumstance of infection (e.g., ventriculitis) or intraventricular hemorrhage the ependymal layer is overtly and often widely disrupted. This can have serious consequences if the cerebral aqueduct becomes scarred, leading to hydrocephalus which, in turn, further disrupts the ependyma.
The enduring questions I have about ependyma in the context of clinical neuropathology are the following:
(1) Considering their relationship to periventricular germinal cells, are ependymal cells critical during brain development and merely vestigial in the adult?
(2) If gaps in ependymal coverage are ubiquitous, does this create a weakness in a brain barrier that should be targeted for intervention, or does it mean that in some regions of the ventricular system that these cells are not necessary after a certain developmental stage? Furthermore, to what extent does the thickened periventricular astroglial layer replace the function of the ependyma?
(3) During human development (or in the brains of small animals) do ependymal cilia serve to keep narrow CSF channels (i.e., the cerebral aqueduct) clear of debris, which might act as a nidus for scarring? Why does ciliary dyskinesia cause hydrocephalus in so many murine genetic disease models but in so few humans (Sakamoto et al., 2021)? To what extent are ependymal cilia critical in mature humans for microdistribution of small molecules within the ventricular system?
(4) Do we pay enough attention to “minor” viral illnesses and their possible effects on the brain?
(5) Do we understand enough about the resident supraependymal macrophage population and its responses to intraventricular hemorrhage or infection? In the context of hydrocephalus, does this population contribute to the foreign body type inflammatory reaction that is associated with surgical shunt systems used to treat hydrocephalus (Del Bigio, 1998)?
Finally, reflecting back on Virchow’s suggestion that the term ependyma should include the surface epithelium along with the underlying “connective tissue” (Virchow, 1863). I must disagree. Unless we clearly define the cell populations we talk and write about, subsequent generations of researchers might be confused unless they read the primary work in detail. Please reserve the term “ependyma” for the mature, simple ciliated epithelium of the central nervous system.
Author contributions
MD: Conceptualization, Writing – original draft, Writing – review and editing.
Funding
The author(s) declare that no financial support was received for the research, authorship, and/or publication of this article.
Conflict of interest
The author declares that the research was conducted in the absence of any commercial or financial relationships that could be construed as a potential conflict of interest.
Publisher’s note
All claims expressed in this article are solely those of the authors and do not necessarily represent those of their affiliated organizations, or those of the publisher, the editors and the reviewers. Any product that may be evaluated in this article, or claim that may be made by its manufacturer, is not guaranteed or endorsed by the publisher.
References
Abdi, K., Lai, C. H., Paez-Gonzalez, P., Lay, M., Pyun, J., and Kuo, C. T. (2018). Uncovering inherent cellular plasticity of multiciliated ependyma leading to ventricular wall transformation and hydrocephalus. Nat. Commun. 9:1655. doi: 10.1038/s41467-018-03812-w
Abney, E. R., Bartlett, P. P., and Raff, M. C. (1981). Astrocytes, ependymal cells, and oligodendrocytes develop on schedule in dissociated cell cultures of embryonic rat brain. Dev. Biol. 83, 301–310. doi: 10.1016/0012-1606(81)90476-0
Aboitiz, F., and Montiel, J. F. (2021). The enigmatic Reissner’s fiber and the origin of chordates. Front. Neuroanat. 15:703835. doi: 10.3389/fnana.2021.703835
Adair, T. S. (1896). The ependyma: an enquiry into its anatomy, physiology, and pathology. Edinburgh: University of Edinburgh.
Addison, A. (1862). On the pathological anatomy of the brain in insanity. J. Mental Sci. 8, 37–61. doi: 10.1192/bjp.8.41.37
Agduhr, E. (1932). “Chorioid [sic] plexus and ependyma,” in Cytology and Cellular Pathology of the Nervous System, ed. W. Penfield (New York, NY: Paul B. Hoeber Inc).
Aghajanian, G. K., and Gallager, D. W. (1975). Raphe origin of serotonergic nerves terminating in the cerebral ventricles. Brain Res. 88, 221–231. doi: 10.1016/0006-8993(75)90386-8
Albrecht, R. M., and Bleier, R. (1979). Histochemical, functional, and structural features of isolated adherent supraependymal cells: characterization as resident mononuclear phagocytes. Scan. Electron Microsc. 3, 55–61.
Allen, D. J. (1975). Scanning electron microscopy of epiplexus macrophages (Kolmer cells) in the dog. J Comp. Neurol. 161, 197–213. doi: 10.1002/cne.901610205
Allen, D. J., and Low, F. N. (1973). The ependymal surface of the lateral ventricle of the dog as revealed by scanning electron microscopy. Am. J. Anat. 137, 483–489. doi: 10.1002/aja.1001370410
Altschul, R. (1941). The growth of ependyma. J. Comp. Neurol. 75, 245–253. doi: 10.1002/cne.900750205
Alvarez, L. A., Kato, T., Llena, J. F., and Hirano, A. (1987). Ependymal foldings and other related ependymal structures in the cerebral aqueduct and fourth ventricle of man. Acta Anat. 129, 305–309. doi: 10.1159/000146420
Alvarez-Morujo, A. J., Toranzo, D., Blazquez, J. L., Pelaez, B., Sanchez, A., Pastor, F. E., et al. (1992). The ependymal surface of the fourth ventricle of the rat: a combined scanning and transmission electron microscopic study. Histol. Histopathol. 7, 259–266.
Anderson, M. J., and Waxman, S. G. (1983). Regeneration of spinal neurons in inframammalian vertebrates: morphological and developmental aspects. J. Hirnforsch. 24, 371–398.
Araki, M., Sato, F., and Saito, T. (1983). Primary monolayer culture of rat ependymal cells: an ultrastructural study. Arch. Histol. Jpn. 46, 191–201. doi: 10.1679/aohc.46.191
Arnold, J. G. (1934). Primary ependymitis: subacute type with occlusion of the foramina of monro and hydrocephalus of the lateral ventricles. Arch. Neurol. Psychiatr. 32, 143–153. doi: 10.1001/archneurpsyc.1934.02250070149008
Bailey, P. (1924). A study of tumors arising from ependymal cells. Arch. Neurol. Psychiatr. 11, 1–27. doi: 10.1001/archneurpsyc.1924.02190310007001
Baird, H. (1910). Ependymal alterations in general paralysis. J. Mental Sci. 56, 89–95. doi: 10.1192/bjp.56.232.89
Bargmann, W., Oksche, A., Fix, J. D., Adams, R. D., and Haymaker, W. (1982). “Meninges, choroid plexuses, ependyma, and their reactions (Chapter 7). Part 1. Histology and functional considerations. Part 2. Age-related changes and pathology,” in Histology and Histopathology of the Nervous System, eds W. Haymaker and R. D. Adams (Springfield IL: Charles C. Thomas).
Beadles, C. (1895). On the degenerative lesions of the arterial system in the insane, with remarks upon the nature of granular ependyma. J. Ment. Sci. 41, 32–50. doi: 10.1192/bjp.41.172.32
Benda, C. (1898). Pathologisch-anatomische Bemerkungen zu dem zweiten vorstehenden Fall A. Fraenkel’s. Deutsche Med. Wochensch. 24, 476–478. doi: 10.1055/s-0028-1139190
Blachford, J. (1903). Frequency of occurrence of granular ependyma in general paralysis. J. Mental Sci. 49, 483–486. doi: 10.1192/bjp.49.206.483
Bock, C. E. (1840). “Handbuch der Anatomie des Menschen mit Berücksichtigung der Physiologie und chirurgischen Anatomie,” in Enthält: Nerven-und Eingeweidelehre; topographische Anatomie, (Leipzig Germany: Friedrich Volckmar).
Borit, A., and Sidman, R. L. (1972). New mutant mouse with communicating hydrocephalus and secondary aqueductal stenosis. Acta Neuropathol. 21, 316–331. doi: 10.1007/BF00685139
Brightman, M. W. (1965). The distribution within the brain of ferritin injected into cerebrospinal fluid compartments. I. Ependymal distribution. J. Cell Biol. 26, 99–123. doi: 10.1083/jcb.26.1.99
Brightman, M. W., and Palay, S. L. (1963). The fine structure of ependyma in the brain of the rat. J. Cell Biol. 19, 415–439. doi: 10.1083/jcb.19.2.415
Brightman, M. W., and Reese, T. S. (1969). Junctions between intimately apposed cell membranes in the vertebrate brain. J. Cell Biol. 40, 648–677. doi: 10.1083/jcb.40.3.648
Bruni, J. E. (1974). Scanning and transmission electron microscopy of the ependymal lining of the third ventricle. Can. J. Neurol. Sci. 1, 59–73. doi: 10.1017/S0317167100019570
Bruni, J. E. (1998). Ependymal development, proliferation, and functions: a review. Microsc. Res. Tech. 41, 2–13. doi: 10.1002/(SICI)1097-0029(19980401)41:1<2::AID-JEMT2>3.0.CO;2-Z
Bruni, J. E., Clattenburg, R. E., and Paterson, J. A. (1983). Ependymal cells of the rat fourth ventricle: response to injury. Scanning Electron Microsc. 2, 649–661.
Bruni, J. E., Del Bigio, M. R., and Clattenburg, R. E. (1985). Ependyma: normal and pathological. A review of the literature. Brain Res. 356, 1–19. doi: 10.1016/0165-0173(85)90016-5
Bruni, J. E., Montemurro, D. G., Clattenburg, R. E., and Singh, R. P. (1972). A scanning electron microscopic study of the ependymal surface of the third ventricle of the rabbit, rat, mouse and human brain. Anat. Rec. 174, 407–420. doi: 10.1002/ar.1091740402
Cammermeyer, J. (1965). The hypependymal microglia cell. Z. Anat. Entwicklungsgesch. 124, 543–561. doi: 10.1007/BF00520846
Cathcart, R. S. III, and Worthington, W. C. Jr. (1964). Ciliary movement in the rat cerebral ventricles: clearing action and directions of currents. J. Neuropathol. Exp. Neurol. 23, 609–618. doi: 10.1097/00005072-196410000-00002
Chan-Palay, V. (1976). Serotonin axons in the supra- and subependymal plexuses and in the leptomeninges; their roles in local alterations of cerebrospinal fluid and vasomotor activity. Brain Res. 102, 103–130. doi: 10.1016/0006-8993(76)90578-3
Clementi, F., and Marini, D. (1972). The surface fine structure of the walls of cerebral ventricles and of choroid plexus in cat. Z. Zellforsch. Mikrosk. Anat. 123, 82–95. doi: 10.1007/BF00337675
Coates, P. W. (1973). Supraependymal cells: light and transmission electron microscopy extends scanning electron microscopic demonstration. Brain Res. 57, 502–507. doi: 10.1016/0006-8993(73)90157-1
Coletti, A. M., Singh, D., Kumar, S., Shafin, T. N., Briody, P. J., Babbitt, B. F., et al. (2018). Characterization of the ventricular-subventricular stem cell niche during human brain development. Development 145:dev170100. doi: 10.1242/dev.170100
Cserr, H. (1965). Potassium exchange between cerebrospinal fluid, plasma, and brain. Am. J. Physiol. 209, 1219–1226. doi: 10.1152/ajplegacy.1965.209.6.1219
Dalen, H., Schlapfer, W. T., and Mamoon, A. (1971). Cilia on cultured ependymal cells examined by scanning electron microscopy. Exp. Cell Res. 67, 375–379. doi: 10.1016/0014-4827(71)90422-8
Dalton, A. J., Kahler, H., and Lloyd, B. J. (1951). The structure of the free surface of a series of epithelial cell types in the mouse as revealed by the electron microscope. Anat. Rec. 111, 67–77. doi: 10.1002/ar.1091110105
Dalton, A. J., Kahler, H., Striebich, M. J., and Lloyd, B. (1950). Finer structure of hepatic, intestinal and renal cells of the mouse as revealed by the electron microscope. J. Natl. Cancer Inst. 11, 439–461.
Davidoff, L. M. (1946). Coarctation of the walls of the lateral angles of the lateral cerebral ventricles. J. Neurosurg. 3, 250–256. doi: 10.3171/jns.1946.3.3.0250
de Bont, J. M., Packer, R. J., Michiels, E. M., den Boer, M. L., and Pieters, R. (2008). Biological background of pediatric medulloblastoma and ependymoma: a review from a translational research perspective. Neuro. Oncol. 10, 1040–1060. doi: 10.1215/15228517-2008-059
Deecke, T. (1879). On the epithelium of the central canal of the spinal cord and of the ventricles of the brain. J. Insanity 35, 433–444. doi: 10.1176/ajp.35.3.433
Del Bigio, M. R. (1987). Experimental hydrocephalus and cerebrospinal fluid shunting in rabbits. Winnipeg: University of Manitoba.
Del Bigio, M. R. (1993). Neuropathological changes caused by hydrocephalus. Acta Neuropathol. 85, 573–585. doi: 10.1007/BF00334666
Del Bigio, M. R. (1995b). The ependyma: a protective barrier between brain and cerebrospinal fluid. Glia 14, 1–13. doi: 10.1002/glia.440140102
Del Bigio, M. R. (1995a). Ependymal reactions to injury. A review. J. Neuropathol. Exp. Neurol. 54, 405–406.
Del Bigio, M. R. (1998). Biological reactions to cerebrospinal fluid shunt devices: a review of the cellular pathology. Neurosurgery 42, 319–325. doi: 10.1097/00006123-199802000-00064
Del Bigio, M. R. (2010). Ependymal cells: biology and pathology. Acta Neuropatho.l 119, 55–73. doi: 10.1007/s00401-009-0624-y
Del Bigio, M. R. (2011). Cell proliferation in human ganglionic eminence and suppression after prematurity-associated haemorrhage. Brain 134, 1344–1361. doi: 10.1093/brain/awr052
Del Bigio, M. R. (2014). “Neuropathology of human hydrocephalus,” in Adult Hydrocephalus, ed. D. Rigamonti (Cambridge: Cambridge University Press). doi: 10.1017/CBO9781139382816.003
Del Bigio, M. R., and Bruni, J. E. (1986). Reaction of rabbit lateral periventricular tissue to shunt tubing implants. J. Neurosurg. 64, 932–940. doi: 10.3171/jns.1986.64.6.0932
del Río-Hortega, P. (1919). Hortega: El “tercer elemento” de los centros nerviosos. I. La microglía en estado normal. Bol. Soc. Esp. Biol. 8, 67–82.
del Río-Hortega, P. (1920). Hortega: La microglia y su transformacion en células en bastoncito y cuerpos granulo-adiposos. Trab. Lab. Invest. Biol. Univ. Madrid 18, 37–82.
Delgehyr, N., Meunier, A., Faucourt, M., Bosch Grau, M., Strehl, L., Janke, C., et al. (2015). Ependymal cell differentiation, from monociliated to multiciliated cells. Methods Cell Biol. 127, 19–35. doi: 10.1016/bs.mcb.2015.01.004
Deng, S., Gan, L., Liu, C., Xu, T., Zhou, S., Guo, Y., et al. (2023). Roles of ependymal cells in the physiology and pathology of the central nervous system. Aging Dis. 14, 468–483.
Dewulf, A. (1937). La microglie normale chez le singe (Macacus rhesus). J. Belge Neurol. Psychiat. 37, 341–365.
Dominguez-Pinos, M. D., Paez, P., Jimenez, A. J., Weil, B., Arraez, M. A., Perez-Figares, J. M., et al. (2005). Ependymal denudation and alterations of the subventricular zone occur in human fetuses with a moderate communicating hydrocephalus. J. Neuropathol. Exp. Neurol. 64, 595–604. doi: 10.1097/01.jnen.0000171648.86718.bb
Dooling, E. C., Chi, J. G., and Gilles, F. H. (1977). Ependymal changes in the human fetal brain. Ann. Neurol. 1, 535–541. doi: 10.1002/ana.410010605
Emery, J. L., and Staschak, M. C. (1972). The size and form of the cerebral aqueduct in children. Brain 95, 591–598. doi: 10.1093/brain/95.3.591
Faubel, R., Westendorf, C., Bodenschatz, E., and Eichele, G. (2016). Cilia-based flow network in the brain ventricles. Science 353, 176–178. doi: 10.1126/science.aae0450
Fish, P. A. (1891). The epithelium of the brain cavities. Proc. Am. Soc. Microscopists 12, 140–145. doi: 10.2307/3220684
Fleischhauer, K. (1961). “Regional differences in the structure of the ependyma and subependymal layers of the cerebral ventricles of the cat,” in Regional Neurochemistry. The Regional Chemistry, Physiology, and Pharmacology of the Nervous System, eds S. S. Kety and J. Elkes (New York: Pergamon Press).
Frey, E., and Stoll, E. (1947). Über die Innervation des Ependyms. Vjschr. naturforsch. Ges. Zürich 92, 103–115.
Friede, R. (1955). [Studies of the scintillating ependyma in tissue culture]. Arch. Psychiatr. Nervenkr. Z. Gesamte Neurol. Psychiatr. 193, 295–302. doi: 10.1007/BF00412785
Friedreich, N. (1858). Einiges über die Structur der Cylinder-und Flimmerepithelien. Arch. pathol. Anat. Physiol. klin. Med. 15, 535–539. doi: 10.1007/BF01914889
Gierlich, J. (1936). Veränderungen am Ependym und den subependymären Zonen des Gehirns bei frischen stumpfen Kopfverletzungen. Dtsch. Z. ges. gerichtl. Med. 26, 100–129. doi: 10.1007/BF01749266
Haeckel, E. (1859). Beiträge zur normalen und pathologischen Anatomie der Plexus choroides. Virchows. Archiv. 16, 253–289. doi: 10.1007/BF01945135
Hamburger, V., and Habel, K. (1947). Teratogenetic and lethal effects of influenza-A and mumps viruses on early chick embryos. Proc. Soc. Exp. Biol. Med. 66, 608–617. doi: 10.3181/00379727-66-16172
Hamilton, A. (1901). The division of differentiated cells in the central nervous system of the white rat. J. Comp. Neurol. 11, 297–320. doi: 10.1002/cne.910110403
Hao, K., Chen, Y., Yan, X., and Zhu, X. (2021). Cilia locally synthesize proteins to sustain their ultrastructure and functions. Nat. Commun. 12:6971. doi: 10.1038/s41467-021-27298-1
Henle, G., and Henle, W. (1946). Studies on the toxicity of influenza viruses: I. The effect of intracerebral injection of influenza viruses. J. Exp. Med. 84, 623–637. doi: 10.1084/jem.84.6.623
Hild, W. (1957). Ependymal cells in tissue culture. Z. Zellforsch. Mikrosk. Anat. 46, 259–271. doi: 10.1007/BF00340099
Hild, W., Takenaka, T., and Walker, F. (1965). Electrophysiological properties of ependymal cells from the mammalian brain in tissue culture. Exp. Neurol. 11, 493–501. doi: 10.1016/0014-4886(65)90063-4
Hogue, M. J. (1947). Human fetal ependymal cells in tissue cultures. Anat. Rec. 99, 523–529. doi: 10.1002/ar.1090990407
Hooker, D. (1915). Studies on regeneration in the spinal cords. I. An analysis of the processes leading to its reunion after it has been completely severed in frog embryos at the stage of closed neural folds. J.. Comp. Neurol. 25, 469–495. doi: 10.1002/cne.900250503
Jaques, W. E., Coalson, J., and Zervins, A. (1965). Application of the scanning electron microscope to human tissues. A preliminary study. Exp. Mol. Pathol. 4, 576–580. doi: 10.1016/0014-4800(65)90038-9
Johnson, K. P., and Johnson, R. T. (1972). Granular ependymitis. Occurrence in myxovirus infected rodents and prevalence in man. Am. J. Pathol. 67, 511–526.
Johnson, R. T., Johnson, K. P., and Edmonds, C. J. (1967). Virus-induced hydrocephalus: development of aqueductal stenosis in hamsters after mumps infection. Science 157, 1066–1067. doi: 10.1126/science.157.3792.1066
Jorge, L. R. (1977). Morphological evidence for possible functional role of supra-ependymal nerves on ependyma. Brain Res. 125, 362–368. doi: 10.1016/0006-8993(77)90631-X
Junger, S. T., Timmermann, B., and Pietsch, T. (2021). Pediatric ependymoma: an overview of a complex disease. Childs Nerv. Syst. 37, 2451–2463. doi: 10.1007/s00381-021-05207-7
Kandel, R., Jung, J., and Neal, S. (2023). Proteotoxic stress and the ubiquitin proteasome system. Semin. Cell. Dev. Biol. doi: 10.1016/j.semcdb.2023.08.002 [Epub ahead of print].
Kasantikul, V., Netsky, M. G., and James, A. E. Jr. (1979). Relation of age and cerebral ventricle size to central canal in man. Morphological analysis. J. Neurosurg. 51, 85–93. doi: 10.3171/jns.1979.51.1.0085
Kawanishi, R., Mizutani, T., Yamada, H., Minami, M., Kakimi, S., Yamada, T., et al. (2003). Ubiquitin-positive inclusions in ependymal cells. Acta Neuropathol. 106, 129–136. doi: 10.1007/s00401-003-0712-3
Kernohan, J. W., Woltman, H. W., and Adson, A. W. (1933). Gliomas arising from the region of the cauda equina: clinical, surgical and histologic considerations. Arch. Neurol. Psychiatr. 29, 287–307. doi: 10.1001/archneurpsyc.1933.02240080077007
Klinkerfuss, G. H. (1964). An electron microscopic study of the ependyma and subependymal glia of the lateral ventricle of the cat. Am. J. Anat. 115, 71–97. doi: 10.1002/aja.1001150106
Kolliker, A. (1854). Manual of Human Microscopical Anatomy. Philadelphia: Lippincott, Grambo & Co. doi: 10.5962/bhl.title.20694
Kyrousi, C., Lygerou, Z., and Taraviras, S. (2017). How a radial glial cell decides to become a multiciliated ependymal cell. Glia 65, 1032–1042. doi: 10.1002/glia.23118
Laabich, A., Sensenbrenner, M., and Delaunoy, J. P. (1989). Monolayer cultures of ependymal cells on porous bottom dishes. A tool for transport studies across the brain cerebrospinal barrier. Neurosci. Lett. 103, 157–161. doi: 10.1016/0304-3940(89)90568-5
Leonhardt, H. (1972). [Electron microscopical differences between subependymal labyrinths of basal membrane in the human, in the rat, and in the rabbit]. Verh. Anat. Ges. 67, 605–607.
Leonhardt, H., and Lindemann, B. (1973). [A supraependymal nerve cell-, axon- and glia cell-system. A study on the 4th ventricle (apertura lateralis) with scanning- and transmission-electron microscopy in rabbit brain]. Z. Zellforsch. Mikrosk. Anat. 139, 285–302. doi: 10.1007/BF00306527
Leonhardt, H., and Lindner, E. (1967). [Nonmyelinated fibers in the 3d and 4th ventricle of the rabbit and cat brain]. Z. Zellforsch. Mikrosk. Anat. 78, 1–18. doi: 10.1007/BF00344399
Long, H., Wang, Q., and Huang, K. (2015). Ciliary/flagellar protein ubiquitination. Cells 4, 474–482. doi: 10.3390/cells4030474
Lorencova, M., Mitro, A., Jurikova, M., Galfiova, P., Mikusova, R., Krivosikova, L., et al. (2020). Ependymal cells surface of human third brain ventricle by scanning electron microscopy. Bratisl. Lek. Listy 121, 437–443. doi: 10.4149/BLL_2020_071
Lorez, H. P. (1976). Do supra-ependymal serotonergic (5-HT) nerves regulate ciliary activity in the rat brain? Experientia 32:802.
Louis, D. N., Perry, A., Wesseling, P., Brat, D. J., Cree, I. A., Figarella-Branger, D., et al. (2021). The 2021 WHO Classification of Tumors of the Central Nervous System: a summary. Neuro. Oncol. 23, 1231–1251. doi: 10.1093/neuonc/noab106
Luse, S. A. (1956). Electron microscopic observations of the central nervous system. J. Biophys. Biochem. Cytol. 2, 531–542. doi: 10.1083/jcb.2.5.531
Mack, S. C., and Taylor, M. D. (2017). Put away your microscopes: the ependymoma molecular era has begun. Curr. Opin. Oncol. 29, 443–447. doi: 10.1097/CCO.0000000000000411
Malinsky, J. (1968). Fine structure of ependyma in lateral ventricles of human brain. Acta Univers. Palack. Olomuc. 48, 65–72.
Mallory, F. B. (1902). Three gliomata of ependymal origin; two in the fourth ventricle, one subcutaneous over the coccyx. J. Med. Res. 8, 1–10.
Margulis, M. S. (1913). Ueber ependymäre Gliomatose der Hirnventrikel. Archiv. Psychiatr. Nervenkrankh. 50, 788–844. doi: 10.1007/BF02116900
Marichal, N., Reali, C., Rehermann, M. I., Trujillo-Cenoz, O., and Russo, R. E. (2017). Progenitors in the ependyma of the spinal cord: a potential resource for self-repair after injury. Adv. Exp. Med. Biol. 1015, 241–264. doi: 10.1007/978-3-319-62817-2_13
Maxwell, D. S., and Pease, D. C. (1956). The electron microscopy of the choroid plexus. J. Biophys. Biochem. Cytol. 2, 467–474. doi: 10.1083/jcb.2.4.467
Merchant, R. E., and Merchant, L. H. (1980a). The cerebral ventricles of the dog. I. Ultrastructural features of supraependymal cells during the inflammatory response. Acta Neuropathol. 50, 97–102. doi: 10.1007/BF00692858
Merchant, R. E., and Merchant, L. H. (1980b). The cerebral ventricles of the dog. II. Evidence of a hematogenous origin of supraependymal cells during the inflammatory response. Acta Neuropathol. 50, 103–107. doi: 10.1007/BF00692859
Milhorat, T. H., Clark, R. G., Hammock, M. K., and McGrath, P. P. (1970). Structural, ultrastructural, and permeability changes in the ependyma and surrounding brain favoring equilibration in progressive hydrocephalus. Arch. Neurol. 22, 397–407. doi: 10.1001/archneur.1970.00480230015002
Milhorat, T. H., and Kotzen, R. M. (1994). Stenosis of the central canal of the spinal cord following inoculation of suckling hamsters with reovirus type I. J. Neurosurg. 81, 103–106. doi: 10.3171/jns.1994.81.1.0103
Milhorat, T. H., Kotzen, R. M., and Anzil, A. P. (1994). Stenosis of central canal of spinal cord in man: incidence and pathological findings in 232 autopsy cases. J. Neurosurg. 80, 716–722. doi: 10.3171/jns.1994.80.4.0716
Mims, C. A., and Murphy, F. A. (1973). Parainfluenza virus Sendai infection in macrophages, ependyma, choroid plexus, vascular endothelium and respiratory tract of mice. Am. J. Pathol. 70, 315–328.
Mitchell, J. A., and Ham, S. (1998). Scanning electron microscopy of central nervous system cerebrospinal-fluid-contacting surfaces: A bibliography (1963–1995). Microsc. Res. Tech. 41, 158–171. doi: 10.1002/(SICI)1097-0029(19980415)41:2<158::AID-JEMT4>3.0.CO;2-Q
Mollgard, K., Balslev, Y., Lauritzen, B., and Saunders, N. R. (1987). Cell junctions and membrane specializations in the ventricular zone (germinal matrix) of the developing sheep brain: a CSF-brain barrier. J. Neurocytol. 16, 433–444. doi: 10.1007/BF01668498
Mollgard, K., and Wiklund, L. (1979). Serotoninergic synapses on ependymal and hypendymal cells of the rat subcommissural organ. J. Neurocytol. 8, 445–467. doi: 10.1007/BF01214802
Moore, S. A. (2016). The spinal ependymal layer in health and disease. Vet. Pathol. 53, 746–753. doi: 10.1177/0300985815618438
Mortazavi, M. M., Adeeb, N., Griessenauer, C. J., Sheikh, H., Shahidi, S., Tubbs, R. I., et al. (2014). The ventricular system of the brain: a comprehensive review of its history, anatomy, histology, embryology, and surgical considerations. Childs Nerv. Syst. 30, 19–35. doi: 10.1007/s00381-013-2321-3
Nelles, D. G., and Hazrati, L. N. (2022). Ependymal cells and neurodegenerative disease: outcomes of compromised ependymal barrier function. Brain Commun. 4:fcac288. doi: 10.1093/braincomms/fcac288
Nelson, D. J., and Wright, E. M. (1974). The distribution, activity, and function of the cilia in the frog brain. J. Physiol. 243, 63–78. doi: 10.1113/jphysiol.1974.sp010742
Nguyen, T., Chin, W. C., O’Brien, J. A., Verdugo, P., and Berger, A. J. (2001). Intracellular pathways regulating ciliary beating of rat brain ependymal cells. J. Physiol. 531, 131–140. doi: 10.1111/j.1469-7793.2001.0131j.x
Nielsen, S. L., and Gauger, G. E. (1974). Experimental hydrocephalus: surface alterations of the lateral ventricle. Scanning electron microscopic studies. Lab. Invest. 30, 618–625.
Noack, W., Dumitrescu, L., and Schweichel, J. U. (1972). Scanning and electron microscopical investigations of the surface structures of the lateral ventricles in the cat. Brain Res. 46, 121–129. doi: 10.1016/0006-8993(72)90010-8
Ohata, S., and Alvarez-Buylla, A. (2016). Planar organization of multiciliated ependymal (E1) cells in the brain ventricular epithelium. Trends Neurosci. 39, 543–551. doi: 10.1016/j.tins.2016.05.004
Paez, P., Batiz, L. F., Roales-Bujan, R., Rodriguez-Perez, L. M., Rodriguez, S., Jimenez, A. J., et al. (2007). Patterned neuropathologic events occurring in hyh congenital hydrocephalic mutant mice. J Neuropathol. Exp. Neurol. 66, 1082–1092. doi: 10.1097/nen.0b013e31815c1952
Page, R. B. (1975). Scanning electron microscopy of the ventricular system in normal and hydrocephalic rabbits. Preliminary report and atlas. J. Neurosurg. 42, 646–664. doi: 10.3171/jns.1975.42.6.0646
Paniagua-Torija, B., Norenberg, M., Arevalo-Martin, A., Carballosa-Gautam, M. M., Campos-Martin, Y., Molina-Holgado, E., et al. (2018). Cells in the adult human spinal cord ependymal region do not proliferate after injury. J. Pathol. 246, 415–421. doi: 10.1002/path.5151
Pease, D. C. (1956). Infolded basal plasma membranes found in epithelia noted for their water transport. J Biophys. Biochem. Cytol. 2, 203–208. doi: 10.1083/jcb.2.4.203
Peaslee, E. R. (1957). Human Histology in its Relations to Descriptive Anatomy, Physiology, and Pathology. Philadelphia: Blanchard and Lea.
Piatt, J. (1955). Regeneration of the spinal cord in the salamander. J. Exp. Zool. 129, 177–207. doi: 10.1002/jez.1401290109
Pollay, M. (1967). The processes affecting the distribution of bromide in blood, brain, and cerebrospinal fluid. Exp. Neurol. 17, 74–85. doi: 10.1016/0014-4886(67)90123-9
Pollay, M., and Davson, H. (1963). The passage of certain substances out of the cerebrosphinal fluid. Brain 86, 137–150. doi: 10.1093/brain/86.1.137
Purkinje, J. E. (1836). Ueber Flimmerbewegungen im Gehirn. Arch. Anat. Physiol. Wiss. Med. 183, 289–290.
Purkinje, J. E., and Valentin, G. (1835). Bemerkung über die Unabhängigkeit der Flimmerbewegungen der Wirbelthiere von der Integrität des centralen Nervensystems. Archiv. Anatom. Physiol. Wissenschaftl. Med. 1935, 159–160.
Purkinje, J. E., and Valentin, G. G. (1834). Entdeckung continuerlicher durch Wimperhaare erzeugter Flimmerbewegungen, als eines allgemeinen Phänomens in den Klassen der Amphibien. Vögel und Säugethiere. Arch Anat Physiol Wiss Med 1, 391–400.
Putnam, T. J., and Ask-Upmark, E. (1934). The cerebral circulation: XXIX. Microscopic observations on the living choroid plexus and ependyma of the cat. Arch. Neurol. Psychiatr. 32, 72–80. doi: 10.1001/archneurpsyc.1934.02250070078005
Rall, D. P. (1968). Transport through the ependymal linings. Prog. Brain Res. 29, 159–172. doi: 10.1016/S0079-6123(08)64154-0
Rand, C. W., and Courville, C. B. (1931). Histologic studies of the brain in cases of fatal injury to the head: II. Changes in the choroid plexus and ependyma. Arch. Surg. 23, 357–425. doi: 10.1001/archsurg.1931.01160090002001
Ranson, S. W. (1935). The Anatomy Of The Nervous System. From The Standpoint Of Development And Function, Fifth Edn. Philadelphia: W.B. Saunders Company.
Redmond, S. A., Figueres-Onate, M., Obernier, K., Nascimento, M. A., Parraguez, J. I., Lopez-Mascaraque, L., et al. (2019). Development of ependymal and postnatal neural stem cells and their origin from a common embryonic progenitor. Cell Rep. 27, 429–441. doi: 10.1016/j.celrep.2019.01.088
Reissner, E. (1860). Beiträge zur Kenntnis vom Bau des Rückenmarkes von Petromyzon fluviatilis L. Arch. Anat. Physiol.Wiss Med. 77, 545–588.
Richards, J. G., Lorez, H. P., Colombo, V. E., Guggenheim, R., and Kiss, D. (1980). Supraependymal nerve fibres in human brain: correlative transmission and scanning electron microscopical and fluorescence histochemical studies. Neuroscience 5, 1489–1502. doi: 10.1016/0306-4522(80)90011-1
Rokitansky, C. (1856). Lehrbuch der pathologischen Anatomie Specielle Pathologische Anatomie. Wien: Wilhelm Braumüller.
Roth, Y., Kimhi, Y., Edery, H., Aharonson, E., and Priel, Z. (1985). Ciliary motility in brain ventricular system and trachea of hamsters. Brain Res. 330, 291–297. doi: 10.1016/0006-8993(85)90688-2
Roy, S., Hirano, A., and Zimmerman, H. M. (1974). Ultrastructural demonstration of cilia in the adult human ependyma. Anat. Rec. 180, 547–550. doi: 10.1002/ar.1091800313
Russell, D. S. (1950). Book review: Pathology of Neurosurgery. Neurosurgical Pathology. By I. Mark Scheinker. Briet. Med. J. 1:770. doi: 10.1136/bmj.1.4656.770
Sakamoto, K., Nakajima, M., Kawamura, K., Nakamura, E., Tada, N., Kondo, A., et al. (2021). Ependymal ciliary motion and their role in congenital hydrocephalus. Childs Nerv. Syst. 37, 3355–3364. doi: 10.1007/s00381-021-05194-9
Saland, L. C., Ortiz, E., and Samora, A. (1984). Chronic infusion of opiate peptides to rat cerebrospinal fluid with osmotic minipumps. Anat. Rec. 210, 115–123. doi: 10.1002/ar.1092100114
Saland, L. C., Van Epps, D. E., Ortiz, E., and Samora, A. (1983). Acute injections of opiate peptides into the rat cerebral ventricle: a macrophage-like cellular response. Brain Res. Bull. 10, 523–528. doi: 10.1016/0361-9230(83)90150-8
Sanders, A. (1878). Contributions to the anatomy of the central nervous system vertebrate animals. Phil. Trans. Royal Soc. London 169, 735–776. doi: 10.1098/rstl.1878.0022
Santi, M., Viaene, A. N., and Hawkins, C. (2022). Ependymal tumors. Pediatr. Dev. Pathol. 25, 59–67. doi: 10.1177/10935266211018928
Sarnat, H. B. (1992). Role of the human fetal ependyma. Pediatr. Neurol. 8, 163–178. doi: 10.1016/0887-8994(92)90063-5
Sarnat, H. B. (1995). Ependymal reactions to injury. A review. J. Neuropathol. Exp. Neurol. 54, 1–15. doi: 10.1097/00005072-199501000-00001
Sarnat, H. B. (1998). Histochemistry and immunocytochemistry of the developing ependyma and choroid plexus. Microsc. Res. Tech. 41, 14–28. doi: 10.1002/(SICI)1097-0029(19980401)41:1<14::AID-JEMT3>3.0.CO;2-U
Scheinker, I. M. (1945). Subependymoma: a newly recognized tumor of subependymal derivation. J. Neurosurg. 2, 232–240. doi: 10.3171/jns.1945.2.3.0232
Schultze, F. (1882). Beiträge zur Pathologie und pathologischen Anatomie des centralen Nervensystems. Archiv. Pathol. Anat. Physiol. Klin. Med. 87, 510–540. doi: 10.1515/9783112404508-031
Schwarze, E. W. (1975). The origin of (Kolmer’s) epiplexus cells. A combined histomorphological and histochemical study. Histochemistry 44, 103–104. doi: 10.1007/BF00490425
Scott, D. E., Paull, W. K., and Dudley, G. K. (1972). A comparative scanning electron microscopic analysis of the human cerebral ventricular system. I. The third ventricle. Z. Zellforsch. Mikrosk. Anat. 132, 203–215. doi: 10.1007/BF00307011
Shah, P. T., Stratton, J. A., Stykel, M. G., Abbasi, S., Sharma, S., Mayr, K. A., et al. (2018). Single-cell transcriptomics and fate mapping of ependymal cells reveals an absence of neural stem cell function. Cell 173, 1045–1057. doi: 10.1016/j.cell.2018.03.063
Šimon, F. (2016). On the origin of the term ependyma. J. Hist. Neurosci. 25, 437–443. doi: 10.1080/0964704X.2015.1117894
Singer, I., and Goodman, S. J. (1966). Mammalian ependyma: some physico-chemical determinants of ciliary activity. Exp. Cell Res. 43, 367–380. doi: 10.1016/0014-4827(66)90064-4
Spassky, N., Merkle, F. T., Flames, N., Tramontin, A. D., Garcia-Verdugo, J. M., and Alvarez-Buylla, A. (2005). Adult ependymal cells are postmitotic and are derived from radial glial cells during embryogenesis. J. Neurosci. 25, 10–18. doi: 10.1523/JNEUROSCI.1108-04.2005
Stensaas, L. J., and Gilson, B. C. (1972). Ependymal and subependymal cells of the caudato-pallial junction in the lateral ventricle of the neonatal rabbit. Z. Zellforsch. Mikrosk. Anat. 132, 297–322. doi: 10.1007/BF02450711
Stephens, R. E. (1999). Turnover of tubulin in ciliary outer doublet microtubules. Cell Struct. Funct. 24, 413–418. doi: 10.1247/csf.24.413
Stratton, J. A., Shah, P., Sinha, S., Crowther, E., and Biernaskie, J. (2019). A tale of two cousins: Ependymal cells, quiescent neural stem cells and potential mechanisms driving their functional divergence. FEBS J. 286, 3110–3116. doi: 10.1111/febs.14930
Streeter, G. L. (1919). Factors involved in the formation of the filum terminale. Am. J. Anat. 25, 1–11. doi: 10.1002/aja.1000250102
Studnička, F. K. (1900). Untersuchungen über den Bau des Ependyms der nervösen Centralorgane. Anat. Hefte 15, 301–430. doi: 10.1007/BF02109682
Sturrock, R. R. (1979). A comparison of the processes of ventricular coarctation and choroid and ependymal fusion in the mouse brain. J. Anat. 129, 235–242.
Svanidze, I. K., and Bregvadze, I. A. (1968). Effect of neurotropic drugs on nucleic acid content of glial and nerve cells in tissue culture and on neuroglial motor activity. Neurosci. Translations 2, 977–984. doi: 10.1007/BF01124750
Tardieu, M., and Weiner, H. L. (1982). Viral receptors on isolated murine and human ependymal cells. Science 215, 419–421. doi: 10.1126/science.6276976
Tennyson, V. M., and Pappas, G. D. (1962). An electron microscope study of ependymal cells of the fetal, early postnatal and adult rabbit. Z. Zellforsch. Mikrosk. Anat. 56, 595–618. doi: 10.1007/BF00540584
Tong, C. K., Chen, J., Cebrian-Silla, A., Mirzadeh, Z., Obernier, K., Guinto, C. D., et al. (2014). Axonal control of the adult neural stem cell niche. Cell Stem Cell 14, 500–511. doi: 10.1016/j.stem.2014.01.014
Torrillas de la Cal, A., Paniagua-Torija, B., Arevalo-Martin, A., Faulkes, C. G., Jiménez, A. J., Ferrer, I., et al. (2021). The structure of the spinal cord ependymal region in adult humans is a distinctive trait among mammals. Cells 10:2235. doi: 10.3390/cells10092235
Tramu, G., Pillez, A., and Leonardelli, J. (1983). Serotonin axons of the ependyma and circumventricular organs in the forebrain of the guinea pig. An immunohistochemical study. Cell Tissue Res. 228, 297–311. doi: 10.1007/BF00204880
Valentin, G. (1836). Fortgesetzte Untersuchungen über die Flimmerbewegung. Repertor. Anatom. Physiol. 1, 148–159. doi: 10.1515/9783111441252-010
Vidovic, D., Piper, M., and Harvey, T. J. (2016). “Ependymal cells in development and disease,” in Hydrocephalus, Chap. 3, ed. M. Reeves (Hauppauge, NY: Nova Science Publishers, Inc).
Vigh, B., and Vigh-Teichmann, I. (1971). Structure of the medullo-spinal liquor-contacting neuronal system. Acta Biol. Acad. Sci. Hung. 22, 227–243.
Vigh, B., and Vigh-Teichmann, I. (1998). Actual problems of the cerebrospinal fluid-contacting neurons. Microsc. Res. Tech. 41, 57–83. doi: 10.1002/(SICI)1097-0029(19980401)41:1<57::AID-JEMT6>3.0.CO;2-R
Virchow, R. (1853). Ueber eine im Gehirn und Rückenmark des Menschen aufgefundene Substanz mit der chemischen Reaction der Cellulose. Arch. pathol. Anat. Physiol. klin. Med. 6, 135–138. doi: 10.1007/BF01930815
Virchow, R. (1854). On a substance presenting the chemical reaction of cellulose, found in the brain and spinal cord of man. J. Cell Sci. 1, 101–108. doi: 10.1242/jcs.s1-2.6.101
Virchow, R. (1858). Die Cellularpathologie in ihrer Begründung auf physiologische und pathologische Gewebelehre. Berlin: August Hirschwald.
Virchow, R. (1863). Cellular Pathology as Based Upon Physiological and Pathological Histology. Nutr. Rev. 47, 23–25. doi: 10.1111/j.1753-4887.1989.tb02747.x
Walther, K. (1897). Ueber chronische und akute Ependymentzündungen der Grosshirnventrikel. Wiesbaden: Albert Ludwigs Universitat.
Weindl, A. (1965). Zur Morphologie und Histochemie von Subfornicalorgan, Organum vasculosum laminae terminalis und Area postrema bei Kaninchen und Ratte. Z. Zellforsch Mikroskop Anat 67, 740–775. doi: 10.1007/BF00339300
Weindl, A., and Joynt, R. J. (1972). Ultrastructure of the ventricular walls. Three-dimensional study of regional specialization. Arch. Neurol. 26, 420–427. doi: 10.1001/archneur.1972.00490110054005
Wenzel, J., and Wenzel, K. (1806). Prodromus eines Werkes über das Hirn des Menschen und der Thiere. Tubingen: J.G. Cotta.
Willis, P., Berry, M., and Riches, A. C. (1976). Effects of trauma on cell production in the subependymal layer of the rat neocortex. Neuropathol. Appl. Neurobiol. 2, 377–388. doi: 10.1111/j.1365-2990.1976.tb00511.x
Worthington, W. C. Jr., and Cathcart, R. S. III (1963). Ependymal cilia: distribution and activity in the adult human brain. Science 139, 221–222. doi: 10.1126/science.139.3551.221
Worthington, W. C. Jr., and Cathcart, R. S. III (1966). Ciliary currents on ependymal surfaces. Ann. N. Y. Acad. Sci. 130, 944–950. doi: 10.1111/j.1749-6632.1966.tb12638.x
Keywords: brain, cerebrospinal fluid, cilia, cytology, ependyma, neuropathology, ventricular system
Citation: Del Bigio MR (2024) History of research concerning the ependyma: a view from inside the human brain. Front. Cell. Neurosci. 17:1320369. doi: 10.3389/fncel.2023.1320369
Received: 12 October 2023; Accepted: 08 December 2023;
Published: 08 January 2024.
Edited by:
Jo Anne Stratton, McGill University, CanadaReviewed by:
Adam Michael Russell Groh, McGill University, CanadaNicolas Guérout, Université de Rouen, France
Copyright © 2024 Del Bigio. This is an open-access article distributed under the terms of the Creative Commons Attribution License (CC BY). The use, distribution or reproduction in other forums is permitted, provided the original author(s) and the copyright owner(s) are credited and that the original publication in this journal is cited, in accordance with accepted academic practice. No use, distribution or reproduction is permitted which does not comply with these terms.
*Correspondence: Marc R. Del Bigio, marc.delbigio@umanitoba.ca