- 1Department of Laboratory Medicine, Affiliated Hangzhou First People’s Hospital, Zhejiang University School of Medicine, Hangzhou, Zhejiang, China
- 2School of Basic Medical Sciences and Forensic Medicine, Hangzhou Medical College, Hangzhou, Zhejiang, China
- 3Key Laboratory of Clinical Cancer Pharmacology and Toxicology Research of Zhejiang Province, Translational Medicine Research Center, Affiliated Hangzhou First People’s Hospital, Zhejiang University School of Medicine, Hangzhou, China
- 4Department of Cardiology, Affiliated Hangzhou First People’s Hospital, Zhejiang University School of Medicine, Hangzhou, Zhejiang, China
Obstructive sleep apnea (OSA) is a severe sleep disorder associated with intermittent hypoxia and sleep fragmentation. Cognitive impairment is a signifi- cant and common OSA complication often described in such patients. The most commonly utilized methods in clinical OSA treatment are oral appliances and continuous positive airway pressure (CPAP). However, the current therapeutic methods for improving cognitive function could not achieve the expected efficacy in same patients. Therefore, further understanding the molecular mechanism behind cognitive dysfunction in OSA disease will provide new treatment methods and targets. This review briefly summarized the clinical manifestations of cognitive impairment in OSA disease. Moreover, the pathophysiological molecular mechanism of OSA was outlined. Our study concluded that both SF and IH could induce cognitive impairment by multiple signaling pathways, such as oxidative stress activation, inflammation, and apoptosis. However, there is a lack of effective drug therapy for cognitive impairment in OSA. Finally, the therapeutic potential of some novel compounds and herbal medicine was evaluated on attenuating cognitive impairment based on certain preclinical studies.
1. Introduction
Obstructive sleep apnea (OSA) is a common sleep disorder characterized by intermittent hypoxia (IH) and sleep fragmentation (SF) due to upper airway collapse during sleep (Hoyos et al., 2017). Clinical studies indicate that OSA has a high incidence rate, with an estimated prevalence of 7% among adult men and 2–5% among adult women (Lumeng and Chervin, 2008). OSA patients have many symptoms related cognitive impairment, including spatial learning and memory impairment, executive function decline, and behavioral changes (Patel and Chong, 2021).
The most commonly used methods in the clinical treatment of OSA are oral appliances, surgery, and continuous positive airway pressure (CPAP) (Li et al., 2019; Toraldo et al., 2019). However, these methods have their shortcomings. First, surgical treatment must be strictly applied, as it needs to consider other factors, including apnea-hypopnea index (AHI), age, and mental state (Epstein et al., 2009). Second, although oral appliances and CPAP are commonly used practices for OSA therapy, it is ineffective in some patients. Some patients refuse to use them due to uncomfortable feelings (Carlucci et al., 2015). Finally, cognitive impairment or cognitive dysfunction could not be fully recovered in OSA patients through current therapy methods (Epstein et al., 2009). For example, one clinical trial described that most of scores neuropsychological tests for did not significantly improve after CPAP treatment in OSA patients (Bardwell et al., 2001). It is consistent with other RCTs demonstrating that CPAP treatment did not depict overall beneficial cognitive effects (Hui et al., 2000; Lim et al., 2007). Therefore, an in-depth understanding of the molecular mechanism of cognitive dysfunction in OSA disease will provide new treatment methods and targets. This article reviews the progress mechanism of cognitive dysfunction in OSA patients and summarizes some novel compounds and herbal medicine for treating cognitive impairment due to OSA.
2. Disease definition
Overnight polysomnography (PSG) is the standard diagnostic test for obstructive sleep apnea (St Louis, 2010). During PSG, electroencephalogram, electrooculogram, electromyogram, oronasal airflow, and oxyhemoglobin saturation can identify sleep stages, airflow, respiratory effort, body position, limb movements, ECG, and oxygen saturation. Whether the pharyngeal collapse is completely blocked or not, it is called sleep-related apnea and hypopnea, respectively (Jordan et al., 2014). An apnea is the complete cessation of airflow for at least 10 s. Hypopnea is defined as airflow reduction. “Obstructive” means breathing is frequently interrupted by upper airway obstruction, and more than 90% of airflow is reduced. AHI measures the number of apneas and hypopneas per hour of sleep to assess the severity of OSA disease. Based on the guidelines of the American Academy of Sleep Medicine (AASM), AHI < 5 indicates no disease, 5 ≤ AHI < 15 depicts a mild disease, 15 ≤ AHI < 30 represents a moderate disease, and AHI ≥ 30 characterizes a severe disease form (Muraja-Murro et al., 2014).
3. Symptoms of cognitive impairment in OSA
Obstructive sleep apnea is commonly associated with cognitive impairments, such as attention, verbal and visual episodic memory, and executive function (Sateia, 2003; Bucks et al., 2013). A meta-analysis revealed that vigilance, motor coordination, and executive functions were significantly impaired in OSA adults, whereas intelligence, verbal, and visual perceptual abilities were unaffected (Beebe et al., 2003). Some studies have characterized attention (Aloia et al., 2004; Bubu et al., 2020; Vanek et al., 2020), episodic memory, working memory, and executive functions (Olaithe and Bucks, 2013) as the most affected cognitive domains in OSA.
3.1. Attention
Attention refers to the psychological abilities of people to focus on relevant stimuli. Attention processing involves multiple aspects, such as reaction time, selective attention, and divided attention (Gagnon et al., 2014). These processes are associated with midline frontal areas and dorsolateral prefrontal cortices (Muller-Oehring and Schulte, 2014). Several studies have indicated that OSA subjects have attention impairment in all aspects (Aloia et al., 2004; Bubu et al., 2020; Vanek et al., 2020). For instance, OSA patients have more lapses and longer reaction times in tasks demanding sustained attention (Mazza et al., 2005; Gelir et al., 2014; Karimi et al., 2015) while significantly less reaction time after CPAP treatment (Djonlagic et al., 2015). Furthermore, according to the Test of Attentional Performance (TAP), OSA patients manifest deficits in divided and selective attention processes (Angelelli et al., 2020; Alkan et al., 2021).
3.2. Executive function
Executive function is an individually controlled and conscious effort to escort the operation of various cognitive processes. These include different cognitive abilities, such as concept formation, decision-making, mental flexibility, and problem-solving. A meta-analysis reported that executive functions across multiple tasks were impaired among OSA patients (Olaithe and Bucks, 2013).
Concept formation is a high cognitive function often operationalized as transferring the matching rule to new stimuli in a matching-to-sample task (Sukova et al., 2013). Concept formation is clinically assessed using the Wechsler Adult Intelligence Scale-Revised (WAIS-R) with these subtests: information, digit span, similarities, picture completion, block design, and digit symbol. WAIS-R demonstrated that OSA subjects had poorer scores than controls on block design, digit symbol, and picture completion (Saunamaki et al., 2009a,b, 2010). However, CPAP treatment did not significantly improve neuropsychological assessment (Saunamaki et al., 2009b,2010).
Decision-making is reaching decisions assessed with the Iowa Gambling Task (IGT), in which participants select cards from one of four decks. IGT characterized that scores were significantly lower in patients than in controls (Daurat et al., 2013). Furthermore, a higher rate of road traffic accidents was observed in OSA patients, impairing decision-making (Udholm et al., 2022). Moreover, OSA patients are inattentive, showing reduced reaction times on choice reaction tests (George, 2004) and decreased brain activation during an attention task involving decision-making leading to mistakes while driving (Ayalon et al., 2009).
Mental flexibility is an essential executive function underlying the ability to adapt to changing situations and respond to new information. Several investigations demonstrated a significant reduction of mental flexibility in OSA subjects (Verstraeten and Cluydts, 2004; Olaithe and Bucks, 2013). Meanwhile, the speed of mental flexibility was also enhanced after CPAP treatment (Dalmases et al., 2015).
Problem-solving is evaluating and selecting a sequence of actions to achieve a goal clinically assessed by tower test with more steps for OSA patients to solve problems (Naegele et al., 1995). Additionally, a deficit of executive functions in other aspects was observed in OSA patients, including easy impulsivity, reduced processing speed, and elevated perseverance.
3.3. Working memory
Working memory is the cognitive system temporarily maintaining and storing information, a short-term memory. Working memory impairment is always observed in OSA (Cosentino et al., 2008; Lau et al., 2015). Although the underlying mechanism is not fully classified, it could be related to the damage of frontoparietal connectivity since complete working memory tasks recruited a frontoparietal network of brain areas (Owen et al., 2005). A neuroimaging study revealed that the functional connectivity of the frontoparietal network showed abnormality in OSA patients (Liu et al., 2022).
3.4. Episodic memory
Episodic memory is remembering verbal or visual information in a space-time long-term memory. Multiple tasks could assess the ability of episodic memory, such as immediate recall, total recall for multiple steps or learning, delayed recall, free recall, and auditory task. OSA patients suffered impairment in free recall, delayed free recall, and transformed auditory span (Naegele et al., 2006). Moreover, based on the visuospatial episodic memory tasks results, there was a deficit in immediate and delayed recalls (Wallace and Bucks, 2013). Although CPAP improved the immediate and delayed memory performances, it could not ameliorate all the episodic memory components (Alchanatis et al., 2004; Bucks et al., 2013).
As discussed above, multiple cognitive ability was affected in OSA patients. However, OSA prevalence varied between 11 and 71% with cognitive impairment which was affected by OSA diagnostic methods. For example, the prevalence rates of cognitive impairment in OSA were 11, 27, 59, and 71%, respectively detected by self-report, home sleep apnea testing, Berlin questionnaire and polysomnography (Mubashir et al., 2019). Furthermore, the prevalence of cognitive impairment in OSA is related with other factors, such as severity of OSA, age and gender. Patients POSSESSING moderate to severe OSA had more severe sleep disturbances and a lower score on delayed recall test than the mild OSA group (Cai et al., 2023). Moreover, age is a significant risk factor for cognitive decline. Therefore, middle-aged OSA individuals are more likely to suffer cognitive impairment than younger ones with the similar severity of OSA (Alchanatis et al., 2008; Mathieu et al., 2008). Although several previous studies have assessed the gender-specific relationship between OSA and cognitive impairment, the conclusion need more consistency. One study described that female OSA patients had a higher risk of possessing poor prospective memory (Qiu et al., 2022). Meanwhile, OSA in women significantly reduced cortical and subcortical white matter than in men (Macey et al., 2012). However, another study indicated OSA men displayed had decreased power of extensive frequency range (sigma, beta and gamma) during sleep than in women, which plays a critical role in cognition formation (Munoz-Torres et al., 2020).
4. Pathophysiology of cognitive impairment in OSA
The causal mechanism of cognitive impairment remains debatable, and the existing literature has been primarily descriptive rather than based on well-defined theories. SF and intermittent blood gas abnormalities have been the most immediate physiological disturbances. They are associated with the exaggerated enhancement in upper airway resistance with sleep onset in OSA patients (Lin et al., 2019). Therefore, SF and IH are the two independent factors affecting cognitive function in OSA patients (Sforza and Roche, 2012).
4.1. Sleep fragmentation
Sleep fragmentation refers to sleep architecture disruption in OSA disease with poor sleep efficiency in OSA patients. This included a smaller proportion of sleep period time and reduced slow-wave sleep (SWS) (Walter et al., 2011). SF in OSA patients results in significant cognitive impairments, such as decreased mental flexibility, sustained attention, and spatial memory (Stepanski, 2002; Djonlagic et al., 2014). However, the underlying mechanisms remain poorly understood. The primary theory is that SF elicits oxidative stress and cellular damage (Shamsuzzaman et al., 2003). Since increased antioxidant activity promotes brain protection against free radicals during sleep, and wakefulness, reactive oxygen species (ROS) and other oxidative stress markers could be accumulated in the brain tissue (Mamelak, 2022). A study reported spatial learning deficits in mice exposed to SF by significantly activating oxidative stress. This could be associated with NADPH oxidase activity since mice without NADPH oxidase had normal learning after SF exposure (Nair et al., 2011b). NADPH oxidase is a vital source of generating intracellular ROS. SF could induce oxidative stress by activating NADPH oxidase to impair cognition and learning ability. The activity of nitric oxide synthase (iNOS), which regulate electron flow to enhance ROS production, was also increased in the SF model (Pandey and Kar, 2018). Then, oxidative stress results in cognitive impairment by inhibiting some neurotrophic factors expression and antioxidant genes, including BDNF and Nrf-2 (Zhang et al., 2013; Lee et al., 2022). Some synapse proteins, such as growth-associated protein 43 (GAP-43), post-synaptic density-95 (PSD-95), synapsin 1 (SYN-1), and synaptophysin (SYP), were also inhibited by SF-induced oxidative stress (Farajdokht et al., 2021).
Sleep fragmentation could also trigger an inflammatory response (Mishra et al., 2022). SF induced the expression of pro-inflammatory cytokines, such as IL-1 and TNF-α (Bertrand et al., 2020). On the other hand, long-term SF could cause vascular endothelial dysfunction by enhancing the recruitment of inflammatory cells and IL-6 expression (Carreras et al., 2014). In the SF model, multiple signaling pathways were responsible for pro-inflammatory cytokines expression, such as Toll-like receptor 4 (TLR4)/myeloid differentiation primary response protein 88 (MyD88) pathway (Xu et al., 2021b), TNF-α/NF-κB pathway (Zhang et al., 2022) and p38 MAPK pathway (Cui et al., 2019). One report indicated that SF could activate some microglial expression, vital in the inflammatory response (Kaneshwaran et al., 2019). Additionally, SF induced a selective increase in pro-inflammatory M1 macrophages by enhancing the NADPH oxidase 2 (NOX2) activity (Zhang et al., 2014).
Furthermore, SF significantly reduced rapid eye movement (REM) sleep in the SF rodent model, which is associated with impairing spatial learning and the losing the NMDA receptors (Tartar et al., 2006). Therefore, the loss of the NMDA receptor could be another underlying mechanism of inducing cognitive impairment in the SF model. SF could also disrupt neurotransmitter release, such as adenosine, monoamine, and dopamine (Ramesh et al., 1999; Proenca et al., 2014). Adenosine receptor antagonists could attenuate the decline in memory-induced sleep deprivation by increasing BDNF expression in the hippocampus region (Chauhan et al., 2016). Activating the dopaminergic D2 receptor helped counteract memory impairment in the sleep deprivation model (Proenca et al., 2014; Figure 1).
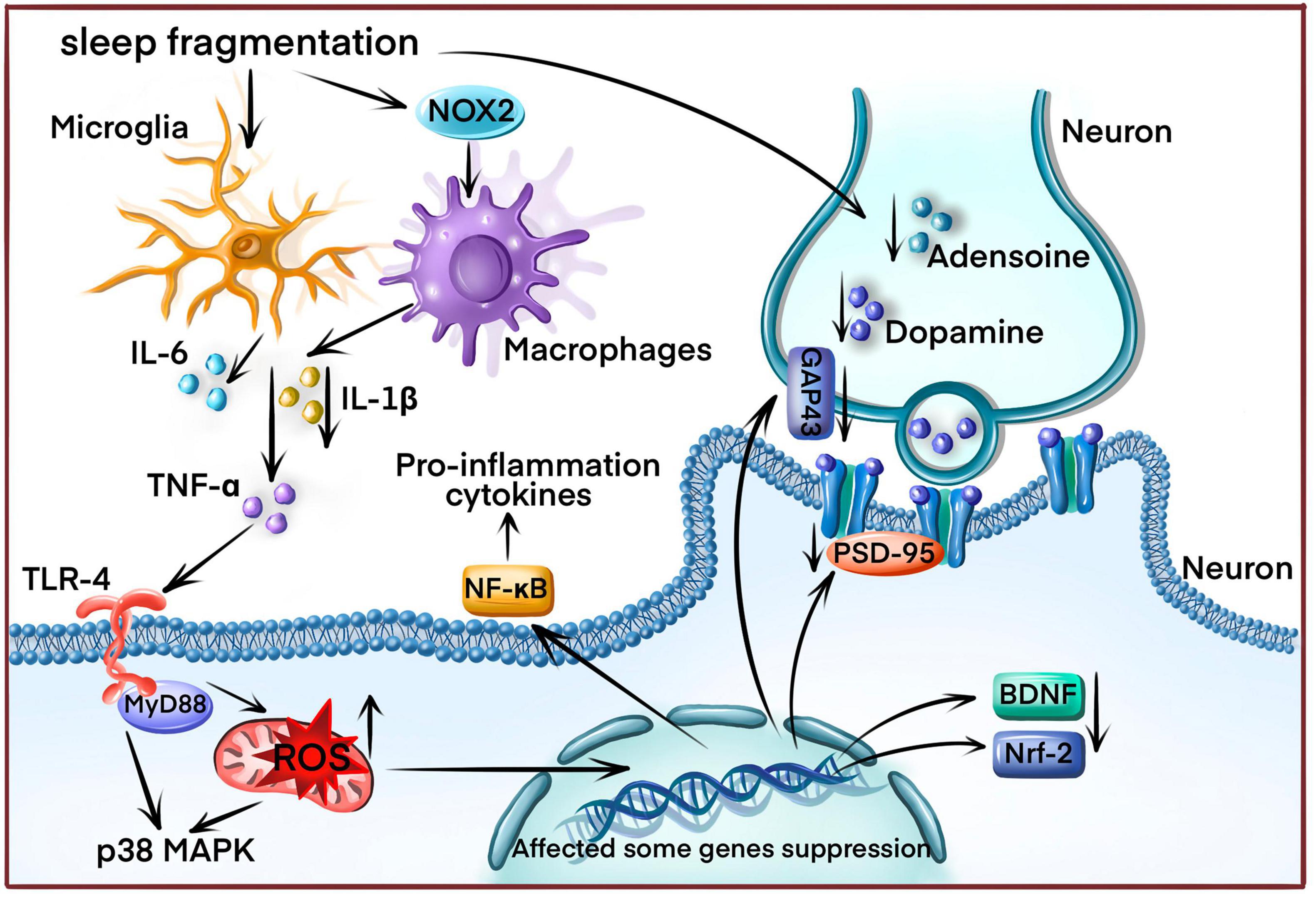
Figure 1. The underlying sleep fragmentation (SF) mechanism induces cognitive impairment in OSA disease. SF increases reactive oxygen species (ROS) production by enhancing NADPH oxidase activity, triggering the inflammatory response and releasing inflammatory cytokines (IL-1β, IL-6, TNF-α) from microglial and macrophages. ROS overproduction also affects various gene expressions, including neurotrophic factors, antioxidant genes, and synapse proteins. Finally, SF disrupts neurotransmitter release.
4.2. Intermittent hypoxia
Intermittent hypoxia (IH) is also a substantial variable associated with cognitive deficits (Dewan et al., 2015). However, IH and SF simultaneously occur in OSA, dissecting the influences of these two factors on cognitive functions, which is challenging in human subjects. An IH animal model was developed to assess the neurobehavioral effects of IH in the absence of SF, which many researchers widely accepted (Gozal et al., 2001; Row et al., 2002). Multiple mechanisms, including oxidative stress, inflammation, apoptosis, and reduction of neurotrophic factor, have been proposed to induce neurocognitive deficits due to IH (Figure 2).
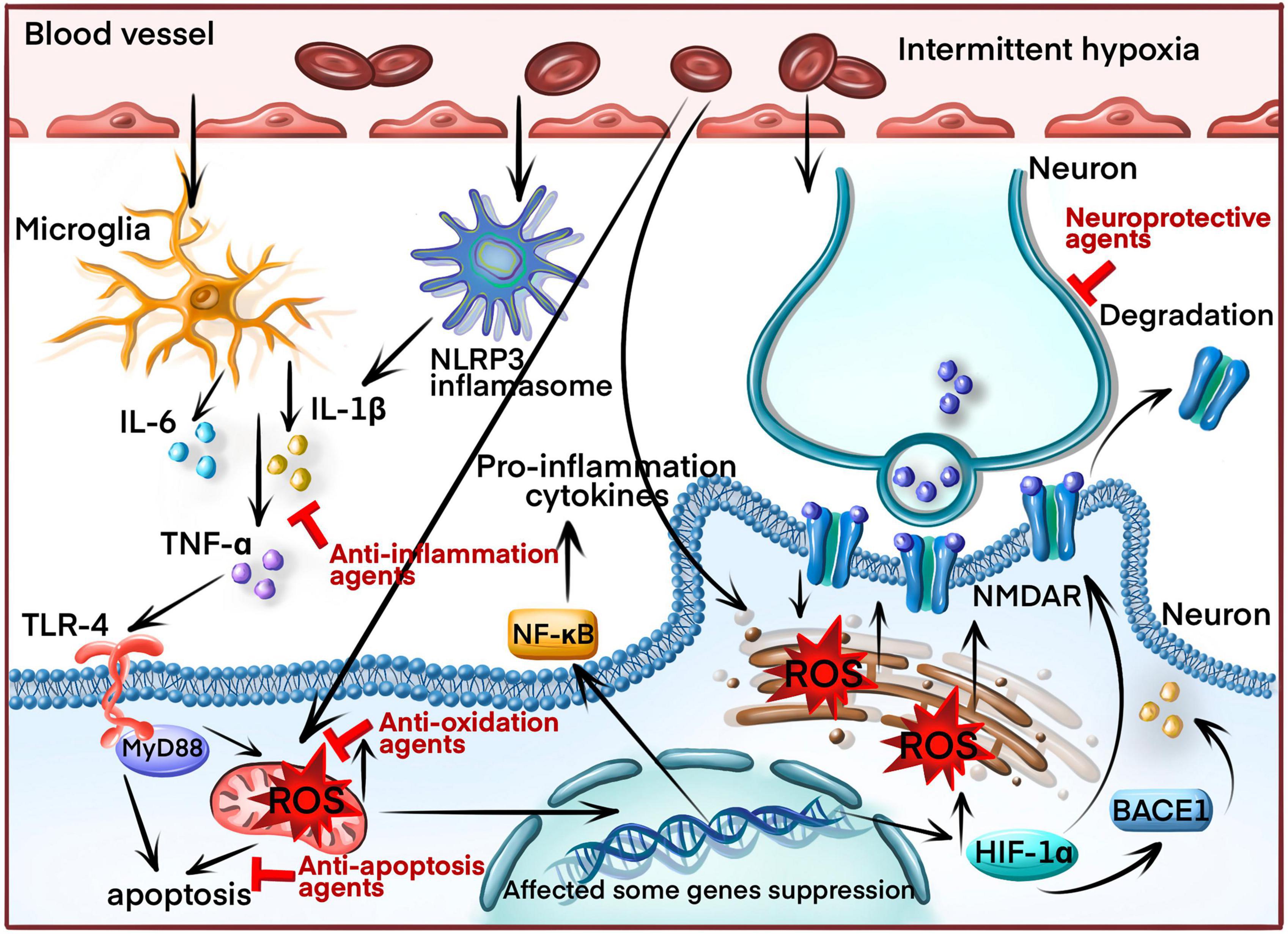
Figure 2. The molecular mechanism of inducing cognitive impairment in OSA patients through intermittent hypoxia (IH). IH increases reactive oxygen species (ROS) production and the nucleotide-binding domain-like receptor protein 3 (NLRP3) inflammasomes, triggering the inflammatory response and releasing inflammatory cytokines (IL-1β, IL-6, TNF-α) by activating the nuclear factor kappa B (NF-κB) signaling pathway. ROS overproduction induces apoptosis by causing mitochondrial damage and endoplasmic reticulum (ER) stress. Additionally, ROS overproduction induces the production of hypoxia-inducible factor-1α (HIF 1α) and beta-secretase 1 (BACE1). HIF-1α downregulates the N-methyl d-aspartate receptor (NMDAR) and BACE1, generating amyloid β (Aβ). Finally, the inflammatory cytokines aggravate neuronal axons, leading to synaptic damage. Blocking arrow indicated the molecular target by current therapeutic drugs.
4.2.1. Oxidative stress
Previous studies have demonstrated increased oxidative stress in OSA, including MDA and protein carbonyl upregulation (Xu et al., 2015), excessive lipid peroxidation production (Maniaci et al., 2021) and decline of reduced glutathione (Almendros et al., 2011). Therefore, inhibiting oxidative stress is a potential therapeutic target. Under IH conditions, ROS production, as indicated by oxidative stress, is elevated due to the reduced activity of oxidoreductases in mitochondrial respiration (Xu et al., 2020). Then, elevated ROS could affect some important gene expression, such as heme oxygenase-1 (HO-1), hypoxia-inducible factor-1α (HIF-1α), and NF-κB, which also aggravate oxidative stress (Lavie, 2012). For example, stabilizing HIF-1α by IH promotes ROS synthesis in mitochondria to induce apoptosis. The inhibition of HIF-1α can reduce neuronal apoptosis (da Rosa et al., 2015). In addition, Beta-secretase 1 (BACE1), as a primary agonist to generate amyloid β (Aβ), is activated by HIF-1α. Therefore, OSA patients were highly associated with Alzheimer’s (Andrade et al., 2018). Finally, increased HIF-1α production disrupts long-term potentiation (LTP) of the hippocampus and impaired spatial memory function by downregulating the N-methyl d-aspartate receptor (NMDAR) (Arias-Cavieres et al., 2020). Thus, HIF-1α could be a potential target for future OSA therapy.
Other than altering the expression of some genes, oxidative stress could cause mitochondrial dysfunction since ROS is primarily generated in mitochondria. In IH conditions, enhanced ROS production inhibits the electron transport chain activity in mitochondria and damages mitochondrial function (Prabhakar, 2011). However, suppressing ROS production rescued the mitochondrial morphology and function in the brain (Xu et al., 2015). A previous study observed a significant correlation between OSA severity and a significant decrease in mitochondrial DNA (mtDNA) copy number in OSA patients associated with oxidative stress (Kim et al., 2014). This finding is consistent with another study that revealed that mitochondrial bioenergetics are impaired in the frontal brain regions in OSA patients (Vakulin et al., 2022).
Furthermore, ER is another region partially producing ROS. Approximately 25% of ROS are derived from the ER and are required for oxidative protein folding (Gorlach et al., 2015). Furthermore, an oxidative environment favors protein folding, particularly the formation of disulfide bonds between two cysteine residues in proteins through thiol oxidation. Therefore, increased ROS production may lead to ER homeostasis loss and accumulation of misfolded proteins. This process is called ER stress (Mello et al., 2016). Moreover, additional synthesis of misfolded or unfolded proteins could deplete glutathione (GSH) due to ER stress (Tu and Weissman, 2002). After GSH is utilized, the oxidizing environment facilitates the reoxidation of protein thiols by interacting with protein disulfide isomerase (PDI) and endoplasmic reticulum oxidoreduction (ERO-1) (Bhandary et al., 2012). These steps lead to repetitive cycles of disulfide bond breakage and formation, with each process generating additional ROS as a byproduct (Higa and Chevet, 2012). This evidence strongly implies that ER stress and ROS could reciprocally activate each other under chronic IH conditions. Increased oxidative and ER stress levels were confirmed by our previous works, contributing to the impairment of learning and memory by inducing neuronal apoptosis (Xu et al., 2015, 2021a). Thus, decreasing oxidative stress could attenuate cognitive deficits induced by hypoxia.
4.2.2. Inflammation
A large number of inflammatory cytokines, such as interleukin (IL)-1, IL-6, IL-8, tumor necrosis factor-α (TNF-α), nuclear factor kappa B (NF-κB), etc., are activated in OSA patients (Liu et al., 2020). Although many factors could be implicated in the activation and progression of inflammation in OSA patients, a close relationship exists between inflammation and chronic IH (Dewan et al., 2015).
Hypoxia-inducible factor-1α, induced under IH condition, could increase NO synthesis by activating iNOS gene expression. NO is critical in initiating and regulating the inflammatory process (Abe et al., 2017). Then, excessive NO generation induced by IH could enhance neuronal apoptosis in the hippocampal CA1 region by generating lipid peroxidation (Yuan et al., 2015b). Moreover, the pro-inflammatory transcription factor NF-κB was enhanced in neutrophils and monocytes of OSA patients (Htoo et al., 2006). Meanwhile, IH treatment increased NF-kB expression in hippocampal neurons of rodent OSA model (Fei et al., 2021; Zhang C. Q. et al., 2021). However, the underlying mechanism was not elusive, with two significant explanations. One is that ROS could directly elevate NF-κB expression by activating the phosphorylation of IκBα and releasing p50 and RelA, binding to the DNA-binding domains of NF-κB and activating NF-κB transcription (Hayden and Ghosh, 2008). Another reason is that the dysregulation of leptin levels in OSA patients could increase the production of TNF-α, stimulating NF-κB activity (Berger and Polotsky, 2018).
How could these inflammatory cytokines aggravate cognitive deficits in chronic IH? There were two major theories to classify the inflammation mechanism leading to cognitive impairment under the IH condition. One is activating microglia-mediated neuroinflammation. Microglia, as inflammatory cells in the CNS, were also activated, leading to in neurocognitive and behavioral deficits caused by the IH of the animal model. IH exposure could significantly increase the density and morphological features of microglia, secreting cytokines such as IL-1β, IL-6, TNF-α, adhesion molecules, and other signaling mediators (Kiernan et al., 2016). These high cytokine levels produced by microglia can aggravate neuronal axon and synaptic damage, impairing the integrity of white matter across multiple brain regions (Hong et al., 2016). BDNF levels, crucial in neural plasticity, were decreased under IH conditions (Xie et al., 2010). Although pro-BDNF was partially released by microglia, pro-BDNF cannot change into BDNF during the persistent inflammatory phase to impair spatial memory performance (Mohammadi et al., 2020). Increasing BDNF expression can improve synaptic plasticity and decrease apoptosis caused by IH (Yin et al., 2015).
The mRNA levels of toll-like receptors-4 (TLR-4) were significantly upregulated by IH (Smith et al., 2013). Meanwhile, the monocytes from OSA patients significantly increased TLR-4 surface expression (Akinnusi et al., 2013). Therefore, TLR4 is an essential factor in IH-induced inflammation, produced by glial cells, and promotes inflammatory disorders. Glial cells are another significant category of cells activated by the IH condition (Liu et al., 2020). Then, the NF-κB signaling pathway can be activated by TLR4, enhancing the release of TNF-α and IL-1β. Moreover, TLR4 could bind with myeloid differentiation protein (MyD88) to induce cell apoptosis (Xue et al., 2017). Meanwhile, suppressing TLR4 expression could attenuate IH-induced neuronal apoptosis (Deng et al., 2015).
The nucleotide-binding domain-like receptor protein 3 (NLRP3) is a necessary inflammation interacting with procaspase-1 and apoptosis-associated speck-like protein (ASC) within the NLRP3 inflammation complex. Then, it leads to the release of caspase-1 and IL-1β (He et al., 2016). A recent study indicated that the NLRP3 inflammasome expression was increased in the brain tissue after IH treatment (She et al., 2022). Meanwhile, NLRP3 deletion elicited neuroprotection against IH treatment eliminating damaged mitochondria and reducing oxidative stress levels (Wu et al., 2021). Finally, inhibiting the NLRP3 inflammasome could suppress neuroinflammation and enhance cognitive function which was impaired by IH (Zhang et al., 2023). Therefore, NLRP3 inflammasome may be a potential target to ameliorate cognitive impairment.
4.2.3. Apoptosis
Many factors involved apoptosis under IH conditions, including oxidative stress, ER stress, and inflammation response (da Rosa et al., 2015; Deng et al., 2015; Xu et al., 2015, 2021a). However, there were also other signaling pathways involved in IH-induced apoptosis. For instance, cyclic AMP response element-binding protein (CREB) activity decreased in the hippocampal CA1 after IH exposure with increased cleaved caspases-3-positive cells. Meanwhile, enhanced phosphorylation of CREB could attenuate IH-induced neurocognitive impairments by suppressing neuronal apoptosis (Wang et al., 2015). Moreover, IH-induced autophagy attenuates apoptosis by activating AMP-activated protein kinase (AMPK) and enhancing the expression levels of Bax and cleaved caspase 3. Furthermore, 3-methyladenine, as an autophagy inhibitor, could suppress these alterations (Guo et al., 2021). More factors and signaling pathways would be associated with IH-induced apoptosis with further research.
5. Current drug therapies for neurocognitive dysfunction in OSA patients
Presently, some drugs are adjunctive therapy for treating OSA disease, achieving good efficacy in improving cognitive impairment (Table 1).
5.1. Modafinil
Modafinil is a novel wake-promoting agent that improves wakefulness in various clinical models. The American Academy of Sleep Medicine has recommended modafinil as a ‘golden standard’ treatment for this patient population (Littner et al., 2001). Modafinil is used as an adjunct therapy in OSA disease. A randomized, double-blind, placebo-controlled trial indicated that modafinil improved performance on behavioral alertness tests and reduced functional impairments in OSA patients assessed using the psychomotor vigilance task (PVT) and the Functional Outcomes of Sleep Questionnaire (Dinges and Weaver, 2003). Meanwhile, modafinil also reduces the incidence of adverse events, including headaches, nervousness (Pack et al., 2001), and daytime sleepiness (Schwartz et al., 2003; Bittencourt et al., 2008; Inoue et al., 2013). Although modafinil did not affect sleepiness measured by the Epworth Sleepiness Scale or the Multiple Sleep Latency Test, a significant improvement in alertness was observed on the Maintenance of Wakefulness Test (Kingshott et al., 2001).
5.2. Armodafinil
Armodafinil is the (R)-enantiomer of the wake-promoting compound modafinil, approved for treating excessive sleepiness, OSA, and shift work disorder (Nishino and Okuro, 2008). In this 12-week, randomized, double-blind study, armodafinil significantly enhanced episodic secondary memory, patient-estimated wakefulness, and decreased fatigue with fewer adverse events (Hirshkowitz et al., 2007). Furthermore, armodafinil enhanced simulated driving safety performance in OSA patients awaiting CPA therapy (Kay and Feldman, 2013). Although the underlying mechanism is elusive, one study indicated that it could be related to increased sleep latency (Roth et al., 2006). However, no significant differences were observed in armodafinil treatment for 2 weeks compared with the placebo group according to the 2-back working memory task. Meanwhile, the neuroimaging study also indicated that armodafinil could not improve functional magnetic resonance imaging (fMRI)-measured functional brain activation (Greve et al., 2014). Another clinical trial also described that 6 months of armodafinil treatment could not improve driving task performance but affected weight loss (Chapman et al., 2018). Therefore, armodafinil did not improve all the cognitive ability aspects.
5.3. Anti-inflammatory drugs
Inflammation is essential in cognitive impairment, with some anti-inflammatory drugs used in clinical trials. Though AHI and rhinorrhea symptoms in OSA patients significantly decreased after treatment with intranasal fluticasone propionate, a common corticosteroid, cognitive function was not assessed (Kiely et al., 2004; Segsarnviriya et al., 2021). Montelukast, a leukotriene receptor antagonist, also affects reducing AHI (Goldbart et al., 2012). Although fluticasone and montelukast did not decrease AHI in one study, total sleep time and percent of rapid eye movement (REM) sleep were significantly elevated (Smith et al., 2019). Additionally, intranasal budesonide, another effective anti-inflammatory drug, enhances sleep latency, SWS, and REM sleep among children (Kheirandish-Gozal and Gozal, 2008). However, these clinical trials did not reveal the effect of anti-inflammatory drugs on cognitive function, which needs further investigation.
5.4. Atomoxetine
Atomoxetine is a selective norepinephrine reuptake inhibitor reducing hypoglossal motoneuron excitability by blocking G-coupled inwardly rectifying the potassium channels (Taranto-Montemurro et al., 2017a). Oxybutynin is an antimuscarinic with mixed effects on suppressing exceeding nicotinic excitation (Liu et al., 2005). One study demonstrated that a combination of atomoxetine with oxybutynin could decrease the number of obstructive events, enhance the overnight oxygen desaturation, elevate the genioglossus muscle activity, and reduce AHI (Taranto-Montemurro et al., 2019, 2020). However, the effect of atomoxetine and oxybutynin on reducing cognitive function is still unknown.
5.5. Tiagabine
Tiagabine is a γ-aminobutyric acid (GABA) reuptake receptor inhibitor increasing GABA concentration at the synaptic level of the central nervous system. Tiagabine enhanced slow-wave activity (SWA) (Taranto-Montemurro et al., 2017b) with a crucial cognitive role (Wilckens et al., 2018). On the other hand, γ-hydroxybutyrate derived from GABA also increases SWS and REM sleep time (Series et al., 1992).
5.6. Desipramine
Desipramine is a common tricyclic antidepressant reducing the sleep-related loss of genioglossus activity and improving pharyngeal collapsibility (Taranto-Montemurro et al., 2016a). A placebo-controlled, double-blind, randomized trial described that desipramine could mitigate the sleep-related loss of muscle activity and AHI (Taranto-Montemurro et al., 2016b). There is direct evidence to enhance the protective effect on rescuing the cognition ability of OSA patients. However, some literature depicts that desipramine improves working memory (Clinton et al., 2006; Wang et al., 2016). The impact of desipramine on improving cognition ability in OSA requires further investigation. Furthermore, other neurological drugs, such as physostigmine and mirtazapine, could reduce the AHI in diabetes patients. However, these studies did not investigate whether these drugs could improve cognitive function (Hedner et al., 2003; Carley et al., 2007).
6. The therapeutic mechanisms of other agents to attenuate cognitive impairment due to OSA
A few drugs were used to ameliorate cognitive deficits induced by OSA. However, experimental studies on OSA animal models indicate that chemical substances and natural products from Chinese herbs improve cognitive impairment (Table 2). Based on the action and molecular target mechanisms, these compounds are divided into: anti-oxidative properties, anti- inflammatory effects and anti-apoptosis effects.
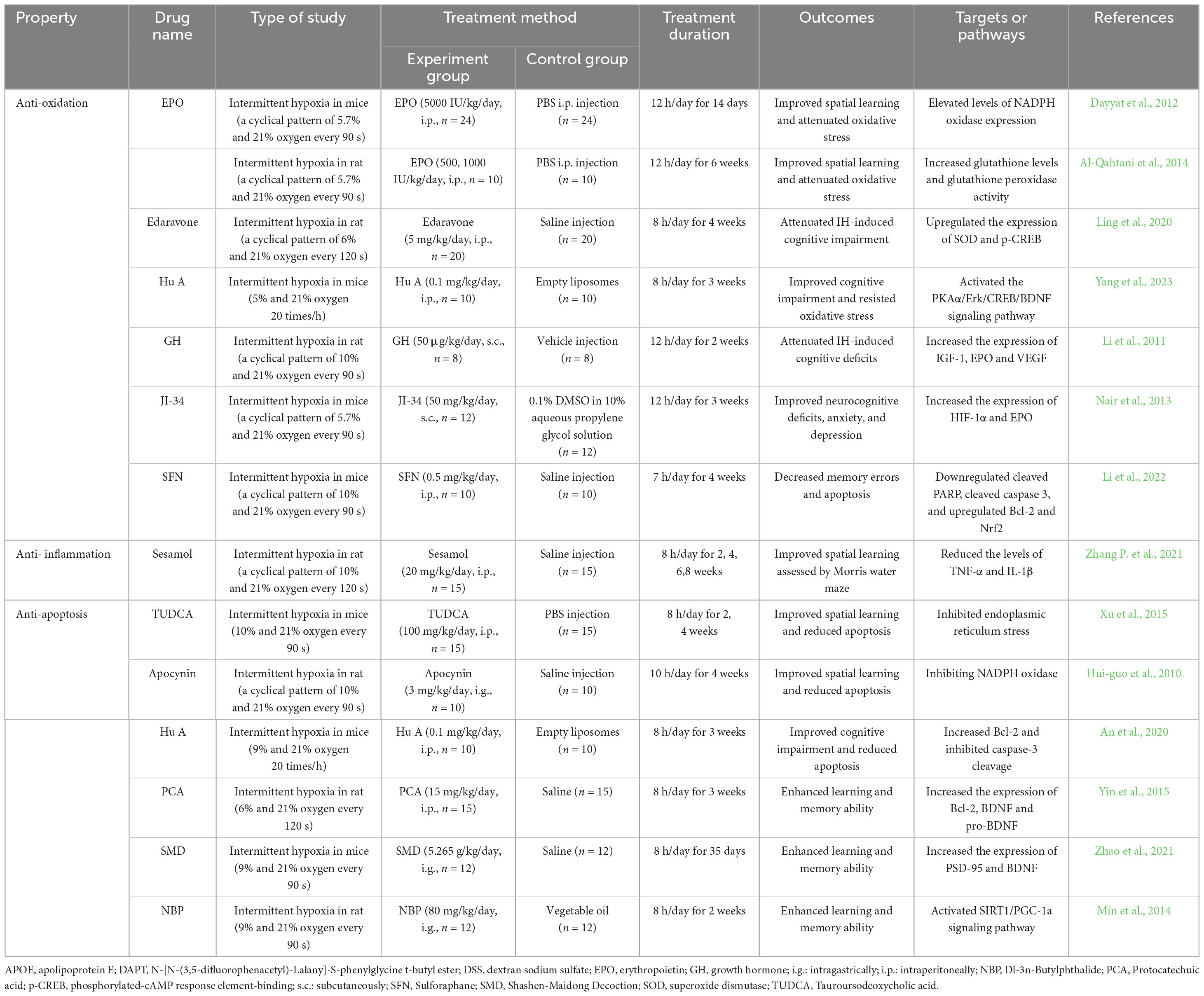
Table 2. The mechanisms by which some chemical substances and herbs medicine treat cognitive impairment in OSA animal model.
6.1. Anti-oxidative property
Erythropoietin (EPO), a prototypic cytokine and hypoxia-sensitive gene, has been implicated in improving cognitive ability through multiple signaling pathways (Sanchez et al., 2009; Dayyat et al., 2012). For instance, mice treated with exogenously administered erythropoietin (EPO) had protection from IH-induced spatial learning deficits caused by attenuating oxidative stress responses and suppressing NADPH oxidase expression (Dayyat et al., 2012). Another study indicated that this beneficial effect elevated glutathione levels and glutathione peroxidase activity (Al-Qahtani et al., 2014).
Edaravone is another potent free radical scavenger used to treat acute attacks of cerebral infarction and improve neurological symptoms with cognitive impairment. Edaravone attenuated IH-induced cognitive impairment and elevated the number of mitochondria by upregulating the expression of SOD and phosphorylated-cAMP response element-binding (p-CREB) (Ling et al., 2020).
One study revealed Huperzine A (Hu A) elevated T-SOD and GSH-Px abilities and reduced MDA content to resist oxidative stress damage with PKAα/Erk/CREB/BDNF signaling pathway (Yang et al., 2023).
Growth hormone (GH) modulates memory and cognitive functions and is impaired in OSA (Gianotti et al., 2002). GH could attenuate IH-induced cognitive deficits by elevating the expression of IGF-1, EPO, and VEGF (Li et al., 2011). GH secretion is controlled by growth hormone-releasing hormone (GHRH) (Schussler et al., 2006). JI-34 is an agonist of GHRH, attenuating IH- induced neurocognitive deficits. The underlying mechanism is associated with increased expression of HIF-1α and EPO (Nair et al., 2013).
Sulforaphane (SFN) is extracted from cruciferous vegetables of the Brassica genus, exerting neuroprotective effects by activating autophagy or transcription factor Nrf2 (Uddin et al., 2020). SFN treatment ameliorated neurocognitive dysfunction within IH mice by downregulated cleaved PARP, cleaved caspase 3, and upregulated Bcl-2 and Nrf2 (Li et al., 2022).
6.2. Anti-inflammatory effects
Sesamol can alleviate cognitive impairments in chronic IH-exposed rats. This beneficial effect could reduce hippocampal TNF-α and IL-1β levels (Zhang P. et al., 2021).
6.3. Anti-apoptosis effects
Based on our previous research, Tauroursodeoxycholic acid (TUDCA) can decrease neuronal apoptosis and enhance hippocampal synaptic plasticity by inhibiting endoplasmic reticulum stress activation (Xu et al., 2015).
Some natural products extracted from herbal medicine are also beneficial. Apocynin is a plant drug derived from Picrorhiza kurroa. Apocynin attenuated IH-induced spatial learning deficits and oxidative stress by inhibiting NADPH oxidase subunit p47phox mRNA and ameliorating cell apoptosis (Hui-guo et al., 2010; Yuan et al., 2015a).
Hu A is isolated from the Chinese herb Huperzia serrata and could cross the blood-brain barrier (BBB). Hu A could improve cognitive impairment and neuronal damage induced by IH by increasing the Bcl-2/Bax ratio and inhibiting caspase-3 cleavage (An et al., 2020).
Protocatechuic acid (PCA) is abundant in edible fruits and vegetables and is naturally present in various herbal medicine, including Hibiscus sabdariffa and Salvia miltiorrhiza. PCA could enhance learning and memory ability and alleviate oxidative stress and apoptosis in IH-treated rats by improving the expression of Bcl-2, BDNF, and pro-BDNF and reducing cleaved caspase-3 and IL-1β (Yin et al., 2015).
Shashen-Maidong Decoction (SMD) is an herbal formula with eight Chinese medicines [Ophiopogon japonicus (Thunb.) Ker Gawl. (9 g); Glehnia littoralis (A.Gray) F.Schmidt ex Miq. (9 g); Lablab purpureus (L.) Sweet (4.5 g); Morus indica L. (4.5 g); Polygonatum odoratum (Mill.) Druce (6 g); Trichosanthes kirilowii Maxim (4.5 g); Glycyrrhiza uralensis Fisch. Ex DC. (3 g)]. SMD treatment in a previous study improved performance assessed using the Morris Water Maze and Y-Maze test in mice exposed to IH by enhancing ERK/CREB phosphorylation and elevating PSD-95 and BDNF expression (Zhao et al., 2021).
Finally, Dl-3n-Butylphthalide (NBP) is extracted from Apium graveolens L with a broad spectrum of neuroprotective properties. One study described that NBP could inhibit apoptosis and promote IH-induced autophagy by activating the SIRT1/PGC-1a signaling pathway (Min et al., 2014).
7. Discussion
Cognitive impairment is a common symptom of OSA, irrespective of age (Vardanian and Ravdin, 2022). Neuroimaging studies depicted cerebral cortex morphology in multiple affected regions (Kizilgoz et al., 2013), with clinical impairment across various cognition aspects. Since the cognitive impairment mechanism in OSA is complex, SF and IH are significant factors activating multiple downstream signaling pathways while causing cognitive impairment (Figures 1, 2). Some signaling pathways and proteins were targeted by SF and IH, such as excessive NADPH oxidase activity (Nair et al., 2011a,b), inducing the expression of pro-inflammatory cytokines (Bertrand et al., 2020; Liu et al., 2020) and activating the TNF-α/NF-κB pathway (Berger and Polotsky, 2018; Zhang et al., 2022). These processes do not function alone and are affected by each other. For example, HIF-1α an oxidative stress marker, could activate inflammation response by inducing NO expression (Abe et al., 2017). Initially, oxidative stress is induced by impaired antioxidant capacity. Then, some pro-inflammation cytokines are generated, accelerating oxidative stress injury and triggering neuronal apoptosis.
Although some traditional methods, including oral appliances, surgery, and CPAP, have improved certain aspects of cognitive functioning, they do not fully alleviate cognitive complaints (Vardanian and Ravdin, 2022). Therefore, drug therapy could enhance treatment outcomes and be used with other therapy methods. Some drugs have beneficial effects on improving sleep quality (Taranto-Montemurro et al., 2016b,2017b). However, more large clinical trials are required to validate these findings. Meanwhile, some chemical substances and herbal medicine could improve cognitive ability in animal studies, while could become a complementary method based on successful clinical trials.
8. Conclusion and perspectives
Multiple cognitive aspects are affected in OSA, which current therapy cannot improve. This review summarized the randomized controlled trials of drugs for treating OSA-related cognitive impairment. Although these drugs could improve cognition, the studies have several limitations.
First, the sample size is very small for clinical studies. There is a lack of large-scale, multicenter, randomized controlled trials on drugs treating OSA-induced cognitive impairment. Second, a wide variation occurs in the characteristics of referred patients, such as age, disease history, and disease severity. Third, the drug treatment duration is short, and the prolonged effect has yet to be investigated. Fourth, there is a lack of preclinical studies examining the possible drug targets.
Future research should be directed toward these aspects to overcome these limitations. First, some large-scale, multicenter, and controlled trials are required to determine the efficacy of these drugs. Second, standardization of the clinical drug use process should be established in treating OSA-induced cognitive impairment. Lastly, additional studies should be performed in clinical trials for some novel chemical substances and herbal medicine.
Author contributions
DH and JC wrote the manuscript. XD and LX conceptualized the research work. LX revised the manuscript. All authors read and approved the final manuscript.
Funding
This work was supported by the National Natural Science Foundation of China (No: 81601972), the Zhejiang Provincial Natural Science Foundation of China under Grant (No: LQ21H090006), the Zhejiang Province Traditional Chinese Medicine Science and Technology Projection under Grant (No: 2021ZQ071) and the Zhejiang Special Health Funding Program of Hangzhou Medical College (No: YS2022002).
Conflict of interest
The authors declare that the research was conducted in the absence of any commercial or financial relationships that could be construed as a potential conflict of interest.
Publisher’s note
All claims expressed in this article are solely those of the authors and do not necessarily represent those of their affiliated organizations, or those of the publisher, the editors and the reviewers. Any product that may be evaluated in this article, or claim that may be made by its manufacturer, is not guaranteed or endorsed by the publisher.
References
Abe, H., Semba, H., and Takeda, N. (2017). The roles of hypoxia signaling in the pathogenesis of cardiovascular diseases. J. Atheroscler. Thromb. 24, 884–894. doi: 10.5551/jat.RV17009
Akinnusi, M., Jaoude, P., Kufel, T., and El-Solh, A. A. (2013). Toll-like receptor activity in patients with obstructive sleep apnea. Sleep Breath 17, 1009–1016. doi: 10.1007/s11325-012-0791-2
Alchanatis, M., Deligiorgis, N., Zias, N., Amfilochiou, A., Gotsis, E., Karakatsani, A., et al. (2004). Frontal brain lobe impairment in obstructive sleep apnoea: A proton MR spectroscopy study. Eur. Respir. J. 24, 980–986. doi: 10.1183/09031936.04.00127603
Alchanatis, M., Zias, N., Deligiorgis, N., Liappas, I., Chroneou, A., Soldatos, C., et al. (2008). Comparison of cognitive performance among different age groups in patients with obstructive sleep apnea. Sleep Breath 12, 17–24. doi: 10.1007/s11325-007-0133-y
Alkan, U., Nachalon, Y., Weiss, P., Ritter, A., Feinmesser, R., Gilat, H., et al. (2021). Effects of surgery for obstructive sleep apnea on cognitive function and driving performance. Sleep Breath 25, 1593–1600. doi: 10.1007/s11325-020-02285-w
Almendros, I., Farre, R., Planas, A. M., Torres, M., Bonsignore, M. R., Navajas, D., et al. (2011). Tissue oxygenation in brain, muscle, and fat in a rat model of sleep apnea: Differential effect of obstructive apneas and intermittent hypoxia. Sleep 34, 1127–1133. doi: 10.5665/SLEEP.1176
Aloia, M. S., Arnedt, J. T., Davis, J. D., Riggs, R. L., and Byrd, D. (2004). Neuropsychological sequelae of obstructive sleep apnea-hypopnea syndrome: A critical review. J. Int. Neuropsychol. Soc. 10, 772–785. doi: 10.1017/S1355617704105134
Al-Qahtani, J. M., Abdel-Wahab, B. A., and Abd El-Aziz, S. M. (2014). Long-term moderate dose exogenous erythropoietin treatment protects from intermittent hypoxia-induced spatial learning deficits and hippocampal oxidative stress in young rats. Neurochem. Res. 39, 161–171. doi: 10.1007/s11064-013-1201-2
An, J. R., Zhao, Y. S., Luo, L. F., Guan, P., Tan, M., and Ji, E. S. (2020). Huperzine A, reduces brain iron overload and alleviates cognitive deficit in mice exposed to chronic intermittent hypoxia. Life Sci. 250:117573. doi: 10.1016/j.lfs.2020.117573
Andrade, A. G., Bubu, O. M., Varga, A. W., and Osorio, R. S. (2018). The relationship between obstructive sleep apnea and Alzheimer’s disease. J. Alzheimers Dis. 64, S255–S270. doi: 10.3233/JAD-179936
Angelelli, P., Macchitella, L., Toraldo, D. M., Abbate, E., Marinelli, C. V., Arigliani, M., et al. (2020). The neuropsychological profile of attention deficits of patients with obstructive sleep apnea: An update on the daytime attentional impairment. Brain Sci. 10:325. doi: 10.3390/brainsci10060325
Arias-Cavieres, A., Khuu, M. A., Nwakudu, C. U., Barnard, J. E., Dalgin, G., and Garcia, A. J. III (2020). A HIF1a-dependent pro-oxidant state disrupts synaptic plasticity and impairs spatial memory in response to intermittent hypoxia. eNeuro 7, ENEURO.24–ENEURO.20. doi: 10.1523/ENEURO.0024-20.2020
Ayalon, L., Ancoli-Israel, S., Aka, A. A., McKenna, B. S., and Drummond, S. P. (2009). Relationship between obstructive sleep apnea severity and brain activation during a sustained attention task. Sleep 32, 373–381. doi: 10.1093/sleep/32.3.373
Bardwell, W. A., Ancoli-Israel, S., Berry, C. C., and Dimsdale, J. E. (2001). Neuropsychological effects of one-week continuous positive airway pressure treatment in patients with obstructive sleep apnea: A placebo-controlled study. Psychosom. Med. 63, 579–584. doi: 10.1097/00006842-200107000-00010
Beebe, D. W., Groesz, L., Wells, C., Nichols, A., and McGee, K. (2003). The neuropsychological effects of obstructive sleep apnea: A meta-analysis of norm-referenced and case-controlled data. Sleep 26, 298–307. doi: 10.1093/sleep/26.3.298
Berger, S., and Polotsky, V. Y. (2018). Leptin and leptin resistance in the pathogenesis of obstructive sleep apnea: A possible link to oxidative stress and cardiovascular complications. Oxid. Med. Cell Longev. 2018:5137947. doi: 10.1155/2018/5137947
Bertrand, S. J., Zhang, Z., Patel, R., O’Ferrell, C., Punjabi, N. M., Kudchadkar, S. R., et al. (2020). Transient neonatal sleep fragmentation results in long-term neuroinflammation and cognitive impairment in a rabbit model. Exp. Neurol. 327:113212. doi: 10.1016/j.expneurol.2020.113212
Bhandary, B., Marahatta, A., Kim, H. R., and Chae, H. J. (2012). An involvement of oxidative stress in endoplasmic reticulum stress and its associated diseases. Int. J. Mol. Sci. 14, 434–456. doi: 10.3390/ijms14010434
Bittencourt, L. R., Lucchesi, L. M., Rueda, A. D., Garbuio, S. A., Palombini, L. O., Guilleminault, C., et al. (2008). Placebo and modafinil effect on sleepiness in obstructive sleep apnea. Prog. Neuropsychopharmacol. Biol. Psychiatry 32, 552–559. doi: 10.1016/j.pnpbp.2007.10.016
Bubu, O. M., Andrade, A. G., Umasabor-Bubu, O. Q., Hogan, M. M., Turner, A. D., de Leon, M. J., et al. (2020). Obstructive sleep apnea, cognition and Alzheimer’s disease: A systematic review integrating three decades of multidisciplinary research. Sleep Med. Rev. 50:101250. doi: 10.1016/j.smrv.2019.101250
Bucks, R. S., Olaithe, M., and Eastwood, P. (2013). Neurocognitive function in obstructive sleep apnoea: A meta-review. Respirology 18, 61–70. doi: 10.1111/j.1440-1843.2012.02255.x
Cai, S., Li, Z., Wang, J., Wang, Q., and Chen, R. (2023). Excessive daytime sleepiness in young and middle-aged Chinese adults with obstructive sleep apnea: Implications for cognitive dysfunction. Sleep Breath doi: 10.1007/s11325-023-02854-9 [Epub ahead of print].
Carley, D. W., Olopade, C., Ruigt, G. S., and Radulovacki, M. (2007). Efficacy of mirtazapine in obstructive sleep apnea syndrome. Sleep 30, 35–41. doi: 10.1093/sleep/30.1.35
Carlucci, A., Ceriana, P., Mancini, M., Cirio, S., Pierucci, P., D’Artavilla Lupo, N., et al. (2015). Efficacy of bilevel-auto treatment in patients with obstructive sleep apnea not responsive to or intolerant of continuous positive airway pressure ventilation. J. Clin. Sleep Med. 11, 981–985. doi: 10.5664/jcsm.5008
Carreras, A., Zhang, S. X., Peris, E., Qiao, Z., Gileles-Hillel, A., Li, R. C., et al. (2014). Chronic sleep fragmentation induces endothelial dysfunction and structural vascular changes in mice. Sleep 37, 1817–1824. doi: 10.5665/sleep.4178
Chapman, J. L., Cayanan, E. A., Hoyos, C. M., Serinel, Y., Comas, M., Yee, B. J., et al. (2018). Does armodafinil improve driving task performance and weight loss in sleep apnea? A randomized trial. Am. J. Respir. Crit Care Med. 198, 941–950. doi: 10.1164/rccm.201712-2439OC
Chauhan, G., Ray, K., Sahu, S., Roy, K., Jain, V., Wadhwa, M., et al. (2016). Adenosine A1 receptor antagonist mitigates deleterious effects of sleep deprivation on adult neurogenesis and spatial reference memory in rats. Neuroscience 337, 107–116. doi: 10.1016/j.neuroscience.2016.09.007
Clinton, S. M., Sucharski, I. L., and Finlay, J. M. (2006). Desipramine attenuates working memory impairments induced by partial loss of catecholamines in the rat medial prefrontal cortex. Psychopharmacology 183, 404–412. doi: 10.1007/s00213-005-0221-2
Cosentino, F. I., Bosco, P., Drago, V., Prestianni, G., Lanuzza, B., Iero, I., et al. (2008). The APOE epsilon4 allele increases the risk of impaired spatial working memory in obstructive sleep apnea. Sleep Med. 9, 831–839. doi: 10.1016/j.sleep.2007.10.015
Cui, L., Xue, R., Zhang, X., Chen, S., Wan, Y., and Wu, W. (2019). Sleep deprivation inhibits proliferation of adult hippocampal neural progenitor cells by a mechanism involving IL-17 and p38 MAPK. Brain Res. 1714, 81–87. doi: 10.1016/j.brainres.2019.01.024
da Rosa, D. P., Forgiarini, L. F., Silva, M. B., Fiori, C. Z., Andrade, C. F., Martinez, D., et al. (2015). Antioxidants inhibit the inflammatory and apoptotic processes in an intermittent hypoxia model of sleep apnea. Inflamm. Res. 64, 21–29. doi: 10.1007/s00011-014-0778-5
Dalmases, M., Sole-Padulles, C., Torres, M., Embid, C., Nunez, M. D., Martinez-Garcia, M. A., et al. (2015). Effect of CPAP on cognition, brain function, and structure among elderly patients with OSA: A randomized pilot study. Chest 148, 1214–1223. doi: 10.1378/chest.15-0171
Daurat, A., Ricarrere, M., and Tiberge, M. (2013). Decision making is affected in obstructive sleep apnoea syndrome. J. Neuropsychol. 7, 139–144. doi: 10.1111/j.1748-6653.2012.02039.x
Dayyat, E. A., Zhang, S. X., Wang, Y., Cheng, Z. J., and Gozal, D. (2012). Exogenous erythropoietin administration attenuates intermittent hypoxia-induced cognitive deficits in a murine model of sleep apnea. BMC Neurosci. 13:77. doi: 10.1186/1471-2202-13-77
Deng, Y., Yuan, X., Guo, X. L., Zhu, D., Pan, Y. Y., and Liu, H. G. (2015). Efficacy of atorvastatin on hippocampal neuronal damage caused by chronic intermittent hypoxia: Involving TLR4 and its downstream signaling pathway. Respir. Physiol. Neurobiol. 218, 57–63. doi: 10.1016/j.resp.2015.07.006
Dewan, N. A., Nieto, F. J., and Somers, V. K. (2015). Intermittent hypoxemia and OSA: Implications for comorbidities. Chest 147, 266–274. doi: 10.1378/chest.14-0500
Dinges, D. F., and Weaver, T. E. (2003). Effects of modafinil on sustained attention performance and quality of life in OSA patients with residual sleepiness while being treated with nCPAP. Sleep Med. 4, 393–402. doi: 10.1016/s1389-9457(03)00108-4
Djonlagic, I., Guo, M., Matteis, P., Carusona, A., Stickgold, R., and Malhotra, A. (2014). Untreated sleep-disordered breathing: Links to aging-related decline in sleep-dependent memory consolidation. PLoS One 9:e85918. doi: 10.1371/journal.pone.0085918
Djonlagic, I., Guo, M., Matteis, P., Carusona, A., Stickgold, R., and Malhotra, A. (2015). First night of CPAP: Impact on memory consolidation attention and subjective experience. Sleep Med. 16, 697–702. doi: 10.1016/j.sleep.2015.01.017
Epstein, L. J., Kristo, D., Strollo, P. J. Jr., Friedman, N., Malhotra, A., Patil, S. P., et al. (2009). Clinical guideline for the evaluation, management and long-term care of obstructive sleep apnea in adults. J. Clin. Sleep Med. 5, 263–276.
Farajdokht, F., Vatandoust, S. M., Hosseini, L., Fekri, K., Rahigh Aghsan, S., Majdi, A., et al. (2021). Sericin protects against acute sleep deprivation-induced memory impairment via enhancement of hippocampal synaptic protein levels and inhibition of oxidative stress and neuroinflammation in mice. Brain Res. Bull. 174, 203–211. doi: 10.1016/j.brainresbull.2021.06.013
Fei, W., Jiao, W., Feng, X., Chen, X., and Wang, Y. (2021). Intermittent hypoxia mimicking obstructive sleep apnea aggravates early brain injury following ICH via neuroinflammation and apoptosis. Mol. Med. Rep. 24:12464. doi: 10.3892/mmr.2021.12464
Gagnon, K., Baril, A. A., Gagnon, J. F., Fortin, M., Decary, A., Lafond, C., et al. (2014). Cognitive impairment in obstructive sleep apnea. Pathol. Biol. 62, 233–240. doi: 10.1016/j.patbio.2014.05.015
Gelir, E., Basaran, C., Bayrak, S., Yagcioglu, S., Budak, M. T., Firat, H., et al. (2014). Electrophysiological assessment of the effects of obstructive sleep apnea on cognition. PLoS One 9:e90647. doi: 10.1371/journal.pone.0090647
George, C. F. (2004). Sleep. 5: Driving and automobile crashes in patients with obstructive sleep apnoea/hypopnoea syndrome. Thorax 59, 804–807. doi: 10.1136/thx.2003.007187
Gianotti, L., Pivetti, S., Lanfranco, F., Tassone, F., Navone, F., Vittori, E., et al. (2002). Concomitant impairment of growth hormone secretion and peripheral sensitivity in obese patients with obstructive sleep apnea syndrome. J. Clin. Endocrinol. Metab. 87, 5052–5057. doi: 10.1210/jc.2001-011441
Goldbart, A. D., Greenberg-Dotan, S., and Tal, A. (2012). Montelukast for children with obstructive sleep apnea: A double-blind, placebo-controlled study. Pediatrics 130, e575–e580. doi: 10.1542/peds.2012-0310
Gorlach, A., Bertram, K., Hudecova, S., and Krizanova, O. (2015). Calcium and ROS: A mutual interplay. Redox Biol. 6, 260–271. doi: 10.1016/j.redox.2015.08.010
Gozal, D., Daniel, J. M., and Dohanich, G. P. (2001). Behavioral and anatomical correlates of chronic episodic hypoxia during sleep in the rat. J. Neurosci. 21, 2442–2450.
Greve, D. N., Duntley, S. P., Larson-Prior, L., Krystal, A. D., Diaz, M. T., Drummond, S. P., et al. (2014). Effect of armodafinil on cortical activity and working memory in patients with residual excessive sleepiness associated with CPAP-Treated OSA: A multicenter fMRI study. J. Clin. Sleep Med. 10, 143–153. doi: 10.5664/jcsm.3440
Guo, H., Ding, H., Yan, Y., Chen, Q., Zhang, J., Chen, B., et al. (2021). Intermittent hypoxia-induced autophagy via AMPK/mTOR signaling pathway attenuates endothelial apoptosis and dysfunction in vitro. Sleep Breath 25, 1859–1865. doi: 10.1007/s11325-021-02297-0
Hayden, M. S., and Ghosh, S. (2008). Shared principles in NF-kappaB signaling. Cell 132, 344–362. doi: 10.1016/j.cell.2008.01.020
He, Y., Hara, H., and Nunez, G. (2016). Mechanism and regulation of NLRP3 inflammasome activation. Trends Biochem. Sci. 41, 1012–1021. doi: 10.1016/j.tibs.2016.09.002
Hedner, J., Kraiczi, H., Peker, Y., and Murphy, P. (2003). Reduction of sleep-disordered breathing after physostigmine. Am. J. Respir. Crit. Care Med. 168, 1246–1251. doi: 10.1164/rccm.200211-1344OC
Higa, A., and Chevet, E. (2012). Redox signaling loops in the unfolded protein response. Cell Signal 24, 1548–1555. doi: 10.1016/j.cellsig.2012.03.011
Hirshkowitz, M., Black, J. E., Wesnes, K., Niebler, G., Arora, S., and Roth, T. (2007). Adjunct armodafinil improves wakefulness and memory in obstructive sleep apnea/hypopnea syndrome. Respir. Med. 101, 616–627. doi: 10.1016/j.rmed.2006.06.007
Hong, S., Beja-Glasser, V. F., Nfonoyim, B. M., Frouin, A., Li, S., Ramakrishnan, S., et al. (2016). Complement and microglia mediate early synapse loss in Alzheimer mouse models. Science 352, 712–716. doi: 10.1126/science.aad8373
Hoyos, C. M., Drager, L. F., and Patel, S. R. (2017). OSA and cardiometabolic risk: What’s the bottom line? Respirology 22, 420–429. doi: 10.1111/resp.12984
Htoo, A. K., Greenberg, H., Tongia, S., Chen, G., Henderson, T., Wilson, D., et al. (2006). Activation of nuclear factor kappaB in obstructive sleep apnea: A pathway leading to systemic inflammation. Sleep Breath 10, 43–50. doi: 10.1007/s11325-005-0046-6
Hui, D. S., Chan, J. K., Choy, D. K., Ko, F. W., Li, T. S., Leung, R. C., et al. (2000). Effects of augmented continuous positive airway pressure education and support on compliance and outcome in a Chinese population. Chest 117, 1410–1416. doi: 10.1378/chest.117.5.1410
Hui-guo, L., Kui, L., Yan-ning, Z., and Yong-jian, X. (2010). Apocynin attenuate spatial learning deficits and oxidative responses to intermittent hypoxia. Sleep Med. 11, 205–212. doi: 10.1016/j.sleep.2009.05.015
Inoue, Y., Takasaki, Y., and Yamashiro, Y. (2013). Efficacy and safety of adjunctive modafinil treatment on residual excessive daytime sleepiness among nasal continuous positive airway pressure-treated japanese patients with obstructive sleep apnea syndrome: A double-blind placebo-controlled study. J. Clin. Sleep Med. 9, 751–757. doi: 10.5664/jcsm.2912
Jordan, A. S., McSharry, D. G., and Malhotra, A. (2014). Adult obstructive sleep apnoea. Lancet 383, 736–747. doi: 10.1016/S0140-6736(13)60734-5
Kaneshwaran, K., Olah, M., Tasaki, S., Yu, L., Bradshaw, E. M., Schneider, J. A., et al. (2019). Sleep fragmentation, microglial aging, and cognitive impairment in adults with and without Alzheimer’s dementia. Sci. Adv. 5, eaax7331. doi: 10.1126/sciadv.aax7331
Karimi, M., Hedner, J., Zou, D., Eskandari, D., Lundquist, A. C., and Grote, L. (2015). Attention deficits detected in cognitive tests differentiate between sleep apnea patients with or without a motor vehicle accident. Sleep Med. 16, 528–533. doi: 10.1016/j.sleep.2014.11.015
Kay, G. G., and Feldman, N. (2013). Effects of armodafinil on simulated driving and self-report measures in obstructive sleep apnea patients prior to treatment with continuous positive airway pressure. J. Clin. Sleep Med. 9, 445–454. doi: 10.5664/jcsm.2662
Kheirandish-Gozal, L., and Gozal, D. (2008). Intranasal budesonide treatment for children with mild obstructive sleep apnea syndrome. Pediatrics 122, e149–e155. doi: 10.1542/peds.2007-3398
Kiely, J. L., Nolan, P., and McNicholas, W. T. (2004). Intranasal corticosteroid therapy for obstructive sleep apnoea in patients with co-existing rhinitis. Thorax 59, 50–55.
Kiernan, E. A., Smith, S. M., Mitchell, G. S., and Watters, J. J. (2016). Mechanisms of microglial activation in models of inflammation and hypoxia: Implications for chronic intermittent hypoxia. J. Physiol. 594, 1563–1577. doi: 10.1113/JP271502
Kim, Y. S., Kwak, J. W., Lee, K. E., Cho, H. S., Lim, S. J., Kim, K. S., et al. (2014). Can mitochondrial dysfunction be a predictive factor for oxidative stress in patients with obstructive sleep apnea? Antioxid. Redox Signal 21, 1285–1288. doi: 10.1089/ars.2014.5955
Kingshott, R. N., Vennelle, M., Coleman, E. L., Engleman, H. M., Mackay, T. W., and Douglas, N. J. (2001). Randomized, double-blind, placebo-controlled crossover trial of modafinil in the treatment of residual excessive daytime sleepiness in the sleep apnea/hypopnea syndrome. Am. J. Respir. Crit Care Med. 163, 918–923. doi: 10.1164/ajrccm.163.4.2005036
Kizilgoz, V., Aydin, H., Tatar, I. G., Hekimoglu, B., Ardic, S., Firat, H., et al. (2013). Proton magnetic resonance spectroscopy of periventricular white matter and hippocampus in obstructive sleep apnea patients. Pol. J. Radiol. 78, 7–14. doi: 10.12659/PJR.889923
Lau, E. Y., Choi, E. W., Lai, E. S., Lau, K. N., Au, C. T., Yung, W. H., et al. (2015). Working memory impairment and its associated sleep-related respiratory parameters in children with obstructive sleep apnea. Sleep Med. 16, 1109–1115. doi: 10.1016/j.sleep.2015.04.025
Lavie, L. (2012). Oxidative stress inflammation and endothelial dysfunction in obstructive sleep apnea. Front. Biosci. 4:1391–1403. doi: 10.2741/469
Lee, J. M., Lee, J. H., Song, M. K., and Kim, Y. J. (2022). NXP032 ameliorates aging-induced oxidative stress and cognitive impairment in mice through activation of Nrf2 signaling. Antioxidants 11:10130. doi: 10.3390/antiox11010130
Li, R. C., Guo, S. Z., Raccurt, M., Moudilou, E., Morel, G., Brittian, K. R., et al. (2011). Exogenous growth hormone attenuates cognitive deficits induced by intermittent hypoxia in rats. Neuroscience 196, 237–250. doi: 10.1016/j.neuroscience.2011.08.029
Li, X., Ying, H., Zhang, Z., Yang, Z., You, C., Cai, X., et al. (2022). Sulforaphane attenuates chronic intermittent hypoxia-induced brain damage in mice via augmenting Nrf2 nuclear translocation and autophagy. Front. Cell Neurosci. 16:827527. doi: 10.3389/fncel.2022.827527
Li, Y., Ye, J., Han, D., Zhao, D., Cao, X., Orr, J., et al. (2019). The effect of upper airway surgery on loop gain in obstructive sleep apnea. J. Clin. Sleep Med. 15, 907–913. doi: 10.5664/jcsm.7848
Lim, W., Bardwell, W. A., Loredo, J. S., Kim, E. J., Ancoli-Israel, S., Morgan, E. E., et al. (2007). Neuropsychological effects of 2-week continuous positive airway pressure treatment and supplemental oxygen in patients with obstructive sleep apnea: A randomized placebo-controlled study. J. Clin. Sleep Med. 3, 380–386.
Lin, H., Wang, C., Zhang, H., Xiong, H., Li, Z., Huang, X., et al. (2019). Threshold of the upper airway cross-section for hypopnea onset during sleep and its identification under waking condition. Respir. Res. 20:280. doi: 10.1186/s12931-019-1250-4
Ling, J., Yu, Q., Li, Y., Yuan, X., Wang, X., Liu, W., et al. (2020). Edaravone improves intermittent hypoxia-induced cognitive impairment and hippocampal damage in rats. Biol. Pharm Bull. 43, 1196–1201. doi: 10.1248/bpb.b20-00085
Littner, M., Johnson, S. F., McCall, W. V., Anderson, W. M., Davila, D., Hartse, S. K., et al. (2001). Practice parameters for the treatment of narcolepsy: An update for 2000. Sleep 24, 451–466.
Liu, X., Chen, L., Duan, W., Li, H., Kong, L., Shu, Y., et al. (2022). Abnormal functional connectivity of hippocampal subdivisions in obstructive sleep apnea: A resting-state functional magnetic resonance imaging study. Front. Neurosci. 16:850940. doi: 10.3389/fnins.2022.850940
Liu, X., Ma, Y., Ouyang, R., Zeng, Z., Zhan, Z., Lu, H., et al. (2020). The relationship between inflammation and neurocognitive dysfunction in obstructive sleep apnea syndrome. J. Neuroinflammation 17:229. doi: 10.1186/s12974-020-01905-2
Liu, X., Sood, S., Liu, H., and Horner, R. L. (2005). Opposing muscarinic and nicotinic modulation of hypoglossal motor output to genioglossus muscle in rats in vivo. J. Physiol. 565, 965–980. doi: 10.1113/jphysiol.2005.084657
Lumeng, J. C., and Chervin, R. D. (2008). Epidemiology of pediatric obstructive sleep apnea. Proc. Am. Thorac. Soc. 5, 242–252. doi: 10.1513/pats.200708-135MG
Macey, P. M., Kumar, R., Yan-Go, F. L., Woo, M. A., and Harper, R. M. (2012). Sex differences in white matter alterations accompanying obstructive sleep apnea. Sleep 35, 1603–1613. doi: 10.5665/sleep.2228
Mamelak, M. (2022). Sleep, narcolepsy, and sodium oxybate. Curr. Neuropharmacol. 20, 272–291. doi: 10.2174/1570159X19666210407151227
Maniaci, A., Iannella, G., Cocuzza, S., Vicini, C., Magliulo, G., Ferlito, S., et al. (2021). Oxidative stress and inflammation biomarker expression in obstructive sleep apnea patients. J. Clin. Med. 10:277. doi: 10.3390/jcm10020277
Mathieu, A., Mazza, S., Decary, A., Massicotte-Marquez, J., Petit, D., Gosselin, N., et al. (2008). Effects of obstructive sleep apnea on cognitive function: A comparison between younger and older OSAS patients. Sleep Med. 9, 112–120. doi: 10.1016/j.sleep.2007.03.014
Mazza, S., Pepin, J. L., Naegele, B., Plante, J., Deschaux, C., and Levy, P. (2005). Most obstructive sleep apnoea patients exhibit vigilance and attention deficits on an extended battery of tests. Eur. Respir. J. 25, 75–80. doi: 10.1183/09031936.04.00011204
Mello, T., Zanieri, F., Ceni, E., and Galli, A. (2016). Oxidative stress in the healthy and wounded hepatocyte: A cellular organelles perspective. Oxid. Med. Cell Longev. 2016:8327410. doi: 10.1155/2016/8327410
Min, J. J., Huo, X. L., Xiang, L. Y., Qin, Y. Q., Chai, K. Q., Wu, B., et al. (2014). Protective effect of Dl-3n-butylphthalide on learning and memory impairment induced by chronic intermittent hypoxia-hypercapnia exposure. Sci. Rep. 4:5555. doi: 10.1038/srep05555
Mishra, I., Pullum, K. B., Eads, K. N., Strunjas, A. R., and Ashley, N. T. (2022). Peripheral sympathectomy alters neuroinflammatory and microglial responses to sleep fragmentation in female mice. Neuroscience 505, 111–124. doi: 10.1016/j.neuroscience.2022.09.022
Mohammadi, M., Manaheji, H., Maghsoudi, N., Danyali, S., Baniasadi, M., and Zaringhalam, J. (2020). Microglia dependent BDNF and proBDNF can impair spatial memory performance during persistent inflammatory pain. Behav. Brain Res. 390:112683. doi: 10.1016/j.bbr.2020.112683
Mubashir, T., Abrahamyan, L., Niazi, A., Piyasena, D., Arif, A. A., Wong, J., et al. (2019). The prevalence of obstructive sleep apnea in mild cognitive impairment: A systematic review. BMC Neurol. 19:195. doi: 10.1186/s12883-019-1422-3
Muller-Oehring, E. M., and Schulte, T. (2014). Cognition, emotion, and attention. Handb. Clin. Neurol. 125, 341–354. doi: 10.1016/B978-0-444-62619-6.00020-3
Munoz-Torres, Z., Jimenez-Correa, U., and Montes-Rodriguez, C. J. (2020). Sex differences in brain oscillatory activity during sleep and wakefulness in obstructive sleep apnea. J. Sleep Res. 29, e12977. doi: 10.1111/jsr.12977
Muraja-Murro, A., Kulkas, A., Hiltunen, M., Kupari, S., Hukkanen, T., Tiihonen, P., et al. (2014). Adjustment of apnea-hypopnea index with severity of obstruction events enhances detection of sleep apnea patients with the highest risk of severe health consequences. Sleep Breath 18, 641–647. doi: 10.1007/s11325-013-0927-z
Naegele, B., Launois, S. H., Mazza, S., Feuerstein, C., Pepin, J. L., and Levy, P. (2006). Which memory processes are affected in patients with obstructive sleep apnea? An evaluation of 3 types of memory. Sleep 29, 533–544. doi: 10.1093/sleep/29.4.533
Naegele, B., Thouvard, V., Pepin, J. L., Levy, P., Bonnet, C., Perret, J. E., et al. (1995). Deficits of cognitive executive functions in patients with sleep apnea syndrome. Sleep 18, 43–52.
Nair, D., Dayyat, E. A., Zhang, S. X., Wang, Y., and Gozal, D. (2011a). Intermittent hypoxia-induced cognitive deficits are mediated by NADPH oxidase activity in a murine model of sleep apnea. PLoS One 6:e19847. doi: 10.1371/journal.pone.0019847
Nair, D., Zhang, S. X., Ramesh, V., Hakim, F., Kaushal, N., Wang, Y., et al. (2011b). Sleep fragmentation induces cognitive deficits via nicotinamide adenine dinucleotide phosphate oxidase-dependent pathways in mouse. Am. J. Respir. Crit. Care Med. 184, 1305–1312. doi: 10.1164/rccm.201107-1173OC
Nair, D., Ramesh, V., Li, R. C., Schally, A. V., and Gozal, D. (2013). Growth hormone releasing hormone (GHRH) signaling modulates intermittent hypoxia-induced oxidative stress and cognitive deficits in mouse. J Neurochem 127, 531–540. doi: 10.1111/jnc.12360
Nishino, S., and Okuro, M. (2008). Armodafinil for excessive daytime sleepiness. Drugs Today 44, 395–414. doi: 10.1358/dot.2008.44.6.1195861
Olaithe, M., and Bucks, R. S. (2013). Executive dysfunction in OSA before and after treatment: A meta-analysis. Sleep 36, 1297–1305. doi: 10.5665/sleep.2950
Owen, A. M., McMillan, K. M., Laird, A. R., and Bullmore, E. (2005). N-back working memory paradigm: A meta-analysis of normative functional neuroimaging studies. Hum. Brain Mapp. 25, 46–59. doi: 10.1002/hbm.20131
Pack, A. I., Black, J. E., Schwartz, J. R., and Matheson, J. K. (2001). Modafinil as adjunct therapy for daytime sleepiness in obstructive sleep apnea. Am. J. Respir. Crit. Care Med. 164, 1675–1681. doi: 10.1164/ajrccm.164.9.2103032
Pandey, A., and Kar, S. K. (2018). Rapid eye movement sleep deprivation of rat generates ROS in the hepatocytes and makes them more susceptible to oxidative stress. Sleep Sci. 11, 245–253. doi: 10.5935/1984-0063.20180039
Patel, A., and Chong, D. J. (2021). Obstructive sleep apnea: Cognitive outcomes. Clin. Geriatr. Med. 37, 457–467. doi: 10.1016/j.cger.2021.04.007
Prabhakar, N. R. (2011). Sensory plasticity of the carotid body: Role of reactive oxygen species and physiological significance. Respir. Physiol. Neurobiol. 178, 375–380. doi: 10.1016/j.resp.2011.05.012
Proenca, M. B., Dombrowski, P. A., Da Cunha, C., Fischer, L., Ferraz, A. C., and Lima, M. M. (2014). Dopaminergic D2 receptor is a key player in the substantia nigra pars compacta neuronal activation mediated by REM sleep deprivation. Neuropharmacology 76, 118–126. doi: 10.1016/j.neuropharm.2013.08.024
Qiu, K., Mao, M., Hu, Y., Yi, X., Zheng, Y., Ying, Z., et al. (2022). Gender-specific association between obstructive sleep apnea and cognitive impairment among adults. Sleep Med. 98, 158–166. doi: 10.1016/j.sleep.2022.07.004
Ramesh, V., Lakshmana, M. K., Rao, S., Raju, T. R., and Kumar, V. M. (1999). Alterations in monoamine neurotransmitters and dendritic spine densities at the medial preoptic area after sleep deprivation. Sleep Res. Online 2, 49–55.
Roth, T., White, D., Schmidt-Nowara, W., Wesnes, K. A., Niebler, G., Arora, S., et al. (2006). Effects of armodafinil in the treatment of residual excessive sleepiness associated with obstructive sleep apnea/hypopnea syndrome: A 12-week, multicenter, double-blind, randomized, placebo-controlled study in nCPAP-adherent adults. Clin. Ther. 28, 689–706. doi: 10.1016/j.clinthera.2006.05.013
Row, B. W., Kheirandish, L., Neville, J. J., and Gozal, D. (2002). Impaired spatial learning and hyperactivity in developing rats exposed to intermittent hypoxia. Pediatr. Res. 52, 449–453. doi: 10.1203/00006450-200209000-00024
Sanchez, P. E., Fares, R. P., Risso, J. J., Bonnet, C., Bouvard, S., Le-Cavorsin, M., et al. (2009). Optimal neuroprotection by erythropoietin requires elevated expression of its receptor in neurons. Proc. Natl. Acad. Sci. U. S. A. 106, 9848–9853. doi: 10.1073/pnas.0901840106
Sateia, M. J. (2003). Neuropsychological impairment and quality of life in obstructive sleep apnea. Clin. Chest Med. 24, 249–259. doi: 10.1016/s0272-5231(03)00014-5
Saunamaki, T., Himanen, S. L., Polo, O., and Jehkonen, M. (2009a). Executive dysfunction in patients with obstructive sleep apnea syndrome. Eur. Neurol. 62, 237–242. doi: 10.1159/000232156
Saunamaki, T., Jehkonen, M., Huupponen, E., Polo, O., and Himanen, S. L. (2009b). Visual dysfunction and computational sleep depth changes in obstructive sleep apnea syndrome. Clin. EEG Neurosci. 40, 162–167. doi: 10.1177/155005940904000308
Saunamaki, T., Himanen, S. L., Polo, O., and Jehkonen, M. (2010). Executive dysfunction and learning effect after continuous positive airway pressure treatment in patients with obstructive sleep apnea syndrome. Eur. Neurol. 63, 215–220. doi: 10.1159/000278301
Schussler, P., Yassouridis, A., Uhr, M., Kluge, M., Weikel, J., Holsboer, F., et al. (2006). Growth hormone-releasing hormone and corticotropin-releasing hormone enhance non-rapid-eye-movement sleep after sleep deprivation. Am. J. Physiol. Endocrinol. Metab. 291, E549–E556. doi: 10.1152/ajpendo.00641.2005
Schwartz, J. R., Hirshkowitz, M., Erman, M. K., and Schmidt-Nowara, W. (2003). Modafinil as adjunct therapy for daytime sleepiness in obstructive sleep apnea: A 12-week, open-label study. Chest 124, 2192–2199. doi: 10.1378/chest.124.6.2192
Segsarnviriya, C., Chumthong, R., and Mahakit, P. (2021). Effects of intranasal steroids on continuous positive airway pressure compliance among patients with obstructive sleep apnea. Sleep Breath 25, 1293–1299. doi: 10.1007/s11325-020-02236-5
Series, F., Series, I., and Cormier, Y. (1992). Effects of enhancing slow-wave sleep by gamma-hydroxybutyrate on obstructive sleep apnea. Am. Rev. Respir. Dis. 145, 1378–1383. doi: 10.1164/ajrccm/145.6.1378
Sforza, E., and Roche, F. (2012). Sleep apnea syndrome and cognition. Front. Neurol. 3:87. doi: 10.3389/fneur.2012.00087
Shamsuzzaman, A. S., Gersh, B. J., and Somers, V. K. (2003). Obstructive sleep apnea: Implications for cardiac and vascular disease. JAMA 290, 1906–1914. doi: 10.1001/jama.290.14.1906
She, N., Shi, Y., Feng, Y., Ma, L., Yuan, Y., Zhang, Y., et al. (2022). NLRP3 inflammasome regulates astrocyte transformation in brain injury induced by chronic intermittent hypoxia. BMC Neurosci. 23:70. doi: 10.1186/s12868-022-00756-2
Smith, D. F., Sarber, K. M., Spiceland, C. P., Ishman, S. L., Augelli, D. M., and Romaker, A. M. (2019). Effects of medical therapy on mild obstructive sleep apnea in adult patients. J. Clin. Sleep Med. 15, 979–983. doi: 10.5664/jcsm.7876
Smith, S. M., Friedle, S. A., and Watters, J. J. (2013). Chronic intermittent hypoxia exerts CNS region-specific effects on rat microglial inflammatory and TLR4 gene expression. PLoS One 8:e81584. doi: 10.1371/journal.pone.0081584
St Louis, E. K. (2010). Diagnosing and treating co-morbid sleep apnea in neurological disorders. Part II. Pract. Neurol. 9, 26–31.
Stepanski, E. J. (2002). The effect of sleep fragmentation on daytime function. Sleep 25, 268–276. doi: 10.1093/sleep/25.3.268
Sukova, K., Uchytilova, M., and Lindova, J. (2013). Abstract concept formation in African grey parrots (Psittacus erithacus) on the basis of a low number of cues. Behav. Processes 96, 36–41. doi: 10.1016/j.beproc.2013.02.008
Taranto-Montemurro, L., Edwards, B. A., Sands, S. A., Marques, M., Eckert, D. J., White, D. P., et al. (2016a). Desipramine increases genioglossus activity and reduces upper airway collapsibility during Non-REM sleep in healthy subjects. Am. J. Respir. Crit. Care Med. 194, 878–885. doi: 10.1164/rccm.201511-2172OC
Taranto-Montemurro, L., Sands, S. A., Edwards, B. A., Azarbarzin, A., Marques, M., de Melo, C., et al. (2016b). Desipramine improves upper airway collapsibility and reduces OSA severity in patients with minimal muscle compensation. Eur. Respir. J. 48, 1340–1350. doi: 10.1183/13993003.00823-2016
Taranto-Montemurro, L., Messineo, L., Azarbarzin, A., Vena, D., Hess, L. B., Calianese, N. A., et al. (2020). Effects of the combination of atomoxetine and oxybutynin on OSA endotypic traits. Chest 157, 1626–1636. doi: 10.1016/j.chest.2020.01.012
Taranto-Montemurro, L., Messineo, L., Sands, S. A., Azarbarzin, A., Marques, M., Edwards, B. A., et al. (2019). The combination of atomoxetine and oxybutynin greatly reduces obstructive sleep apnea severity. A randomized, placebo-controlled, double-blind crossover trial. Am. J. Respir. Crit. Care Med. 199, 1267–1276. doi: 10.1164/rccm.201808-1493OC
Taranto-Montemurro, L., Sands, S. A., Azarbarzin, A., Marques, M., de Melo, C. M., Edwards, B. A., et al. (2017a). Effect of 4-aminopyridine on genioglossus muscle activity during sleep in healthy adults. Ann Am. Thorac. Soc. 14, 1177–1183. doi: 10.1513/AnnalsATS.201701-006OC
Taranto-Montemurro, L., Sands, S. A., Edwards, B. A., Azarbarzin, A., Marques, M., de Melo, C., et al. (2017b). Effects of tiagabine on slow wave sleep and arousal threshold in patients with obstructive sleep apnea. Sleep 40, zsw047. doi: 10.1093/sleep/zsw047
Tartar, J. L., Ward, C. P., McKenna, J. T., Thakkar, M., Arrigoni, E., McCarley, R. W., et al. (2006). Hippocampal synaptic plasticity and spatial learning are impaired in a rat model of sleep fragmentation. Eur. J. Neurosci. 23, 2739–2748. doi: 10.1111/j.1460-9568.2006.04808.x
Toraldo, D. M., Di Michele, L., Ralli, M., Arigliani, M., Passali, G. C., De Benedetto, M., et al. (2019). Obstructive sleep apnea syndrome in the pediatric age: The role of the pneumologist. Eur. Rev. Med. Pharmacol. Sci. 1, 15–18. doi: 10.26355/eurrev_201903_17342
Tu, B. P., and Weissman, J. S. (2002). The FAD- and O2-dependent reaction cycle of Ero1-mediated oxidative protein folding in the endoplasmic reticulum. Mol. Cell 10, 983–994. doi: 10.1016/s1097-2765(02)00696-2
Uddin, M. S., Mamun, A. A., Jakaria, M., Thangapandiyan, S., Ahmad, J., Rahman, M. A., et al. (2020). Emerging promise of sulforaphane-mediated Nrf2 signaling cascade against neurological disorders. Sci. Total Environ. 707:135624. doi: 10.1016/j.scitotenv.2019.135624
Udholm, N., Rex, C. E., Fuglsang, M., Lundbye-Christensen, S., Bille, J., and Udholm, S. (2022). Obstructive sleep apnea and road traffic accidents: A Danish nationwide cohort study. Sleep Med. 96, 64–69. doi: 10.1016/j.sleep.2022.04.003
Vakulin, A., Green, M. A., D’Rozario, A. L., Stevens, D., Openshaw, H., Bartlett, D., et al. (2022). Brain mitochondrial dysfunction and driving simulator performance in untreated obstructive sleep apnea. J. Sleep Res. 31, e13482. doi: 10.1111/jsr.13482
Vanek, J., Prasko, J., Genzor, S., Ociskova, M., Kantor, K., Holubova, M., et al. (2020). Obstructive sleep apnea, depression and cognitive impairment. Sleep Med. 72, 50–58. doi: 10.1016/j.sleep.2020.03.017
Vardanian, M., and Ravdin, L. (2022). Cognitive Complaints and Comorbidities in Obstructive Sleep Apnea. Sleep Med. Clin. 17, 647–656. doi: 10.1016/j.jsmc.2022.07.009
Verstraeten, E., and Cluydts, R. (2004). Executive control of attention in sleep apnea patients: Theoretical concepts and methodological considerations. Sleep Med. Rev. 8, 257–267. doi: 10.1016/j.smrv.2004.01.001
Wallace, A., and Bucks, R. S. (2013). Memory and obstructive sleep apnea: A meta-analysis. Sleep 36, 203–220. doi: 10.5665/sleep.2374
Walter, L. M., Nixon, G. M., Davey, M. J., O’Driscoll, D. M., Trinder, J., and Horne, R. S. (2011). Sleep disturbance in pre-school children with obstructive sleep apnoea syndrome. Sleep Med. 12, 880–886. doi: 10.1016/j.sleep.2011.07.007
Wang, D. D., Li, J., Yu, L. P., Wu, M. N., Sun, L. N., and Qi, J. S. (2016). Desipramine improves depression-like behavior and working memory by up-regulating p-CREB in Alzheimer’s disease associated mice. J. Integr. Neurosci. 15, 247–260. doi: 10.1142/S021963521650014X
Wang, J., Ming, H., Chen, R., Ju, J. M., Peng, W. D., Zhang, G. X., et al. (2015). CIH-induced neurocognitive impairments are associated with hippocampal Ca2+ overload, apoptosis, and dephosphorylation of ERK1/2 and CREB that are mediated by overactivation of NMDARs. Brain Res. 1625, 64–72. doi: 10.1016/j.brainres.2015.08.012
Wilckens, K. A., Ferrarelli, F., Walker, M. P., and Buysse, D. J. (2018). Slow-wave activity enhancement to improve cognition. Trends Neurosci. 41, 470–482. doi: 10.1016/j.tins.2018.03.003
Wu, X., Gong, L., Xie, L., Gu, W., Wang, X., Liu, Z., et al. (2021). NLRP3 deficiency protects against intermittent hypoxia-induced neuroinflammation and mitochondrial ROS by promoting the PINK1-parkin pathway of mitophagy in a murine model of sleep apnea. Front. Immunol. 12:628168. doi: 10.3389/fimmu.2021.628168
Xie, H., Leung, K. L., Chen, L., Chan, Y. S., Ng, P. C., Fok, T. F., et al. (2010). Brain-derived neurotrophic factor rescues and prevents chronic intermittent hypoxia-induced impairment of hippocampal long-term synaptic plasticity. Neurobiol. Dis. 40, 155–162. doi: 10.1016/j.nbd.2010.05.020
Xu, L., Bi, Y., Xu, Y., Wu, Y., Du, X., Mou, Y., et al. (2021a). Suppression of CHOP reduces neuronal apoptosis and rescues cognitive impairment induced by intermittent hypoxia by inhibiting bax and bak activation. Neural Plast. 2021:4090441. doi: 10.1155/2021/4090441
Xu, Y. P., Tao, Y. N., Wu, Y. P., Zhang, J., Jiao, W., Wang, Y. H., et al. (2021b). Sleep deprivation aggravates brain injury after experimental subarachnoid hemorrhage via TLR4-MyD88 pathway. Aging 13, 3101–3111. doi: 10.18632/aging.202503
Xu, L., Yang, Y., and Chen, J. (2020). The role of reactive oxygen species in cognitive impairment associated with sleep apnea. Exp. Ther. Med. 20:4. doi: 10.3892/etm.2020.9132
Xu, L. H., Xie, H., Shi, Z. H., Du, L. D., Wing, Y. K., Li, A. M., et al. (2015). Critical role of endoplasmic reticulum stress in chronic intermittent hypoxia-induced deficits in synaptic plasticity and long-term memory. Antioxid. Redox. Signal 23, 695–710. doi: 10.1089/ars.2014.6122
Xue, X., Qiu, Y., and Yang, H. L. (2017). Immunoregulatory role of MicroRNA-21 in macrophages in response to bacillus calmette-guerin infection involves modulation of the TLR4/MyD88 signaling pathway. Cell Physiol. Biochem. 42, 91–102. doi: 10.1159/000477118
Yang, X. Y., Geng, L., Li, R., Song, J. X., Jia, C. L., An, J. R., et al. (2023). Huperzine A-liposomes efficiently improve neural injury in the hippocampus of mice with chronic intermittent hypoxia. Int. J. Nanomed. 18, 843–859. doi: 10.2147/IJN.S393346
Yin, X., Zhang, X., Lv, C., Li, C., Yu, Y., Wang, X., et al. (2015). Protocatechuic acid ameliorates neurocognitive functions impairment induced by chronic intermittent hypoxia. Sci. Rep. 5:14507. doi: 10.1038/srep14507
Yuan, L., Wu, J., Liu, J., Li, G., and Liang, D. (2015a). Intermittent hypoxia-induced parvalbumin-immunoreactive interneurons loss and neurobehavioral impairment is mediated by NADPH-oxidase-2. Neurochem. Res. 40, 1232–1242. doi: 10.1007/s11064-015-1586-1
Yuan, X., Guo, X., Deng, Y., Zhu, D., Shang, J., and Liu, H. (2015b). Chronic intermittent hypoxia-induced neuronal apoptosis in the hippocampus is attenuated by telmisartan through suppression of iNOS/NO and inhibition of lipid peroxidation and inflammatory responses. Brain Res. 1596, 48–57. doi: 10.1016/j.brainres.2014.11.035
Zhang, C. Q., Yi, S., Chen, B. B., Cui, P. P., Wang, Y., and Li, Y. Z. (2021). mTOR/NF-kappaB signaling pathway protects hippocampal neurons from injury induced by intermittent hypoxia in rats. Int J Neurosci 131, 994–1003. doi: 10.1080/00207454.2020.1766460
Zhang, D. D., Li, H. J., Zhang, H. R., and Ye, X. C. (2022). Poria cocos water-soluble polysaccharide modulates anxiety-like behavior induced by sleep deprivation by regulating the gut dysbiosis, metabolic disorders and TNF-alpha/NF-kappaB signaling pathway. Food Funct. 13, 6648–6664. doi: 10.1039/d2fo00811d
Zhang, L., Zhang, H. Q., Liang, X. Y., Zhang, H. F., Zhang, T., and Liu, F. E. (2013). Melatonin ameliorates cognitive impairment induced by sleep deprivation in rats: Role of oxidative stress. BDNF and CaMKII. Behav. Brain Res. 256, 72–81. doi: 10.1016/j.bbr.2013.07.051
Zhang, P., Wang, Y., Wang, H., and Cao, J. (2021). Sesamol alleviates chronic intermittent hypoxia-induced cognitive deficits via inhibiting oxidative stress and inflammation in rats. Neuroreport 32, 105–111. doi: 10.1097/WNR.0000000000001564
Zhang, S. X., Khalyfa, A., Wang, Y., Carreras, A., Hakim, F., Neel, B. A., et al. (2014). Sleep fragmentation promotes NADPH oxidase 2-mediated adipose tissue inflammation leading to insulin resistance in mice. Int. J. Obes. 38, 619–624. doi: 10.1038/ijo.2013.139
Zhang, Y., Miao, Y., Xiong, X., Tan, J., Han, Z., Chen, F., et al. (2023). Microglial exosomes alleviate intermittent hypoxia-induced cognitive deficits by suppressing NLRP3 inflammasome. Biol. Direct. 18:29. doi: 10.1186/s13062-023-00387-5
Keywords: obstructive sleep apnea, intermittent hypoxia, sleep fragmentation, cognitive impairment, drug therapy
Citation: He D, Chen J, Du X and Xu L (2023) Summary of drug therapy to treat cognitive impairment-induced obstructive sleep apnea. Front. Cell. Neurosci. 17:1222626. doi: 10.3389/fncel.2023.1222626
Received: 15 May 2023; Accepted: 01 August 2023;
Published: 04 September 2023.
Edited by:
Robert Warren Gould, Wake Forest University, United StatesReviewed by:
Anne Briançon-Marjollet, Université Grenoble Alpes, FranceShiyong Li, Huazhong University of Science and Technology, China
Copyright © 2023 He, Chen, Du and Xu. This is an open-access article distributed under the terms of the Creative Commons Attribution License (CC BY). The use, distribution or reproduction in other forums is permitted, provided the original author(s) and the copyright owner(s) are credited and that the original publication in this journal is cited, in accordance with accepted academic practice. No use, distribution or reproduction is permitted which does not comply with these terms.
*Correspondence: Xiaoxue Du, ZHhpYW94dWVAemp1LmVkdS5jbg==; Linhao Xu, eHVsaW5oYW9sdWNrQDE2My5jb20=
†These authors have contributed equally to this work