- 1Department of Psychiatry and Human Behavior, University of Mississippi Medical Center, Jackson, MS, United States
- 2Graduate Program in Neuroscience, University of Mississippi Medical Center, Jackson, MS, United States
Substance use disorders are a global health problem with increasing prevalence resulting in significant socioeconomic burden and increased mortality. Converging lines of evidence point to a critical role of brain extracellular matrix (ECM) molecules in the pathophysiology of substance use disorders. An increasing number of preclinical studies highlight the ECM as a promising target for development of novel cessation pharmacotherapies. The brain ECM is dynamically regulated during learning and memory processes, thus the time course of ECM alterations in substance use disorders is a critical factor that may impact interpretation of the current studies and development of pharmacological therapies. This review highlights the evidence for the involvement of ECM molecules in reward learning, including drug reward and natural reward such as food, as well as evidence regarding the pathophysiological state of the brain’s ECM in substance use disorders and metabolic disorders. We focus on the information regarding time-course and substance specific changes in ECM molecules and how this information can be leveraged for the development of therapeutic strategies.
Introduction
Substance use disorders (SUD) are a debilitating group of psychiatric disorders that affect approximately 7% of people in the United States each year (SAMHSA, 2018; Grant et al., 2016). Relapse is a major factor limiting recovery from SUD and points to the strength of memory circuits involved in reward memory processing (Niaura et al., 1988; Carter and Tiffany, 1999; Weiss, 2005; Zironi et al., 2006; Janak and Chaudhri, 2010). Converging evidence from rodent models and human postmortem studies suggest a key role of extracellular matrix (ECM) molecules in the formation and maintenance of reward memories (Brown et al., 2007; Smith et al., 2011, 2015; Xue et al., 2014; Slaker et al., 2015, 2016a; Chioma et al., 2021; Seney et al., 2021; Browne et al., 2023). In addition, ECM abnormalities have been reported in several psychiatric disorders in which dysfunction in memory processing and synaptic regulation are key features, including schizophrenia (Eastwood and Harrison, 2006; Pantazopoulos et al., 2010, 2015; Enwright et al., 2016; Steullet et al., 2018), post-traumatic stress disorder (Gogolla et al., 2009), major depressive disorder (MDD) (Riga et al., 2017; Alaiyed et al., 2020; Blanco and Conant, 2021), and bipolar disorder (BD) (Fatemi et al., 2000; Pantazopoulos et al., 2015; Steullet et al., 2018) – each of which have significant comorbidity with SUD.
Involvement of the ECM in the regulation of reward memories may represent a shared feature across reward processes involved in strengthening memories necessary for survival such as food seeking. Several lines of evidence indicate that food and drugs of abuse activate overlapping brain circuitry (Blum et al., 2012; Volkow et al., 2013a). Furthermore, as the incidence of obesity and metabolic disorders continues to mount in the U.S., there has been an increasing interest in combining habitual overeating and drug abuse within a common diagnostic framework of disorders of addiction (Barry et al., 2009; Alsio et al., 2012; Blum et al., 2012; Baik, 2013; Volkow et al., 2013a,b). Growing evidence supports the involvement of ECM molecules in reward processes involved in high fat, high calorie diets that may contribute to metabolic disorders including obesity. The strength of reward memories contributes to relapse and habit forming, and targeting ECM processes to alleviate reward memory strength represents a promising strategy for the development of new treatments for SUD and obesity (Niaura et al., 1988; Carter and Tiffany, 1999; Lu et al., 2004; Weiss, 2005; Zironi et al., 2006; Janak and Chaudhri, 2010).
A growing number of studies demonstrate a dynamic regulation of the ECM during learning processes, including fear and reward learning (Gogolla et al., 2009; Slaker et al., 2016a). Evidence from over two decades of research indicates that the ECM is regulated by rewarding substances in a complex experience-dependent manner which may impact interpretation of the disease processes in SUD and metabolic disorders as well as treatment strategies. We summarize the current evidence for the involvement of the ECM in reward memory processes, SUD and obesity with an emphasis on how the ECM is modulated in a substance- and time-specific manner.
The brain extracellular matrix
The brain extracellular matrix (ECM) is a network of chondroitin sulfate proteoglycans (CSPGs), heparan sulfate proteoglycans, glycoproteins, hyaluronan, and other molecules including axon guidance and cell adhesion molecules such as semaphorins and integrins (Table 1; Figure 1). In the brain, ECM molecules form several specialized structures including perineuronal nets (PNNs), perisynaptic ECM, perivascular ECM, and axonal coats (Schuster et al., 2001; Bruckner et al., 2008; Bekku et al., 2009; Dours-Zimmermann et al., 2009; Morawski et al., 2010; Baeten and Akassoglou, 2011; Lendvai et al., 2012; Susuki et al., 2013; Thomsen et al., 2017; Pantazopoulos et al., 2022; Tabet et al., 2022; Miguel-Hidalgo, 2023; Figure 1). These ECM structures are involved in a wide range of processes implicated in SUD including stabilization of synapses, axon guidance during neurodevelopment, regulation of diffusion of molecules such as neurotransmitters, ions, and metabolites, neuronal firing rates, receptor trafficking, protection from oxidative stress, and regulation of the blood-brain barrier (Hartig et al., 1999; Ishii and Maeda, 2008; Maeda, 2015). ECM molecules also have broad, complex roles in neurodevelopmental processes and brain injury [for reviews see Silver and Silver, 2014; Peters and Sherman, 2020; Carulli and Verhaagen, 2021; Fawcett and Kwok, 2022; Siddiqui et al., 2022; Schwartz and Domowicz, 2023]. CSPGs have been the primary focus of preclinical addiction studies and are one of the key proteoglycan families in the central nervous system (CNS). CSPGs are composed of a core protein with a varying number of covalently attached chondroitin sulfated glycosaminoglycan chains consisting of repeated pairs of glucuronic acid (GlcA) and N-acetyl-galactosamine (GalNAc) (Figure 1). The predominant CSPGs within the CNS are aggrecan, brevican, neurocan, phosphacan, neuron-glial antigen 2 (NG2), neuroglycan-C and versican (Milev et al., 1998; Deepa et al., 2006; Giamanco and Matthews, 2012). In addition to the specific functions of the core proteins, the glycosaminoglycan chains can vary in number and length, and are sulfated in various positions, which significantly contributes to the function of CSPGs (Asher et al., 2001; Wang et al., 2008; Maeda, 2010; Karus et al., 2012; Miyata et al., 2012; Alonge et al., 2019). For example, synaptic plasticity is regulated by chondroitin sulfation. CS-4 sulfation inhibits axonal growth (Smith-Thomas et al., 1995; Wang et al., 2008), whereas CS-6 is permissive for axonal growth (Lin et al., 2011; Miyata et al., 2012).
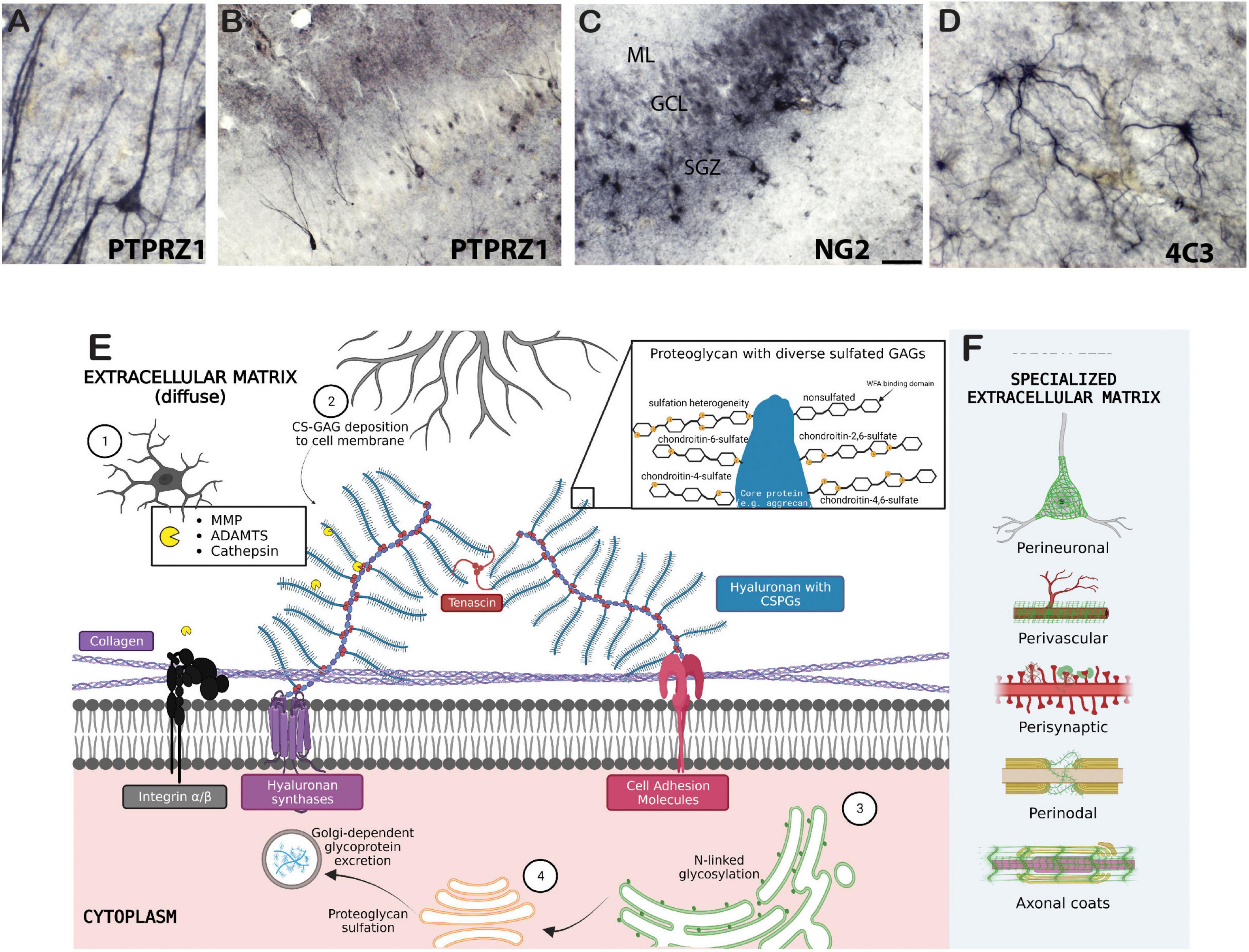
Figure 1. Biology of the extracellular matrix. Examples of chondroitin sulfate proteoglycan labeling in several cell types in the human brain include (A,B) immunohistochemical labeling of PTPRZ1 (phosphacan) neurons and glia in the human hippocampus; (C) NG2 labeled glial cells in the human hippocampus; and (D) 4C3 (specific CS sulfation motif) glial cell labeling in the human amygdala. (E) 1, microglia; 2, astrocyte; 3, rough endoplasmic reticulum (ER); 4, Golgi complex. Collagen proteins are tethered to the cell membrane by transmembrane integrins and other cell adhesion molecules. Cell adhesion molecules also attach to hyaluronan and CSPGs, which are attached to other CSPGs via tenascins. CSPGs are cleaved by endogenous proteases secreted by microglia, and are formed by intraneuronal rough-ER and Golgi-dependent processes. Further deposition of CS-glycosaminoglycans (GAGs) on neuron cell membranes is extraneously controlled astrocytes and oligodendrocytes. The zoomed in panel depicts sulfation heterogeneity of CS-GAGs as well as the non-sulfated binding domain of Wisteria floribunda agglutinin (WFA) lectin. (F) Specialized ECM structures are illustrated in green. Created with BioRender.com.
Chondroitin sulfate proteoglycans form several specialized structures in the brain in addition to the diffuse ECM which exists around all cells within the CNS (Figure 1). PNNs represent the most well-studied of these structures. PNNs are composed of highly condensed ternary ECM molecules including CSPGs, hyaluronan, and tenascin and surround the soma, proximal dendrites, and axon initial segments of neurons, typically inhibitory fast-firing interneurons expressing parvalbumin (Figure 2; Hartig et al., 1994, 2022; Adams et al., 2001; Pantazopoulos et al., 2006). PNNs are involved in a broad range of functions including stabilization of synaptic plasticity, protection from oxidative stress, regulation of neuronal firing properties, N-methyl-D-aspartate (NMDA) receptor trafficking, and maintenance of ionic homeostasis (Kalb and Hockfield, 1988; Pizzorusso et al., 2002; Morawski et al., 2004; Sugiyama et al., 2008; Frischknecht et al., 2009; Gogolla et al., 2009; Dityatev et al., 2010; Gundelfinger et al., 2010; Frischknecht and Gundelfinger, 2012; Cabungcal et al., 2013; Wingert and Sorg, 2021). The role of PNNs as stabilizers of synaptic connections places them at the intersection of formation and consolidation of memories. PNNs are commonly labeled using the plant lectin Wisteria floribunda agglutinin (WFA), which binds to non-sulfated N-acetylgalactosamine residues at the terminal ends of CSPG saccharide chains (Hartig et al., 1994; Nadanaka et al., 2020). However, PNNs are complex structures with diverse composition, and labeling with antibodies directed against core CSPG proteins, glycoproteins, or specific sulfation motifs detects varying, partially overlapping populations of PNNs (Ajmo et al., 2008; Pantazopoulos et al., 2015; Dauth et al., 2016; Hartig et al., 2022; Scarlett et al., 2022). Several studies have demonstrated that the distribution of ECM molecules, including CSPGs and PNNs in the brain, varies greatly in a brain region and age specific manner (Mauney et al., 2013; Dauth et al., 2016; Ueno et al., 2017, 2018, 2019; Rogers et al., 2018; Mafi et al., 2020; Lupori et al., 2023).
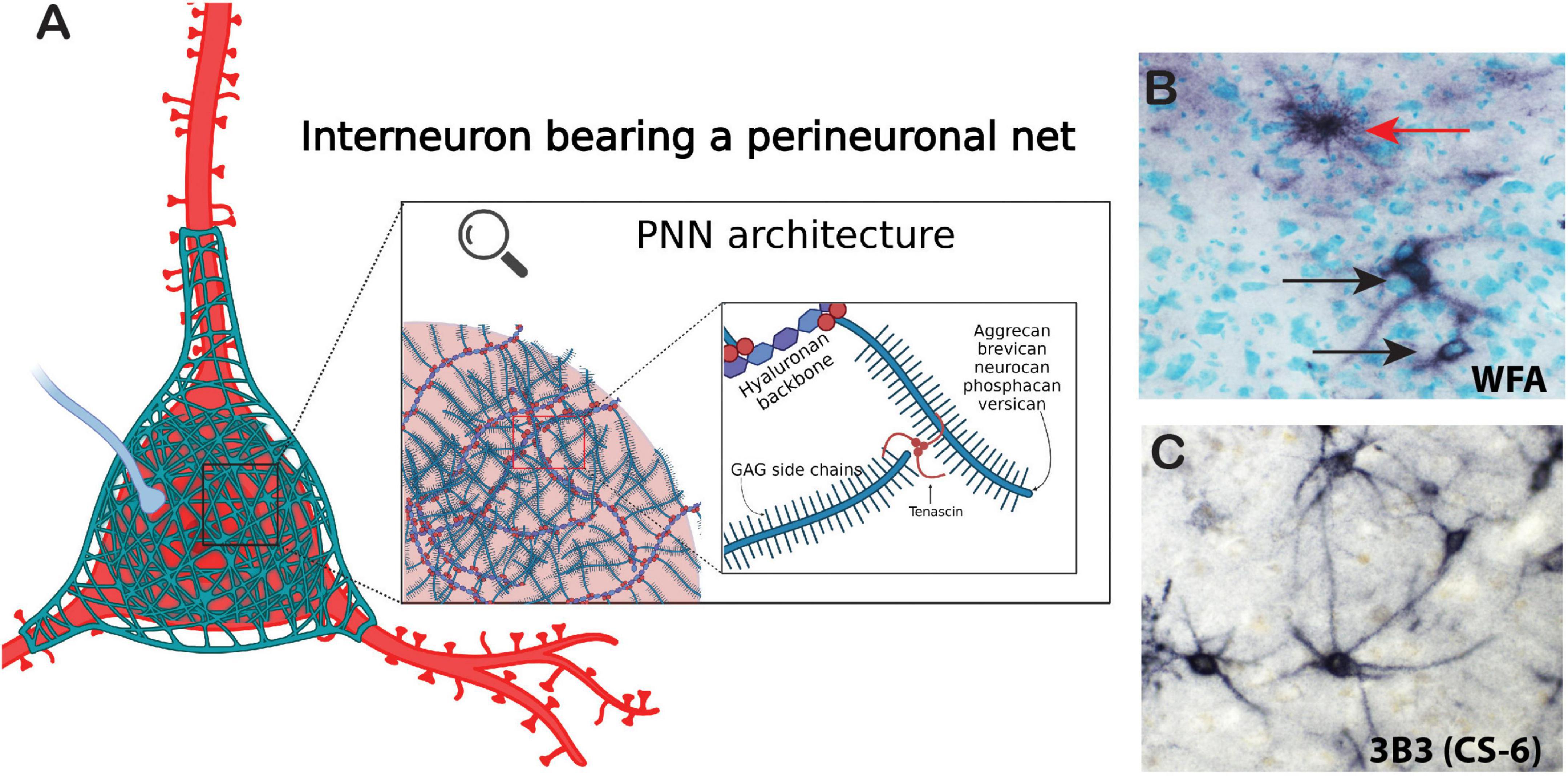
Figure 2. Architecture of the perineuronal net. Perineuronal nets are a meshwork of ECM components including chondroitin sulfate proteoglycan core proteins with varying numbers of CS-GAG chains, semaphorins, and tenascin-R. (A) Chondroitin sulfate core proteins are linked together by tenascins and to a hyaluronan backbone by hyaluronan link proteins (red spheres). (B) Immunohistochemical labeling of WFA (non-sulfated N-acetylgalactosamine). PNNs (black arrows) and astrocyte (red arrow) in the human brain. (C) Immunohistochemical labeling of the chondroitin-6-sulfation motif on PNNs in the human brain with labeled with the antibody 3B3. Created with BioRender.com.
Chondroitin sulfate proteoglycans also form several distinct structures in the brain in addition to PNNs (Figure 1). For example, ECM molecules including CSPGs condense as perisynaptic ECM aggregates on dendritic spines and contribute to synaptic homeostasis (Schuster et al., 2001). ECM molecules form perinodal structures at nodes of Ranvier along myelinated axons, where they contribute to axonal conductance through regulation of gap junctions by several processes including sodium channel clustering (Bekku et al., 2009; Dours-Zimmermann et al., 2009; Susuki et al., 2013; Pantazopoulos et al., 2022; Miguel-Hidalgo, 2023). CSPGs also interweave within myelin sheaths to form periaxonal aggregates called axonal coats (Bruckner et al., 2008; Morawski et al., 2010; Lendvai et al., 2012; Pantazopoulos et al., 2022). Furthermore, ECM molecules are critically involved in regulation of the blood-brain barrier and immune signaling through perivascular ECM structures (Baeten and Akassoglou, 2011; Thomsen et al., 2017; Tabet et al., 2022).
Recent studies demonstrate that the ECM, once thought to represent a stable component of the brain, is regularly modified in an activity-dependent manner through a complex combination of proteolytic remodeling and ECM recycling processes (Nagy et al., 2007; Brown et al., 2009; Ganguly et al., 2013; Dankovich and Rizzoli, 2022). Matrix metalloproteinases (MMP), a disintegrin and metalloproteinase with thrombospondin motifs (ADAMTS), and cathepsins are the putative endogenous proteases involved in degradation of ECMs (Page-McCaw et al., 2007; Mohamedi et al., 2020; Tran and Silver, 2021). These proteases are secreted by astrocytes, microglia, and neurons, indicating that individual neurons may, in part, be able to regulate the composition of their own PNNs (Rossier et al., 2015; Nguyen et al., 2020). MMPs, part of the metzincin superfamily, consist of several functional classes including collagenases, stromelysins, and gelatinases, with the gelatinases MMP-2, and MMP-9 and the stromelysin MMP-3 representing the predominant MMPs in the brain [for review see Rivera et al., 2010 and Brzdak et al., 2017]. ADAMTSs also serve as the substrate for tissue inhibitors of metalloproteinases (TIMP) which bind to these proteases and inhibit ECM proteolysis (Brew et al., 2000). Other proteolytic enzymes include tissue plasminogen activator (tPA) and urokinase plasminogen activator (uPA), which cleave and activate plasminogens that can degrade various ECM molecules, including proteoglycans (Saksela, 1985). Endogenous ECM proteases have been reported to modify the ECM during learning, which may facilitate formation of new synapses in response to environmental stimuli (Nagy et al., 2007; Brown et al., 2009; Ganguly et al., 2013). Recent studies have also demonstrated that ECM molecules, including PNNs, are modified in a diurnal manner, which may contribute to memory consolidation processes (Harkness et al., 2019; Pantazopoulos et al., 2020; Gisabella et al., 2021). Taken together, these studies suggest that the ECM is modified in a time-dependent manner which may impact interpretation of studies regarding the role of the ECM in SUD and the development of therapeutic strategies targeting the ECM. Within this context, we review the current literature regarding the involvement of the ECM in reward learning, including drugs of abuse and natural rewards such as high fat diet, and the current evidence for ECM pathology in SUD with an emphasis on time-course dependent findings.
Dynamic extracellular matrix regulation by drugs of abuse
A number of studies have investigated the role of the ECM in animal models and human studies of SUD. Investigations have spanned an array of brain regions, including the prefrontal cortex (PFC) (Goldstein and Volkow, 2011), nucleus accumbens (NAc) (Quintero, 2013), ventral tegmental area (VTA) (Oliva and Wanat, 2016), hippocampus (Kutlu and Gould, 2016), amygdala (Warlow et al., 2017), cerebellum (Moulton et al., 2014), and hypothalamus (Zhang et al., 2020). Together, these brain regions are involved in regulating the rewarding and reinforcing effects of drugs, withdrawal symptoms, and the integration of memories of the rewarding effect of the drug and the associated context. Importantly, several studies cited throughout this review have varying definitions of the terms “acute” and “chronic.” For the purpose of this review, we define acute drug exposures as less than a period of 24-h (<2 h for “immediate”), and chronic drug exposure as greater than 3 consecutive daily sessions.
Psychostimulants
Stimulant drugs such as cocaine and methamphetamine produce profound effects on CNS function including euphoria and increased motor activity/endurance (Nestler, 2005; Vazquez-Sanroman et al., 2015). Overuse of stimulant drugs can progress to the development of SUD and can also contribute to other serious conditions including psychosis, mood disorders, anxiety, and sleep/circadian rhythm dysfunction (American Psychiatric Association, 2013). Comorbidity of stimulant use with these disorders greatly complicates clinical interpretations and interventions for SUD as well as the comorbid conditions. Currently, there are no FDA-approved cessation pharmacotherapies for stimulant use disorder. Substantial evidence exists for a key role of the ECM in the formation and maintenance of stimulant use disorder endophenotypes (e.g., pervasive memories surrounding stimulant intoxication, withdrawal, and maintained use) (Table 2; Slaker et al., 2016a).
Acute stimulant exposure
An intriguing collection of studies have examined the acute response of ECM molecules to psychostimulant drugs. Integrins serve as a tether for cell membrane-bound condensed ECM and also are involved in cytoskeleton signaling cascades that create a bidirectional interplay of the local ECM and intraneuronal signaling pathways, sometimes serving as the substrate for MMPs (ffrench-Constant and Colognato, 2004; Mezu-Ndubuisi and Maheshwari, 2021; Samuel et al., 2023). Temporally, β1-integrin protein levels in the NAc of mice are significantly decreased 30 min after a cocaine injection, but not at zero or 120 min (Wiggins et al., 2009). Conditional knockdown of β3 integrins in the mouse NAc during cocaine self-administration training prevents cue-induced cocaine seeking and transient excitatory synaptic potentiation (Garcia-Keller et al., 2019), suggesting that the acute increase of β-integrins may be involved in formation of cocaine associated memories. Activating endogenous ECM proteases, such as MMPs, using tPA increases cue-induced cocaine seeking (Garcia-Keller et al., 2019). In line with this, lentivirus-mediated upregulation of uPA (another endogenous MMP activator) in rats potentiates cocaine place preference acquisition (Bahi et al., 2008). Interestingly, knockdown of β3-integrin ameliorates the effect of tPA-induced seeking (Garcia-Keller et al., 2019). Decreased integrin during reward learning may not only inhibit the consolidation of cocaine-associated cues, but also the functions of neurons that are typically ensheathed by PNNs. Another study in rats measured hippocampal MMP protein levels 3 h following nicotine place preference conditioning, demonstrating relatively mild increases of MMP-2 and -9 during the early training sessions, and a much greater nicotine-induced MMP-9 upregulation during later conditioning sessions (Natarajan et al., 2013). Importantly, nicotine acts through mechanisms which are distinct from other psychostimulants of abuse (i.e., cholinergic stimulation of mesolimbic dopamine) and its effects as a reinforcer rely heavily on learning the cues paired with its administration (McClernon et al., 2016). Thus, progressively increased MMP induction over the course of nicotine CPP training may reflect contextual learning mechanisms induced by nicotine.
While acute exposure to stimulants appears to upregulate many ECM proteases, PNN fluorescent intensity 2 h after initial cocaine exposure is significantly decreased in the rat PFC coinciding with increased inhibitory glutamic acid decarboxylase- (GAD) 65/67 synaptic puncta on PNN-coated parvalbumin neurons (Slaker et al., 2018). Reduced PNN composition at 2 h corresponds with findings from a prior study which identified increased expression of tPA, an upstream promoter of MMPs (Tsuji et al., 2005), 30 min following a cocaine injection (Maiya et al., 2009). In comparison, differential effects of semaphorin 3a, a related ECM molecule, were observed between the rat hippocampus and NAc (Bahi and Dreyer, 2005). One day after receiving a bolus cocaine injection, expression of semaphorin 3a, a chemorepulsive protein involved in regulating PNN plasticity (Dick et al., 2013; de Winter et al., 2016), is significantly increased in the hippocampus, but decreased in the NAc (Bahi and Dreyer, 2005). Semaphorin 3a binds to the 4,6-sulfated CS in PNNs and decreased semaphorin 3a is associated with enhanced plasticity of neurons surrounded by PNNs (Dick et al., 2013; de Winter et al., 2016), suggesting that PNNs in these regions are differentially regulated by acute cocaine exposure. Further studies regarding brain region-specific changes in PNN components as well as perisynaptic ECM and axonal coats following acute exposure to psychostimulants will provide insight into the specific role of the ECM in synaptic plasticity during psychostimulant use.
Chronic stimulant use
Extracellular matrix molecules have been extensively studied during chronic stimulant models of self-administration, extinction, and reinstatement. Additionally, effects of PNN digestion using the exogenous enzyme chondroitinase ABC (chABC) have been examined at various timepoints across drug administration protocols by several groups [for review see Slaker et al. (2016a)]. Neuroinflammation is a hallmark of chronic stimulant use, and is induced by direct actions of these drugs on microglia resulting in upregulated inflammatory cytokines (Kohno et al., 2019). However, evidence suggests that stimulant use does not result in persistent microgliosis (Narendran et al., 2014). There is a current lack of evidence regarding how neuroinflammation and ECM alterations intersect in the acute vs. chronic phases of stimulant use disorder.
Protracted exposure to cocaine increases the numerical density and intensity of PNNs in several brain regions, such as the PFC (prelimbic/infralimbic cortex) and cerebellum, whereas nicotine decreases numerical densities of PNNs in the VTA and orbitofrontal cortex (Slaker et al., 2015, 2018; Vazquez-Sanroman et al., 2015, 2017; Guarque-Chabrera et al., 2022). Increased expression of several semaphorin genes was reported in the hippocampus, NAc, caudate putamen, and VTA of rats during chronic cocaine exposure and relapse (Bahi and Dreyer, 2005), suggesting that semaphorin expression may contribute to increased PNNs in these regions during chronic cocaine exposure. In addition to changes in PNNs and semaphorins, HSPGs, such as syndecan-3, are altered after prolonged exposure to cocaine and methamphetamine, increasing both in disaccharide content and total sulfation (Chen et al., 2013, 2017). Interestingly, another study reported no significant effect of 5 days of cocaine exposure on sulfation of diffuse CS, HS or hyaluronan (Slaker et al., 2018). The composition and functional properties of HS and CS proteoglycan/GAG families have a great deal of similarity, both subserving synaptic homeostasis and acting as structural chemorepellent barriers to neuroplasticity (Maeda et al., 2011). β1-integrin protein levels also undergo a ∼1.5-fold increase after prolonged withdrawal from chronic cocaine exposure (Wiggins et al., 2009). Further, expression of tissue inhibitors of MMPs (TIMPs) is significantly upregulated following repeated methamphetamine exposures (Mizoguchi et al., 2007) indicating that suppression of MMPs after prolonged stimulant use may reduce PNN degradation and contribute to the reported increases in PNNs following chronic drug use. In comparison, multiple studies converge on upregulation of proteolytic activity, including increased MMP expression, upon re-exposure to either stimulant-associated cues (i.e., stimulus lights in self-administration chambers, drug-paired CPP chambers) or stimulants alone (Brown et al., 2007, 2008; Smith et al., 2014; Garcia-Keller et al., 2020; Lewandowski et al., 2023). Importantly, the increase in inhibitory (GAD-65/67) synaptic puncta in the PFC during acute cocaine exposure is retained throughout chronic exposure (Slaker et al., 2018). Removal of PNNs using chABC in the anterior dorsal lateral hypothalamus, amygdala or PFC inhibits stimulant-induced place preference and reinstatement of stimulant self-administration (Xue et al., 2014; Slaker et al., 2015; Blacktop et al., 2017; Marchant, 2019). A human postmortem study of the hippocampus in subjects with history of cocaine use identified significantly decreased protein levels of the active form of MMP-9, along with a corresponding upregulation of several cell adhesion genes (Mash et al., 2007). Taken together, studies on stimulants suggest a biphasic modification of ECM over the course of stimulant use. MMPs increase during the acute phase of drug exposure, presumably to degrade PNNs to allow the formation of new synaptic connections involved in reward memory encoding. After prolonged use, MMPs decrease in activity which contributes to decreased modification of CSPGs and HSPGs and in turn the reported increase in PNN composition, which may stabilize the newly formed synapses involved in reward memory and contribute to relapse.
Opioids
The significant abuse liability and mortality of opioids has gained attention over the past couple of decades due to the rise in overdose deaths from illicit and synthetic opioid analogs, particularly heroin and fentanyl (Spencer et al., 2022). Prescription opioids are highly effective pain-relieving medicines; however, they can also lead to dependence, resulting in misuse and development of opioid use disorder (OUD). Thus, the development of new prescription opioids, or additives to opioids, that retain analgesic qualities and reduce the risk of dependence is a current research focus (Negus and Freeman, 2018). A majority of heroin users report using opioids for the first time early in life (Jones, 2013), a critical period of brain circuit wiring where ECM molecules are involved in shaping later stage maturation of brain circuits including the formation of PNNs (Pizzorusso et al., 2002; Gogolla et al., 2009; Mauney et al., 2013; O’Connor et al., 2019; Nguyen et al., 2020; Gisabella et al., 2021). Furthermore, several lines of evidence propose a critical role of the ECM in the analgesic and rewarding effects of opioids (Table 3; Kruyer et al., 2020; Ray et al., 2022).
Acute opioid exposure
There is extensive evidence suggesting that the ECM in the CNS is altered by pain, Nakamoto et al. (2012); Ishiguro et al. (2014); Tajerian and Clark (2015); Hu et al. (2021); Mascio et al. (2022); Tansley et al. (2022). Together these studies indicate that microglia degrade PNNs in the spinal cord when pain levels are high to disinhibit the spinal cord nociceptive afferents that evoke pain perception. Chronic pain results in an increased abundance of PNNs, possibly to stabilize newly formed synapses involved in the discriminative and emotional aspects of pain (Mascio et al., 2022). There is, however, relatively limited evidence regarding the immediate effects (0 to 2 h) of opioids on the ECM, particularly regarding the potential role of the ECM as it pertains to pain relief processes by opioids in multiple modalities of nociception (e.g., thermal, inflammatory, neuropathic). Studies indicate that acute administration of morphine dose-dependently decreases MMP-2, MMP-9, and laminin-4 (LAMA-4) expression in both breast cancer and human brain microvascular endothelial cell lines from 3 h up to 72 h (Gach et al., 2011; Vujic et al., 2022). Speculatively, the 3-h timepoint examined in cell lines may capture a period after prior increases in MMP expression, considering that levels of laminins are also decreased. In line with this, MMP-2 and -9 display increased activity around dendritic spines of dopamine 1 (D1) receptor- medium spiny neurons in the rat NAc during heroin reinstatement (Chioma et al., 2021). Rapid proteolytic induction may also reflect opioid activation of interleukin-33 expression and intracellular microglial cascades downstream of the IL1RL1/ST2 receptor involved in memory processing, which would increase expression of several ECM proteases to facilitate synaptic plasticity (Nguyen et al., 2020; Hu et al., 2021). Alternatively, acute decreases of MMP activity in response to opioids may contribute to their mechanism of analgesia, as acute pain is associated with increased MMP expression and degradation of the ECM (Tansley et al., 2022). Whether effects on the ECM incurred by opioids in the spinal cord and brain are congruent or divergent is yet to be determined. It is possible that opioids have regional effects on the ECM. For example, in the brain, opioids may contribute to synaptic plasticity underlying drug-associated memories, whereas in the spinal cord, opioids may act on the ECM to inhibit excitatory nociceptive afferents. As evidence in this field continues to mount, it will be important for future studies to improve our understanding of the temporal ECM changes in response to opioids and how they interact with the effect of pain on the ECM.
Chronic opioid use
Early work examined ECM molecules in the brain of rats following heroin self-administration (Van den Oever et al., 2010). Acute abstinence from opioids (1 day) is associated with an increased numerical density of PNNs in the PFC. Long-term abstinence, however, (21-30 days), is associated with a decrease in protein levels of the PNN components tenascin-R and brevican in the medial PFC and NAc, but no significant difference in WFA labeled PNN numerical densities compared to control animals (Van den Oever et al., 2010; Roura-Martinez et al., 2020). This suggests that PNN composition may be modified, or the changes in tenascin-R and brevican occur on other ECM structures distinct from PNNs. Alternatively, multiple studies point to upregulation of MMPs in the NAc during cue-induced heroin relapse (Liu et al., 2010; Smith et al., 2014; Chioma et al., 2021). Interestingly, inhibiting PNN degradation during reinstatement with intracerebroventricular FN-439 administration or enhancing PNN degradation during extinction with chABC in the amygdala impairs cue-induced heroin reinstatement (Van den Oever et al., 2010; Xue et al., 2014).
Two recent human postmortem studies provide critical evidence regarding alterations of ECM and neuroimmune gene expression pathways in the brain of people with OUD (Seney et al., 2021; Wei et al., 2023). Transcriptional profiling of the dorsolateral PFC and NAc revealed a shared, significant upregulation of transcripts related to CSPG and GAG biosynthesis in these two brain regions in subjects with OUD (Seney et al., 2021). Notably, pathways involved in cytokine-mediated inflammation and synapse remodeling were also upregulated (Seney et al., 2021). Moreover, a recent postmortem study of the ventral midbrain transcriptome identified significant upregulation of the IL-4 receptor in microglia in individuals who died by opioid overdose, providing further support for neuroinflammation in the brain of people with OUD (Wei et al., 2023). Collectively, studies on the effects of opioids on brain ECM molecules highlight an interplay of the brain’s immune system and the ECM in regulating both reward stabilization and analgesia.
Alcohol
Alcohol use disorder (AUD) is the most prevalent form of SUD in the U.S. (American Psychiatric Association, 2013). Chronic, heavy alcohol use results in an array of health issues affecting several organ systems including the brain and increases mortality risk. A growing number of studies have investigated ECM alterations in response to alcohol early in adolescence, as well as acute, and chronic alcohol use (Table 4; Lasek, 2016).
Acute alcohol exposure
One of the first studies to examine brain levels of ECM molecules in response to drugs of abuse focused on alcohol’s effect on MMP-9 in the rat brain during water maze training (Wright et al., 2003). Alcohol administration resulted in reduced MMP-9 activity in the hippocampus and prefrontal cortex. This reduction was evident after 2 days and became progressively more severe after 4 and 6 days of alcohol administration (Wright et al., 2003). The progressive decrease in MMP-9 activity was accompanied by impaired water maze performance, suggesting that alcohol impairs hippocampal learning by downregulating MMP-9 activity (Wright et al., 2003). Furthermore, intracerebroventricular administration of the broad spectrum MMP inhibitor, FN-439, during ethanol withdrawal, was reported to prevent relapse to alcohol self-administration in rats (Smith et al., 2011). Alternatively, a recent study using chronic adenovirus-mediated overexpression of MMP-9 in the hippocampus demonstrated that mice with chronic MMP-9 overexpression had reduced alcohol-induced place preference (Yin et al., 2023). Persistent MMP-9 upregulation also perturbed ethanol-induced increases in NMDA receptor subtypes (Yin et al., 2023). Inhibitory effects of prolonged upregulation of MMP-9 on alcohol place preference points to the importance of temporal specificity of MMP regulation during phases of reward integration. Upregulation of MMPs, when prolonged, may cause aberrant synaptogenesis that inhibits the normal synaptic refinement and GABAergic (i.e., parvalbumin) modulatory processes which aid in reward memory precision and consolidation (Beroun et al., 2019).
While no studies have examined PNNs after acute exposure to alcohol (i.e., <24 h), exposure to alcohol for one week does not significantly alter the fluorescent intensity of PNNs, or mRNA expression of the PNN components aggrecan, brevican, and phosphacan (Chen et al., 2015). Lack of changes in CSPG levels at this timepoint may indicate that ECM remodeling occurs earlier than 2 days, and at 7 days ECM remodeling remains in an interim phase where neither MMPs nor PNNs/their components are significantly altered.
Chronic alcohol use
Several studies have investigated how chronic alcohol exposure regulates the structure of the ECM. Adolescent exposure to alcohol in mice results in increased densities of PNNs and increased immunoreactivity of brevican and neurocan in the orbitofrontal cortex (OFC) that is sustained into adulthood (Coleman et al., 2014; Dannenhoffer et al., 2022). In adult rats, 6 weeks of alcohol self-administration significantly increases the intensity of WFA-labeled PNNs and mRNA levels of aggrecan, brevican, and phosphacan in the insula (Chen et al., 2015). A set of experiments from the same group used cocktails of quinine, an aversive and bitter additive, with alcohol to investigate whether the ECM regulates aversion-resistant drinking (Chen and Lasek, 2020). Ablating PNNs in the insula with chABC 3 days prior to the onset of alcohol self-administration rendered animals more sensitive to aversive quinine cocktails (Chen and Lasek, 2020). As the insula is part of the gustatory cortex, increased PNNs in this area may contribute to integration of the non-pharmacologically relevant aspects of alcohol into the memory trace (i.e., bitter taste). In comparison, the ECM neural cell adhesion molecules (NrCAMs) may be involved in the contextual aspects of alcohol reward circuits. NrCAM knockout mice display reduced alcohol place preference (Ishiguro et al., 2014), and pharmacological inhibition of this signaling pathway via systemic administration of prolyl-leucyl-glycinamide also impaired alcohol place preference, suggesting a potential ECM-based therapeutic approach for alleviating context-induced relapse. Chronic alcohol consumption is also associated with increased neuroinflammation, such as heightened recruitment of several pro-inflammatory cytokines that are known to impact the ECM (Kelley and Dantzer, 2011; Lowe et al., 2020; Xiong et al., 2022). Genetic studies of AUD identified associations of genes encoding ECM molecules with increased risk of AUD. Two genome-wide association studies implicate genetic polymorphisms of several ECM genes including tenascin-N and -R and MMP-9 with AUD (Samochowiec et al., 2010; Zuo et al., 2012), suggesting that ECM molecules may be involved in the susceptibility for developing AUD.
Psychoplastogens
Psychoplastogens encompass a variety of chemical classes (e.g., dissociatives, psychedelics) which produce profound, long-lasting effects on neural plasticity with single exposures (Ly et al., 2018; Ballentine et al., 2022). This feature of psychoplastogens has made them attractive candidates as potential treatments for SUD and MDD in controlled, clinical settings in combination with psychotherapy, such as cognitive behavioral therapy (CBT) (Carhart-Harris et al., 2012; Talin and Sanabria, 2017; Rieser et al., 2022; Sloshower et al., 2023).
Ketamine, an NMDA receptor antagonist originally used as an anesthetic, has psychotomimetic effects including hallucinations (Powers et al., 2015). It is also used recreationally and can lead to SUD (Strous et al., 2022). Chronic recreational use reportedly results in structural gray and white matter alterations and memory impairment (Strous et al., 2022). Several studies, including clinical trials, provide support for the use of low dose ketamine administration for treatment-resistant depression (Zarate et al., 2006; Phillips et al., 2019; Can et al., 2021), which is often comorbid with SUD (Shin and Kim, 2020). Repeated exposure to ketamine at anesthetic doses results in decreased composition of PNNs via microglia-mediated proteolytic ECM degradation which promotes synapse plasticity (Venturino and Siegert, 2021; Venturino et al., 2021). Further, ketamine and phencyclidine (PCP) administration alter expression of a number of ECM genes, including the collagen type IX alpha 2 chain, NrCAM-1, decorin, and heparan sulfate 6-O-sulfotransferase, in the striatum between one and 8 h following administration (Oommen et al., 2023), suggesting that effects on the ECM are much broader than reported PNN composition changes.
The conventional serotonergic psychedelics, lysergic acid diethylamide (LSD), N,N-dimethyltryptamine (DMT), and psilocybin, are suggested to have potentially rapid antidepressant effects with a relatively limited side effect profile (Carhart-Harris et al., 2016; Rucker et al., 2016). In the rat PFC, both in vivo and in vitro, LSD, DMT, and the substituted amphetamine psychedelic 2,5-dimethoxy-4-iodoamphetamine (DOI) promote neuritogenesis, spinogenesis and synaptogenesis (Ly et al., 2018). Furthermore, the synaptic changes induced by psychedelics may be long term. For example, the reported increase in dendritic remodeling detected within 24 h was reported to last for at least 1 month following a single dose of psilocybin (Shao et al., 2021). To date, there are no studies of how these compounds regulate the ECM. Acute administration of DOI produces robust transcriptional responses by recruiting multiple cell types including glia, and significantly increases expression of parvalbumin and somatostatin in activated neuronal ensembles (Martin and Nichols, 2016). In line with this, both psilocybin and ketamine upregulate parvalbumin expression in neurons expressing the immediate early gene cFos (Davoudian et al., 2023). Upregulated somatostatin, a neurotransmitter expressed by a subset of interneurons ensheathed by PNNs (Willis et al., 2022), may potentially alleviate molecular alterations in the case of SUD, as our recent study identified decreased hippocampal somatostatin expression in subjects with SUD (Valeri et al., 2022). Taken together, we speculate that psychedelic drugs may have similar actions on the ECM as psychostimulant drugs, mediating rapid and sustained ECM disassembly upon administration. However, serotonergic psychedelics are not reinforcing, and therefore likely do not carry risk of addiction (Nichols, 2016). Several studies suggest that psychedelics combined with CBT may be promising candidates for improving SUD outcomes and preventing relapse, as they may promote plasticity of brain circuits involved in reward and goal-directed behavior (DiVito and Leger, 2020), potentially in part through ECM remodeling.
Obesity and metabolic disorders
Obesity is a global health problem that impacts virtually all aspects of health and results in a significant personal and societal burden (Main et al., 2010). Obesity also increases the risk of a range of diseases, including metabolic disorders, which encompasses cardiovascular disease, diabetes mellitus, and hypertension (Colditz et al., 1995; Calle et al., 2003; GBD 2015 Obesity Collaborators et al., 2017). Several psychiatric disorders associated with increased risk of SUD share comorbidity with metabolic disorders (Luppino et al., 2010; Jimenez et al., 2019; Trevino-Alvarez et al., 2023). For example, MDD is often comorbid with type 2 diabetes (T2D), and several lines of evidence point to a core metabolic pathology across mood disorders (Fagiolini et al., 2002; Dona et al., 2020; Norwitz et al., 2020). Additionally, conditions associated with metabolic disorders such as painful diabetic neuropathy are commonly managed with opioid medications, which pose a risk of addiction (Callaghan et al., 2012; Jensen et al., 2021). In addition, many people with T2D (over 40%) also have a comorbid SUD (Wu et al., 2018; Feldman et al., 2019). The brain regions primarily responsible for food-seeking behaviors include the hypothalamus (e.g., arcuate nucleus, median eminence, lateral hypothalamus), hippocampus (Martin and Davidson, 2014; Parent et al., 2014), and PFC (Garcia-Garcia et al., 2014). Obesogenic diets have been reported to result in inflammation and alterations in synaptic plasticity and blood-brain barrier regulation, all of which are regulated in part by the ECM (Gustafson et al., 2007; Guillemot-Legris and Muccioli, 2017; Matikainen-Ankney and Kravitz, 2018; Brown and Sorg, 2023).
Several studies support a key role of PNNs in the arcuate nucleus in obesity and metabolic disorders (Table 5). Formation of PNNs in the arcuate nucleus coincides with the closure of the critical period of agouti-related peptide neuron maturation in this region, in a leptin-dependent manner (Mirzadeh et al., 2019). Furthermore, Zucker diabetic fatty rats, which carry spontaneous missense mutations of the leptin receptor gene causing hyperglycemia and rapid development of T2D, have significantly reduced PNNs and altered sulfation of CSPGs in the arcuate nucleus (Alonge et al., 2020). These effects on PNNs are reversed by intracerebroventricular injections of fibroblast growth factor 1, which induces sustained diabetes remission in Zucker rats (Scarlett et al., 2016; Alonge et al., 2020).
Several studies also report ECM alterations in several brain areas outside of the hypothalamus in preclinical models of obesity (Table 5). For example, chronic (3 weeks) exposure to a high-diet in adult male rats decreased the fluorescent intensity of PNNs labeled with WFA in the prelimbic and orbitofrontal cortices, an effect that was not contingent on weight gain (Dingess et al., 2018). In a report from the same group, Sprague-Dawley rats which were bred either prone to obesity or resistant to obesity had differential responses a high-fat diet in the OFC, with decreases in PNN intensity in obese-prone rats and increased PNN intensity in obese-resistant rats (Dingess et al., 2020). Chronic exposure (5 weeks) to a high-fat/high-sugar diet decreased PNN density in the CA1 field of the hippocampus of adult male mice, along with corresponding increases in adiposity and abundance of activated microglia (Reichelt et al., 2021). However, no changes were observed in PNNs in the PFC in the same animals (Reichelt et al., 2021). Although high-fat/high-sugar diet formulations induce changes in PNNs in the PFC, sugar alone does not appear to have any effect on PNNs (Slaker et al., 2016b; Roura-Martinez et al., 2020). Data regarding short term effects of feeding and specific diets on the ECM is greatly limited. A recent study suggests that PNN composition in the median eminence of the hypothalamus is decreased during fasting but PNNs increase after a brief feeding following the fasting period (Kohnke et al., 2021).
A relatively limited set of studies have investigated sexual dimorphic effects of diet on the ECM. In contrast to the data in males, both outbred and obese-prone female rats exhibit an increase in PNN intensity in the infralimbic cortex following a high fat diet, whereas obese-resistant females show a decrease in PNN intensity (Dingess et al., 2020). In contrast, in the arcuate nucleus, sex hormones have been shown to affect the ECM independent of dietary manipulation (Zhang et al., 2021).
Obesity as well as T2D contribute to neuroinflammation (Miller and Spencer, 2014; Van Dyken and Lacoste, 2018). Brain-wide decreases of PNNs in male subjects exposed to a chronic high-fat diet is in line with heightened inflammatory activity from microglia and potential proteolysis of ECM. There is likely deep complexity between multiple factors that correspond with diet-induced differences in the architecture of the ECM, such as genetic predisposition, age, and sex. Future studies examining these relationships and how they interact in comorbid conditions will provide key insight into the involvement of the ECM in metabolic disorders and how this information can be leveraged for therapeutic strategies. As mentioned previously, metabolic dysfunction is a characteristic of some psychiatric disorders, such as MDD and BD. The ketogenic (low carb) diet is rapidly gaining support as a treatment for psychiatric disorders including mood disorders, autism spectrum disorder, and schizophrenia (Brietzke et al., 2018; Campbell and Campbell, 2020; Sarnyai and Palmer, 2020; Danan et al., 2022). Intriguingly, the ketogenic diet has been shown to alter the ECM by downregulating gene expression of fibrinogen, but upregulating myelin basic protein expression in the rat hippocampus (Noh et al., 2004).
Current challenges and future directions
Despite the growing evidence for a key role of ECM molecules in substance use disorders, there are several knowledge gaps that limit the development of ECM-based pharmacotherapies. First, as indicated in this review, despite the growing number of studies, there is a lack of sufficient information regarding the temporal ECM changes in specific brain regions for specific substances. In addition, despite evidence for WFA labeled PNN alterations, there is a lack of information regarding changes in PNN composition and specific ECM structures such as perisynaptic ECM, axonal coats and/or perivascular ECM. Moreover, few studies thus far have examined the role of the ECM in neurodevelopmental factors prior to the onset of SUD that can contribute to risk of SUD, including genetic factors, chronic stress, exposure to a culture of drug use, traumatic experiences, and socio-economic status. Future studies on the ECM that consider such etiological factors would provide critical insight into the role of the ECM in enhanced risk to SUDs.
Furthermore, the vast majority of current evidence is largely based on animal model studies, there is currently a lack of information regarding these ECM structures in the brain of individuals with SUD. Human postmortem studies may provide critical information that can support preclinical studies and guide development of therapeutic strategies. Polysubstance use disorder is the most common clinical presentation of SUD, thus preclinical studies of polysubstance use may provide key insight into potential interactions of various drugs of abuse on the ECM and allow for development of more translatable therapeutic strategies. In terms of developing or repurposing drugs for ECM based treatments of SUD, the current evidence suggests that MMP inhibitors such as doxycycline may inhibit ECM degradation and formation of reward memories associated with initial drug exposure. In comparison, short-term upregulation of MMPs may represent an effective strategy to treat chronic SUD, especially in combination with CBT. Temporary removal of PNNs may allow for reorganization or weakening of reward associated synapses, and may prevent feedforward modulation of neuronal ensembles by parvalbumin, thus creating more glutamatergic noise and less coherence of the neurons that are associated with drug memories (Brown and Sorg, 2023). Furthermore, several lines of evidence indicate that inhibiting MMP activity may be useful in preventing relapse following a period of abstinence. The development of pharmacotherapies with selectivity for brain ECM structures which can be delivered systemically, provide temporal control, and limit potential off-target side effects remains a major challenge for ECM-based therapeutic strategies.
Regarding the role of the ECM in metabolic disorders, a limitation of rodent models of T2D (e.g., the Zucker rat) is the relatively abrupt development of hyperglycemia, which is not characteristic of the gradual human disease progression (Oltman et al., 2005; Hinder et al., 2018). As rodent models of T2D evolve, examining the role of the ECM in the progression from prediabetic-like states to T2D may provide evidence for temporal ECM alterations that can guide preventative and treatment strategies. As previously mentioned, many individuals with mood disorders often suffer from metabolic disorders and eating disorders, such as binge-eating disorder. In this context, there is currently a lack of evidence providing a link between mood dysregulation, HFD, and the ECM. Studies focused on these associations may enhance our understanding of potential ECM-based precipitating factors for metabolic disorders.
Concluding remarks
Current evidence suggests that the ECM is at the intersection of synaptic regulation and neuroimmune responses to drug reward and food reward. Synaptic regulation and inflammation are hallmarks of the neuroadaptations induced by both SUD and metabolic disorders (Cui et al., 2014; Hao et al., 2016). These processes may impact overlapping neuroanatomical circuits and cellular mechanisms. Food is essential for survival, and evolutionarily conserved processes may promote strengthening of brain circuits involved in integrating environmental cues associated with biologically relevant rewards such as food, especially food that is high in fat and calories. In comparison, drugs of abuse are not necessary for survival, and initial exposure to drugs most commonly occurs after the closure of critical periods of plasticity associated with maturation of PNNs. Therefore, enhanced PNNs by chronic drug (unnatural) reward may be the result of synaptic reorganization occurring later in development, recruiting additional ECM components to stabilize synapses that participate in integration of novel, potent reward experiences engaging brain circuits that are normally involved in regulating memories for experiences that are necessary for survival. Intriguingly, a similar temporal relationship of PNN alterations was reported in the rat hippocampus across stages of social defeat stress (Riga et al., 2017), suggesting that this temporal regulation of PNNs may be a shared mechanism underlying memory processing across positive and negative experiences. Decreased PNNs in obesity and metabolic disorders may instead reflect increased neuroinflammation associated with these conditions.
In summary, ECM molecules may represent key contributors to the pathogenesis of SUD and metabolic disorders. Acute exposure to substances of abuse render PNNs unstable through the activity of endogenous proteases, and chronic exposure generally increases PNNs and ECM molecules (Figure 3). Pathologically, SUD is an ingrained memory trace that maladaptively triggers activation of brain circuits that recurrently promote relapse. Thus, enhanced PNNs may reflect an inflexibility of memory systems pertaining to reward-associated stimuli which perpetuates the cycle of relapse and addiction.
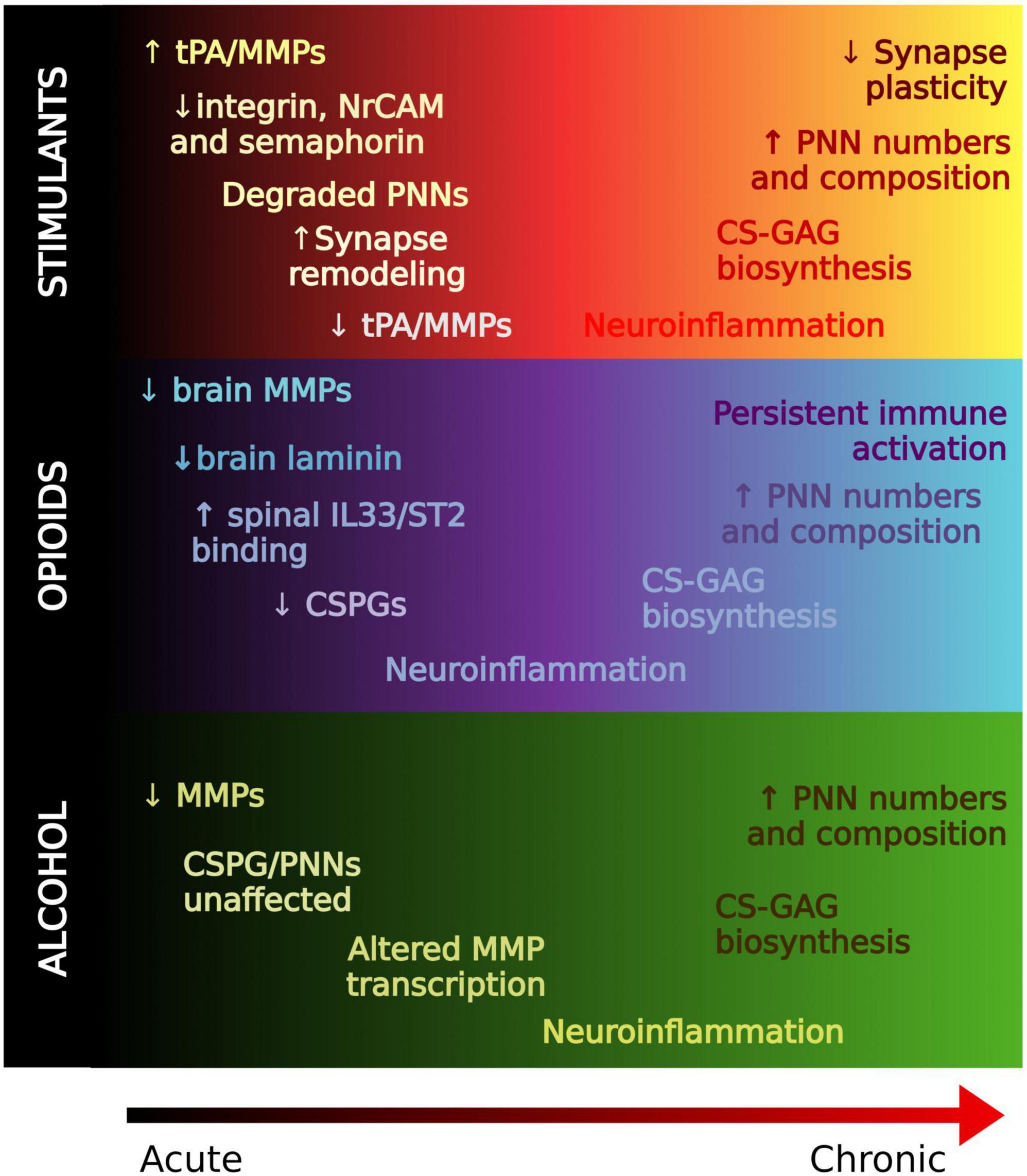
Figure 3. Regulation of the extracellular matrix in drug memory processing. A working hypothesis of ECM alterations from acute to chronic stages of substance use. In general, ECM molecules are degraded in acute stages, possibly to allow for formation of new synapses. PNNs and ECM molecules are generally increased with chronic use which may contribute to the strength of reward memories associated with SUD that confer relapse.
Author contributions
All authors listed have made a substantial, direct, and intellectual contribution to the work, and approved it for publication.
Funding
This work was funded by the National Institute of Mental Health (MH125833 and MH117460) and the National Institute on Alcohol Abuse and Alcoholism F31AA030166.
Conflict of interest
The authors declare that the research was conducted in the absence of any commercial or financial relationships that could be construed as a potential conflict of interest.
Publisher’s note
All claims expressed in this article are solely those of the authors and do not necessarily represent those of their affiliated organizations, or those of the publisher, the editors and the reviewers. Any product that may be evaluated in this article, or claim that may be made by its manufacturer, is not guaranteed or endorsed by the publisher.
References
Adams, I., Brauer, K., Arelin, C., Hartig, W., Fine, A., Mader, M., et al. (2001). Perineuronal nets in the rhesus monkey and human basal forebrain including basal ganglia. Neuroscience 108, 285–298. doi: 10.1016/s0306-4522(01)00419-5
Ajmo, J., Eakin, A., Hamel, M., and Gottschall, P. (2008). Discordant localization of WFA reactivity and brevican/ADAMTS-derived fragment in rodent brain. BMC Neurosci. 9:14. doi: 10.1186/1471-2202-9-14
Alaiyed, S., McCann, M., Mahajan, G., Rajkowska, G., Stockmeier, C., Kellar, K., et al. (2020). Venlafaxine stimulates an MMP-9-dependent increase in excitatory/inhibitory balance in a stress model of depression. J. Neurosci. 40, 4418–4431. doi: 10.1523/JNEUROSCI.2387-19.2020
Alonge, K., Logsdon, A., Murphree, T., Banks, W., Keene, C., Edgar, J., et al. (2019). Quantitative analysis of chondroitin sulfate disaccharides from human and rodent fixed brain tissue by electrospray ionization-tandem mass spectrometry. Glycobiology 29, 847–860. doi: 10.1093/glycob/cwz060
Alonge, K., Mirzadeh, Z., Scarlett, J., Logsdon, A., Brown, J., Cabrales, E., et al. (2020). Hypothalamic perineuronal net assembly is required for sustained diabetes remission induced by fibroblast growth factor 1 in rats. Nat. Metab. 2, 1025–1033. doi: 10.1038/s42255-020-00275-6
Alsio, J., Olszewski, P., Levine, A., and Schioth, H. (2012). Feed-forward mechanisms: addiction-like behavioral and molecular adaptations in overeating. Front. Neuroendocrinol. 33:127–139. doi: 10.1016/j.yfrne.2012.01.002
American Psychiatric Association (2013). Diagnostic and statistical manual of mental disorders, (DSM-5), Fifth Edn. Virginia: American Psychiatric Publishing, Inc.
Asher, R., Morgenstern, D., Moon, L., and Fawcett, J. (2001). Chondroitin sulphate proteoglycans: inhibitory components of the glial scar. Prog. Brain Res. 132, 611–619. doi: 10.1016/S0079-6123(01)32106-4
Baeten, K., and Akassoglou, K. (2011). Extracellular matrix and matrix receptors in blood-brain barrier formation and stroke. Dev. Neurobiol. 71, 1018–1039.
Bahi, A., and Dreyer, J. (2005). Cocaine-induced expression changes of axon guidance molecules in the adult rat brain. Mol. Cell Neurosci. 28, 275–291. doi: 10.1016/j.mcn.2004.09.011
Bahi, A., Kusnecov, A., and Dreyer, J. (2008). Effects of urokinase-type plasminogen activator in the acquisition, expression and reinstatement of cocaine-induced conditioned-place preference. Behav. Brain Res. 191, 17–25. doi: 10.1016/j.bbr.2008.03.004
Baik, J. (2013). Dopamine signaling in food addiction: role of dopamine D2 receptors. BMB Rep. 46, 519–526. doi: 10.5483/bmbrep.2013.46.11.207
Ballentine, G., Friedman, S., and Bzdok, D. (2022). Trips and neurotransmitters: discovering principled patterns across 6850 hallucinogenic experiences. Sci. Adv. 8:eabl6989. doi: 10.1126/sciadv.abl6989
Barry, D., Clarke, M., and Petry, N. (2009). Obesity and its relationship to addictions: is overeating a form of addictive behavior? Am. J. Addict. 18, 439–451. doi: 10.3109/10550490903205579
Bekku, Y., Rauch, U., Ninomiya, Y., and Oohashi, T. (2009). Brevican distinctively assembles extracellular components at the large diameter nodes of Ranvier in the CNS. J. Neurochem. 108, 1266–1276. doi: 10.1111/j.1471-4159.2009.05873.x
Beroun, A., Mitra, S., Michaluk, P., Pijet, B., Stefaniuk, M., and Kaczmarek, L. (2019). MMPs in learning and memory and neuropsychiatric disorders. Cell Mol. Life Sci. 76, 3207–3228. doi: 10.1007/s00018-019-03180-8
Blacktop, J., Todd, R., and Sorg, B. (2017). Role of perineuronal nets in the anterior dorsal lateral hypothalamic area in the acquisition of cocaine-induced conditioned place preference and self-administration. Neuropharmacology 118, 124–136. doi: 10.1016/j.neuropharm.2017.03.018
Blanco, I., and Conant, K. (2021). Extracellular matrix remodeling with stress and depression: studies in human, rodent and zebrafish models. Eur. J. Neurosci. 53, 3879–3888. doi: 10.1111/ejn.14910
Blum, K., Werner, T., Carnes, S., Carnes, P., Bowirrat, A., Giordano, J., et al. (2012). Sex, drugs, and rock ‘n’ roll: hypothesizing common mesolimbic activation as a function of reward gene polymorphisms. J. Psychoactive Drugs. 44, 38–55. doi: 10.1080/02791072.2012.662112
Brew, K., Dinakarpandian, D., and Nagase, H. (2000). Tissue inhibitors of metalloproteinases: evolution, structure and function. Biochim. Biophys. Acta 1477, 267–283. doi: 10.1016/s0167-4838(99)00279-4
Brietzke, E., Mansur, R., Subramaniapillai, M., Balanzá-Martínez, V., Vinberg, M., González-Pinto, A., et al. (2018). Ketogenic diet as a metabolic therapy for mood disorders: evidence and developments. Neurosci. Biobehav. Rev. 94, 11–16. doi: 10.1016/j.neubiorev.2018.07.020
Brown, T., and Sorg, B. (2023). Net gain and loss: influence of natural rewards and drugs of abuse on perineuronal nets. Neuropsychopharmacology 48, 3–20. doi: 10.1038/s41386-022-01337-x
Brown, T., Forquer, M., Cocking, D., Jansen, H., Harding, J., and Sorg, B. (2007). Role of matrix metalloproteinases in the acquisition and reconsolidation of cocaine-induced conditioned place preference. Learn. Mem. 14, 214–223. doi: 10.1101/lm.476207
Brown, T., Forquer, M., Harding, J., Wright, J., and Sorg, B. (2008). Increase in matrix metalloproteinase-9 levels in the rat medial prefrontal cortex after cocaine reinstatement of conditioned place preference. Synapse 62, 886–889. doi: 10.1002/syn.20562
Brown, T., Wilson, A., Cocking, D., and Sorg, B. (2009). Inhibition of matrix metalloproteinase activity disrupts reconsolidation but not consolidation of a fear memory. Neurobiol. Learn. Mem. 91, 66–72. doi: 10.1016/j.nlm.2008.09.003
Browne, C., Futamura, R., Minier-Toribio, A., Hicks, E., Ramakrishnan, A., Martínez-Rivera, F., et al. (2023). Transcriptional signatures of heroin intake and seeking throughout the brain reward circuit. bioRxiv [Preprint] doi: 10.1101/2023.01.11.523688
Bruckner, G., Morawski, M., and Arendt, T. (2008). Aggrecan-based extracellular matrix is an integral part of the human basal ganglia circuit. Neuroscience 151, 489–504. doi: 10.1016/j.neuroscience.2007.10.033
Brzdak, P., Nowak, D., Wiera, G., and Mozrzymas, J. (2017). Multifaceted roles of metzincins in CNS physiology and pathology: from synaptic plasticity and cognition to neurodegenerative disorders. Front. Cell. Neurosci. 11:178. doi: 10.3389/fncel.2017.00178
Cabungcal, J., Steullet, P., Morishita, H., Kraftsik, R., Cuenod, M., Hensch, T., et al. (2013). Perineuronal nets protect fast-spiking interneurons against oxidative stress. Proc. Natl. Acad. Sci. U.S.A. 110, 9130–9135. doi: 10.1073/pnas.1300454110
Callaghan, B., Cheng, H., Stables, C., Smith, A., and Feldman, E. (2012). Diabetic neuropathy: clinical manifestations and current treatments. Lancet Neurol. 11, 521–534. doi: 10.1016/S1474-4422(12)70065-0
Calle, E., Rodriguez, C., Walker-Thurmond, K., and Thun, M. (2003). Overweight, obesity, and mortality from cancer in a prospectively studied cohort of U.S. adults. N. Engl. J. Med. 348, 1625–1638. doi: 10.1056/NEJMoa021423
Campbell, I., and Campbell, H. (2020). Mechanisms of insulin resistance, mitochondrial dysfunction and the action of the ketogenic diet in bipolar disorder. Focus on the PI3K/AKT/HIF1-a pathway. Med. Hypotheses 145:110299. doi: 10.1016/j.mehy.2020.110299
Can, A., Hermens, D., Dutton, M., Gallay, C., Jensen, E., Jones, M., et al. (2021). Low dose oral ketamine treatment in chronic suicidality: an open-label pilot study. Transl. Psychiatry 11:101. doi: 10.1038/s41398-021-01230-z
Carhart-Harris, R., Bolstridge, M., Rucker, J., Day, C., Erritzoe, D., Kaelen, M., et al. (2016). Psilocybin with psychological support for treatment-resistant depression: an open-label feasibility study. Lancet Psychiatry 3, 619–627. doi: 10.1016/S2215-0366(16)30065-7
Carhart-Harris, R., Leech, R., Williams, T., Erritzoe, D., Abbasi, N., Bargiotas, T., et al. (2012). Implications for psychedelic-assisted psychotherapy: functional magnetic resonance imaging study with psilocybin. Br. J. Psychiatry 200, 238–244. doi: 10.1192/bjp.bp.111.103309
Carter, B., and Tiffany, S. (1999). Meta-analysis of cue-reactivity in addiction research. Addiction 94, 327–340.
Carulli, D., and Verhaagen, J. (2021). An extracellular perspective on CNS maturation: perineuronal nets and the control of plasticity. Int. J. Mol. Sci. 22:2434. doi: 10.3390/ijms22052434
Chen, H., and Lasek, A. (2020). Perineuronal nets in the insula regulate aversion-resistant alcohol drinking. Addict. Biol. 25:e12821. doi: 10.1111/adb.12821
Chen, H., He, D., and Lasek, A. (2015). Repeated binge drinking increases perineuronal nets in the insular cortex. Alcohol Clin. Exp. Res. 39, 1930–1938. doi: 10.1111/acer.12847
Chen, J., Kawamura, T., Sethi, M., Zaia, J., Repunte-Canonigo, V., and Sanna, P. (2017). Heparan sulfate: resilience factor and therapeutic target for cocaine abuse. Sci. Rep. 7:13931. doi: 10.1038/s41598-017-13960-6
Chen, J., Repunte-Canonigo, V., Kawamura, T., Lefebvre, C., Shin, W., Howell, L., et al. (2013). Hypothalamic proteoglycan syndecan-3 is a novel cocaine addiction resilience factor. Nat. Commun. 4:1955. doi: 10.1038/ncomms2955
Chioma, V., Kruyer, A., Bobadilla, A., Angelis, A., Ellison, Z., Hodebourg, R., et al. (2021). Heroin seeking and extinction from seeking activate matrix metalloproteinases at synapses on distinct subpopulations of accumbens cells. Biol. Psychiatry 89, 947–958. doi: 10.1016/j.biopsych.2020.12.004
Colditz, G., Willett, W., Rotnitzky, A., and Manson, J. (1995). Weight gain as a risk factor for clinical diabetes mellitus in women. Ann. Intern. Med. 122, 481–486. doi: 10.7326/0003-4819-122-7-199504010-00001
Coleman, L. Jr., Liu, W., Oguz, I., Styner, M., and Crews, F. (2014). Adolescent binge ethanol treatment alters adult brain regional volumes, cortical extracellular matrix protein and behavioral flexibility. Pharmacol. Biochem. Behav. 116, 142–151. doi: 10.1016/j.pbb.2013.11.021
Cui, C., Shurtleff, D., and Harris, R. (2014). Neuroimmune mechanisms of alcohol and drug addiction. Int. Rev. Neurobiol. 118, 1–12. doi: 10.1016/b978-0-12-801284-0.00001-4
Danan, A., Westman, E., Saslow, L., and Ede, G. (2022). The ketogenic diet for refractory mental illness: a retrospective analysis of 31 inpatients. Front. Psychiatry. 13:951376. doi: 10.3389/fpsyt.2022.951376
Dankovich, T., and Rizzoli, S. (2022). Extracellular matrix recycling as a novel plasticity mechanism with a potential role in disease. Front. Cell. Neurosci. 16:854897. doi: 10.3389/fncel.2022.854897
Dannenhoffer, C., Gomez, A., Macht, V., Jawad, R., Sutherland, E., Vetreno, R., et al. (2022). Impact of adolescent intermittent ethanol exposure on interneurons and their surrounding perineuronal nets in adulthood. Alcohol Clin. Exp. Res. 46, 759–769. doi: 10.1111/acer.14810
Dauth, S., Grevesse, T., Pantazopoulos, H., Campbell, P., Maoz, B., Berretta, S., et al. (2016). Extracellular matrix protein expression is brain region dependent. J. Comp. Neurol. 524, 1309–1336. doi: 10.1002/cne.23965
Davoudian, P., Shao, L., and Kwan, A. (2023). Shared and distinct brain regions targeted for immediate early gene expression by ketamine and psilocybin. ACS Chem. Neurosci. 14, 468–480. doi: 10.1021/acschemneuro.2c00637
de Guglielmo, G., Iemolo, A., Nur, A., Turner, A., Montilla-Perez, P., Martinez, A., et al. (2022). Reelin deficiency exacerbates cocaine-induced hyperlocomotion by enhancing neuronal activity in the dorsomedial striatum. Genes Brain Behav. 21:e12828. doi: 10.1111/gbb.12828
de Winter, F., Kwok, J., Fawcett, J., Vo, T., Carulli, D., and Verhaagen, J. (2016). The chemorepulsive protein semaphorin 3A and perineuronal net-mediated plasticity. Neural Plasticity 2016:3679545. doi: 10.1155/2016/3679545
Deepa, S., Carulli, D., Galtrey, C., Rhodes, K., Fukuda, J., Mikami, T., et al. (2006). Composition of perineuronal net extracellular matrix in rat brain: a different disaccharide composition for the net-associated proteoglycans. J. Biol. Chem. 281, 17789–17800. doi: 10.1074/jbc.M600544200
Dick, G., Tan, C., Alves, J., Ehlert, E., Miller, G., Hsieh-Wilson, L., et al. (2013). Semaphorin 3A binds to the perineuronal nets via chondroitin sulfate type E motifs in rodent brains. J. Biol. Chem. 288, 27384–27395. doi: 10.1074/jbc.M111.310029
Dingess, P., Harkness, J., Slaker, M., Zhang, Z., Wulff, S., Sorg, B., et al. (2018). Consumption of a high-fat diet alters perineuronal nets in the prefrontal cortex. Neural Plast. 2018:2108373. doi: 10.1155/2018/2108373
Dingess, P., Zhang, Z., Sorg, B., Ferrario, C., and Brown, T. (2020). Sex and region-specific effects of high fat diet on PNNs in obesity susceptible rats. Physiol. Behav. 222:112963. doi: 10.1016/j.physbeh.2020.112963
Dityatev, A., Schachner, M., and Sonderegger, P. (2010). The dual role of the extracellular matrix in synaptic plasticity and homeostasis. Nat. Rev. Neurosci. 11, 735–746. doi: 10.1038/nrn2898
DiVito, A., and Leger, R. (2020). Psychedelics as an emerging novel intervention in the treatment of substance use disorder: a review. Mol. Biol. Rep. 47, 9791–9799. doi: 10.1007/s11033-020-06009-x
Dona, A., DeLouize, A., Eick, G., Thiele, E., Salinas Rodriguez, A., Manrique Espinoza, B., et al. (2020). Inflammation and central adiposity as mediators of depression and uncontrolled diabetes in the study on global AGEing and adult health (SAGE). Am. J. Hum. Biol. 32:e23413. doi: 10.1002/ajhb.23413
Dours-Zimmermann, M., Maurer, K., Rauch, U., Stoffel, W., Fassler, R., and Zimmermann, D. (2009). Versican V2 assembles the extracellular matrix surrounding the nodes of ranvier in the CNS. J. Neurosci. 29, 7731–7742. doi: 10.1523/JNEUROSCI.4158-08.2009
Eastwood, S., and Harrison, P. (2006). Cellular basis of reduced cortical reelin expression in schizophrenia. Am. J. Psychiatry 163, 540–542. doi: 10.1176/appi.ajp.163.3.540
Enwright, J., Sanapala, S., Foglio, A., Berry, R., Fish, K., and Lewis, D. (2016). Reduced labeling of parvalbumin neurons and perineuronal nets in the dorsolateral prefrontal cortex of subjects with schizophrenia. Neuropsychopharmacology 41, 2206–2214. doi: 10.1038/npp.2016.24
Fagiolini, A., Frank, E., Houck, P., Mallinger, A., Swartz, H., Buysse, D., et al. (2002). Prevalence of obesity and weight change during treatment in patients with bipolar I disorder. J. Clin. Psychiatry 63, 528–533. doi: 10.4088/jcp.v63n0611
Fatemi, S., Earle, J., and McMenomy, T. (2000). Reduction in Reelin immunoreactivity in hippocampus of subjects with schizophrenia, bipolar disorder and major depression. Mol. Psychiatry 5, 654–663. doi: 10.1038/sj.mp.4000783
Fawcett, J., and Kwok, J. (2022). Proteoglycan sulphation in the function of the mature central nervous system. Front. Integr. Neurosci. 16:895493. doi: 10.3389/fnint.2022.895493
Feldman, E., Callaghan, B., Pop-Busui, R., Zochodne, D., Wright, D., Bennett, D., et al. (2019). Diabetic neuropathy. Nat. Rev. Dis. Primers 5:41. doi: 10.1038/s41572-019-0092-1
ffrench-Constant, C., and Colognato, H. (2004). Integrins: versatile integrators of extracellular signals. Trends Cell Biol. 14, 678–686. doi: 10.1016/j.tcb.2004.10.005
Frischknecht, R., and Gundelfinger, E. (2012). The brain’s extracellular matrix and its role in synaptic plasticity. Adv. Exp. Med. Biol. 970, 153–171. doi: 10.1007/978-3-7091-0932-8_7
Frischknecht, R., Heine, M., Perrais, D., Seidenbecher, C., Choquet, D., and Gundelfinger, E. D. (2009). Brain extracellular matrix affects AMPA receptor lateral mobility and short-term synaptic plasticity. Nat. Neurosci. 12, 897–904. doi: 10.1038/nn.2338
Gach, K., Szemraj, J., Wyrebska, A., and Janecka, A. (2011). The influence of opioids on matrix metalloproteinase-2 and -9 secretion and mRNA levels in MCF-7 breast cancer cell line. Mol. Biol. Rep. 38, 1231–1236. doi: 10.1007/s11033-010-0222-z
Ganguly, K., Rejmak, E., Mikosz, M., Nikolaev, E., Knapska, E., and Kaczmarek, L. (2013). Matrix metalloproteinase (MMP) 9 transcription in mouse brain induced by fear learning. J. Biol. Chem. 288, 20978–20991. doi: 10.1074/jbc.M113.457903
Garcia-Garcia, I., Horstmann, A., Jurado, M., Garolera, M., Chaudhry, S., Margulies, D., et al. (2014). Reward processing in obesity, substance addiction and non-substance addiction. Obes. Rev. 15, 853–869. doi: 10.1111/obr.12221
Garcia-Keller, C., Neuhofer, D., Bobadilla, A., Spencer, S., Chioma, V., Monforton, C., et al. (2019). Extracellular matrix signaling through beta3 integrin mediates cocaine cue-induced transient synaptic plasticity and relapse. Biol. Psychiatry. 86, 377–387. doi: 10.1016/j.biopsych.2019.03.982
Garcia-Keller, C., Scofield, M., Neuhofer, D., Varanasi, S., Reeves, M., Hughes, B., et al. (2020). Relapse-associated transient synaptic potentiation requires integrin-mediated activation of focal adhesion kinase and cofilin in D1-expressing neurons. J. Neurosci. 40, 8463–8477. doi: 10.1523/JNEUROSCI.2666-19.2020
GBD 2015 Obesity Collaborators, Afshin, A., Forouzanfar, M., Reitsma, M., Sur, P., Estep, K., et al. (2017). Health effects of overweight and obesity in 195 countries over 25 years. N. Engl. J. Med. 377, 13–27. doi: 10.1056/NEJMoa1614362
Giamanco, K., and Matthews, R. (2012). Deconstructing the perineuronal net: cellular contributions and molecular composition of the neuronal extracellular matrix. Neuroscience 218, 367–384. doi: 10.1016/j.neuroscience.2012.05.055
Gisabella, B., Babu, J., Valeri, J., Rexrode, L., and Pantazopoulos, H. (2021). Sleep and memory consolidation dysfunction in psychiatric disorders: evidence for the involvement of extracellular matrix molecules. Front. Neurosci. 15:646678. doi: 10.3389/fnins.2021.646678
Gogolla, N., Caroni, P., Luthi, A., and Herry, C. (2009). Perineuronal nets protect fear memories from erasure. Science 325, 1258–1261. doi: 10.1126/science.1174146
Goldstein, R., and Volkow, N. (2011). Dysfunction of the prefrontal cortex in addiction: neuroimaging findings and clinical implications. Nat. Rev. Neurosci. 12, 652–669. doi: 10.1038/nrn3119
Grant, B., Saha, T., Ruan, W., Goldstein, R., Chou, S., Jung, J., et al. (2016). Epidemiology of DSM-5 drug use disorder: results from the national epidemiologic survey on alcohol and related conditions-III. JAMA Psychiatry 73, 39–47. doi: 10.1001/jamapsychiatry.2015.2132
Guarque-Chabrera, J., Sanchez-Hernandez, A., Ibanez-Marin, P., Melchor-Eixea, I., and Miquel, M. (2022). Role of perineuronal nets in the cerebellar cortex in cocaine-induced conditioned preference, extinction, and reinstatement. Neuropharmacology 218:109210. doi: 10.1016/j.neuropharm.2022.109210
Guillemot-Legris, O., and Muccioli, G. (2017). Obesity-induced neuroinflammation: beyond the hypothalamus. Trends Neurosci. 40, 237–253. doi: 10.1016/j.tins.2017.02.005
Gundelfinger, E., Frischknecht, R., Choquet, D., and Heine, M. (2010). Converting juvenile into adult plasticity: a role for the brain’s extracellular matrix. Eur. J. Neurosci. 31, 2156–2165. doi: 10.1111/j.1460-9568.2010.07253.x
Gustafson, D., Karlsson, C., Skoog, I., Rosengren, L., Lissner, L., and Blennow, K. (2007). Mid-life adiposity factors relate to blood-brain barrier integrity in late life. J. Intern. Med. 262, 643–650. doi: 10.1111/j.1365-2796.2007.01869.x
Hao, S., Dey, A., Yu, X., and Stranahan, A. (2016). Dietary obesity reversibly induces synaptic stripping by microglia and impairs hippocampal plasticity. Brain Behav. Immun. 51, 230–239. doi: 10.1016/j.bbi.2015.08.023
Harkness, J., Bushana, P., Todd, R., Clegern, W., Sorg, B., and Wisor, J. (2019). Sleep disruption elevates oxidative stress in parvalbumin-positive cells of the rat cerebral cortex. Sleep 42:zsy201. doi: 10.1093/sleep/zsy201
Hartig, W., Brauer, K., Bigl, V., and Bruckner, G. (1994). Chondroitin sulfate proteoglycan-immunoreactivity of lectin-labeled perineuronal nets around parvalbumin-containing neurons. Brain Res. 635, 307–311. doi: 10.1016/0006-8993(94)91452-4
Hartig, W., Derouiche, A., Welt, K., Brauer, K., Grosche, J., Mader, M., et al. (1999). Cortical neurons immunoreactive for the potassium channel Kv3.1b subunit are predominantly surrounded by perineuronal nets presumed as a buffering system for cations. Brain Res. 842, 15–29. doi: 10.1016/s0006-8993(99)01784-9
Hartig, W., Meinicke, A., Michalski, D., Schob, S., and Jager, C. (2022). Update on perineuronal net staining with wisteria floribunda agglutinin (WFA). Front. Integr. Neurosci. 16:851988. doi: 10.3389/fnint.2022.851988
Hinder, L., Murdock, B., Park, M., Bender, D., O’Brien, P., Rumora, A., et al. (2018). Transcriptional networks of progressive diabetic peripheral neuropathy in the db/db mouse model of type 2 diabetes: an inflammatory story. Exp. Neurol. 305, 33–43. doi: 10.1016/j.expneurol.2018.03.011
Hu, X., Yang, W., Zhang, M., Du, L., Tian, J., Zhu, J., et al. (2021). Glial IL-33 signaling through an ST2-to-CXCL12 pathway in the spinal cord contributes to morphine-induced hyperalgesia and tolerance. Sci. Signal. 14:eabe3773. doi: 10.1126/scisignal.abe3773
Hume, C., Massey, S., and van den Buuse, M. (2020). The effect of chronic methamphetamine treatment on schizophrenia endophenotypes in heterozygous reelin mice: implications for schizophrenia. Biomolecules 10:940. doi: 10.3390/biom10060940
Ishiguro, H., Hall, F., Horiuchi, Y., Sakurai, T., Hishimoto, A., Grumet, M., et al. (2014). NrCAM-regulating neural systems and addiction-related behaviors. Addict. Biol. 19, 343–353. doi: 10.1111/j.1369-1600.2012.00469.x
Ishii, M., and Maeda, N. (2008). Oversulfated chondroitin sulfate plays critical roles in the neuronal migration in the cerebral cortex. J. Biol. Chem. 283, 32610–32620. doi: 10.1074/jbc.M806331200
Janak, P., and Chaudhri, N. (2010). The potent effect of environmental context on relapse to alcohol-seeking after extinction. Open Addict. J. 3, 76–87. doi: 10.2174/1874941001003010076
Jensen, T., Karlsson, P., Gylfadottir, S., Andersen, S., Bennett, D., Tankisi, H., et al. (2021). Painful and non-painful diabetic neuropathy, diagnostic challenges and implications for future management. Brain. 144, 1632–1645. doi: 10.1093/brain/awab079
Jimenez, D., Thomas, L., and Bartels, S. (2019). The role of serious mental illness in motivation, participation and adoption of health behavior change among obese/sedentary Latino adults. Ethn. Health 24, 889–896. doi: 10.1080/13557858.2017.1390552
Jones, C. (2013). Heroin use and heroin use risk behaviors among nonmedical users of prescription opioid pain relievers - United States, 2002-2004 and 2008-2010. Drug Alcohol Depend. 132, 95–100. doi: 10.1016/j.drugalcdep.2013.01.007
Kalb, R., and Hockfield, S. (1988). Molecular evidence for early activity-dependent development of hamster motor neurons. J. Neurosci. 8, 2350–2360. doi: 10.1523/JNEUROSCI.08-07-02350.1988
Karus, M., Samtleben, S., Busse, C., Tsai, T., Dietzel, I., Faissner, A., et al. (2012). Normal sulfation levels regulate spinal cord neural precursor cell proliferation and differentiation. Neural Dev. 7:20. doi: 10.1186/1749-8104-7-20
Kelley, K., and Dantzer, R. (2011). Alcoholism and inflammation: neuroimmunology of behavioral and mood disorders. Brain Behav. Immun. 25 Suppl. 1, S13–S20. doi: 10.1016/j.bbi.2010.12.013
Kohnke, S., Buller, S., Nuzzaci, D., Ridley, K., Lam, B., Pivonkova, H., et al. (2021). Nutritional regulation of oligodendrocyte differentiation regulates perineuronal net remodeling in the median eminence. Cell Rep. 36:109362. doi: 10.1016/j.celrep.2021.109362
Kohno, M., Link, J., Dennis, L., McCready, H., Huckans, M., Hoffman, W., et al. (2019). Neuroinflammation in addiction: a review of neuroimaging studies and potential immunotherapies. Pharmacol. Biochem. Behav. 179, 34–42. doi: 10.1016/j.pbb.2019.01.007
Kruyer, A., Chioma, V., and Kalivas, P. (2020). The opioid-addicted tetrapartite synapse. Biol. Psychiatry 87, 34–43. doi: 10.1016/j.biopsych.2019.05.025
Kutlu, M., and Gould, T. (2016). Effects of drugs of abuse on hippocampal plasticity and hippocampus-dependent learning and memory: contributions to development and maintenance of addiction. Learn. Mem. 23, 515–533. doi: 10.1101/lm.042192.116
Lasek, A. (2016). Effects of ethanol on brain extracellular matrix: implications for alcohol use disorder. Alcohol Clin. Exp. Res. 40, 2030–2042. doi: 10.1111/acer.13200
Lendvai, D., Morawski, M., Bruckner, G., Negyessy, L., Baksa, G., Glasz, T., et al. (2012). Perisynaptic aggrecan-based extracellular matrix coats in the human lateral geniculate body devoid of perineuronal nets. J. Neurosci. Res. 90, 376–387. doi: 10.1002/jnr.22761
Lewandowski, S., Hodebourg, R., Wood, S., Carter, J., Nelson, K., Kalivas, P., et al. (2023). Matrix metalloproteinase activity during methamphetamine cued relapse. Addict. Biol. 28:e13279. doi: 10.1111/adb.13279
Lin, R., Rosahl, T., Whiting, P., Fawcett, J., and Kwok, J. (2011). 6-Sulphated chondroitins have a positive influence on axonal regeneration. PLoS One 6:e21499. doi: 10.1371/journal.pone.0021499
Liu, W., Han, Y., Liu, Y., Song, A., Barnes, B., and Song, X. (2010). Spinal matrix metalloproteinase-9 contributes to physical dependence on morphine in mice. J. Neurosci. 30, 7613–7623. doi: 10.1523/JNEUROSCI.1358-10.2010
Lowe, P., Morel, C., Ambade, A., Iracheta-Vellve, A., Kwiatkowski, E., Satishchandran, A., et al. (2020). Chronic alcohol-induced neuroinflammation involves CCR2/5-dependent peripheral macrophage infiltration and microglia alterations. J. Neuroinflamm. 17:296. doi: 10.1186/s12974-020-01972-5
Lu, L., Grimm, J., Hope, B., and Shaham, Y. (2004). Incubation of cocaine craving after withdrawal: a review of preclinical data. Neuropharmacology 47 Suppl. 1, 214–226. doi: 10.1016/j.neuropharm.2004.06.027
Lupori, L., Totaro, V., Cornuti, S., Ciampi, L., Carrara, F., Grilli, E., et al. (2023). A comprehensive atlas of perineuronal net distribution and colocalization with parvalbumin in the adult mouse brain. bioRxiv [Preprint] doi: 10.1101/2023.01.24.525313
Luppino, F., de Wit, L., Bouvy, P., Stijnen, T., Cuijpers, P., Penninx, B., et al. (2010). Overweight, obesity, and depression: a systematic review and meta-analysis of longitudinal studies. Arch. Gen. Psychiatry 67, 220–229. doi: 10.1001/archgenpsychiatry.2010.2
Ly, C., Greb, A., Cameron, L., Wong, J., Barragan, E., Wilson, P., et al. (2018). Psychedelics promote structural and functional neural plasticity. Cell Rep. 23, 3170–3182. doi: 10.1016/j.celrep.2018.05.022
Maeda, N. (2010). Structural variation of chondroitin sulfate and its roles in the central nervous system. Cent. Nerv. Syst. Agents Med. Chem. 10, 22–31.
Maeda, N. (2015). Proteoglycans and neuronal migration in the cerebral cortex during development and disease. Frontiers in neuroscience. 9:98. doi: 10.3389/fnins.2015.00098
Maeda, N., Ishii, M., Nishimura, K., and Kamimura, K. (2011). Functions of chondroitin sulfate and heparan sulfate in the developing brain. Neurochem. Res. 36, 1228–1240. doi: 10.1007/s11064-010-0324-y
Mafi, A., Hofer, L., Russ, M., Young, J., and Mellott, J. (2020). The density of perineuronal nets increases with age in the inferior colliculus in the fischer brown norway rat. Front. Aging Neurosci. 12:27. doi: 10.3389/fnagi.2020.00027
Main, M., Rao, S., and O’Keefe, J. (2010). Trends in obesity and extreme obesity among US adults. JAMA 303, 1695;authorrely–6. doi: 10.1001/jama.2010.517
Maiya, R., Zhou, Y., Norris, E., Kreek, M., and Strickland, S. (2009). Tissue plasminogen activator modulates the cellular and behavioral response to cocaine. Proc. Natl. Acad. Sci. U.S.A. 106, 1983–1988. doi: 10.1073/pnas.0812491106
Marchant, N. (2019). Break the net, break the cycle: removal of perineuronal nets in the lateral hypothalamus decreases cocaine relapse. Neuropsychopharmacology 44, 835–836. doi: 10.1038/s41386-018-0245-z
Martin, A., and Davidson, T. (2014). Human cognitive function and the obesogenic environment. Physiol. Behav. 136, 185–193. doi: 10.1016/j.physbeh.2014.02.062
Martin, D., and Nichols, C. (2016). Psychedelics recruit multiple cellular types and produce complex transcriptional responses within the brain. Ebiomedicine. 11, 262–277. doi: 10.1016/j.ebiom.2016.08.049
Mascio, G., Notartomaso, S., Martinello, K., Liberatore, F., Bucci, D., Imbriglio, T., et al. (2022). A progressive build-up of perineuronal nets in the somatosensory cortex is associated with the development of chronic pain in mice. J. Neurosci. 42, 3037–3048. doi: 10.1523/JNEUROSCI.1714-21.2022
Mash, D., ffrench-Mullen, J., Adi, N., Qin, Y., Buck, A., and Pablo, J. (2007). Gene expression in human hippocampus from cocaine abusers identifies genes which regulate extracellular matrix remodeling. PLoS One 2:e1187. doi: 10.1371/journal.pone.0001187
Matikainen-Ankney, B., and Kravitz, A. (2018). Persistent effects of obesity: a neuroplasticity hypothesis. Ann. N.Y. Acad. Sci. 1428, 221–239. doi: 10.1111/nyas.13665
Mauney, S., Athanas, K., Pantazopoulos, H., Shaskan, N., Passeri, E., Berretta, S., et al. (2013). Developmental pattern of perineuronal nets in the human prefrontal cortex and their deficit in schizophrenia. Biol. Psychiatry 74, 427–435. doi: 10.1016/j.biopsych.2013.05.007
McClernon, F., Conklin, C., Kozink, R., Adcock, R., Sweitzer, M., Addicott, M., et al. (2016). Hippocampal and insular response to smoking-related environments: neuroimaging evidence for drug-context effects in nicotine dependence. Neuropsychopharmacology 41, 877–885. doi: 10.1038/npp.2015.214
Mezu-Ndubuisi, O., and Maheshwari, A. (2021). The role of integrins in inflammation and angiogenesis. Pediatr. Res. 89, 1619–1626. doi: 10.1038/s41390-020-01177-9
Miguel-Hidalgo, J. (2023). Astrocytes as context for the involvement of myelin and nodes of ranvier in the pathophysiology of depression and stress-related disorders. J. Psychiatr. Brain Sci. 8:e230001. doi: 10.20900/jpbs.20230001
Milev, P., Maurel, P., Chiba, A., Mevissen, M., Popp, S., Yamaguchi, Y., et al. (1998). Differential regulation of expression of hyaluronan-binding proteoglycans in developing brain: aggrecan, versican, neurocan, and brevican. Biochem. Biophys. Res. Commun. 247, 207–212. doi: 10.1006/bbrc.1998.8759
Miller, A., and Spencer, S. (2014). Obesity and neuroinflammation: a pathway to cognitive impairment. Brain Behav. Immun. 42, 10–21. doi: 10.1016/j.bbi.2014.04.001
Mirzadeh, Z., Alonge, K., Cabrales, E., Herranz-Perez, V., Scarlett, J., Brown, J., et al. (2019). Perineuronal net formation during the critical period for neuronal maturation in the hypothalamic arcuate nucleus. Nat. Metab. 1, 212–221. doi: 10.1038/s42255-018-0029-0
Miyata, S., Komatsu, Y., Yoshimura, Y., Taya, C., and Kitagawa, H. (2012). Persistent cortical plasticity by upregulation of chondroitin 6-sulfation. Nat. Neurosci. 15, S1–S2. doi: 10.1038/nn.3023
Mizoguchi, H., Yamada, K., Mouri, A., Niwa, M., Mizuno, T., Noda, Y., et al. (2007). Role of matrix metalloproteinase and tissue inhibitor of MMP in methamphetamine-induced behavioral sensitization and reward: implications for dopamine receptor down-regulation and dopamine release. J. Neurochem. 102, 1548–1560. doi: 10.1111/j.1471-4159.2007.04623.x
Mohamedi, Y., Fontanil, T., Cobo, T., Cal, S., and Obaya, A. (2020). New insights into ADAMTS metalloproteases in the central nervous system. Biomolecules 10:403. doi: 10.3390/biom10030403
Morawski, M., Bruckner, G., Jager, C., Seeger, G., and Arendt, T. (2010). Neurons associated with aggrecan-based perineuronal nets are protected against tau pathology in subcortical regions in Alzheimer’s disease. Neuroscience 169, 1347–1363. doi: 10.1016/j.neuroscience.2010.05.022
Morawski, M., Bruckner, M., Riederer, P., Bruckner, G., and Arendt, T. (2004). Perineuronal nets potentially protect against oxidative stress. Exp. Neurol. 188, 309–315. doi: 10.1016/j.expneurol.2004.04.017
Moulton, E., Elman, I., Becerra, L., Goldstein, R., and Borsook, D. (2014). The cerebellum and addiction: insights gained from neuroimaging research. Addict. Biol. 19, 317–331. doi: 10.1111/adb.12101
Nadanaka, S., Miyata, S., Yaqiang, B., Tamura, J., Habuchi, O., and Kitagawa, H. (2020). Reconsideration of the Semaphorin-3A binding motif found in chondroitin sulfate using Galnac4s-6st-Knockout mice. Biomolecules 10:1499. doi: 10.3390/biom10111499
Nagy, V., Bozdagi, O., and Huntley, G. (2007). The extracellular protease matrix metalloproteinase-9 is activated by inhibitory avoidance learning and required for long-term memory. Learn. Mem. 14, 655–664. doi: 10.1101/lm.678307
Nakamoto, K., Kawasaki, S., Kobori, T., Fujita-Hamabe, W., Mizoguchi, H., Yamada, K., et al. (2012). Involvement of matrix metalloproteinase-9 in the development of morphine tolerance. Eur. J. Pharmacol. 683, 86–92. doi: 10.1016/j.ejphar.2012.03.006
Narendran, R., Lopresti, B., Mason, N., Deuitch, L., Paris, J., Himes, M., et al. (2014). Cocaine abuse in humans is not associated with increased microglial activation: an 18-kDa translocator protein positron emission tomography imaging study with [11C]PBR28. J. Neurosci. 34, 9945–9950. doi: 10.1523/jneurosci.0928-14.2014
Natarajan, R., Harding, J., and Wright, J. W. (2013). A role for matrix metalloproteinases in nicotine-induced conditioned place preference and relapse in adolescent female rats. J. Exp. Neurosci. 7, 1–14. doi: 10.4137/JEN.S11381
Negus, S., and Freeman, K. (2018). Abuse potential of biased mu opioid receptor agonists. Trends Pharmacol. Sci. 39, 916–919. doi: 10.1016/j.tips.2018.08.007
Nestler, E. (2005). The neurobiology of cocaine addiction. Sci. Pract. Perspect. 3, 4–10. doi: 10.1151/spp05314
Nguyen, P., Dorman, L., Pan, S., Vainchtein, I., Han, R., Nakao-Inoue, H., et al. (2020). Microglial remodeling of the extracellular matrix promotes synapse plasticity. Cell 182, 388–403.e15. doi: 10.1016/j.cell.2020.05.050
Niaura, R., Rohsenow, D., Binkoff, J., Monti, P., Pedraza, M., and Abrams, D. (1988). Relevance of cue reactivity to understanding alcohol and smoking relapse. J. Abnorm. Psychol. 97, 133–152. doi: 10.1037//0021-843x.97.2.133
Noh, H., Lee, H., Kim, D., Kang, S., Cho, G., Rho, J., et al. (2004). A cDNA microarray analysis of gene expression profiles in rat hippocampus following a ketogenic diet. Brain Res. Mol. Brain Res. 129, 80–87. doi: 10.1016/j.molbrainres.2004.06.020
Norwitz, N., Sethi, S., and Palmer, C. (2020). Ketogenic diet as a metabolic treatment for mental illness. Curr. Opin. Endocrinol. Diabetes Obes. 27, 269–274. doi: 10.1097/MED.0000000000000564
O’Connor, A., Burton, T., Mansuri, H., Hand, G., Leamey, C., and Sawatari, A. (2019). Environmental enrichment from birth impacts parvalbumin expressing cells and wisteria floribunda agglutinin labelled peri-neuronal nets within the developing murine striatum. Front. Neuroanat. 13:90. doi: 10.3389/fnana.2019.00090
Oliva, I., and Wanat, M. (2016). Ventral tegmental area afferents and drug-dependent behaviors. Front. Psychiatry 7:30. doi: 10.3389/fpsyt.2016.00030
Oltman, C., Coppey, L., Gellett, J., Davidson, E., Lund, D., and Yorek, M. (2005). Progression of vascular and neural dysfunction in sciatic nerves of Zucker diabetic fatty and Zucker rats. Am. J. Physiol. Endocrinol. Metab. 289, E113–E122. doi: 10.1152/ajpendo.00594.2004
Oommen, A., Roberts, K., Joshi, L., and Cunningham, S. (2023). Transcriptomic analysis of glycosylation and neuroregulatory pathways in rodent models in response to psychedelic molecules. Int. J. Mol. Sci. 24:1200. doi: 10.3390/ijms24021200
Page-McCaw, A., Ewald, A., and Werb, Z. (2007). Matrix metalloproteinases and the regulation of tissue remodelling. Nat. Rev. Mol. Cell Biol. 8, 221–233. doi: 10.1038/nrm2125
Pantazopoulos, H., Gisabella, B., Rexrode, L., Benefield, D., Yildiz, E., Seltzer, P., et al. (2020). Circadian rhythms of perineuronal net composition. eNeuro 7, ENEURO.34–ENEURO.19. doi: 10.1523/ENEURO.0034-19.2020
Pantazopoulos, H., Hossain, N., Chelini, G., Durning, P., Barbas, H., Zikopoulos, B., et al. (2022). Chondroitin sulphate proteoglycan axonal coats in the human mediodorsal thalamic nucleus. Front. Integr. Neurosci. 16:934764. doi: 10.3389/fnint.2022.934764
Pantazopoulos, H., Lange, N., Hassinger, L., and Berretta, S. (2006). Subpopulations of neurons expressing parvalbumin in the human amygdala. J. Comp. Neurol. 496, 706–722. doi: 10.1002/cne.20961
Pantazopoulos, H., Markota, M., Jaquet, F., Ghosh, D., Wallin, A., Santos, A., et al. (2015). Aggrecan and chondroitin-6-sulfate abnormalities in schizophrenia and bipolar disorder: a postmortem study on the amygdala. Transl. Psychiatry 5:e496. doi: 10.1038/tp.2014.128
Pantazopoulos, H., Woo, T., Lim, M., Lange, N., and Berretta, S. (2010). Extracellular matrix-glial abnormalities in the amygdala and entorhinal cortex of subjects diagnosed with schizophrenia. Arch. Gen. Psychiatry 67, 155–166. doi: 10.1001/archgenpsychiatry.2009.196
Parent, M., Darling, J., and Henderson, Y. (2014). Remembering to eat: hippocampal regulation of meal onset. Am. J. Physiol. Regul. Integr. Comp. Physiol. 306, R701–R713. doi: 10.1152/ajpregu.00496.2013
Peters, A., and Sherman, L. (2020). Diverse roles for hyaluronan and hyaluronan receptors in the developing and adult nervous system. Int. J. Mol. Sci. 21, 5988. doi: 10.3390/ijms21175988
Phillips, J., Norris, S., Talbot, J., Birmingham, M., Hatchard, T., Ortiz, A., et al. (2019). Single, repeated, and maintenance ketamine infusions for treatment-resistant depression: a randomized controlled trial. Am. J. Psychiatry 176, 401–409. doi: 10.1176/appi.ajp.2018.18070834
Pizzorusso, T., Medini, P., Berardi, N., Chierzi, S., Fawcett, J., and Maffei, L. (2002). Reactivation of ocular dominance plasticity in the adult visual cortex. Science 298, 1248–1251. doi: 10.1126/science.1072699
Powers, A. III, Gancsos, M., Finn, E., Morgan, P., and Corlett, P. (2015). Ketamine-induced hallucinations. Psychopathology 48, 376–385. doi: 10.1159/000438675
Quintero, G. (2013). Role of nucleus accumbens glutamatergic plasticity in drug addiction. Neuropsychiatr. Dis. Treat. 9, 1499–1512. doi: 10.2147/NDT.S45963
Ray, M., Williams, B., Kuppe, M., Bryant, C., and Logan, R. W. A. (2022). Glitch in the matrix: the role of extracellular matrix remodeling in opioid use disorder. Front. Integr. Neurosci. 16:899637. doi: 10.3389/fnint.2022.899637
Reichelt, A., Lemieux, C., Princz-Lebel, O., Singh, A., Bussey, T., and Saksida, L. (2021). Age-dependent and region-specific alteration of parvalbumin neurons, perineuronal nets and microglia in the mouse prefrontal cortex and hippocampus following obesogenic diet consumption. Sci. Rep. 11:5593. doi: 10.1038/s41598-021-85092-x
Rieser, N., Herdener, M., and Preller, K. (2022). Psychedelic-assisted therapy for substance use disorders and potential mechanisms of action. Curr. Top. Behav. Neurosci. 56, 187–211. doi: 10.1007/7854_2021_284
Riga, D., Kramvis, I., Koskinen, M., van Bokhoven, P., van der Harst, J., Heistek, T., et al. (2017). Hippocampal extracellular matrix alterations contribute to cognitive impairment associated with a chronic depressive-like state in rats. Sci. Transl. Med. 9:eaai8753. doi: 10.1126/scitranslmed.aai8753
Rivera, S., Khrestchatisky, M., Kaczmarek, L., Rosenberg, G., and Jaworski, D. (2010). Metzincin proteases and their inhibitors: foes or friends in nervous system physiology? J. Neurosci. 30, 15337–15357. doi: 10.1523/JNEUROSCI.3467-10.2010
Rogers, S., Rankin-Gee, E., Risbud, R., Porter, B., and Marsh, E. (2018). Normal development of the perineuronal net in humans; in patients with and without epilepsy. Neuroscience 384, 350–360. doi: 10.1016/j.neuroscience.2018.05.039
Rossier, J., Bernard, A., Cabungcal, J., Perrenoud, Q., Savoye, A., Gallopin, T., et al. (2015). Cortical fast-spiking parvalbumin interneurons enwrapped in the perineuronal net express the metallopeptidases Adamts8, Adamts15 and Neprilysin. Mol. Psychiatry 20, 154–161. doi: 10.1038/mp.2014.162
Roura-Martinez, D., Diaz-Bejarano, P., Ucha, M., Paiva, R., Ambrosio, E., and Higuera-Matas, A. (2020). Comparative analysis of the modulation of perineuronal nets in the prefrontal cortex of rats during protracted withdrawal from cocaine, heroin and sucrose self-administration. Neuropharmacology 180:108290. doi: 10.1016/j.neuropharm.2020.108290
Rucker, J., Jelen, L., Flynn, S., Frowde, K., and Young, A. (2016). Psychedelics in the treatment of unipolar mood disorders: a systematic review. J. Psychopharmacol. 30, 1220–1229. doi: 10.1177/0269881116679368
Saksela, O. (1985). Plasminogen activation and regulation of pericellular proteolysis. Biochim. Biophys. Acta 823, 35–65. doi: 10.1016/0304-419x(85)90014-9
SAMHSA (2018). Key substance use and mental health indicators in the United States: results from the 2017 national survey on drug use and health. Rockville, MD: SAMHSA.
Samochowiec, A., Grzywacz, A., Kaczmarek, L., Bienkowski, P., Samochowiec, J., Mierzejewski, P., et al. (2010). Functional polymorphism of matrix metalloproteinase-9 (MMP-9) gene in alcohol dependence: family and case control study. Brain Res. 1327, 103–106. doi: 10.1016/j.brainres.2010.02.072
Samuel, V., Rajeev, T., Ramesh, L., and Sundararaman, A. (2023). Integrin receptor trafficking in health and disease. Prog. Mol. Biol. Transl. Sci. 196, 271–302. doi: 10.1016/bs.pmbts.2022.09.008
Sarnyai, Z., and Palmer, C. (2020). Ketogenic therapy in serious mental illness: emerging evidence. Int. J. Neuropsychopharmacol. 23, 434–439. doi: 10.1093/ijnp/pyaa036
Scarlett, J., Hu, S., and Alonge, K. (2022). The “Loss” of perineuronal nets in Alzheimer’s disease: missing or hiding in plain sight? Front. Integr. Neurosci. 16:896400. doi: 10.3389/fnint.2022.896400
Scarlett, J., Rojas, J., Matsen, M., Kaiyala, K., Stefanovski, D., Bergman, R., et al. (2016). Central injection of fibroblast growth factor 1 induces sustained remission of diabetic hyperglycemia in rodents. Nat. Med. 22, 800–806. doi: 10.1038/nm.4101
Schuster, T., Krug, M., Stalder, M., Hackel, N., Gerardy-Schahn, R., and Schachner, M. (2001). Immunoelectron microscopic localization of the neural recognition molecules L1, NCAM, and its isoform NCAM180, the NCAM-associated polysialic acid, beta1 integrin and the extracellular matrix molecule tenascin-R in synapses of the adult rat hippocampus. J. Neurobiol. 49, 142–158. doi: 10.1002/neu.1071
Schwartz, N., and Domowicz, M. (2023). Chemistry and function of glycosaminoglycans in the nervous system. Adv. Neurobiol. 29, 117–162. doi: 10.1007/978-3-031-12390-0_5
Seney, M., Kim, S., Glausier, J., Hildebrand, M., Xue, X., Zong, W., et al. (2021). Transcriptional alterations in dorsolateral prefrontal cortex and nucleus accumbens implicate neuroinflammation and synaptic remodeling in opioid use disorder. Biol. Psychiatry 90, 550–562. doi: 10.1016/j.biopsych.2021.06.007
Shao, L., Liao, C., Gregg, I., Davoudian, P., Savalia, N., Delagarza, K., et al. (2021). Psilocybin induces rapid and persistent growth of dendritic spines in frontal cortex in vivo. Neuron 109, 2535–44.e4. doi: 10.1016/j.neuron.2021.06.008
Shin, C., and Kim, Y. (2020). Ketamine in major depressive disorder: mechanisms and future perspectives. Psychiatry Investig. 17, 181–192. doi: 10.30773/pi.2019.0236
Siddiqui, N., Oshima, K., and Hippensteel, J. (2022). Proteoglycans and glycosaminoglycans in central nervous system injury. Am. J. Physiol. Cell Physiol. 323, C46–C55. doi: 10.1152/ajpcell.00053.2022
Silver, D., and Silver, J. (2014). Contributions of chondroitin sulfate proteoglycans to neurodevelopment, injury, and cancer. Curr. Opin. Neurobiol. 27, 171–178. doi: 10.1016/j.conb.2014.03.016
Slaker, M., Barnes, J., Sorg, B., and Grimm, J. (2016a). Impact of environmental enrichment on perineuronal nets in the prefrontal cortex following early and late abstinence from sucrose self-administration in rats. PLoS One 11:e0168256. doi: 10.1371/journal.pone.0168256
Slaker, M., Blacktop, J., and Sorg, B. (2016b). Caught in the net: perineuronal nets and addiction. Neural Plast. 2016:7538208. doi: 10.1155/2016/7538208
Slaker, M., Churchill, L., Todd, R., Blacktop, J., Zuloaga, D., Raber, J., et al. (2015). Removal of perineuronal nets in the medial prefrontal cortex impairs the acquisition and reconsolidation of a cocaine-induced conditioned place preference memory. J. Neurosci. 35, 4190–4202. doi: 10.1523/JNEUROSCI.3592-14.2015
Slaker, M., Jorgensen, E., Hegarty, D., Liu, X., Kong, Y., Zhang, F., et al. (2018). Cocaine exposure modulates perineuronal nets and synaptic excitability of fast-spiking interneurons in the medial prefrontal cortex. eNeuro 5:ENEURO.221–ENEURO.218. doi: 10.1523/ENEURO.0221-18.2018
Sloshower, J., Skosnik, P., Safi-Aghdam, H., Pathania, S., Syed, S., Pittman, B., et al. (2023). Psilocybin-assisted therapy for major depressive disorder: an exploratory placebo-controlled, fixed-order trial. J. Psychopharmacol. 2698811231154852. doi: 10.1177/02698811231154852 [Epub ahead of print].
Smith, A., Kupchik, Y., Scofield, M., Gipson, C., Wiggins, A., Thomas, C., et al. (2014). Synaptic plasticity mediating cocaine relapse requires matrix metalloproteinases. Nat. Neurosci. 17, 1655–1657. doi: 10.1038/nn.3846
Smith, A., Nealey, K., Wright, J., and Walker, B. (2011). Plasticity associated with escalated operant ethanol self-administration during acute withdrawal in ethanol-dependent rats requires intact matrix metalloproteinase systems. Neurobiol. Learn. Mem. 96, 199–206. doi: 10.1016/j.nlm.2011.04.011
Smith, A., Scofield, M., and Kalivas, P. (2015). The tetrapartite synapse: extracellular matrix remodeling contributes to corticoaccumbens plasticity underlying drug addiction. Brain Res. 1628(Pt. A), 29–39. doi: 10.1016/j.brainres.2015.03.027
Smith-Thomas, L., Stevens, J., Fok-Seang, J., Faissner, A., Rogers, J., and Fawcett, J. (1995). Increased axon regeneration in astrocytes grown in the presence of proteoglycan synthesis inhibitors. J. Cell Sci. 108(Pt. 3), 1307–1315. doi: 10.1242/jcs.108.3.1307
Spencer, M., Minino, A., and Warner, M. (2022). Drug overdose deaths in the United States, 2001-2021. NCHS Data Brief 457, 1–8.
Steullet, P., Cabungcal, J., Bukhari, S., Ardelt, M., Pantazopoulos, H., Hamati, F., et al. (2018). The thalamic reticular nucleus in schizophrenia and bipolar disorder: role of parvalbumin-expressing neuron networks and oxidative stress. Mol. Psychiatry. 23, 2057–2065. doi: 10.1038/mp.2017.230
Strous, J., Weeland, C., van der Draai, F., Daams, J., Denys, D., Lok, A., et al. (2022). Brain changes associated with long-term ketamine abuse, a systematic review. Front. Neuroanat. 16:795231. doi: 10.3389/fnana.2022.795231
Sugiyama, S., Di Nardo, A., Aizawa, S., Matsuo, I., Volovitch, M., Prochiantz, A., et al. (2008). Experience-dependent transfer of Otx2 homeoprotein into the visual cortex activates postnatal plasticity. Cell 134, 508–520. doi: 10.1016/j.cell.2008.05.054
Susuki, K., Chang, K., Zollinger, D., Liu, Y., Ogawa, Y., Eshed-Eisenbach, Y., et al. (2013). Three mechanisms assemble central nervous system nodes of Ranvier. Neuron 78, 469–482. doi: 10.1016/j.neuron.2013.03.005
Tabet, A., Apra, C., Stranahan, A., and Anikeeva, P. (2022). Changes in brain neuroimmunology following injury and disease. Front. Integr. Neurosci. 16:894500. doi: 10.3389/fnint.2022.894500
Tajerian, M., and Clark, J. (2015). The role of the extracellular matrix in chronic pain following injury. Pain 156, 366–370. doi: 10.1097/01.j.pain.0000460323.80020.9d
Talin, P., and Sanabria, E. (2017). Ayahuasca’s entwined efficacy: an ethnographic study of ritual healing from ‘addiction’. Int. J. Drug Policy 44, 23–30. doi: 10.1016/j.drugpo.2017.02.017
Tansley, S., Gu, N., Guzman, A., Cai, W., Wong, C., Lister, K., et al. (2022). Microglia-mediated degradation of perineuronal nets promotes pain. Science 377, 80–86. doi: 10.1126/science.abl6773
Thomsen, M., Routhe, L., and Moos, T. (2017). The vascular basement membrane in the healthy and pathological brain. J. Cereb. Blood Flow Metab. 37, 3300–3317. doi: 10.1177/0271678X17722436
Tran, A., and Silver, J. (2021). Cathepsins in neuronal plasticity. Neural Regen. Res. 16, 26–35. doi: 10.4103/1673-5374.286948
Trevino-Alvarez, A., Sanchez-Ruiz, J., Barrera, F., Rodriguez-Bautista, M., Romo-Nava, F., McElroy, S., et al. (2023). Weight changes in adults with major depressive disorder: a systematic review and meta-analysis of prospective studies. J. Affect. Disord. 332, 1–8. doi: 10.1016/j.jad.2023.03.050
Tsuji, K., Aoki, T., Tejima, E., Arai, K., Lee, S., Atochin, D., et al. (2005). Tissue plasminogen activator promotes matrix metalloproteinase-9 upregulation after focal cerebral ischemia. Stroke 36, 1954–1959. doi: 10.1161/01.STR.0000177517.01203.eb
Ueno, H., Suemitsu, S., Murakami, S., Kitamura, N., Wani, K., Matsumoto, Y., et al. (2019). Layer-specific expression of extracellular matrix molecules in the mouse somatosensory and piriform cortices. IBRO Rep. 6, 1–17. doi: 10.1016/j.ibror.2018.11.006
Ueno, H., Suemitsu, S., Murakami, S., Kitamura, N., Wani, K., Okamoto, M., et al. (2017). Postnatal development of GABAergic interneurons and perineuronal nets in mouse temporal cortex subregions. Int. J. Dev. Neurosci. 63, 27–37. doi: 10.1016/j.ijdevneu.2017.08.003
Ueno, H., Takao, K., Suemitsu, S., Murakami, S., Kitamura, N., Wani, K., et al. (2018). Age-dependent and region-specific alteration of parvalbumin neurons and perineuronal nets in the mouse cerebral cortex. Neurochem. Int. 112, 59–70. doi: 10.1016/j.neuint.2017.11.001
Valeri, J., O’Donovan, S., Wang, W., Sinclair, D., Bollavarapu, R., Gisabella, B., et al. (2022). Altered expression of somatostatin signaling molecules and clock genes in the hippocampus of subjects with substance use disorder. Front. Neurosci. 16:903941. doi: 10.3389/fnins.2022.903941
Van den Oever, M., Lubbers, B., Goriounova, N., Li, K., Van der Schors, R., Loos, M., et al. (2010). Extracellular matrix plasticity and GABAergic inhibition of prefrontal cortex pyramidal cells facilitates relapse to heroin seeking. Neuropsychopharmacology 35, 2120–2133. doi: 10.1038/npp.2010.90
Van Dyken, P., and Lacoste, B. (2018). Impact of metabolic syndrome on neuroinflammation and the blood-brain barrier. Front. Neurosci. 12:930. doi: 10.3389/fnins.2018.00930
Vazquez-Sanroman, D., Leto, K., Cerezo-Garcia, M., Carbo-Gas, M., Sanchis-Segura, C., Carulli, D., et al. (2015). The cerebellum on cocaine: plasticity and metaplasticity. Addict. Biol. 20, 941–955. doi: 10.1111/adb.12223
Vazquez-Sanroman, D., Monje, R., and Bardo, M. (2017). Nicotine self-administration remodels perineuronal nets in ventral tegmental area and orbitofrontal cortex in adult male rats. Addict. Biol. 22, 1743–1755. doi: 10.1111/adb.12437
Venturino, A., and Siegert, S. (2021). Minimally invasive protocols and quantification for microglia-mediated perineuronal net disassembly in mouse brain. STAR Protoc. 2:101012. doi: 10.1016/j.xpro.2021.101012
Venturino, A., Schulz, R., De Jesus-Cortes, H., Maes, M., Nagy, B., Reilly-Andujar, F., et al. (2021). Microglia enable mature perineuronal nets disassembly upon anesthetic ketamine exposure or 60-Hz light entrainment in the healthy brain. Cell Rep. 36:109313. doi: 10.1016/j.celrep.2021.109313
Volkow, N., Wang, G., Tomasi, D., and Baler, R. (2013a). Obesity and addiction: neurobiological overlaps. Obes. Rev. 14, 2–18. doi: 10.1111/j.1467-789X.2012.01031.x
Volkow, N., Wang, G., Tomasi, D., and Baler, R. (2013b). The addictive dimensionality of obesity. Biol. Psychiatry 73, 811–818. doi: 10.1016/j.biopsych.2012.12.020
Vujic, T., Schvartz, D., Furlani, I., Meister, I., Gonzalez-Ruiz, V., Rudaz, S., et al. (2022). Oxidative stress and extracellular matrix remodeling are signature pathways of extracellular vesicles released upon morphine exposure on human brain microvascular endothelial cells. Cells 11:3926. doi: 10.3390/cells11233926
Wang, H., Katagiri, Y., McCann, T., Unsworth, E., Goldsmith, P., Yu, Z., et al. (2008). Chondroitin-4-sulfation negatively regulates axonal guidance and growth. J. Cell Sci. 121(Pt. 18), 3083–3091. doi: 10.1242/jcs.032649
Warlow, S., Robinson, M., and Berridge, K. (2017). Optogenetic central amygdala stimulation intensifies and narrows motivation for cocaine. J. Neurosci. 37, 8330–8348. doi: 10.1523/JNEUROSCI.3141-16.2017
Wei, J., Lambert, T. Y., Valada, A., Patel, N., Walker, K., Lenders, J., et al. (2023). Single nucleus transcriptomics reveals pervasive glial activation in opioid overdose cases. bioRxiv [Preprint]. doi: 10.1101/2023.03.07.531400
Weiss, F. (2005). Neurobiology of craving, conditioned reward and relapse. Curr. Opin. Pharmacol. 5, 9–19. doi: 10.1016/j.coph.2004.11.001
Wiggins, A., Pacchioni, A., and Kalivas, P. (2009). Integrin expression is altered after acute and chronic cocaine. Neurosci. Lett. 450, 321–323. doi: 10.1016/j.neulet.2008.12.006
Willis, A., Pratt, J., and Morris, B. (2022). Enzymatic degradation of cortical perineuronal nets reverses GABAergic interneuron maturation. Mol. Neurobiol. 59, 2874–2893. doi: 10.1007/s12035-022-02772-z
Wingert, J., and Sorg, B. (2021). Impact of perineuronal nets on electrophysiology of parvalbumin interneurons, principal neurons, and brain oscillations: a review. Front. Synaptic Neurosci. 13:673210. doi: 10.3389/fnsyn.2021.673210
Wright, J., Masino, A., Reichert, J., Turner, G., Meighan, S., Meighan, P., et al. (2003). Ethanol-induced impairment of spatial memory and brain matrix metalloproteinases. Brain Res. 963, 252–261. doi: 10.1016/s0006-8993(02)04036-2
Wu, L., Ghitza, U., Zhu, H., Spratt, S., Swartz, M., and Mannelli, P. (2018). Substance use disorders and medical comorbidities among high-need, high-risk patients with diabetes. Drug Alcohol Depend. 186, 86–93. doi: 10.1016/j.drugalcdep.2018.01.008
Xiong, T., Wang, X., Zha, Y., and Wang, Y. (2022). Interleukin-33 regulates the functional state of microglia. Front. Cell. Neurosci. 16:1012968. doi: 10.3389/fncel.2022.1012968
Xue, Y., Xue, L., Liu, J., He, J., Deng, J., Sun, S., et al. (2014). Depletion of perineuronal nets in the amygdala to enhance the erasure of drug memories. J. Neurosci. 34, 6647–6658. doi: 10.1523/JNEUROSCI.5390-13.2014
Yin, L., Feng, R., Xie, X., Yang, X., Yang, Z., Hu, J., et al. (2023). Matrix metalloproteinase-9 overexpression in the hippocampus reduces alcohol-induced conditioned-place preference by regulating synaptic plasticity in mice. Behav. Brain Res. 442:114330. doi: 10.1016/j.bbr.2023.114330
Zarate, C. Jr., Singh, J., Carlson, P., Brutsche, N., Ameli, R., Luckenbaugh, D., et al. (2006). A randomized trial of an N-methyl-D-aspartate antagonist in treatment-resistant major depression. Arch. Gen. Psychiatry 63, 856–864. doi: 10.1001/archpsyc.63.8.856
Zhang, N., Yan, Z., Liu, H., Yu, M., He, Y., Liu, H., et al. (2021). Hypothalamic perineuronal nets are regulated by sex and dietary interventions. Front Physiol. 12:714104. doi: 10.3389/fphys.2021.714104
Zhang, S., Zhornitsky, S., Angarita, G., and Li, C. (2020). Hypothalamic response to cocaine cues and cocaine addiction severity. Addict. Biol. 25:e12682. doi: 10.1111/adb.12682
Zironi, I., Burattini, C., Aicardi, G., and Janak, P. (2006). Context is a trigger for relapse to alcohol. Behav. Brain Res. 167, 150–155. doi: 10.1016/j.bbr.2005.09.007
Keywords: perineuronal net, extracellular matrix, substance use disorder, metabolic disorder, synaptic plasticity
Citation: Valeri J, Gisabella B and Pantazopoulos H (2023) Dynamic regulation of the extracellular matrix in reward memory processes: a question of time. Front. Cell. Neurosci. 17:1208974. doi: 10.3389/fncel.2023.1208974
Received: 20 April 2023; Accepted: 30 May 2023;
Published: 16 June 2023.
Edited by:
Bhanu P. Tewari, University of Virginia, United StatesReviewed by:
Víctor Carriel, University of Granada, SpainBarbara A. Sorg, Legacy Research Institute, United States
Copyright © 2023 Valeri, Gisabella and Pantazopoulos. This is an open-access article distributed under the terms of the Creative Commons Attribution License (CC BY). The use, distribution or reproduction in other forums is permitted, provided the original author(s) and the copyright owner(s) are credited and that the original publication in this journal is cited, in accordance with accepted academic practice. No use, distribution or reproduction is permitted which does not comply with these terms.
*Correspondence: Harry Pantazopoulos, Y3BhbnRhem9wb3Vsb3NAdW1jLmVkdQ==