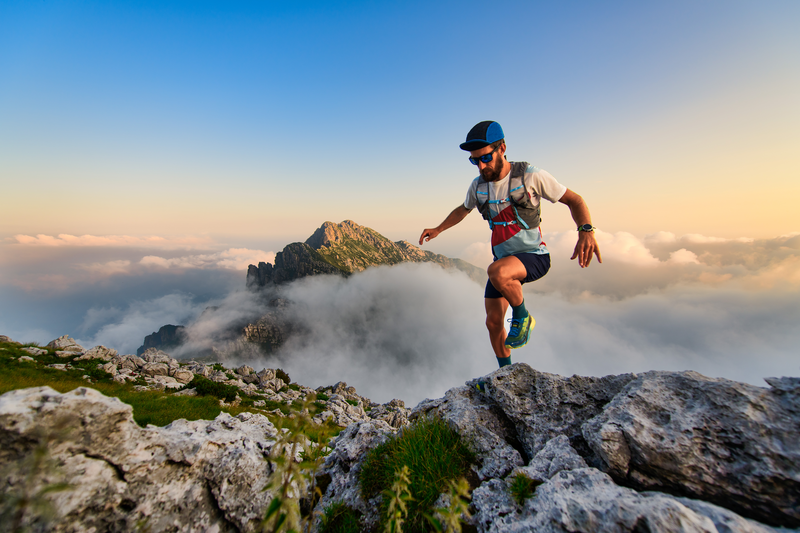
94% of researchers rate our articles as excellent or good
Learn more about the work of our research integrity team to safeguard the quality of each article we publish.
Find out more
REVIEW article
Front. Cell. Neurosci. , 28 July 2022
Sec. Cellular Neurophysiology
Volume 16 - 2022 | https://doi.org/10.3389/fncel.2022.951202
This article is part of the Research Topic Reviews in Cellular Neurophysiology 2022: Neurophysiological Mechanisms in the Aging Brain View all 5 articles
Neurological disorders are a group of disorders with motor, sensory or cognitive damage, caused by dysfunction of the central or peripheral nervous system. Cyclin-dependent kinases 5 (Cdk5) is of vital significance for the development of the nervous system, including the migration and differentiation of neurons, the formation of synapses, and axon regeneration. However, when the nervous system is subject to pathological stimulation, aberrant activation of Cdk5 will induce abnormal phosphorylation of a variety of substrates, resulting in a cascade signaling pathway, and thus lead to pathological changes. Cdk5 is intimately related to the pathological mechanism of a variety of neurological disorders, such as A-β protein formation in Alzheimer’s disease, mitochondrial fragmentation in cerebral ischemia, and apoptosis of dopaminergic neurons in Parkinson’s disease. It is worth noting that Cdk5 inhibitors have been reported to have neuroprotective effects by inhibiting related pathological processes. Therefore, in this review, we will briefly introduce the physiological and pathological mechanisms of Cdk5 in the nervous system, focusing on the recent advances of Cdk5 in neurological disorders and the prospect of targeted Cdk5 for the treatment of neurological disorders.
Neurological disorders are featured by impairment movement, sensation, cognition, and behavior, which is caused by damage to the peripheral nervous system or central nervous system. These diseases share similar pathophysiological mechanisms, such as oxidative stress, cytotoxicity, mitochondrial fragmentation, autophagy, endoplasmic reticulum stress, neuroinflammation, and calcium overload. However, divergent pathological manifestations exist in the different disorders (Sorensen, 2019). Cyclin-dependent kinase 5 (Cdk5) is a proline-directed serine/threonine kinase, which is a unique component of the family of cyclin-dependent kinases (Dhavan and Tsai, 2001; Malumbres, 2014). Cdk5 plays a pivotal role in the nervous system, including cortex layer formation, synaptic growth and maturation, synaptic vesicular transport (Liu et al., 2022; Takahashi et al., 2022a), stress-enhanced memory consolidation, dendritic spine formation, neuronal migration and differentiation, neurite outgrowth and length (Chen et al., 2017; Huang et al., 2017; Shinmyo et al., 2017; Lee et al., 2018; Nishino et al., 2019; Rao et al., 2020; Im et al., 2022), learning and long-term behavioral changes, axonal regeneration (Xu et al., 2017; Hwang and Namgung, 2021), brain microtubule network and actin cytoskeleton remodeling (Shah and Lahiri, 2017; Shah and Rossie, 2018), as well as normal cerebellar development and functions (Lee et al., 2019; Li et al., 2019; Kodani et al., 2020; Ouyang et al., 2020). Additionally, Cdk5 also plays a key role in gene expression, cell differentiation, angiogenesis, and aging (Arif, 2012).
Cdk5 plays an important role in the pathological process of neurological diseases. Cdk5 binds to specific partners p35 and p39, after the pathological stimulus. P35 and p39 are cleaved to p25 and p29 by calpain, with the increase in calcium concentration. The association of Cdk5/p25 is more stable and leads to aberrant hyperphosphorylation of substantial Cdk5 substrates, resulting in cell death or apoptosis (Asada et al., 2012; Nie et al., 2022). Cdk5 has also been implicated in the development and progression of a variety of cancers, including breast, lung, colon, pancreatic, melanoma, thyroid, and brain tumors, making it a promising drug target for new anticancer treatments (Pozo and Bibb, 2016).
When Cdk5 inhibitors are given, they show neuroprotective effects on numerous cell and animal models. Cdk5 inhibitors have great potential to be a therapeutic target for neurological system diseases. In this review, we will summarize recent advances in the molecular mechanisms of Cdk5 in neurological diseases as well as the therapeutic potential of Cdk5 in these neurological diseases.
Under normal circumstances, Cdk5 is in an inactive state. After binding to p35, it is normally activated, phosphorylates many substrates, and plays normal physiological functions such as neuron development and development, axonal dendrite growth, and prominent functions. When neurons are pathologically stimulated, the influx of intracellular calcium ions increases, and after combining with calcium, p35 is split into p25. The combination of p25 and CDK5 will cause CDK5 to be in an over-activated state, thereby hyperphosphorylating various substrates in cells, causing abnormal pathophysiological responses, and leading to neurological diseases (Figure 1).
Cdk5 hyperactivation contributes to several neurodegenerative diseases, including Alzheimer’s disease (AD), Parkinson’s disease (PD), and Huntington’s disease (HD; Cheung and Ip, 2012).
Aβ protein deposition is not only the pathological feature of AD but also the key factor of AD progression. Aβ is produced through sequential amyloidogenic cleavage of precursor protein APP by β-secretase BACE1 and γ-secretase, with the oligomer form considered to be the toxic form (Mawuenyega et al., 2010). The mechanisms underlying Cdk5 in Aβ generation and neurotoxicity are multifaceted. Cdk5 activation promotes Aβ generation and accumulation in neurons. PPAR-β, a nuclear receptor with a key role in metabolic processes, is a phosphorylated substrate of Cdk5 and involved in Aβ generation (Quan et al., 2019; Ribeiro Filho et al., 2019). Hypoxia leads to abnormal phosphorylation of Cdk5 and accelerates the process of AD (Fang et al., 2019). Cdk5/HIF-1 contributes to Aβ generation (Chao et al., 2020). Cdk5 regulates Aβ-induced mitochondrial fission and neurotoxicity by phosphorylating Drp1 (Guo et al., 2018), which may be mediated by P27 (Jaiswal and Sharma, 2017). APP, GSK-3 β, and TrkA play important roles in Aβ pathological process, which is at least partly mediated by Cdk5 activation (Palop and Mucke, 2010). Aβ regulates the activity of Cdk5 as well (Lapresa et al., 2019). Aβ protein physiological aggregation regulates Cdk5/Calcineurin signaling (Lazarevic et al., 2017). Therefore, inhibiting the activity of Cdk5 is of great significance in reducing Aβ generation and delaying the progress of AD. Roscovitine, an inhibitor of Cdk5, effectively reverses Aβ formation. Ginsenoside Rg1 can decrease Aβ level via inhibiting the Cdk5/ PPAR γ pathway (Quan et al., 2020). Some other drugs, such as streptozotocin, ketamine, and phosphocreatine, also decrease Aβ levels through Cdk5 related pathway (Li et al., 2020a; Park et al., 2020; Ai et al., 2022). Therefore, targeting Cdk5 and the related pathways is a potential strategy for AD therapy.
Tau phosphorylation and the formation of neurofibrillary tangles in neurons is one of the notable hallmarks of AD pathology. Aberrant phosphorylation and deposition of tau, which is a cellular microtubule associated protein, will affect the activity of microtubule and destroy cytoskeleton structure, leading to neuronal apoptosis. Tau is a substrate of different kinases, such as Cdk5, GSK-3β, or PKA. Cdk5 plays a key role in tau phosphorylation and neurofibrillary tangles formation (Seo et al., 2017). The miR-148a-3p/p35/PTEN signaling pathway is an important pathway for tau hyperphosphorylation in AD (Zeng et al., 2021). Many factors such as MARK4 (Saito et al., 2019), and RPS23RG1 (Zhao et al., 2021), have been proved to be related to tan pathology through regulating Cdk5 activity. Glutamate induces Cdk5 and p35 mRNA transcription. The glutamate-responsive increase of the Cdk5/p25 complex contributes to tau hyperphosphorylation (Tanaka et al., 2022). Cdk5 is also associated with early inflammation of AD (Wilkaniec et al., 2018). Leukotriene, an inflammatory factor, may accelerate tau pathological accumulation through the Cdk5 pathway (Giannopoulos et al., 2019). Tau affects the function of subcellular organelles, such as mitochondria and Golgi apparatus, through Cdk5 (Mohamed et al., 2017). Cdk5 inhibitory peptide (CIP) can not only prevent the loss of neurons and alleviate behavioral changes but also reduce tau hyperphosphorylation and inflammation (Xu et al., 2019; Huang et al., 2020). Drugs like Quercetin can inhibit the pathological process of tau through the Ca2+-calpain-p25-Cdk5 pathway (Shen et al., 2018). For the pivotal role of Cdk5 in tau phosphorylation and the formation of neurofibrillary tangles, Cdk5 is supposed to be a promising therapeutic target for AD.
Cdk5 inhibitors can delay the pathological progression of AD and prevent neuronal apoptosis. They have not been put into clinical trials because of poor selectivity. More and more studies continue to try to find new Cdk5 inhibitors and new substrates of Cdk5 (Zhuang et al., 2020b). Recent studies have found some new substrates and signaling pathways related to Cdk5 in AD, such asCdk5-Mcl-1axis (Nikhil and Shah, 2017), ALDH1A1 (Nikhil et al., 2019), miR-125b (Zhuang et al., 2020a), and miR-504-3p (Chen et al., 2022). New Cdk5 inhibitors are also being studied in various AD models like pyrrolidine-2,3-dione, and TFP5 (Shukla et al., 2017; Zeb et al., 2019a, b). It is also found that a traditional Chinese medicine, Nano-HO, improves cognitive function in AD by modulating the signaling pathway JNK/cdk5/GSK-3β (Qu et al., 2021). Similarly, Kaixinsan, a traditional Chinese medicine for insomnia, is found to attenuate tau hyperphosphorylation and neuroinflammation by inhibiting GSK3β and CDK5 activation (Jiao et al., 2022). All of these chemicals have shown neuroprotective effect but the specific mechanism is still not fully elucidated.
The main pathological change of Parkinson’s disease is the degeneration of substantia nigra pars compacta neurons. However, the mechanism of Lewy body formation is still unclear. Moreover, there is no effective treatment to slow down the process of neurodegeneration (Kalia and Lang, 2015). Dysregulation of Cdk5 is supposed to be related to the loss of dopaminergic neurons and the progression of PD. Aberrant p25/Cdk5 signaling was found in early-stage PD (He et al., 2020). Previous studies found that Cdk5 can promote oxidative stress, and lead to mitochondrial dysfunction and autophagy dysfunction in PD. Moreover, in the mouse model of PD induced by MTPP, aberrant Cdk5 results in activation of inflammation (Cheng et al., 2020), immune hyperactivity (Shukla et al., 2019), mitochondrial fission (Park et al., 2019), and degradation of ubiquitin ligases (Wang et al., 2018), leading to the loss of dopaminergic neurons. Cdk5 phosphorylation induced nuclear translocation of SIRT2 also leads to the loss of dopaminergic neurons (Yan et al., 2022). Similarly, Cdk5 inhibitors have exerted neuroprotective effects in PD (He et al., 2018). Luteolin was reported to confer neuroprotective effect on the PD model, which was also mediated by Cdk5 (Reudhabibadh et al., 2021). Therefore, based on previous studies, Cdk5 has been demonstrated to play a critical role in the development of PD, thus making it to be a pivotal target for PD therapy (Table 1).
Table 1. Summary of the main targets of cdk5 in neurological diseases and mechanism of Cdk5 and nervous system diseases.
Huntington’s disease (HD) is an autosomal dominant disease with a combination of motor, cognitive, and behavioral characteristics. HD is caused by the extended CAG trinucleotide repeat (variable length) in HTT (the gene encoding protein huntingtin; Bates et al., 2015). Huntington protein leads to neuronal dysfunction and death through a variety of mechanisms, including proteinase deposition, destruction of transcription and mitochondrial function, and direct toxicity of mutant protein (McColgan and Tabrizi, 2018). Cdk5 has been confirmed to participate in the pathological process (Bowles and Jones, 2014). It was suggested that, unlike in AD and PD, Cdk5 exerts neuroprotective effects in HD (Kaminosono et al., 2008). However, some other studies have yielded different results. It was reported that Cdk5 knockout with overexpression of mutated huntingtin (MHTT) alleviated cortical striatal learning deficits and hippocampus dependent memory decline (Alvarez-Periel et al., 2018). P25/Cdk5 signaling is an important mediator of dopamine and glutamate neurotoxicity associated with HD (Paoletti et al., 2008). Cdk5 mediates dopaminergic neurotoxicity by regulating Drp1, which induces mitochondrial fragmentation in HD pathology (Cherubini et al., 2015). In the nucleus accumbens, Cdk5 dysfunction regulates DARPP-32 phosphorylation, which contributes to depression-like behavior in HD (Brito et al., 2019). Therefore, Cdk5 is supposed to get double-sided nature in HD diseases. How to use its beneficial side and how to prevent its detrimental side is a task worthy of consideration in the future (Figure 2).
Figure 2. The role of Cdk5 in neurodegenerative diseases. →: promote or aggravate; ⊣: inhibit or protect.
Cerebrovascular disease is the most common disease in the nervous system and one of the major threats to human health and life worldwide (Langhorne et al., 2011). In recent years, there is evidence that targeting Cdk5 can protect synaptic plasticity and provide long-term neuroprotection after stroke (Gutierrez-Vargas et al., 2017).
Cerebral ischemia is one of the most serious public health problems worldwide (Tolonen et al., 2005). The important pathophysiological mechanisms of ischemic stroke are neuroinflammation, oxidative stress, calcium overload, mitochondrial fragmentation, and Golgi stress caused by ischemia and hypoxia (Sun et al., 2019). As a unique Cdk in the nervous system, Cdk5 has been demonstrated to play an important role in the pathological process of ischemic stroke. In a large number of animal models of cerebral ischemia or neuronal cell ischemia models, Zincchelator (Tuo et al., 2018), neuregulin-1 β (Zhang et al., 2018), tfp5 (Ji et al., 2017), and tat-Cdk5 CTM (Zhu et al., 2019) are found to have a neuroprotective effect through Cdk5 related pathways. Cdk5 inhibition with scCdk5mir astrocytes (Becerra-Calixto and Cardona-Gomez, 2017), Cdk5 RNAi-based therapy (Munoz-Manco et al., 2018) or transplanted with PTPN21 (Cui et al., 2017) also confers neuroprotection in ischemic stroke. The mechanisms underlying Cdk5 in ischemia stroke are multifaceted, such as protecting cells through the ERK1/2 signaling pathway (Zhao et al., 2017), inhibition of p53 dependent apoptosis (Shin et al., 2019), trkb-erk1/2-creb pathway (Liu et al., 2017), phosphorylation of drp1s616 (Chen et al., 2021) and sponging miR-148b-3p (Chen et al., 2022). Given that reducing the level of Cdk5 in astrocytes could protect against brain damage in cerebrovascular diseases (Becerra-Calixto et al., 2018), it is believed that Cdk5 has great potential in the treatment of ischemic stroke.
Intracerebral hemorrhage (ICH) is a common cerebrovascular disorder, accompanied by a particularly high mortality. The prognosis of ICH is not satisfying. Thus, it is essential to understand the potential molecular mechanisms of ICH-induced brain injury (Wilson et al., 2015). The most serious damage of cerebral hemorrhage to neurons is due to hematoma compression and hemorrhagic inflammation related pathological changes, while most of the subcellular pathological changes are similar to ischemic stroke. Inhibition of Cdk5 activity, such as knockout of Cdk5 kinase activity (Ke et al., 2015) or glycosylated Cdk5 (Ning et al., 2017), also showed neuroprotective effect in intracerebral hemorrhage. The Cdk5-ATM signaling pathway has been demonstrated to protect neurons in the process of cerebral hemorrhage (Wu et al., 2016). Phosphorylation of p35 also attenuated neuronal apoptosis through Cdk5 (Roufayel and Murshid, 2019). Moreover, p75NTR promotedp53 dephosphorylation and induced neuronal apoptosis after intracerebral hemorrhage (Zhou et al., 2019). Therefore, Cdk5 plays an important role in intracerebral hemorrhage and is a potential therapeutic target.
Neuropathic pain is caused by a lesion or disease of the somatosensory system, including peripheral fibers and central neurons (Bouhassira, 2019). The development of NP is caused by many pathophysiological mechanisms that affect pain pathways (Colloca et al., 2017). As a unique Cdk of the nervous system, Cdk5 has been proved to play an important role in the pathogenesis of NP. The role of Cdk5 in NP and its potential substrates, such as channels, proteins involved in neurotransmitter release, and receptors, were discussed in many studies (Gomez et al., 2020b). Researchers have visualized the regulation of trigeminal sensory neurons by Cdk5, showed the expression change of Cdk5 and the accumulation of calcium ions, and provided a strong basis for revealing the pathological mechanism of neuralgia (Hu et al., 2022). The level of Cdk5 and phosphorylated CRMP2 was increased in NP models, and inhibition of CRMP2 could alleviate NP (Moutal et al., 2019). Cdk5 inhibitors can inhibit neuralgia through the Cdk5-NR2A pathway (Yang et al., 2014) or attenuate the response of TRPA1 (Sulak et al., 2018). Cdk5 also plays a critical role in regulating myelin basic protein (MBP) fragment (Chernov et al., 2018), inflammatory pain (Zhu et al., 2021), and calcium channel (Gomez et al., 2020a, 2021) in NP. Cdk5 mediated cyclic AMP response element binding protein (CREB; Li et al., 2014) and regulated NP through Cdk5/PPAR γ pathway (Zhong et al., 2019). Silencing noncoding RNA H19 can relieve by inhibiting Cdk5 mediated phosphorylation of CREB (Li et al., 2020). Based on these findings, Cdk5 is supposed to be a potential target to attenuate neuralgia.
Epilepsy is a brain disease, with more than 70 million people suffering from epilepsy worldwide (Thijs et al., 2019). The pathophysiological mechanism of epilepsy is not fully clarified. There is a high incidence of comorbidity and premature mortality in patients with epilepsy (Yuen et al., 2018). It is important to understand the molecular mechanism of epilepsy in order to find new prognostic/diagnostic biomarkers. Cdk5 naturally plays an important role in the pathological process of epilepsy. Cdk5 maintains the steady-state synaptic plasticity by regulating the synaptic cascade in neurons. In the animal refractory epilepsy model, the expression of the Cdk5 gene at the transcriptional level has been proved to be abnormal (Dixit et al., 2017). Cdk5 plays different roles in different brain regions in patients with mesial temporal lobe epilepsy with hippocampal sclerosis (Banerjee et al., 2021). The occurrence and development of epilepsy are related to the blood-brain barrier. It is verified that endothelial specific Cdk5 knockout induced spontaneous seizures in mice (Liu et al., 2020). In status epilepticus, Cdk5 promotes neuronal apoptosis through excessive mitochondrial fragmentation (Kim and Kang, 2017), regulates neuroinflammation (Hiragi et al., 2018), and endoplasmic reticulum stress (Lee and Kim, 2021). After status epilepticus, Cdk5 was less expressed in CA1 cells in animal models (Kim and Kang, 2018). Roscovitine, a Cdk5 inhibitor, inhibits status epilepticus-induced neuroinflammation by regulating p38 MAPK-mediated microglial response (Kim et al., 2019). P35 and P39, Cdk5 activators, have also been shown to play a significant role in synaptic function and epileptic response (Li et al., 2016). The change of Cdk5/p35 expression in the hippocampus may play a role in epilepsy by affecting mossy fiber germination (Tian et al., 2008, 2010). It is well known that NMDAR is intimately related to epilepsy. NMDAR induced axon injury in temporal lobe epilepsy through regulating GSK-3 β and Cdk5 (Liu et al., 2017; Fan et al., 2020). However, more studies are still needed to unveil the role of Cdk5 in the pathophysiological process of epilepsy.
Glioblastoma (GBM) is an aggressive malignant primary brain tumor. Currently, there are only palliative treatments such as radiotherapy and chemotherapy (Stupp et al., 2009). It is important to find effective therapeutic drugs for GBM. The aberrant activity of Cdk5 is found in various tumors, including GBM. A biosensor for Cdk5 can be used to probe Cdk5 activity in living glioblastoma cells by fluorescence imaging (Peyressatre et al., 2020b). The level of Cdk5 may be a potential biomarker for early diagnosis of GBM (Gonzalez-Vera et al., 2016). Cdk5 is also identified as a valuable predictive marker for tumorigenesis and progression in GBM. Cdk5 can promote the migration, invasion, and progression of GBM by phosphorylating PIKE-A (Liu et al., 2008) and Drp1 (Xie et al., 2015). Cdk5 inhibition by TP5 (Tabouret et al., 2020), AC1MYR2 (Ren et al., 2015), inhibition of DYRK1A (Chen et al., 2021), Cdk5 knockdown (Zhou et al., 2021), and a new quinazolinone family (Peyressatre et al., 2020a) can suppress the progression of GBM. Moreover, it is supposed that targeting the Cdk5/TRIM59 signal axis (Sang et al., 2019) and OGT/CDK5/ACSS2 pathway (Ciraku et al., 2022) may be future strategies for the treatment of GBM (Figure 3).
Figure 3. Molecular mechanisms of Cdk5 in some of the neurological diseases. →: promote or aggravate; ⊣: inhibit or protect.
Cdk5 is essential in regulating the transformation of the precursor cells of adult oligodendrocytes to mature oligodendrocytes (Luo et al., 2014). Cdk5 plays an important role in the formation of myelin sheath for oligodendrocytes. Abnormal Cdk5 activity can lead to demyelination-related diseases, such as multiple sclerosis (Luo et al., 2016). Cdk5 activity in oligodendrocytes contributes to demyelination and cognitive dysfunction in a mouse model of multiple sclerosis (Luo et al., 2018). However, the specific mechanism for Cdk5 in multiple sclerosis is still unclear. It is found that Cdk5 can participate in the pathological process of multiple sclerosis by regulating lymphocyte activation (Pareek et al., 2010).
Cdk5 has been proved to play a key role in many other neurological diseases, such as ALS (Bk et al., 2019), early brain injury (Ding et al., 2022), cerebral amyloidosis (Kiss et al., 2020), fragile X-associated tremor/ataxia syndrome (FXTAS; Robin et al., 2017), spinal muscular atrophy (Tejero et al., 2020), and systemic sclerosis (Wei et al., 2017). Circadian behavior (Zhou et al., 2022), learning disabilities (Kamiki et al., 2018), and aging (Spurrier et al., 2018) are also closely related to Cdk5. Diabetes induces brain damage by regulating Cdk5 phosphorylation (Li et al., 2020b). Inhibition of Cdk5 improves glucose uptake in insulin-resistant neuronal cells via the ERK1/2 pathway (Manglani and Dey, 2022), and alleviates cognitive deficits caused by diabetes (Liu et al., 2019). Inhibition of the Cdk5/caspase-3 pathway by p5-TAT can also attenuate radiation-induced cognitive dysfunction (Zhang et al., 2021). Inhibition of Cdk5 activity ameliorates anxiety and depression in mice (Takahashi et al., 2022b). Cdk5 is found to induce mitochondrial kinetic defects in optic neuropathy (Rong et al., 2020). Cdk5 phosphorylates CRMP-2, which will aggravate optic nerve damage (Chen et al., 2019). Cdk5 contributes to oxidative stress (Guo et al., 2018) and endoplasmic reticulum stress (Shi et al., 2018). Nestin is closely related to Cdk5 signaling (Lindqvist et al., 2017) and the nestin-Cdk5-drp1 axis regulates neural stem cell stemness (Wang et al., 2018). The role of Cdk5 in nervous system diseases may be different in male and female models (Barrett et al., 2019). Estrogen promotes axon regeneration after subcortical axon injury through the PI3K/Akt/CDK5/Tau pathway (Xia et al., 2020). Epigenetic editing of Cdk5 also has been applied to neurological disorders, such as sex-specific regulation of fear memory (Sase et al., 2019). Till now, researchers try to find new phosphorylation substrates of Cdk5 (Roach et al., 2018), and discover new Cdk5 inhibitors by various biotechnology methods (Shukla and Singh, 2020, 2022). In a recent study, a highly selective inhibitor of CDK5, GFB-12811, was discovered and optimized (Daniels et al., 2022). A brain-penetrating Cdk5 inhibitor was also developed and found to alter neurobehavior (Figure 4; Umfress et al., 2022).
Figure 4. Pathologicalmechanisms of Cdk5 in neurological disorders. When neurons are subjected to pathological stimuli, such as ischemia and toxic injury. Cdk5 is highly activated after binding to p25, phosphorylates many substrates, and also induces mitochondrial fragmentation, Golgi apparatus and endoplasmic reticulum dysfunction, and destruction of the cytoskeleton. Cdk5 inhibitors can attenuate or even reverse the above pathological processes.
In general, our understanding of Cdk5 in neurological disorders has made great progress in recent years. A large number of studies have confirmed its important physiological function and its toxic effect after over-activation. It is increasingly clear and certain that Cdk5 plays a pivotal role in the physiological function of the nervous system and the pathological process of neurological disorders. Cdk5 inhibitors have shown promising effects in numerous studies and Cdk5 has great potential as a therapeutic target for neurological disorders. However, many issues, such as the more detailed molecular mechanisms of Cdk5 in different neurological disorders and the development of more selective inhibitors of CDK5, still need to be further clarified before its clinical application.
CA and LZ conceived, organized, and discussed the work. CA contributed to manuscript writing and literature search. CL, JC, JT, and LZ revised the manuscript. All authors contributed to the article and approved the submitted version.
This work has been supported by National Natural Science Foundation of China (Grant no. 81771423 and 81974212) and Natural Science Foundation of Hunan province (Grant no. 2020JJ4822).
The authors declare that the research was conducted in the absence of any commercial or financial relationships that could be construed as a potential conflict of interest.
All claims expressed in this article are solely those of the authors and do not necessarily represent those of their affiliated organizations, or those of the publisher, the editors and the reviewers. Any product that may be evaluated in this article, or claim that may be made by its manufacturer, is not guaranteed or endorsed by the publisher.
Ai, J., Wang, H., Chu, P., Shopit, A., Niu, M., Ahmad, N., et al. (2022). The neuroprotective effects of phosphocreatine on amyloid beta 25-35-induced differentiated neuronal cell death through inhibition of AKT/GSK-3β/Tau/APP/CDK5 pathways in vivo and vitro. Free Radic. Biol. Med. 179, 416–417. doi: 10.1016/j.freeradbiomed.2021.12.306
Alvarez-Periel, E., Puigdellivol, M., Brito, V., Plattner, F., Bibb, J. A., Alberch, J., et al. (2018). Cdk5 contributes to Huntington’s disease learning and memory deficits via modulation of brain region-specific substrates. Mol. Neurobiol. 55, 6250–6268. doi: 10.1007/s12035-017-0828-4
Arif, A. (2012). Extraneuronal activities and regulatory mechanisms of the atypical cyclin-dependent kinase Cdk5. Biochem. Pharmacol. 84, 985–993. doi: 10.1016/j.bcp.2012.06.027
Asada, A., Saito, T., and Hisanaga, S. (2012). Phosphorylation of p35 and p39 by Cdk5 determines the subcellular location of the holokinase in a phosphorylation-site-specific manner. J. Cell Sci. 125, 3421–3429. doi: 10.1242/jcs.100503
Banerjee, J., Srivastava, A., Sharma, D., Dey, S., Tripathi, M., Sharma, M. C., et al. (2021). Differential regulation of excitatory synaptic transmission in the hippocampus and anterior temporal lobe by cyclin dependent kinase 5 (Cdk5) in mesial temporal lobe epilepsy with hippocampal sclerosis (MTLE-HS). Neurosci. Lett. 761:136096. doi: 10.1016/j.neulet.2021.136096
Barrett, T., Marchalant, Y., and Park, H. J. K. (2019). p35 hemizygous deletion in 5xFAD mice increases Aβ plaque load in males but not in females. Neuroscience 417, 45–56. doi: 10.1016/j.neuroscience.2019.08.017
Bates, G. P., Dorsey, R., Gusella, J. F., Hayden, M. R., Kay, C., Leavitt, B. R., et al. (2015). Huntington disease. Nat. Rev. Dis. Primers 1:15005. doi: 10.1038/nrdp.2015.5
Becerra-Calixto, A., and Cardona-Gomez, G. P. (2017). Neuroprotection induced by transplanted CDK5 knockdown astrocytes in global cerebral ischemic rats. Mol. Neurobiol. 54, 6681–6696. doi: 10.1007/s12035-016-0162-2
Becerra-Calixto, A., Posada-Duque, R., and Cardona-Gomez, G. P. (2018). Recovery of neurovascular unit integrity by CDK5-KD astrocyte transplantation in a global cerebral ischemia model. Mol. Neurobiol. 55, 8563–8585. doi: 10.1007/s12035-018-0992-1
Bk, B., Skuntz, S., Prochazkova, M., Kesavapany, S., Amin, N. D., Shukla, V., et al. (2019). Overexpression of the Cdk5 inhibitory peptide in motor neurons rescue of amyotrophic lateral sclerosis phenotype in a mouse model. Hum. Mol. Genet. 28, 3175–3187. doi: 10.1093/hmg/ddz118
Bouhassira, D. (2019). Neuropathic pain: definition, assessment and epidemiology. Rev. Neurol. (Paris) 175, 16–25. doi: 10.1016/j.neurol.2018.09.016
Bowles, K. R., and Jones, L. (2014). Kinase signalling in Huntington’s disease. J. Huntingtons Dis. 3, 89–123. doi: 10.3233/JHD-140106
Brito, V., Giralt, A., Masana, M., Royes, A., Espina, M., Sieiro, E., et al. (2019). Cyclin-dependent kinase 5 dysfunction contributes to depressive-like behaviors in Huntington’s disease by altering the DARPP-32 phosphorylation status in the nucleus accumbens. Biol. Psychiatry 86, 196–207. doi: 10.1016/j.biopsych.2019.03.001
Chao, A. C., Chen, C. H., Wu, M. H., Hou, B. Y., and Yang, D. I. (2020). Roles of Id1/HIF-1 and CDK5/HIF-1 in cell cycle reentry induced by amyloid-beta peptide in post-mitotic cortical neuron. Biochim. Biophys. Acta Mol. Cell Res. 1867:118628. doi: 10.1016/j.bbamcr.2019.118628
Chen, H. R., Juan, H. C., Wong, Y. H., Tsai, J. W., and Fann, M. J. (2017). Cdk12 regulates neurogenesis and late-arising neuronal migration in the developing cerebral cortex. Cereb. Cortex 27, 2289–2302. doi: 10.1093/cercor/bhw081
Chen, D., Lan, G., Li, R., Mei, Y., Shui, X., Gu, X., et al. (2022). Melatonin ameliorates tau-related pathology via the miR-504-3p and CDK5 axis in Alzheimer’s disease. Transl. Neurodegener. 11:27. doi: 10.1186/s40035-022-00302-4
Chen, B., McCuaig-Walton, D., Tan, S., Montgomery, A. P., Day, B. W., Kassiou, M., et al. (2021). DYRK1A negatively regulates CDK5-SOX2 pathway and self-renewal of glioblastoma stem cells. Int. J. Mol. Sci. 22:4011. doi: 10.3390/ijms22084011
Chen, C., Peng, X., Tang, J., Hu, Z., Tan, J., and Zeng, L. (2021). CDK5 inhibition protects against OGDR induced mitochondrial fragmentation and apoptosis through regulation of Drp1S616 phosphorylation. Life Sci. 269:119062. doi: 10.1016/j.lfs.2021.119062
Chen, G., Shan, X., Li, L., Dong, L., Huang, G., and Tao, H. (2022). circHIPK3 regulates apoptosis and mitochondrial dysfunction induced by ischemic stroke in mice by sponging miR-148b-3p via CDK5R1/SIRT1. Exp. Neurol. 355:114115. doi: 10.1016/j.expneurol.2022.114115
Chen, X., Yuan, R., Gao, L., Huang, C., Fan, W., Ye, J., et al. (2019). Induction of CRMP-2 phosphorylation by CDK5 restricts the repair of damaged optic nerve. J. Cell. Physiol. 234, 11240–11246. doi: 10.1002/jcp.27778
Cheng, X., Xu, S., Zhang, C., Qin, K., Yan, J., and Shao, X. (2020). The BRCC3 regulated by Cdk5 promotes the activation of neuronal NLRP3 inflammasome in Parkinson’s disease models. Biochem. Biophys. Res. Commun. 522, 647–654. doi: 10.1016/j.bbrc.2019.11.141
Chernov, A. V., Remacle, A. G., Hullugundi, S. K., Cieplak, P., Angert, M., Dolkas, J., et al. (2018). Amino acid sequence conservation of the algesic fragment of myelin basic protein is required for its interaction with CDK5 and function in pain. FEBS J. 285, 3485–3502. doi: 10.1111/febs.14623
Cherubini, M., Puigdellivol, M., Alberch, J., and Gines, S. (2015). Cdk5-mediated mitochondrial fission: a key player in dopaminergic toxicity in Huntington’s disease. Biochim. Biophys. Acta 1852, 2145–2160. doi: 10.1016/j.bbadis.2015.06.025
Cheung, Z. H., and Ip, N. Y. (2012). Cdk5: a multifaceted kinase in neurodegenerative diseases. Trends Cell Biol. 22, 169–175. doi: 10.1016/j.tcb.2011.11.003
Ciraku, L., Bacigalupa, Z. A., Ju, J., Moeller, R. A., Le Minh, G., Lee, R. H., et al. (2022). O-GlcNAc transferase regulates glioblastoma acetate metabolism via regulation of CDK5-dependent ACSS2 phosphorylation. Oncogene 41, 2122–2136. doi: 10.1038/s41388-022-02237-6
Colloca, L., Ludman, T., Bouhassira, D., Baron, R., Dickenson, A. H., Yarnitsky, D., et al. (2017). Neuropathic pain. Nat. Rev. Dis. Primers 3:17002. doi: 10.1038/nrdp.2017.2
Cui, N., Lu, H., Li, M., and Yan, Q. (2017). PTPN21 protects PC12 cell against oxygen-glucose deprivation by activating cdk5 through ERK1/2 signaling pathway. Eur. J. Pharmacol. 814, 226–231. doi: 10.1016/j.ejphar.2017.08.021
Daniels, M. H., Malojcic, G., Clugston, S. L., Williams, B., Coeffet-Le Gal, M., Pan-Zhou, X. R., et al. (2022). Discovery and optimization of highly selective inhibitors of CDK5. J. Med. Chem. 65, 3575–3596. doi: 10.1021/acs.jmedchem.1c02069
Dhavan, R., and Tsai, L. H. (2001). A decade of CDK5. Nat. Rev. Mol. Cell Biol. 2, 749–759. doi: 10.1038/35096019
Ding, Y., Zhang, L., Zhou, W., Lu, H., Gao, X., Li, J., et al. (2022). Role of cyclin-dependent kinase 5 in early brain injury following experimental subarachnoid hemorrhage. Exp. Ther. Med. 23:147. doi: 10.3892/etm.2021.11070
Dixit, A. B., Banerjee, J., Tripathi, M., Sarkar, C., and Chandra, P. S. (2017). Synaptic roles of cyclin-dependent kinase 5 & its implications in epilepsy. Indian J. Med. Res. 145, 179–188. doi: 10.4103/ijmr.IJMR_1249_14
Fan, C., Gao, Y., Liang, G., Huang, L., Wang, J., Yang, X., et al. (2020). Transcriptomics of Gabra4 knockout mice reveals common NMDAR pathways underlying autism, memory and epilepsy. Mol. Autism 11:13. doi: 10.1186/s13229-020-0318-9
Fang, B., Zhao, Q., Ling, W., Zhang, Y., and Ou, M. (2019). Hypoxia induces HT-22 neuronal cell death via Orai1/CDK5 pathway-mediated Tau hyperphosphorylation. Am. J. Transl. Res. 11, 7591–7603.
Giannopoulos, P. F., Chiu, J., and Pratico, D. (2019). Learning impairments, memory deficits and neuropathology in aged tau transgenic mice are dependent on leukotrienes biosynthesis: role of the cdk5 kinase pathway. Mol. Neurobiol. 56, 1211–1220. doi: 10.1007/s12035-018-1124-7
Gomez, K., Calderon-Rivera, A., Sandoval, A., Gonzalez-Ramirez, R., Vargas-Parada, A., Ojeda-Alonso, J., et al. (2020a). Cdk5-dependent phosphorylation of CaV3.2 t-type channels: possible role in nerve ligation-induced neuropathic allodynia and the compound action potential in primary afferent C fibers. J. Neurosci. 40, 283–296. doi: 10.1523/JNEUROSCI.0181-19.2019
Gomez, K., Vallecillo, T. G. M., Moutal, A., Perez-Miller, S., Delgado-Lezama, R., Felix, R., et al. (2020b). The role of cyclin-dependent kinase 5 in neuropathic pain. Pain 161, 2674–2689. doi: 10.1097/j.pain.0000000000002027
Gomez, K., Vargas-Parada, A., Duran, P., Sandoval, A., Delgado-Lezama, R., Khanna, R., et al. (2021). L5-6 spinal nerve ligation-induced neuropathy changes the location and function of Ca2+ channels and Cdk5 and affects the compound action potential in adjacent intact L4 afferent fibers. Neuroscience 471, 20–31. doi: 10.1016/j.neuroscience.2021.07.013
Gonzalez-Vera, J. A., Fueyo-Gonzalez, F., Alkorta, I., Peyressatre, M., Morris, M. C., and Herranz, R. (2016). Highly solvatochromic and tunable fluorophores based on a 4,5-quinolimide scaffold: novel CDK5 probes. Chem. Commun. (Camb) 52, 9652–9655. doi: 10.1039/c6cc04566a
Guo, M. Y., Shang, L., Hu, Y. Y., Jiang, L. P., Wan, Y. Y., Zhou, Q. Q., et al. (2018). The role of Cdk5-mediated Drp1 phosphorylation in Aβ1-42 induced mitochondrial fission and neuronal apoptosis. J. Cell. Biochem. 119, 4815–4825. doi: 10.1002/jcb.26680
Guo, D., Xie, W., Xiong, P., Li, H., Wang, S., Chen, G., et al. (2018). Cyclin-dependent kinase 5-mediated phosphorylation of chloride intracellular channel 4 promotes oxidative stress-induced neuronal death. Cell Death Dis. 9:951. doi: 10.1038/s41419-018-0983-1
Gutierrez-Vargas, J. A., Moreno, H., and Cardona-Gomez, G. P. (2017). Targeting CDK5 post-stroke provides long-term neuroprotection and rescues synaptic plasticity. J. Cereb. Blood Flow Metab. 37, 2208–2223. doi: 10.1177/0271678X16662476
He, R., Huang, W., Huang, Y., Xu, M., Song, P., Huang, Y., et al. (2018). Cdk5 inhibitory peptide prevents loss of dopaminergic neurons and alleviates behavioral changes in an MPTP induced Parkinson’s disease mouse model. Front. Aging Neurosci. 10:162. doi: 10.3389/fnagi.2018.00162
He, F., Qi, G., Zhang, Q., Cai, H., Li, T., Li, M., et al. (2020). Quantitative phosphoproteomic analysis in alpha-synuclein transgenic mice reveals the involvement of aberrant p25/Cdk5 signaling in early-stage Parkinson’s disease. Cell. Mol. Neurobiol. 40, 897–909. doi: 10.1007/s10571-019-00780-7
Hiragi, T., Ikegaya, Y., and Koyama, R. (2018). Microglia after seizures and in epilepsy. Cells 7:26. doi: 10.3390/cells7040026
Hu, M., Doyle, A. D., Yamada, K. M., and Kulkarni, A. B. (2022). Visualization of trigeminal ganglion sensory neuronal signaling regulated by Cdk5. Cell Rep. 38:110458. doi: 10.1016/j.celrep.2022.110458
Huang, Y., Huang, W., Huang, Y., Song, P., Zhang, M., Zhang, H. T., et al. (2020). Cdk5 inhibitory peptide prevents loss of neurons and alleviates behavioral changes in p25 transgenic mice. J. Alzheimers Dis. 74, 1231–1242. doi: 10.3233/JAD-191098
Huang, H., Lin, X., Liang, Z., Zhao, T., Du, S., Loy, M. M. T., et al. (2017). Cdk5-dependent phosphorylation of liprinα1 mediates neuronal activity-dependent synapse development. Proc. Natl. Acad. Sci. U S A 114, E6992–E7001. doi: 10.1073/pnas.1708240114
Hwang, J., and Namgung, U. (2021). Phosphorylation of STAT3 by axonal Cdk5 promotes axonal regeneration by modulating mitochondrial activity. Exp. Neurol. 335:113511. doi: 10.1016/j.expneurol.2020.113511
Im, D. S., Joselin, A., Svoboda, D., Takano, T., Rousseaux, M. W. C., Callaghan, S., et al. (2022). Cdk5-mediated JIP1 phosphorylation regulates axonal outgrowth through Notch1 inhibition. BMC Biol. 20:115. doi: 10.1186/s12915-022-01312-4
Jaiswal, S., and Sharma, P. (2017). Role and regulation of p27 in neuronal apoptosis. J. Neurochem. 140, 576–588. doi: 10.1111/jnc.13918
Ji, Y. B., Zhuang, P. P., Ji, Z., Huang, K. B., Gu, Y., Wu, Y. M., et al. (2017). TFP5 is comparable to mild hypothermia in improving neurological outcomes in early-stage ischemic stroke of adult rats. Neuroscience 343, 337–345. doi: 10.1016/j.neuroscience.2016.12.009
Jiao, Y. N., Zhang, J. S., Qiao, W. J., Tian, S. Y., Wang, Y. B., Wang, C. Y., et al. (2022). Kai-xin-san inhibits tau pathology and neuronal apoptosis in aged SAMP8 mice. Mol. Neurobiol. 59, 3294–3309. doi: 10.1007/s12035-021-02626-0
Kalia, L. V., and Lang, A. E. (2015). Parkinson’s disease. Lancet 386, 896–912. doi: 10.1016/S0140-6736(14)61393-3
Kamiki, E., Boehringer, R., Polygalov, D., Ohshima, T., and McHugh, T. J. (2018). Inducible knockout of the cyclin-dependent kinase 5 activator p35 alters hippocampal spatial coding and neuronal excitability. Front. Cell. Neurosci. 12:138. doi: 10.3389/fncel.2018.00138
Kaminosono, S., Saito, T., Oyama, F., Ohshima, T., Asada, A., Nagai, Y., et al. (2008). Suppression of mutant Huntingtin aggregate formation by Cdk5/p35 through the effect on microtubule stability. J. Neurosci. 28, 8747–8755. doi: 10.1523/JNEUROSCI.0973-08.2008
Ke, K., Shen, J., Song, Y., Cao, M., Lu, H., Liu, C., et al. (2015). CDK5 contributes to neuronal apoptosis via promoting MEF2D phosphorylation in rat model of intracerebral hemorrhage. J. Mol. Neurosci. 56, 48–59. doi: 10.1007/s12031-014-0466-5
Kim, J. E., and Kang, T. C. (2017). p47Phox/CDK5/DRP1-mediated mitochondrial fission evokes PV cell degeneration in the rat dentate gyrus following status epilepticus. Front. Cell. Neurosci. 11:267. doi: 10.3389/fncel.2017.00267
Kim, J. E., and Kang, T. C. (2018). Suppression of nucleocytoplasmic p27(Kip1) export attenuates CDK4-mediated neuronal death induced by status epilepticus. Neurosci. Res. 132, 46–52. doi: 10.1016/j.neures.2017.10.001
Kim, J. E., Park, H., Choi, S. H., Kong, M. J., and Kang, T. C. (2019). Roscovitine attenuates microglia activation and monocyte infiltration via p38 MAPK inhibition in the rat frontoparietal cortex following status epilepticus. Cells 8:746. doi: 10.3390/cells8070746
Kiss, E., Groeneweg, F., Gorgas, K., Schlicksupp, A., Kins, S., Kirsch, J., et al. (2020). Amyloid-β fosters p35/CDK5 signaling contributing to changes of inhibitory synapses in early stages of cerebral amyloidosis. J. Alzheimers Dis. 74, 1167–1187. doi: 10.3233/JAD-190976
Kodani, A., Kenny, C., Lai, A., Gonzalez, D. M., Stronge, E., Sejourne, G. M., et al. (2020). Posterior neocortex-specific regulation of neuronal migration by CEP85L identifies maternal centriole-dependent activation of CDK5. Neuron 106, 246–255.e6. doi: 10.1016/j.neuron.2020.01.030
Langhorne, P., Bernhardt, J., and Kwakkel, G. (2011). Stroke rehabilitation. Lancet 377, 1693–1702. doi: 10.1016/S0140-6736(11)60325-5
Lapresa, R., Agulla, J., Sanchez-Moran, I., Zamarreno, R., Prieto, E., Bolanos, J. P., et al. (2019). Amyloid-ss promotes neurotoxicity by Cdk5-induced p53 stabilization. Neuropharmacology 146, 19–27. doi: 10.1016/j.neuropharm.2018.11.019
Lazarevic, V., Fienko, S., Andres-Alonso, M., Anni, D., Ivanova, D., Montenegro-Venegas, C., et al. (2017). Physiological concentrations of amyloid β regulate recycling of synaptic vesicles via Alpha7 acetylcholine receptor and CDK5/Calcineurin signaling. Front. Mol. Neurosci. 10:221. doi: 10.3389/fnmol.2017.00221
Lee, D. S., and Kim, J. E. (2021). Regional specific activations of ERK1/2 and CDK5 differently regulate astroglial responses to ER stress in the rat hippocampus following status epilepticus. Brain Res. 1753:147262. doi: 10.1016/j.brainres.2020.147262
Lee, J., Ko, Y. U., Chung, Y., Yun, N., Kim, M., Kim, K., et al. (2018). The acetylation of cyclin-dependent kinase 5 at lysine 33 regulates kinase activity and neurite length in hippocampal neurons. Sci. Rep. 8:13676. doi: 10.1038/s41598-018-31785-9
Lee, D. K., Lee, H., Yoon, J., Hong, S., Lee, Y., Kim, K. T., et al. (2019). Cdk5 regulates N-cadherin-dependent neuronal migration during cortical development. Biochem. Biophys. Res. Commun. 514, 645–652. doi: 10.1016/j.bbrc.2019.04.166
Li, W., Allen, M. E., Rui, Y., Ku, L., Liu, G., Bankston, A. N., et al. (2016). p39 is responsible for increasing Cdk5 activity during postnatal neuron differentiation and governs neuronal network formation and epileptic responses. J. Neurosci. 36, 11283–11294. doi: 10.1523/JNEUROSCI.1155-16.2016
Li, K., Jiao, Y., Ren, X., You, D., and Cao, R. (2020). Long noncoding RNA H19 induces neuropathic pain by upregulating cyclin-dependent kinase 5-mediated phosphorylation of cAMP response element binding protein. J. Pain Res. 13, 2113–2124. doi: 10.2147/JPR.S240273
Li, M. X., Qiao, H., Zhang, M., and Ma, X. M. (2019). Role of Cdk5 in Kalirin7-mediated formation of dendritic spines. Neurochem. Res. 44, 1243–1251. doi: 10.1007/s11064-019-02771-y
Li, Y., Wen, G., Ding, R., Ren, X., Jing, C., Liu, L., et al. (2020a). Effects of single-dose and long-term ketamine administration on tau phosphorylation-related enzymes GSK-3β, CDK5, PP2A and PP2B in the mouse hippocampus. J. Mol. Neurosci. 70, 2068–2076. doi: 10.1007/s12031-020-01613-9
Li, Y., Xiang, Q., Yao, Y. H., Li, J. J., Wang, Y., and Li, X. H. (2020b). Inactivated AMPK-α2 promotes the progression of diabetic brain damage by Cdk5 phosphorylation at Thr485 site. Biochimie 168, 277–284. doi: 10.1016/j.biochi.2019.11.010
Li, K., Zhao, G. Q., Li, L. Y., Wu, G. Z., and Cui, S. S. (2014). Epigenetic upregulation of Cdk5 in the dorsal horn contributes to neuropathic pain in rats. Neuroreport 25, 1116–1121. doi: 10.1097/WNR.0000000000000237
Lindqvist, J., Torvaldson, E., Gullmets, J., Karvonen, H., Nagy, A., Taimen, P., et al. (2017). Nestin contributes to skeletal muscle homeostasis and regeneration. J. Cell Sci. 130, 2833–2842. doi: 10.1242/jcs.202226
Liu, G. T., Kochlamazashvili, G., Puchkov, D., Muller, R., Schultz, C., Mackintosh, A. I., et al. (2022). Endosomal phosphatidylinositol 3-phosphate controls synaptic vesicle cycling and neurotransmission. EMBO J. 41:e109352. doi: 10.15252/embj.2021109352
Liu, Y., Li, C., Wang, J., Fang, Y., Sun, H., Tao, X., et al. (2017). Nafamostat mesilate improves neurological outcome and axonal regeneration after stroke in rats. Mol. Neurobiol. 54, 4217–4231. doi: 10.1007/s12035-016-9999-7
Liu, X., Ou, S., Yin, M., Xu, T., Wang, T., Liu, Y., et al. (2017). N-methyl-D-aspartate receptors mediate epilepsy-induced axonal impairment and tau phosphorylation via activating glycogen synthase kinase-3β and cyclin-dependent kinase 5. Discov. Med. 23, 221–234.
Liu, R., Tian, B., Gearing, M., Hunter, S., Ye, K., and Mao, Z. (2008). Cdk5-mediated regulation of the PIKE-A-Akt pathway and glioblastoma cell invasion. Proc. Natl. Acad. Sci. U S A 105, 7570–7575. doi: 10.1073/pnas.0712306105
Liu, X. X., Yang, L., Shao, L. X., He, Y., Wu, G., Bao, Y. H., et al. (2020). Endothelial Cdk5 deficit leads to the development of spontaneous epilepsy through CXCL1/CXCR2-mediated reactive astrogliosis. J. Exp. Med. 217:e20180992. doi: 10.1084/jem.20180992
Liu, W., Zhou, Y., Liang, R., and Zhang, Y. (2019). Inhibition of cyclin-dependent kinase 5 activity alleviates diabetes-related cognitive deficits. FASEB J. 33, 14506–14515. doi: 10.1096/fj.201901292R
Luo, F., Burke, K., Kantor, C., Miller, R. H., and Yang, Y. (2014). Cyclin-dependent kinase 5 mediates adult OPC maturation and myelin repair through modulation of Akt and GsK-3β signaling. J. Neurosci. 34, 10415–10429. doi: 10.1523/JNEUROSCI.0710-14.2014
Luo, F., Zhang, J., Burke, K., Miller, R. H., and Yang, Y. (2016). The activators of cyclin-dependent kinase 5 p35 and p39 are essential for oligodendrocyte maturation, process formation and myelination. J. Neurosci. 36, 3024–3037. doi: 10.1523/JNEUROSCI.2250-15.2016
Luo, F., Zhang, J., Burke, K., Romito-DiGiacomo, R. R., Miller, R. H., and Yang, Y. (2018). Oligodendrocyte-specific loss of Cdk5 disrupts the architecture of nodes of Ranvier as well as learning and memory. Exp. Neurol. 306, 92–104. doi: 10.1016/j.expneurol.2018.05.001
Manglani, K., and Dey, C. S. (2022). CDK5 inhibition improves glucose uptake in insulin-resistant neuronal cells via ERK1/2 pathway. Cell Biol. Int. 46, 488–497. doi: 10.1002/cbin.11735
Mawuenyega, K. G., Sigurdson, W., Ovod, V., Munsell, L., Kasten, T., Morris, J. C., et al. (2010). Decreased clearance of CNS β-amyloid in Alzheimer’s disease. Science 330:1774. doi: 10.1126/science.1197623
McColgan, P., and Tabrizi, S. J. (2018). Huntington’s disease: a clinical review. Eur. J. Neurol. 25, 24–34. doi: 10.1111/ene.13413
Mohamed, N. V., Desjardins, A., and Leclerc, N. (2017). Tau secretion is correlated to an increase of Golgi dynamics. PLoS One 12:e0178288. doi: 10.1371/journal.pone.0178288
Moutal, A., Luo, S., Largent-Milnes, T. M., Vanderah, T. W., and Khanna, R. (2019). Cdk5-mediated CRMP2 phosphorylation is necessary and sufficient for peripheral neuropathic pain. Neurobiol. Pain 5:100022. doi: 10.1016/j.ynpai.2018.07.003
Munoz-Manco, J. I., Gutierrez-Vargas, J. A., and Cardona-Gomez, G. P. (2018). [Neurogenesis and gliogenesis modulation in cerebral ischemia by CDK5 RNAi-based therapy]. Biomedica 38, 388–397. doi: 10.7705/biomedica.v38i4.3800
Nie, J., Zhang, Y., Ning, L., Yan, Z., Duan, L., Xi, H., et al. (2022). Phosphorylation of p53 by Cdk5 contributes to benzo[a]pyrene-induced neuronal apoptosis. Environ. Toxicol. 37, 17–27. doi: 10.1002/tox.23374
Nikhil, K., and Shah, K. (2017). The Cdk5-Mcl-1 axis promotes mitochondrial dysfunction and neurodegeneration in a model of Alzheimer’s disease. J. Cell Sci. 130, 3023–3039. doi: 10.1242/jcs.205666
Nikhil, K., Viccaro, K., and Shah, K. (2019). Multifaceted regulation of ALDH1A1 by Cdk5 in Alzheimer’s disease pathogenesis. Mol. Neurobiol. 56, 1366–1390. doi: 10.1007/s12035-018-1114-9
Ning, X., Tao, T., Shen, J., Ji, Y., Xie, L., Wang, H., et al. (2017). The O-GlcNAc modification of CDK5 involved in neuronal apoptosis following in vitro intracerebral hemorrhage. Cell. Mol. Neurobiol. 37, 527–536. doi: 10.1007/s10571-016-0391-y
Nishino, H., Saito, T., Wei, R., Takano, T., Tsutsumi, K., Taniguchi, M., et al. (2019). The LMTK1-TBC1D9B-Rab11A cascade regulates dendritic spine formation via endosome trafficking. J. Neurosci. 39, 9491–9502. doi: 10.1523/JNEUROSCI.3209-18.2019
Ouyang, L., Chen, Y., Wang, Y., Chen, Y., Fu, A. K. Y., Fu, W. Y., et al. (2020). p39-associated Cdk5 activity regulates dendritic morphogenesis. Sci. Rep. 10:18746. doi: 10.1038/s41598-020-75264-6
Palop, J. J., and Mucke, L. (2010). Amyloid-β-induced neuronal dysfunction in Alzheimer’s disease: from synapses toward neural networks. Nat. Neurosci. 13, 812–818. doi: 10.1038/nn.2583
Paoletti, P., Vila, I., Rife, M., Lizcano, J. M., Alberch, J., and Gines, S. (2008). Dopaminergic and glutamatergic signaling crosstalk in Huntington’s disease neurodegeneration: the role of p25/cyclin-dependent kinase 5. J. Neurosci. 28, 10090–10101. doi: 10.1523/JNEUROSCI.3237-08.2008
Pareek, T. K., Lam, E., Zheng, X., Askew, D., Kulkarni, A. B., Chance, M. R., et al. (2010). Cyclin-dependent kinase 5 activity is required for T cell activation and induction of experimental autoimmune encephalomyelitis. J. Exp. Med. 207, 2507–2519. doi: 10.1084/jem.20100876
Park, J., Seo, J., Won, J., Yeo, H. G., Ahn, Y. J., Kim, K., et al. (2019). Abnormal mitochondria in a non-human primate model of MPTP-induced Parkinson’s disease: Drp1 and CDK5/p25 signaling. Exp. Neurobiol. 28, 414–424. doi: 10.5607/en.2019.28.3.414
Park, J., Won, J., Seo, J., Yeo, H. G., Kim, K., Kim, Y. G., et al. (2020). Streptozotocin induces Alzheimer’s disease-like pathology in hippocampal neuronal cells via CDK5/Drp1-mediated mitochondrial fragmentation. Front. Cell. Neurosci. 14:235. doi: 10.3389/fncel.2020.00235
Peyressatre, M., Arama, D. P., Laure, A., Gonzalez-Vera, J. A., Pellerano, M., Masurier, N., et al. (2020a). Identification of quinazolinone analogs targeting CDK5 kinase activity and glioblastoma cell proliferation. Front. Chem. 8:691. doi: 10.3389/fchem.2020.00691
Peyressatre, M., Laure, A., Pellerano, M., Boukhaddaoui, H., Soussi, I., and Morris, M. C. (2020b). Fluorescent biosensor of CDK5 kinase activity in glioblastoma cell extracts and living cells. Biotechnol. J. 15:e1900474. doi: 10.1002/biot.201900474
Pozo, K., and Bibb, J. A. (2016). The emerging role of Cdk5 in cancer. Trends Cancer 2, 606–618. doi: 10.1016/j.trecan.2016.09.001
Qu, C., Li, Q. P., Su, Z. R., Ip, S. P., Yuan, Q. J., Xie, Y. L., et al. (2021). Nano-honokiol ameliorates the cognitive deficits in TgCRND8 mice of Alzheimer’s disease via inhibiting neuropathology and modulating gut microbiota. J. Adv. Res. 35, 231–243. doi: 10.1016/j.jare.2021.03.012
Quan, Q., Li, X., Feng, J., Hou, J., Li, M., and Zhang, B. (2020). Ginsenoside Rg1 reduces β-amyloid levels by inhibiting CDK5-induced PPARγ phosphorylation in a neuron model of Alzheimer’s disease. Mol. Med. Rep. 22, 3277–3288. doi: 10.3892/mmr.2020.11424
Quan, Q., Qian, Y., Li, X., and Li, M. (2019). CDK5 participates in amyloid-β production by regulating PPARγ phosphorylation in primary rat hippocampal Neurons. J. Alzheimers Dis. 71, 443–460. doi: 10.3233/JAD-190026
Rao, V. K., Swarnaseetha, A., Tham, G. H., Lin, W. Q., Han, B. B., Benoukraf, T., et al. (2020). Phosphorylation of Tet3 by cdk5 is critical for robust activation of BRN2 during neuronal differentiation. Nucleic Acids Res. 48, 1225–1238. doi: 10.1093/nar/gkz1144
Ren, Y., Zhou, X., Yang, J.-J., Liu, X., Zhao, X.-h., Wang, Q.-X., et al. (2015). AC1MMYR2 impairs high dose paclitaxel-induced tumor metastasis by targeting miR-21/CDK5 axis. Cancer Lett. 362, 174–182. doi: 10.1016/j.canlet.2015.03.038
Reudhabibadh, R., Binlateh, T., Chonpathompikunlert, P., Nonpanya, N., Prommeenate, P., Chanvorachote, P., et al. (2021). Suppressing Cdk5 activity by luteolin inhibits MPP+-induced apoptotic of neuroblastoma through Erk/Drp1 and Fak/Akt/GSK3β pathways. Molecules 26:1307. doi: 10.3390/molecules26051307
Ribeiro Filho, H. V., Guerra, J. V., Cagliari, R., Batista, F. A. H., Le Maire, A., Oliveira, P. S. L., et al. (2019). Exploring the mechanism of PPARgamma phosphorylation mediated by CDK5. J. Struct. Biol. 207, 317–326. doi: 10.1016/j.jsb.2019.07.007
Roach, B. L., Ngo, J. M., Limso, C., Oloja, K. B., and Bhandari, D. (2018). Identification and characterization of a novel phosphoregulatory site on cyclin-dependent kinase 5. Biochem. Biophys. Res. Commun. 504, 753–758. doi: 10.1016/j.bbrc.2018.09.017
Robin, G., Lopez, J. R., Espinal, G. M., Hulsizer, S., Hagerman, P. J., and Pessah, I. N. (2017). Calcium dysregulation and Cdk5-ATM pathway involved in a mouse model of fragile X-associated tremor/ataxia syndrome. Hum. Mol. Genet. 26, 2649–2666. doi: 10.1093/hmg/ddx148
Rong, R., Xia, X., Peng, H., Li, H., You, M., Liang, Z., et al. (2020). Cdk5-mediated Drp1 phosphorylation drives mitochondrial defects and neuronal apoptosis in radiation-induced optic neuropathy. Cell Death Dis. 11:720. doi: 10.1038/s41419-020-02922-y
Roufayel, R., and Murshid, N. (2019). CDK5: key regulator of apoptosis and cell survival. Biomedicines 7:88. doi: 10.3390/biomedicines7040088
Saito, T., Oba, T., Shimizu, S., Asada, A., Iijima, K. M., and Ando, K. (2019). Cdk5 increases MARK4 activity and augments pathological tau accumulation and toxicity through tau phosphorylation at Ser262. Hum. Mol. Genet. 28, 3062–3071. doi: 10.1093/hmg/ddz120
Sang, Y., Li, Y., Zhang, Y., Alvarez, A. A., Yu, B., Zhang, W., et al. (2019). CDK5-dependent phosphorylation and nuclear translocation of TRIM59 promotes macroH2A1 ubiquitination and tumorigenicity. Nat. Commun. 10:4013. doi: 10.1038/s41467-019-12001-2
Sase, A. S., Lombroso, S. I., Santhumayor, B. A., Wood, R. R., Lim, C. J., Neve, R. L., et al. (2019). Sex-specific regulation of fear memory by targeted epigenetic editing of Cdk5. Biol. Psychiatry 85, 623–634. doi: 10.1016/j.biopsych.2018.11.022
Seo, J., Kritskiy, O., Watson, L. A., Barker, S. J., Dey, D., Raja, W. K., et al. (2017). Inhibition of p25/Cdk5 attenuates tauopathy in mouse and iPSC models of frontotemporal dementia. J. Neurosci. 37, 9917–9924. doi: 10.1523/JNEUROSCI.0621-17.2017
Shah, K., and Lahiri, D. K. (2017). A Tale of the good and bad: remodeling of the microtubule network in the brain by Cdk5. Mol. Neurobiol. 54, 2255–2268. doi: 10.1007/s12035-016-9792-7
Shah, K., and Rossie, S. (2018). Tale of the good and the bad Cdk5: remodeling of the actin cytoskeleton in the brain. Mol. Neurobiol. 55, 3426–3438. doi: 10.1007/s12035-017-0525-3
Shen, X.-Y., Luo, T., Li, S., Ting, O.-Y., He, F., Xu, J., et al. (2018). Quercetin inhibits okadaic acid-induced tau protein hyperphosphorylation through the Ca2+-calpain-p25-CDK5 pathway in HT22 cells. Int. J. Mol. Med. 41, 1138–1146. doi: 10.3892/ijmm.2017.3281
Shi, C., Zeng, J., Li, Z., Chen, Q., Hang, W., Xia, L., et al. (2018). Melatonin mitigates kainic acid-induced neuronal tau hyperphosphorylation and memory deficits through alleviating ER stress. Front. Mol. Neurosci. 11:5. doi: 10.3389/fnmol.2018.00005
Shin, B. N., Kim, D. W., Kim, I. H., Park, J. H., Ahn, J. H., Kang, I. J., et al. (2019). Down-regulation of cyclin-dependent kinase 5 attenuates p53-dependent apoptosis of hippocampal CA1 pyramidal neurons following transient cerebral ischemia. Sci. Rep. 9:13032. doi: 10.1038/s41598-019-49623-x
Shinmyo, Y., Terashita, Y., Dinh Duong, T. A., Horiike, T., Kawasumi, M., Hosomichi, K., et al. (2017). Folding of the cerebral cortex requires Cdk5 in upper-layer neurons in gyrencephalic mammals. Cell Rep. 20, 2131–2143. doi: 10.1016/j.celrep.2017.08.024
Shukla, A. K., Spurrier, J., Kuzina, I., and Giniger, E. (2019). Hyperactive innate immunity causes degeneration of dopamine neurons upon altering activity of Cdk5. Cell Rep. 26, 131–144.e4. doi: 10.1016/j.celrep.2018.12.025
Shukla, R., and Singh, T. R. (2022). Identification of small molecules against cyclin dependent kinase-5 using chemoinformatics approach for Alzheimer’s disease and other tauopathies. J. Biomol. Struct. Dyn. 40, 2815–2827. doi: 10.1080/07391102.2020.1844050
Shukla, R., and Singh, T. R. (2020). Virtual screening, pharmacokinetics, molecular dynamics and binding free energy analysis for small natural molecules against cyclin-dependent kinase 5 for Alzheimer’s disease. J. Biomol. Struct. Dyn. 38, 248–262. doi: 10.1080/07391102.2019.1571947
Shukla, V., Seo, J., Binukumar, B. K., Amin, N. D., Reddy, P., Grant, P., et al. (2017). TFP5, a Peptide inhibitor of aberrant and hyperactive Cdk5/p25, attenuates pathological phenotypes and restores synaptic function in CK-p25Tg mice. J. Alzheimers Dis. 56, 335–349. doi: 10.3233/JAD-160916
Sorensen, H. T. (2019). Global burden of neurological disorders: challenges and opportunities with the available data. Lancet Neurol. 18, 420–421. doi: 10.1016/S1474-4422(19)30027-4
Spurrier, J., Shukla, A. K., McLinden, K., Johnson, K., and Giniger, E. (2018). Altered expression of the Cdk5 activator-like protein, Cdk5α, causes neurodegeneration, in part by accelerating the rate of aging. Dis. Model Mech. 11:dmm031161. doi: 10.1242/dmm.031161
Stupp, R., Mayer, M., Kann, R., Weder, W., Zouhair, A., Betticher, D. C., et al. (2009). Neoadjuvant chemotherapy and radiotherapy followed by surgery in selected patients with stage IIIB non-small-cell lung cancer: a multicentre phase II trial. Lancet Oncol. 10, 785–793. doi: 10.1016/S1470-2045(09)70172-X
Sulak, M. A., Ghosh, M., Sinharoy, P., Andrei, S. R., and Damron, D. S. (2018). Modulation of TRPA1 channel activity by Cdk5 in sensory neurons. Channels (Austin) 12, 65–75. doi: 10.1080/19336950.2018.1424282
Sun, H., Cai, J., Shen, S., and Ren, X. (2019). Hypothermia treatment ameliorated cyclin-dependent kinase 5-mediated inflammation in ischemic stroke and improved outcomes in ischemic stroke patients. Clinics (Sao Paulo) 74:e938. doi: 10.6061/clinics/2019/e938
Tabouret, E., Wang, H., Amin, N., Jung, J., Appay, R., Cui, J., et al. (2020). TP5, a peptide inhibitor of aberrant and hyperactive CDK5/p25: a novel therapeutic approach against glioblastoma. Cancers (Basel) 12:1935. doi: 10.3390/cancers12071935
Takahashi, M., Nakabayashi, T., Mita, N., Jin, X., Aikawa, Y., Sasamoto, K., et al. (2022a). Involvement of Cdk5 activating subunit p35 in synaptic plasticity in excitatory and inhibitory neurons. Mol. Brain 15:37. doi: 10.1186/s13041-022-00922-x
Takahashi, M., Takasugi, T., Kawakami, A., Wei, R., Ando, K., Ohshima, T., et al. (2022b). Valproic acid-induced anxiety and depression behaviors are ameliorated in p39 Cdk5 activator-deficient mice. Neurochem. Res. . [Online ahead of print]. doi: 10.1007/s11064-022-03642-9
Tanaka, T., Ohashi, S., Takashima, A., and Kobayashi, S. (2022). Dendritic distribution of CDK5 mRNA and p35 mRNA and a glutamate-responsive increase of CDK5/p25 complex contribute to tau hyperphosphorylation. Biochim. Biophys. Acta Gen. Subj. 1866:130135. doi: 10.1016/j.bbagen.2022.130135
Tejero, R., Balk, S., Franco-Espin, J., Ojeda, J., Hennlein, L., Drexl, H., et al. (2020). R-roscovitine improves motoneuron function in mouse models for spinal muscular atrophy. iScience. 23:100826. doi: 10.1016/j.isci.2020.100826
Thijs, R. D., Surges, R., O’Brien, T. J., and Sander, J. W. (2019). Epilepsy in adults. Lancet 393, 689–701. doi: 10.1016/S0140-6736(18)32596-0
Tian, F. F., Guo, T. H., and Chen, J. M. (2008). [Hippocampal mossy fiber sprouting and Cdk5/p35 expressions in the pentylenetetrazole kindling rat model]. Zhong Nan Da Xue Xue Bao Yi Xue Ban. 33, 1101–1107.
Tian, F.-F., Zeng, C., Ma, Y. F., Guo, T.-H., Chen, J. M., Chen, Y., et al. (2010). Potential roles of Cdk5/p35 and tau protein in hippocampal mossy fiber sprouting in the PTZ kindling model. Clin. Lab. 56, 127–136.
Tolonen, H., Dobson, A., Kulathinal, S., and Project, W. M. (2005). Effect on trend estimates of the difference between survey respondents and non-respondents: results from 27 populations in the WHO MONICA Project. Eur. J. Epidemiol. 20, 887–898. doi: 10.1007/s10654-005-2672-5
Tuo, Q. Z., Liuyang, Z. Y., Lei, P., Yan, X., Shentu, Y. P., Liang, J. W., et al. (2018). Zinc induces CDK5 activation and neuronal death through CDK5-Tyr15 phosphorylation in ischemic stroke. Cell Death Dis. 9:870. doi: 10.1038/s41419-018-0929-7
Umfress, A., Singh, S., Ryan, K. J., Chakraborti, A., Plattner, F., Sonawane, Y., et al. (2022). Systemic administration of a brain permeable Cdk5 inhibitor alters neurobehavior. Front. Pharmacol. 13:863762. doi: 10.3389/fphar.2022.863762
Wang, J., Huang, Y., Cai, J., Ke, Q., Xiao, J., Huang, W., et al. (2018). A nestin-cyclin-dependent kinase 5-dynamin-related protein 1 axis regulates neural stem/progenitor cell stemness via a metabolic shift. Stem Cells 36, 589–601. doi: 10.1002/stem.2769
Wang, Q., Jiao, F., Zhang, P., Yan, J., Zhang, Z., He, F., et al. (2018). CDK5-mediated phosphorylation-dependent ubiquitination and degradation of e3 ubiquitin ligases GP78 accelerates neuronal death in Parkinson’s disease. Mol. Neurobiol. 55, 3709–3717. doi: 10.1007/s12035-017-0579-2
Wei, J., Marangoni, R. G., Fang, F., Wang, W., Huang, J. J., Distler, H. W., et al. (2017). The non-neuronal cyclin-dependent kinase 5 is a fibrotic mediator potentially implicated in systemic sclerosis and a novel therapeutic target. Oncotarget 9, 10294–10306. doi: 10.18632/oncotarget.23516
Wilkaniec, A., Gassowska-Dobrowolska, M., Strawski, M., Adamczyk, A., and Czapski, G. A. (2018). Inhibition of cyclin-dependent kinase 5 affects early neuroinflammatory signalling in murine model of amyloid beta toxicity. J. Neuroinflammation 15:1. doi: 10.1186/s12974-017-1027-y
Wilson, D., Adams, M. E., Robertson, F., Murphy, M., and Werring, D. J. (2015). Investigating intracerebral haemorrhage. BMJ 350:h2484. doi: 10.1136/bmj.h2484
Wu, J., Zhang, X., Yan, Y., Tang, Z., Sun, X., Huo, G., et al. (2016). The crucial role of cyclin-dependent kinase-5-ataxia-telangiectasia mutated axis in ICH-induced neuronal injury of rat model. Mol. Neurobiol. 53, 6301–6308. doi: 10.1007/s12035-015-9524-4
Xia, X., Zhou, C., Sun, X., He, X., Liu, C., and Wang, G. (2020). Estrogen improved the regeneration of axons after subcortical axon injury via regulation of PI3K/Akt/CDK5/Tau pathway. Brain Behav. 10:e01777. doi: 10.1002/brb3.1777
Xie, Q., Wu, Q., Horbinski, C. M., Flavahan, W. A., Yang, K., Zhou, W., et al. (2015). Mitochondrial control by DRP1 in brain tumor initiating cells. Nat. Neurosci. 18, 501–510. doi: 10.1038/nn.3960
Xu, M., Huang, Y., Song, P., Huang, Y., Huang, W., Zhang, H. T., et al. (2019). AAV9-mediated Cdk5 inhibitory peptide reduces hyperphosphorylated tau and inflammation and ameliorates behavioral changes caused by overexpression of p25 in the brain. J. Alzheimers Dis. 70, 573–585. doi: 10.3233/JAD-190099
Xu, B., Kumazawa, A., Kobayashi, S., Hisanaga, S. I., Inoue, T., and Ohshima, T. (2017). Cdk5 activity is required for Purkinje cell dendritic growth in cell-autonomous and non-cell-autonomous manners. Dev. Neurobiol. 77, 1175–1187. doi: 10.1002/dneu.22507
Yan, J., Zhang, P., Tan, J., Li, M., Xu, X., Shao, X., et al. (2022). Cdk5 phosphorylation-induced SIRT2 nuclear translocation promotes the death of dopaminergic neurons in Parkinson’s disease. NPJ Parkinsons Dis. 8:46. doi: 10.1038/s41531-022-00311-0
Yang, L., Gu, X., Zhang, W., Zhang, J., and Ma, Z. (2014). Cdk5 inhibitor roscovitine alleviates neuropathic pain in the dorsal root ganglia by downregulating N-methyl-D-aspartate receptor subunit 2A. Neurol. Sci. 35, 1365–1371. doi: 10.1007/s10072-014-1713-9
Yuen, A. W. C., Keezer, M. R., and Sander, J. W. (2018). Epilepsy is a neurological and a systemic disorder. Epilepsy Behav. 78, 57–61. doi: 10.1016/j.yebeh.2017.10.010
Zeb, A., Kim, D., Alam, S. I., Son, M., Kumar, R., Rampogu, S., et al. (2019a). Computational simulations identify pyrrolidine-2,3-dione derivatives as novel inhibitors of Cdk5/p25 complex to attenuate Alzheimer’s pathology. J. Clin. Med. 8:746. doi: 10.3390/jcm8050746
Zeb, A., Son, M., Yoon, S., Kim, J. H., Park, S. J., and Lee, K. W. (2019b). Computational simulations identified two candidate inhibitors of Cdk5/p25 to abrogate tau-associated neurological disorders. Comput. Struct. Biotechnol. J. 17, 579–590. doi: 10.1016/j.csbj.2019.04.010
Zeng, L., Jiang, H., Ashraf, G. M., Liu, J., Wang, L., Zhao, K., et al. (2021). Implications of miR-148a-3p/p35/PTEN signaling in tau hyperphosphorylation and autoregulatory feedforward of Akt/CREB in Alzheimer’s disease. Mol. Ther. Nucleic Acids 27, 256–275. doi: 10.1016/j.omtn.2021.11.019
Zhang, R., Liu, C., Ji, Y., Teng, L., and Guo, Y. (2018). Neuregulin-1β plays a neuroprotective role by inhibiting the Cdk5 signaling pathway after cerebral ischemia-reperfusion injury in rats. J. Mol. Neurosci. 66, 261–272. doi: 10.1007/s12031-018-1166-3
Zhang, J., Zhang, Y., Xu, M., Miao, Z., and Tian, Y. (2021). Inhibition of the CDK5/caspase-3 pathway by p5-TAT protects hippocampal neurogenesis and alleviates radiation-induced cognitive dysfunction. Neuroscience 463, 204–215. doi: 10.1016/j.neuroscience.2021.03.034
Zhao, W., Yan, J., Gao, L., Zhao, J., Zhao, C., Gao, C., et al. (2017). Cdk5 is required for the neuroprotective effect of transforming growth factor-β1 against cerebral ischemia-reperfusion. Biochem. Biophys. Res. Commun. 485, 775–781. doi: 10.1016/j.bbrc.2017.02.130
Zhao, D., Zhou, Y., Huo, Y., Meng, J., Xiao, X., Han, L., et al. (2021). RPS23RG1 modulates tau phosphorylation and axon outgrowth through regulating p35 proteasomal degradation. Cell Death Differ. 28, 337–348. doi: 10.1038/s41418-020-00620-y
Zhong, Y., Chen, J., Chen, J., Chen, Y., Li, L., and Xie, Y. (2019). Crosstalk between Cdk5/p35 and ERK1/2 signalling mediates spinal astrocyte activity via the PPARgamma pathway in a rat model of chronic constriction injury. J. Neurochem. 151, 166–184. doi: 10.1111/jnc.14827
Zhou, T., Wang, H., Shen, J., Li, W., Cao, M., Hong, Y., et al. (2019). The p35/CDK5 signaling is regulated by p75NTR in neuronal apoptosis after intracerebral hemorrhage. J. Cell. Physiol. . [Online ahead of print]. doi: 10.1002/jcp.28244
Zhou, Y., Wang, X., Lv, P., Yu, H., and Jiang, X. (2021). CDK5 knockdown inhibits proliferation and induces apoptosis and cell cycle arrest in human glioblastoma. J. Cancer 12, 3958–3966. doi: 10.7150/jca.53981
Zhou, H., Zhang, J., Shi, H., Li, P., Sui, X., Wang, Y., et al. (2022). Downregulation of CDK5 signaling in the dorsal striatum alters striatal microcircuits implicating the association of pathologies with circadian behavior in mice. Mol. Brain 15:53. doi: 10.1186/s13041-022-00939-2
Zhu, L.-Q., Lu, Y., Yin, X., Liu, D., Chen, J. G., Wei, N., et al. (2019). The peptide-directed lysosomal degradation of CDK5 exerts therapeutic effects against stroke. Aging Dis. 10, 1140–1145. doi: 10.14336/AD.2018.1225
Zhu, X., Yue, L., Fan, C., Liu, Y., Wang, Y., and Zhao, H. (2021). Mechanism of Cdk5-synaptophysin-SNARE pathway in acute and chronic inflammatory pain. Am. J. Transl. Res. 13, 1075–1084.
Zhuang, J., Cai, P., Chen, Z., Yang, Q., Chen, X., Wang, X., et al. (2020a). Long noncoding RNA MALAT1 and its target microRNA-125b are potential biomarkers for Alzheimer’s disease management via interactions with FOXQ1, PTGS2 and CDK5. Am. J. Transl. Res. 12, 5940–5954.
Zhuang, J., Chen, Z., Cai, P., Wang, R., Yang, Q., Li, L., et al. (2020b). Targeting microRNA-125b promotes neurite outgrowth but represses cell apoptosis and inflammation via blocking PTGS2 and CDK5 in a FOXQ1-dependent way in Alzheimer disease. Front. Cell Neurosci. 14:587747. doi: 10.3389/fncel.2020.587747
Keywords: cyclin-dependent kinases 5 (Cdk5), neurological disorders, therapeutic targets, CDK5 inhibitor, nervous system
Citation: Ao C, Li C, Chen J, Tan J and Zeng L (2022) The role of Cdk5 in neurological disorders. Front. Cell. Neurosci. 16:951202. doi: 10.3389/fncel.2022.951202
Received: 28 May 2022; Accepted: 29 June 2022;
Published: 28 July 2022.
Edited by:
Haitao Wang, Southern Medical University, ChinaCopyright © 2022 Ao, Li, Chen, Tan, and Zeng. This is an open-access article distributed under the terms of the Creative Commons Attribution License (CC BY). The use, distribution or reproduction in other forums is permitted, provided the original author(s) and the copyright owner(s) are credited and that the original publication in this journal is cited, in accordance with accepted academic practice. No use, distribution or reproduction is permitted which does not comply with these terms.
*Correspondence: Liuwang Zeng, emVuZ2xpdXdhbmdAY3N1LmVkdS5jbg==
Disclaimer: All claims expressed in this article are solely those of the authors and do not necessarily represent those of their affiliated organizations, or those of the publisher, the editors and the reviewers. Any product that may be evaluated in this article or claim that may be made by its manufacturer is not guaranteed or endorsed by the publisher.
Research integrity at Frontiers
Learn more about the work of our research integrity team to safeguard the quality of each article we publish.