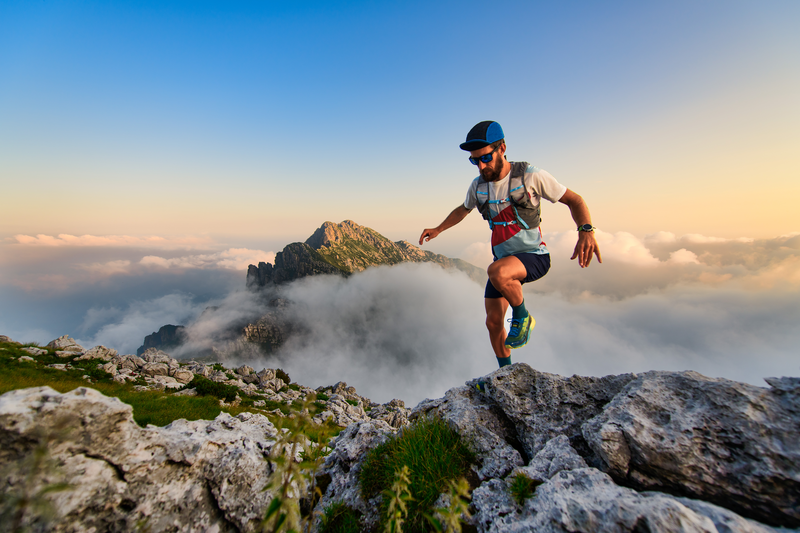
94% of researchers rate our articles as excellent or good
Learn more about the work of our research integrity team to safeguard the quality of each article we publish.
Find out more
REVIEW article
Front. Cell. Neurosci. , 18 May 2022
Sec. Cellular Neuropathology
Volume 16 - 2022 | https://doi.org/10.3389/fncel.2022.839396
This article is part of the Research Topic Hot Topics in Cellular Neuropathology View all 5 articles
Suicide is a complex public health challenge associated worldwide with one death every 40 s. Research advances in the neuropathology of suicidal behaviors (SB) have defined discrete brain changes which may hold the key to suicide prevention. Physiological differences in microglia, the resident immune cells of the brain, are present in post-mortem tissue samples of individuals who died by suicide. Furthermore, microglia are mechanistically implicated in the outcomes of important risk factors for SB, including early-life adversity, stressful life events, and psychiatric disorders. SB risk factors result in inflammatory and oxidative stress activities which could converge to microglial synaptic remodeling affecting susceptibility or resistance to SB. To push further this perspective, in this Review we summarize current areas of opportunity that could untangle the functional participation of microglia in the context of suicide. Our discussion centers around microglial state diversity in respect to morphology, gene and protein expression, as well as function, depending on various factors, namely brain region, age, and sex.
Suicide is a complex behavior resulting from intricate, multi-dimensional interactions between various social, cultural, biological, psychological, and environmental factors (Turecki et al., 2019). Worldwide, suicide is the second and third leading cause of premature death in individuals between 15–29 and 15–44 years of age, respectively (Bachmann, 2018). While the prevalence may vary according to the country, suicide deaths are more common in males, whereas suicide attempts are more frequent in females (Bachmann, 2018). Elevated suicide rates in males could be connected to a preferential use of more lethal methods compared to females, in addition to important differences in socialization between sexes (Tsirigotis et al., 2011). For instance, traditional masculine role norms include self-reliance which may negatively impact their capacity to seek help (Seidler et al., 2021). Beyond age and sex, another crucial risk factor for suicide is the presence of psychiatric disorders such as MDD and SCZ (see Table 1 for full definitions of abbreviations), which are associated with at least 43.2 and 9.2% of suicide deaths, respectively, in North America (Arsenault-Lapierre et al., 2004). Although the categorization of SB is subject to debate (Goodfellow et al., 2019), in this Review we use this term to refer to suicide, suicide attempts and preparatory acts (Nock, 2014), while suicidal ideation is used to specify active or passive thoughts about ending one’s own life (Turecki et al., 2019).
In the hope of identifying novel therapeutic targets to prevent SB, research has looked for changes in the CNS that could hint at the biological alterations responsible for SB and their risk factors. Studies of human brain samples from individuals who died by suicide have revealed direct changes in microglial numbers, morphology, as well as gene and protein expression (see Supplementary Table 1), although their mechanisms of action remain unknown (Suzuki et al., 2019; Baharikhoob and Kolla, 2020). Microglia are the resident immune cells of the CNS (Šimončičová et al., 2022). They perform classic immune roles involving debris clearance (Lampron et al., 2015), phagocytosis of infected or apoptotic cells (Sierra et al., 2010; Györffy et al., 2018) and production of immune mediators (Aloisi, 2001). Their function, however, goes beyond immunity (Šimončičová et al., 2022). Notably, microglia contribute to the development, maintenance, and plasticity of the CNS. These cells modulate the survival of newborn neurons and stimulate the formation of dendritic spines via neurotrophic support (Sierra et al., 2010; Parkhurst et al., 2013). In addition to contributing to synapse formation (Miyamoto et al., 2016), microglia participate in synaptic remodeling through the stripping of pre- and post-synaptic elements (Trapp et al., 2007), pruning of synapses via partial (Weinhard et al., 2018) or full phagocytosis (Linnartz et al., 2012; Schafer et al., 2012; Györffy et al., 2018), and remodeling of the extracellular matrix (Nguyen et al., 2020). Furthermore, microglia assist astrocytic cellular maturation and responses to immune insults (Vainchtein and Molofsky, 2020). Similarly, microglia are needed for the maturation of oligodendrocyte progenitors (Hagemeyer et al., 2017), myelination of axons (Hughes and Appel, 2020), vascular formation and remodeling, as well as homeostatic BBB permeability regulation (Joost et al., 2019). By fine-tuning CNS connectivity and cellular signaling, microglia influence behaviors involved in learning, memory, and sociability both in health and disease (Tay et al., 2018; Bordeleau et al., 2019; Tremblay, 2021).
As microglia are intimately tuned to their microenvironment, the periphery and other environmental influences, these cells are likely responsive to the different SB risk factors (Turecki et al., 2014; Réus et al., 2015; Mondelli et al., 2017; Tay et al., 2018). Suicide risk factors add up across the lifespan and can be categorized according to their temporal distance to the SB’s onset (Turecki et al., 2019). They include distal factors such as ELA and genetic history; developmental factors linked to personality traits or cognitive deficits; and proximal factors comprising stressful life events and psychiatric disorders, such as MDD or SCZ (Turecki et al., 2019). Each one of these factors is further dependent on the individuals’ societal, demographic and economic circumstances (Bachmann, 2018; Turecki et al., 2019). Notably, the biological consequences of microglial responses to SB risk factors are as diverse and complex as suicide etiology itself (Underwood and Arango, 2011). Hence, in this Review, we focus on putative microglial pathways that can help explain why distal and proximal stress may translate into SB risk, taking into account the previous findings and the context under investigation, such as age, sex, and CNS region. In particular, we outline the outcomes connected to inflammation, oxidative stress and trophic balance (see Figure 1), increasingly associated with MDD, SCZ and SB risk (Suzuki et al., 2019; Baharikhoob and Kolla, 2020). We hope our considerations can open venues of future investigation that will uncover targets able to support suicide prevention.
Figure 1. Microglia participate in pathways altered upon suicidal behaviors. (1) As a result of stress-susceptibility, inflammation in the periphery (a) can contribute to blood-brain barrier (BBB) disruption (b) and infiltration of peripheral cells or border-associated macrophages (BAM) (c) to the central nervous system (CNS) parenchyma. Stress-induced inflammatory (d) and oxidative stress (e) molecules, such as cytokines, reactive oxygen species or adenosine triphosphate, work in a positive feedback loop that could affect microglial synaptic plasticity regulation (f), and increase suicidal behaviors (SB) risk. (2) SB risk factors, including distal early-life adversity (ELA) and proximal major depressive disorder (MDD), are associated with microglial priming, in which elevated expression of genes related to phagocytosis, cellular proliferation, and vesicular release result in exacerbated inflammatory responses upon exposure to subsequent challenges, as well as to impaired synaptic development and function. (3) The regional diversity of microglia possibly participates in determining susceptibility to inflammation induced by SB risk factors. Region-specific differences in gene or protein expression of ionized calcium-binding adapter molecule 1 (IBA1), major histocompatibility complex class II, DR (HLA-DR), CX3C chemokine receptor 1 (CX3CR1), transmembrane protein 119 (TMEM119), as well as in density are observed in post-mortem CNS samples from individuals who died by suicide. (4) Similarly, distinct CNS areas likely have different oxidative stress responses to inflammation. Oxidative stress induced by inflammation is thought to affect mitochondrial and tryptophan metabolism, which may lead to an increased activity of microglial indoleamine 2,3-dioxygenase 1 (IDO), an enzyme that breaks tryptophan (TRP) down into kynurenine (KYN). Increased KYN to TRP ratio could decrease serotonin (SER) and gamma-aminobutyric acid (GABA) signaling, whilst elevating glutamate (GLU) excitotoxity in individuals with SB. Altogether, the multiple synaptic plasticity-related pathways implicate microglia in SB, warranting further investigation.
Stress is a physiological response to a variety of biological and psychological challenges (Lucassen et al., 2014). The physiological and cognitive outcomes of stress vary between susceptible and resistant individuals, influenced by their genetic, epigenetic, and behavioral characteristics, together with the duration and type of stressor (Russo et al., 2012; Dantzer et al., 2018). In vulnerable groups, stress is notably marked by unfavorable inflammatory responses (Ménard et al., 2017), which could help explain why distal or proximal stress increases SB risk (Suzuki et al., 2019; Baharikhoob and Kolla, 2020). Meta-analyses often find enhanced circulating protein levels of CRP and pro-inflammatory cytokines, such as IL6 and TNF, as well as reduced circulating protein levels of anti-inflammatory IL2 in individuals with SB (Serafini et al., 2020). Clinical studies indicate a relationship between enhanced plasma protein levels of IL6 and CRP with traits of aggression and impulsivity (Brodsky et al., 2001; Coccaro, 2006; Coccaro et al., 2014), frequently comorbid with psychiatric disorders and SB (Brundin et al., 2017). Moreover, IFNα therapy, regularly used to treat tumor malignancy and chronic viral hepatitis, is commonly associated with the development of SB (Brundin et al., 2017; Serafini et al., 2020). Despite the link between peripheral inflammation and SB (Figure 1), conflicting changes in brain expression of cytokines such as TNF, IL1β, IL6, and IL10 were reported across post-mortem studies of individuals who died by suicide (Supplementary Table 1; Torres-Platas et al., 2014b; Clark et al., 2016; Schiavone et al., 2016; Wang et al., 2018; Snijders et al., 2020). Furthermore, according to gene ontology analysis, the DLPFC, AMY, and TLM of individuals with MDD who died by suicide can present lower expression of gene sets related to microglial immune functions such as “chemokine receptor binding” and “cellular response to LPS” compared to age- and sex-matched individuals with MDD deceased from other causes or healthy controls (Pantazatos et al., 2017; Glavan et al., 2021). Such differences could be reconciled by the largely distinct brain regions studied, such as the VLPFC, ACC, and TLM, which likely present diverse baseline inter-individual immune activity, and therefore, a different susceptibility to peripheral inflammation (Hodes et al., 2014; Wood et al., 2015).
Although more studies are warranted, there is in vivo indication that increased microglial inflammatory activity is present in individuals with SB. TSPO is an outer mitochondrial membrane protein expressed by microglia, macrophages, astrocytes, endothelial and ependymal cells in the brain, which shows a marked upregulation during CNS inflammation, as detected by PET imaging (Nutma et al., 2019; Pannell et al., 2020). Enhanced TSPO binding is observed, notably among the PFC, ACC, and insula, in medication-free patients with MDD actively experiencing a moderate-to-severe major MDD episode (Setiawan et al., 2015). Similarly, significantly greater TSPO binding is found in the ACC and insula of patients with MDD experiencing suicidal ideation compared to patients without suicidal thoughts (Holmes et al., 2018; Supplementary Table 1). In addition to in vivo studies, increased mRNA Tspo expression was detected in the DLPFC but not ACC of individuals with MDD irrespective of the cause of death, compared to age and PMI-matched controls (Zhang L. et al., 2021). Moreover, elevated protein expression, but not mRNA levels (Zhang L. et al., 2021), of HLA-DR were measured in various post-mortem areas, including the ACC, MTN, DLPFC, and DPFWM (Steiner et al., 2006, 2008; Torres-Platas et al., 2014b). HLA-DR is expressed by microglia, peripheral macrophages and BAM in the CNS (Steiner et al., 2006, 2008; Swanson et al., 2020; Prinz et al., 2021). In the periphery, HLA-DR is associated with antigen-presentation, however, within the CNS, although frequently upregulated in pathological contexts, its function remains unknown (Wolf et al., 2018). HLA-DR can be upregulated along CCL2 protein expression in white matter of male and female individuals with multiple sclerosis lesions (Peferoen et al., 2015). Therefore, a direct association of HLA-DR and pro-inflammatory microglial activity could be misleading (Walker and Lue, 2015). Prospective studies are encouraged to better characterize the immune response of microglia upon SB. Notably, the possible outcomes of the inflammatory activity of microglia are diverse and far-reaching in the CNS, as we outline next.
Bidirectional interactions between the peripheral immune system and microglia are facilitated by cytokines crossing the BBB, primarily composed of endothelial cells, sealed together by tight junctions, covered by pericytes, astrocytic endfeet, and microglial processes (Bisht et al., 2016; Joost et al., 2019). A disrupted BBB can increase the influx of inflammatory molecules and cells to the CNS (Figure 1). Studies looking at CSF or serum concentrations of proteins typically found in the CNS, for instance, CSF hyaluronic acid (Ventorp et al., 2016) and albumin CSF/serum ratio (Bayard-Burfield et al., 1996), revealed that the BBB is compromised in individuals with SB and suicide ideation (Falcone et al., 2010; Torres-Platas et al., 2014b). Suggestive of decreased BBB integrity, mRNA downregulation of the tight junction protein Cldn5 is present in the NA of individuals with MDD who died by suicide (Menard et al., 2017; Dudek et al., 2020). Compared to resistant and healthy controls, male mice susceptible to CSDS exhibited increased cortical leakage of intravenously injected dyes (Lehmann et al., 2018). Microglia isolated from the susceptible mice selectively expressed transcripts involved with extracellular matrix breakdown, inflammatory response, cytokine production, ROS and metabolic processes, which highly correlated with the transcriptomic changes associated with aging (Lehmann et al., 2018). Microglial stress susceptibility could be connected to the BBB disruption, requiring future investigation, particularly in older individuals, where this effect might be exacerbated and contribute to increasing SB risk. Indeed, robust data from animal models supports that aging is linked to microglial pro-inflammatory and oxidative stress activities, reduction in CNS surveillance, as well as appearance of microglial states, such as primed microglia (see section “Microglial Priming Is a Hallmark of Early-Life Adversity and May Contribute to Stress Susceptibility”), connected to synaptic dysfunction (Tay et al., 2018; Stratoulias et al., 2019). Correspondingly, although SB, in general, are more common at younger ages, suicide deaths rates are higher in older individuals in almost all countries (Conejero et al., 2018).
Alternatively, microglial resistance to stress could be associated with the protection of the BBB. Microglia were suggested to infiltrate the BBB toward the periphery and rescue its permeability via CLDN5 protein expression early on during systemic inflammation caused by daily LPS injections in adult male mice (Haruwaka et al., 2019). This protective role, however, changed at later time points, whereby microglia expressed higher levels of CD68 protein, a phagolysosomal activity marker present in human microglia/macrophages and associated with inflammation (Walker and Lue, 2015; Haruwaka et al., 2019). Elevated CD68 occurred along with an increased number of microglial inclusions of aquaporin 4, notably expressed in blood vessels and astrocytic endfeet, suggesting their phagocytosis (Haruwaka et al., 2019). Accordingly, CSDS in adult male mice increases the number of brain CD68-high cells, as revealed by flow cytometry (Lehmann et al., 2016). No significant differences in mRNA Cd68 expression and CD68 immunostaining, however, were observed in the dACC and DPFWM or VPFWM, respectively, of individuals who died by suicide compared to PMI- (Torres-Platas et al., 2014b), sex-, psychiatric diagnosis- (Schnieder et al., 2014), and age-matched controls (Supplementary Table 1). Contrastingly, increased mRNA Cd68 expression was detected in the DLPFC but not ACC of individuals with MDD who died by suicide, compared to age and PMI-matched controls (Zhang L. et al., 2021). Despite strong associations between stress, inflammation and phagocytosis, prospective studies are needed to understand if microglia play a detrimental or beneficial role in BBB integrity specifically in the context of SB.
Additional pathways mediated by stress susceptibility and increased inflammation can result in an impaired BBB permeability and elevated SB risk. Conditional knockdown of CLDN5 in NA blood vessels was enough to induce MDD-like behaviors and increase BBB permeability in CSDS-susceptible adult male mice (Menard et al., 2017). Moreover, it caused significant protein increase of IL6 NA levels, albeit not changing the density of NA CX3CR1-positive cells, largely microglia, but possibly representing peripheral macrophages and BAMs (Lehmann et al., 2016; Menard et al., 2017; Prinz et al., 2021). In a follow-up study, epigenetic regulation of CLDN5, detected also in samples of individuals with MDD who died by suicide, as well as changes in TNF/NFκB signaling, were connected to the observed susceptibility and resilience to stress in adult male mice (Dudek et al., 2020). Correspondingly, the NFκB inhibitor alpha was among the top ten genes implicated in the DLPFC individuals with MDD who died by suicide, compared to individuals with MDD who died of other causes and healthy controls (Zeng et al., 2020; Supplementary Table 1). Future studies are warranted to evaluate if and how microglia-dependent and -independent mechanisms of BBB disruption increase SB risk after stress.
BBB permeability appears to be similarly compromised by lifestyle factors, for instance, sleep. Chronic sleep restriction in adult male mice decreased mRNA expression of tight junction proteins, such as Cldn5, and increased cortical and subcortical uptake of intravenously injected dies, indicating lower BBB integrity (He et al., 2014). Circadian rhythms, which notably control sleep-wake patterns and are frequently disturbed in psychiatric disorders (Wulff et al., 2010), play a role in BBB regulation as well. According to studies in flies and mice, as well as in human in vitro models, BBB efflux mechanisms are stronger during the active or awake phases (Zhang et al., 2018; Zhang S. L. et al., 2021). Therefore, the BBB is not only physically restrictive, but also temporally restrictive (Cuddapah et al., 2019), indicating additional factors that can have a modulatory effect on the connection between peripheral and central immune systems. A multi-dimensional interaction between sleep, circadian rhythms, microglia and synaptic plasticity is suggested in the literature (Picard et al., 2021). Evidence from adult male rats support that circadian rhythms control the removal of synapses through complement opsonization (Choudhury et al., 2020). A meta-analysis looking at 42 studies published between 1982 and 2019 found a statistically significant but weak influence of sleep disturbances on SB (Harris et al., 2020). Despite the small effect, probably arising from inter-individual variability, lifestyle factors such as sleep are important elements to consider in the complex synergy of microglial features that convey susceptibility to psychiatric disorders (Picard et al., 2021) and SB (Glavan et al., 2021).
The CNS is populated by resident immune cells, microglia, but can be infiltrated by peripheral immune populations and BAM, such as macrophages from the choroid plexus, meninges and perivascular space (Prinz et al., 2021). Previous research in male adult mice has illustrated how infiltrating neutrophils are linked to inflammatory processes and MDD-like behavior (Aguilar-Valles et al., 2014), whereas monocytes in the CNS display signaling related to resolution and repair, as well as anxiety-like behavior (McKim et al., 2018; Liu et al., 2019). Studies on male mice exposed to CSDS found an increased recruitment of peripheral monocytes into the CNS in association with heightened anxiety- and MDD-like behaviors (Brevet et al., 2010; Wohleb et al., 2011, 2013, 2016; Weber et al., 2019; Yin et al., 2019; Picard et al., 2021). It is suggested, however, that infiltration results from wounding during CSDS. Additionally, in the study by Menard et al. (2017) CSDS caused an accumulation of peripheral CCR2-positive monocytes within blood vessels of the NA, without parenchymal infiltration.
Microglia are important for the recruitment of peripheral cells to the CNS. CSF1R inhibition, which reduces microglial numbers, prevented monocyte recruitment to the brain of adult male mice exposed to CSDS and abrogated anxiety-like symptoms, in a signaling cascade requiring microglial CCL2 and monocyte IL1β protein expression (McKim et al., 2018; Weber et al., 2019). According to studies in adult male mice, IL1R1, the IL1β receptor, is needed for monocyte recruitment to the ependymal and ventricular walls and for neutrophil infiltration through endothelial cells (Liu et al., 2019). IL1R1 expression in both routes of infiltration was linked to adaptations of microglia morphology (e.g., increased soma size in the DG) and protein expression (e.g., upregulated CD45) (Liu et al., 2019). By contrast, no difference in Il1r1 mRNA expression was found in the dACC of individuals with MDD who died by suicide compared to age- and PMI-matched healthy controls (Torres-Platas et al., 2014b). While it remains unclear if similar IL1R1 mechanisms are active in SB, overall, these results indicate that the infiltration of immune cell populations likely results in a functional crosstalk with microglia that could be further investigated in the context of stress susceptibility and SB risk (Figure 1).
Despite the link between stress and CNS immune infiltration, previous post-mortem studies provide conflicting data on the presence of IBA1-positive cells associated with/within vessels, or on CD163-positive cell infiltration in the ACC, DPFWM and VPFWM of individuals who died by suicide compared to PMI- (Torres-Platas et al., 2014b), sex-, psychiatric diagnosis- (Schnieder et al., 2014, 2019), and age-matched controls. CD163 is a marker related to phagocytosis that is expressed by both human microglia and perivascular macrophages, although mainly in the latter (Swanson et al., 2020). CD163 protein expression was decreased in CD11b-positive microglia isolated from individuals with MDD compared to healthy controls, although without a specific effect on the one suicide death included in the study (Snijders et al., 2020). Moreover, elevated mRNA expression of Ccl2, alias Mcp-1, a chemokine involved in the recruitment of peripheral cells, and Cd45 mRNA, usually enriched in peripheral macrophage populations, was found in the dACC of individuals with MDD who died by suicide compared to age- and PMI-matched healthy controls (Torres-Platas et al., 2014b). Contrastingly, it is important to consider that microglial populations can express high levels of CD45 protein in male mouse models of stroke and aging (Honarpisheh et al., 2020). Furthermore, decreased Ccl2 transcripts were instead detected in the VLPFC of individuals with MDD compared to healthy controls, although lacking a suicide subgroup effect (Clark et al., 2016). Numerous factors could underlie the variable peripheral immune cell recruitment in SB, such as CNS regions, sexes, age, as well as inter-individual differences in stress susceptibility and resistance of the cohorts studied (Lehmann et al., 2018). Additionally, elevated CD163 immunostaining in the DPFWM was associated with a suicide-specific effect on microglial migration to abluminal surfaces of vessels, instead of peripheral immune cell infiltration (Schnieder et al., 2014, 2019). Previously reported elevations in IBA1-positive cells adjacent to vessels in the DPFWM of individuals who died by suicide compared to age-, sex- and psychiatric diagnoses-matched controls were not paralleled by changes in CD163-positive densities, indicating they likely arose from resident microglial staining (Schnieder et al., 2014, 2019). Increased association of microglia with vessels was shown upon systemic inflammation caused by daily LPS injections in male mice (Haruwaka et al., 2019) and a similar phenomenon might be present in SB. The dynamic interaction between microglia and blood vessels should be accounted for in future research looking at peripheral immune CNS invasion in SB.
Microglia, perivascular macrophages and subdural leptomeningeal macrophages derive from yolk-sac erythromyeloid progenitors matured into myeloid precursor cells that seed the brain around E9.5 in mouse (Ginhoux et al., 2010; Kierdorf et al., 2013). Peripheral infiltrating monocytes or macrophages, by contrast, arise from erythromyeloid progenitors that travel to the fetal liver and later transition to the bone marrow starting around birth (Hoeffel et al., 2015; Stremmel et al., 2018). Similar ontogeny contributes to the large overlap in markers such as CX3CR1, CSF1R, CD11b, IBA1, HLA-DR, CD163, CD68, CD45 between microglia, BAM, and peripheral monocytes (Walker and Lue, 2015; Snijders et al., 2020; Swanson et al., 2020; Prinz et al., 2021). Region-specific analyses using largely microglia-specific markers such as TMEM119, P2RY12, SALL1, and HEXB (Prinz et al., 2021) are warranted to clarify the contribution of microglia versus non-microglial immune cells possibly acting together during SB.
Stress-induced inflammation can prime microglia to respond more strongly when re-exposed to immune challenges (Neher and Cunningham, 2019). Based on a previous morphometric characterization of IBA1-positive cells in the dACC (Torres-Platas et al., 2014a,b) have suggested an increased density of primed microglia (bigger cell body, ellipsoid-like soma and fewer higher-order branches) in the dACC of individuals with MDD who died by suicide compared to age- and PMI-matched controls. Microglial priming is a hallmark of both MIA, associated with increased SCZ risk, and ELA, or the emotional and physical abuse prior to the age of 18 (Tay et al., 2017; Smith and Pollak, 2020). Accordingly, it is tempting to speculate that microglial priming during prenatal and postnatal development contributes to the key role of ELA in SB risk (Figure 1), particularly via impairing neuronal function (Tay et al., 2017), as we discuss next.
MIA induced using a maternal high-fat diet results in exacerbated responses of plasma cytokines, such as IL6, to LPS, and decreased microglial interactions with the extracellular space in the HIP of male and female adolescent mouse offspring (Bordeleau et al., 2020), which might be relevant for structural remodeling of synapses or myelination (Nguyen et al., 2020). Indeed, in a follow up study, maternal high-fat diet was associated with decreased postnatal presence of structures that support the axon-myelin unit known as cytosolic myelin channels, in the corpus callosum of adolescent males, contrasted by increased microglial synaptic contacts in both sexes (Bordeleau et al., 2021). An altered microglial support of myelination could be connected to the myelin impairment detected in the ACC epigenome and the transcriptome of post-mortem brain samples from individuals with MDD and a history of ELA who died by suicide (Lutz et al., 2017). Considering the marked sexual dimorphism observed in ELA and MIA, it will be crucial that studies addressing this question consider sex differences in microglial behavior.
Male mice receiving an intraperitoneal injection of LPS during postnatal development display microglial upregulation of CX3CR1 which, in a TLR4-dependent manner, causes heightened synaptic engulfment in the ACC and results in MDD-like symptoms upon acute exposure to unpredictable stressors during adolescence (Cao et al., 2021). Notably, elevated TLR4 and TLR3 expression was found in the post-mortem DLPFC of individuals who died by suicide, irrespective of MDD diagnoses (Pandey et al., 2014). Moreover, the TLR2 was among the top ten genes involved in the DLPFC of individuals with MDD who died by suicide, compared to individuals with MDD who died of other causes and healthy controls (Zeng et al., 2020; Supplementary Table 1). According to the results obtained by Cao et al. (2021), TLR expression changes in post-mortem brain tissue could be indicative of increased postnatal peripheral inflammation leading to microglial synaptic pruning and elevated susceptibility to SB, warranting further investigation.
Postnatal exposure to alcohol in adult male rats increased HYP protein expression of CD11b, a component of complement receptor 3 mediating microglial pruning of axon terminals (Schafer et al., 2012; Chastain et al., 2019). Upregulation of CD11b was accompanied by heightened mRNA levels of Tnf, Il6, Tl4, and Csf1r both in vivo and in vitro, as well as protein levels of epigenetic modulators in microglia from the HYP paraventricular nucleus (Chastain et al., 2019). Particularly, Chastain et al. (2019) revealed a decreased global DNA methylation but increased acetylation of factors that upregulate inflammatory gene transcription, such as histone H3 lysine 9, which prevailed throughout adulthood. Less transcriptional repression in microglia was paralleled by an elevated GC response to a LPS challenge during adulthood and reversed by minocycline (Chastain et al., 2019), a tetracycline normalizer of microglial cytokine release and phagocytosis (Šimončičová et al., 2022). GC are produced by the HPA axis following exposure to physiological or biological threats and have numerous functions, largely affecting inflammatory and metabolic processes (de Kloet et al., 2005; Lucassen et al., 2014; Picard et al., 2021), as well as synaptic plasticity, behavior, and memory (McEwen and Magarinos, 2001). Polymorphisms and differential epigenetic regulation of numerous factors along the HPA axis can underlie impaired stress responses, together with increased psychiatric risk, SB and suicide ideation (Roy et al., 2017; Ke et al., 2018; Menke et al., 2018; Berardelli et al., 2020; Nold et al., 2021). It is possible that inflammatory and epigenetic responses mediated by HYP primed microglia and impacting the HPA support the corresponding increase of SB risk in adolescents exposed to alcohol during development (O’Connor et al., 2019), although warranting more substantial evidence. Additionally, this HPA effect could be mediated via CD11b-complement 3 synaptic remodeling. Correspondingly, decreased mRNA Cd11b expression was detected in the ACC of individuals with MDD who died by natural causes but not suicide, compared to age and PMI-matched controls (Zhang L. et al., 2021). Moreover, increased complement protein 3 was detected by microarray in the AMY, HIP, TLM of individuals who died by suicide compared to healthy controls (Glavan et al., 2021). Future studies are needed to explore the role of complement synaptic pruning in SB.
The increased density of primed cells observed by Torres-Platas et al. (2014b) constitutes the only available microglial quantitative morphological analysis in the context of SB. Microglia use cues from the microenvironment to tune their branching processes, as well as the shape and size of their soma, and modulate how motile, mobile and interactive they are with their surroundings (Savage et al., 2019). Enlarged microglial soma size, present in primed cells, was linked to enhanced gene transcription in adult mice (Dungrawala et al., 2010; Kozlowski and Weimer, 2012; Marguerat and Bähler, 2012) and to microglial mobility in aged male and female mice (Hefendehl et al., 2014). Moreover, elevations in IBA1-positive cell area, also detected after priming, were associated with the uptake of neuronal inclusions which coincided with a decreased spine density in the PFC of adult male mice exposed to CUS (Milior et al., 2016; Wohleb et al., 2018). Microglial morphological adaptations related to phagocytosis of neuronal elements were also linked to MDD-like behaviors in CUS-exposed male mice (Milior et al., 2016; Wohleb et al., 2018). Future research is needed to evaluate whether priming-driven changes in microglial morphology are tied to synaptic remodeling and if they could help understand, for example, the impaired reward and decision making, as well as mental pain associated with SB in the DLS and NA (Schmaal et al., 2020). Although microglial morphology is intimately associated to their activity, analyses of microglial morphological states can be highly contradictory, as shown in the stress literature, probably due to experimental variability in the time course and types of stress paradigms used, as well as regions examined and various coping strategies employed (Picard et al., 2021). Qualitative data has argued for a lack of difference in morphology of HLA-DR-positive cells in the DRN of individuals with MDD who died by suicide compared to individuals with MDD who died by other causes and healthy controls (Brisch et al., 2017). In particular, this study compared cells with enlarged cell body and few ramifications, also known as ameboid, and microglia with numerous radial, thin ramifications, defined as ramified (Brisch et al., 2017). Similar lack of SB-specific effects was suggested after comparing the density of HLA-DR ameboid and ramified microglia within the ACC, DLPFC, HIP, and MTN of two individuals with SCZ who died by suicide compared to age-, sex-matched healthy controls (Steiner et al., 2006). Other qualitative assessments, for example, in the ACC and VLPFC, resulted in similar findings (Steiner et al., 2011; Schnieder et al., 2014; Clark et al., 2016; Petrasch-Parwez et al., 2020). Quantitative assessments of microglial morphology are notably required to verify more nuanced differences along the spectrum of morphologies microglia can assume, which goes beyond ramified and ameboid states (Savage et al., 2019). Prospective studies should consider the variety of factors that can influence the shape and size of microglia and contextualize in space and time their morphological and stereological investigations.
Differences of microglial distribution across the CNS are reported in homeostatic contexts, for instance, with higher numbers in cortical regions and lower values in the cerebellum of adult male and female mice (Verdonk et al., 2016; Stowell et al., 2018). Regional microglial heterogeneity is similarly relevant after stress and inflammation (Tan et al., 2020). Upon CRS, only 9 out of 15 CNS regions, including the NA and HIP CA3, presented elevated densities of IBA1-positive cells in adult male rats (Tynan et al., 2010). Similarly, adult male mice showed increased IBA1-positive cell density in the DG 2 days after CUS, while at 5 weeks, a reduction in IBA1-positive cells was observed (Kreisel et al., 2014). In another work, acute but not CSDS resulted in marked increases in CX3CR1-positive cells. Elevated CX3CR1 density was identified in 7 out of 12 regions examined, including the ACC, NA, dorsal DG of adult male mice (Lehmann et al., 2016). Minocycline administration supports that, at least partially, modulation of the inflammatory activity of microglia can rescue changes in density among stress-sensitive areas. In adult male mice receiving IFNα therapy, minocycline rescued increases in density of HIP IBA1-positive cells and mRNA expression of cytokines such as Ifnα, Il6, Il1β, and Tnf (Zheng et al., 2015). In the same animals, it similarly restored decreased immunolabeling for the proliferation markers Ki67 and BrdU, neuronal progenitor marker TBR2 and maturation marker DCX, as well as increased MDD-like behaviors (Zheng et al., 2015). Moreover, this antibiotic rescued the effects of CRS in adult male rats, improving spatial working memory and reducing the PFC immunoreactivity for IBA1 and FOSB, which progressively accumulated in repeatedly activated neurons (Hinwood et al., 2012) and is associated with MDD and suicide risk, according to transcriptomic data (Zeng et al., 2020). Minocycline also attenuated increases in IBA1 and C1q protein expression correlated with decreased DRN serotoninergic signaling caused by social isolation-induced alcohol intake in adult male mice (Lee et al., 2021). In adult male rats exposed to ESI, treatment with minocycline further reverted MDD-like behavior, HIP protein IBA1 increases, as well glutamate receptor subunits reductions (Wang et al., 2017). Overall, these results suggest that, in stress-susceptible regions, inflammation potentially affects the density of microglia and impairs synaptic remodeling, which could be relevant to density changes observed upon SB (Figure 1).
Alike stress, specific CNS regions were more susceptible to changes in IBA1 or HLA-DR upregulation in post-mortem samples. Increased density of cells immunostained for IBA1 or HLA-DR was found in the dACC of male and female individuals with MDD or SCZ who died by suicide (Supplementary Table 1) compared to sex- (Steiner et al., 2006, 2008) and age-matched healthy controls (Torres-Platas et al., 2014b). Elevated HLA-DR was also observed in the DLPFC and MTN of female and male individuals who died by suicide, regardless of their psychiatric diagnosis (Steiner et al., 2006, 2008). Conversely, decreased HLA-DR immunostaining was detected in the DRN of individuals with MDD who did not die by suicide, compared to those who did and to healthy controls (Brisch et al., 2017). By contrast, no significant differences in HIP HLA-DR staining were, however, detected in two individuals with SCZ who died by suicide, compared to healthy matched controls (Gos et al., 2014). Moreover, IBA1-positive cell density in the aMCC did not differ between individuals with SCZ who died by suicide compared to age-matched individuals with SCZ who died by other causes or healthy controls (Petrasch-Parwez et al., 2020).
As previously discussed, HLA-DR expression is associated with immune signaling, however, studies have yet to explore the possible outcomes of the heterogenous HLA-DR upregulation found in SB. Similarly, while widely used to assess microglial reactivity (Ohsawa et al., 2000), the function of the actin-related protein IBA1 marker is still largely elusive (Walker and Lue, 2015). Depletion of IBA1 can impair HIP microglial motility, leading to deficits in synapse engulfment and increased excitatory synapse number in juvenile mice that impact behavior into adulthood (Lituma et al., 2021). The paradoxical reduction in synaptic uptake and number of excitatory synapses requires further investigation but could indicate that IBA1 signaling participates first in synaptogenesis and later in synaptic pruning during postnatal CNS development (Lituma et al., 2021). Accordingly, it is possible that changes in IBA1 expression detected in post-mortem samples from individuals who died by suicide reflect alterations of synaptic plasticity, rather than inflammation per se. Various cognitive alterations are observed in the areas where disturbed post-mortem levels of microglial cells were found, such as the ACC, DLPFC, and MTN. In one study, individuals with MDD and SB displayed a reduced reward anticipation and an increased activation of the ACC after viewing angry faces, as detected by fMRI (Pan et al., 2013; Schmaal et al., 2020). Moreover, a negative correlation between the severity of suicide ideation and resting state fMRI among the MTN was found in adults with MDD (Kim et al., 2017), while deficits in PFC inhibition and flexibility are thought to contribute to the transition between suicide ideation and SB (Schmaal et al., 2020). Possible causal adaptive and maladaptive relationships between the microglial region-specific cell numbers, IBA1, and HLA-DR signaling adaptations (Figure 1) warrant future investigation.
Cx3cr1 transcripts are increased in the ACC but not in the DLPFC of individuals with SCZ who died by suicide compared to individuals with SCZ who died by other causes (Zhang L. et al., 2021; Supplementary Table 1). Further, CD11b-positive cells from MFG, STG, TLM, and SZ tissue presented increased mRNA expression of Cx3cr1 when isolated from individuals with MDD compared to age-matched healthy controls (Snijders et al., 2020). According to a microarray study, increased Cx3cr1 was also detected in the AMY, HIP, and TLM of individuals who died by suicide compared to healthy controls (Glavan et al., 2021). By contrast, no difference in Cx3cr1 mRNA expression was detected in the ACC or DLPFC of individuals with MDD who died by natural causes and suicide, compared to age and PMI-matched controls (Zhang L. et al., 2021). Microglia and neuronal cells use a wide variety of signaling molecules, including fractalkine, to maintain their balanced communication in the CNS, required for optimal function of both cellular types (Paolicelli et al., 2014). Fractalkine signaling relies on binding of CX3CR1, largely expressed by microglia in the CNS, to the neuronal chemokine fractalkine, or CX3CL1, which generally inhibits microglial activity (Harrison et al., 1998; Biber et al., 2007). CX3CR1-CX3CL1 activity is needed for neuronal maturation and survival, plasticity of synapses and behavior (Paolicelli et al., 2014). During postnatal development, CX3CR1 is required for microglial recruitment to the cortex and maturation of postsynaptic glutamate receptors in female and male mice (Hoshiko et al., 2012). Furthermore, CX3CR1 deficiency impairs HIP neuronal long-term potentiation, a common paradigm to study synaptic plasticity, and results in cognitive and neurogenesis deficits in adult male mice (Bachstetter et al., 2011; Rogers et al., 2011). Given that fractalkine signaling is needed for optimal function of both cellular types (Paolicelli et al., 2014), regional mechanisms involving CX3CR1 could participate in susceptibility to stressors and inflammation contributing to SB risk. Despite the robust role of fractalkine signaling in synaptic plasticity, following CSDS in adulthood, CX3CR1 knockout male mice did not develop anxiety-like behavior compared to wild-type mice, an effect attributed to their lack of peripheral immune cell recruitment to the CNS (Wohleb et al., 2013). Moreover, adult CX3CR1 knockout male mice exposed to CUS failed to modify their HIP microglial morphology, phagocytosis, neuronal plasticity and did not show MDD-like behaviors compared to wild-type mice (Milior et al., 2016; Rimmerman et al., 2017). Similar resistance to developing MDD-like behaviors was found in an adult male and female mouse model of chronic behavioral despair (Hellwig et al., 2016) and in adult male mice exposed to ELA and chronic variable stress in adulthood (Winkler et al., 2017). Additionally, in a human induced pluripotent stem cell microglial model, CX3CR1 knockout resulted in heightened phagocytosis, as assessed with a bead assay (Murai et al., 2020). Region-specific elevations in Cx3cr1 expression found in post-mortem samples could confer an increased stress susceptibility to microglia, disrupting phagocytosis and neuronal plasticity and contributing to SB (Figure 1), which warrants further research.
Oxidative stress is a hallmark of sustained and often maladaptive inflammation (Chaudhari et al., 2014). It refers to the unbalanced production of free radicals, including ROS, which possess unpaired electrons that readily oxidize and modify lipids, DNA and proteins (Bakunina et al., 2015; Black et al., 2015). Despite having homeostatic roles in neurogenesis, synaptic plasticity, programmed cell death and pathogen removal, oxidative stress can severely damage CNS cells. The CNS consumes around 20% of the total oxygen levels in the body but has limited anti-oxidant capacity, therefore, it requires ROS levels to be tightly controlled (Black et al., 2015). As most phagocytic cells, microglia are robust sources of ROS (Block and Hong, 2007). Selective depletion of microglia using the CSF1R antagonist PLX5622 protected adult male mice from the negative behavioral outcomes of CSDS, an effect that was specifically attributed to excessive microglial ROS production in the PFC and HIP (Lehmann et al., 2019). Furthermore, compared to resistant and healthy mice, microglia isolated from the HIP and medial PFC of adult male mice susceptible to CSDS presented higher ex vivo labeling with a membrane-permeable dye indicating respiratory burst activity and ROS production (Lehmann et al., 2018). Excessive microglial oxidative stress activity could increase susceptibility to psychiatric and SB risk (Figure 1). Notably, ROS production arises from multiple mechanisms, which could be, simultaneously or not, at play in SB, as we outline next.
Microglial ROS can be produced through the activity of NOX2 in response to pathogens and inflammatory molecules (Block and Hong, 2007). Given the inflammatory profile identified in many individuals with SB, microglial NOX2 signaling could be recruited in suicide. In post-mortem brain samples of individuals who died by asphyxiation suicide, NOX2 protein expression was markedly upregulated in cortical GABAergic inhibitory neurons and to a lesser extent in MAC387-positive cells, representing microglia and macrophages, compared to healthy controls and non-suicide asphyxiation deaths (Schiavone et al., 2016; Supplementary Table 1). NOX2 upregulation was accompanied by elevations in IL6 and 8-OHdG, a by-product of ROS-oxidized guanine (Schiavone et al., 2016). Elevated 8-OHdG levels were similarly detected in the CA1, CA2 and DG of male and female individuals with MDD or SCZ compared to controls (Che et al., 2010). While looking at death by asphyxiation, the work by Schiavone et al. (2016) highlights that specific methods of suicide are likely associated with different impacts on oxidative or inflammatory pathways affecting microglial function, an area that awaits future investigation.
ROS can similarly originate from the activity of the mitochondrial respiratory chain (Wilkinson and Landreth, 2006). Twenty subunits of the mitochondrial oxidative phosphorylation complexes showed increased levels in the DLPFC of individuals with MDD, whilst being usually decreased in SCZ (Martins-de-Souza et al., 2012). Furthermore, a suicide-specific elevation in the expression of DNA-dependent ATPase activity was revealed by gene ontology analysis in the DLPFC, AMY, and TLM (Pantazatos et al., 2017; Supplementary Table 1). Higher oxidative phosphorylation activity was hypothesized to compensate for oxidative stress which depletes ATP production in the DLPFC of individuals with MDD (Martins-de-Souza et al., 2012). Despite looking at post-mortem tissue from suicide deaths, the study by Martins-de-Souza et al. (2012) did not discuss the SB-specific proteomic changes in their cohort, encouraging further research in this topic. Additionally, after CUMS, adult male mice exhibited altered mitochondrial ultrastructure, such as swelling, disrupted cristae and membranes and impaired respiration rates among the cortex, HIP, and HYP, paralleling MDD-like behaviors (Gong et al., 2011). Overall, these results indicate mitochondrial respiratory chain oxidative stress is seen upon SB and stress.
Disrupted mitochondria, dilation of the Golgi apparatus and endoplasmic reticulum, as well as cell shrinkage and abundant endosomes, are hallmarks of DM, a microglial state uncovered by our group (Bisht et al., 2016). DM often encircle synaptic structures and are occasionally surrounded by extracellular space containing debris, altogether suggesting ongoing synaptic pruning and extracellular digestion (Bisht et al., 2016). Albeit nearly absent during mature steady-state conditions in the HIP, PFC, HYP, and BLA, DM become abundant in male mice subjected to MIA and in mouse models of stress, including CSDS and CUMS (Bisht et al., 2016). Given the appearance of DM upon distal and proximal SB risk factors as well as their marked oxidative stress, it is tempting to speculate that DM are potentially found upon SB. Electron microscopy studies assessing post-mortem samples from suicide deaths could clarify this matter. Notably, “dystrophic” microglia that resemble DM are present in the post-mortem PFC of individuals with SCZ (Uranova et al., 2018). Correspondingly, DM could help explain the dendritic atrophy and reduced spine density found in the PFC and HIP of male and female rats and mice exposed to CRS and CUMS (Qiao et al., 2016), similar to what is observed in the same regions of individuals with MDD (Duman et al., 2016; Bollinger and Wohleb, 2019).
In addition to marked oxidative stress, DM are characterized by an electron-dense nucleus with altered nuclear heterochromatin (Bisht et al., 2016; St-Pierre et al., 2020). Nuclear heterochromatin remodeling is a process coupled to DNA methylation and transcriptome adaptations (Robertson, 2002). Notably, DNA methylation is one of the proponent mechanisms by which ELA translates into higher SB risk later in life (Lutz et al., 2017; Zeng et al., 2020). ELA-induced methylation could potentially drive the appearance of DM, albeit more direct evidence is needed to support this hypothesis. Initial studies indicate microglia can be the target of complex epigenetic modulation after exposure to psychological stress, such as ESI and ESS (Wang et al., 2017; Catale et al., 2020), or physiological challenges, for instance early-life alcohol exposure (Chastain et al., 2019). IBA1-positive cells located in the core and shell of the NA, dorsomedial striatum and DLS, CA1 and CA3 of the HIP, as well as BLA and central AMY showed a marked decrease of methylation in ESI mice compared to control and ESS groups (Catale et al., 2020). Additionally, the authors revealed a decreased global methylation in the CA1 of mice exposed to ESS compared to controls from both sexes (Catale et al., 2020). While similar mechanisms might participate in SB, microglial epigenetic studies in the context of SB neuropathology are currently lacking.
Cytokines and oxidative stress molecules work in a positive feedback loop (Black et al., 2015; Figure 1). ROS stimulation results in activation of NF-κB and production of cytokines (Bakunina et al., 2015; Black et al., 2015). In turn, increased protein levels of IFNγ, IL6, IL1β, and TNF enhance oxidative stress (Bakunina et al., 2015; Black et al., 2015). Cytokines and ROS, both notably released by microglia, similarly activate IDO and TDO, which metabolize L-tryptophan through the kynurenine pathway (Heyes et al., 1992, 1996; Alberati-Giani et al., 1996; Fujigaki et al., 2017). In humans, IDO is expressed by microglia (Figure 1), astrocytes and to a lesser extent in neurons, whilst TDO is mostly detected in astrocytes (Wu et al., 2013). Decreased kynurenine:tryptophan ratio was identified in the VLPFC of individuals with MDD compared to healthy controls, irrespective of the cause of death, including suicide (Clark et al., 2016; Supplementary Table 1). In agreement, this study detected lower mRNA expression of Ido1 and Ido2, their homologous genes, as well as Tdo, in the VLPFC of individuals with MDD (Clark et al., 2016). In adult male rats, following LPS injection, mRNA upregulation of Ido was found to be accompanied by increased transcript levels of Tnf and Il6 in the cortex and HIP (O’Connor et al., 2009). While it is unclear if a similar upregulation is present in SB, a polymorphism in the promoter region of the IDO1 gene is associated with higher risk of developing MDD symptoms in individuals receiving IFN therapies (Smith et al., 2012), suggesting that IDO1 plays a significant role in determining the susceptibility to SB.
In the brain, L-kynurenine gets broken down into 3-hydroxykynurenine, QUIN and xanthurenic acid, all synthesized by microglia and peripheral macrophages according to human cells in vitro evidence (Heyes et al., 1992, 1996; Espey et al., 1997; Gos et al., 2014), while kynurenic acid and picolinic acid are produced by astrocytes (Raison et al., 2006; Fujigaki et al., 2017). QUIN is a marker of oxidative stress which was found to be significantly elevated in the CSF of individuals with a history of suicide attempts (Erhardt et al., 2013) and in the ACC of individuals with MDD who died by suicide compared to age- and sex-matched healthy controls (Steiner et al., 2011; Supplementary Table 1). In the same cohort, however, decreased QUIN immunoreactivity was found in the HIP CA1 of individuals with MDD or bipolar disorder who died by suicide compared to age- and sex-matched healthy controls, indicating that different brain regions likely present distinct microglial oxidative balances in SB (Busse et al., 2015). Similarly, individuals with SCZ presented reduced QUIN binding in the CA1 compared to controls without psychiatric diagnoses, despite a lack of specific changes in the two suicide deaths included in this study (Gos et al., 2014). Robust evidence links oxidative stress and SB; however, more studies are warranted to explore the extent through which microglia contribute to modulating region-specific tryptophan metabolism in particular mental health disorders and SB (Suzuki et al., 2019; Baharikhoob and Kolla, 2020).
Tryptophan is a precursor of serotonin (Bakunina et al., 2015; Fujigaki et al., 2017). Increased microglial degradation of tryptophan, supported by changes in IDO isoforms and QUIN levels, could help explain the decreased levels of serotonin metabolites measured in the CSF of individuals with MDD and SB (Brown et al., 1982; Mann et al., 1992; Steiner et al., 2011; Bakunina et al., 2015; Fujigaki et al., 2017; Figure 1). Notably, serotonin depletion is associated with increased impulsivity and aggressive behaviors (Stanley et al., 1982; Stanley and Mann, 1983), low mood, anxiety, as well as suicide risk (Achtyes et al., 2020; Baharikhoob and Kolla, 2020; Koweszko et al., 2020). More research could clarify the specific contribution of microglia to serotonin depletion in the context of SB. Simultaneously, serotonin affects the inflammatory activity, trophic support, phagocytosis and motility of microglia in a context-dependent manner (Turkin et al., 2021). For instance, serotonin treatment enhanced adult mouse cortical microglial process motility toward laser injury but reduced phagocytosis of beads by neonatal amoeboid microglia of the corpus callosum in situ (Krabbe et al., 2012). Adult male mice treated with the selective serotonin reuptake inhibitors fluoxetine exhibited different HIP inflammatory responses according to the quality of their living environment (Alboni et al., 2016). In mice exposed to CUMS, fluoxetine was associated with anti-inflammatory responses, such as reduced Tnf transcripts, increased microglial spacing index and cell body area, but decreased arborization in the HIP CA1 (Alboni et al., 2016). Conversely, in mice housed in an enriched environment, microglia had increased pro-inflammatory responses, involving IL1β protein and Tlr4 mRNA (Alboni et al., 2016). Stress appears to regulate serotonin levels along with microglial activity. Social isolation in adult male mice caused a decrease in DRN serotonin production accompanied by elevated IBA1 and C1q protein expression, as well as depressive-like behavior (Lee et al., 2021). Upregulation in complement expression was paralleled by a downregulation in synaptic proteins, i.e., postsynaptic density protein 95 and synaptophysin, which could indicate synaptic pruning (Lee et al., 2021). It is therefore possible that stress-induced serotonin depletion increases the susceptibility to psychiatric disorders or SB via adaptations in microglial pro-inflammatory activity, cell motility and microglial synaptic remodeling, an area that awaits future investigation.
In LPS-treated adult male mice, minocycline and the IDO antagonist 1-methyltryptophan rescued the development of MDD-like behavior, independently of brain serotonin turnover (O’Connor et al., 2009). Therefore, at least partially, microglial IDO may influence SB independently of serotonin. As previously described, in addition to reducing tryptophan levels, IDO activity results in production of the metabolite QUIN in microglia. QUIN is an agonist of the NMDAR crucial for glutamatergic excitatory signaling in the CNS (Olloquequi et al., 2018). Elevated levels of QUIN, found in association with SB, as discussed above (see section “Microglial Tryptophan Metabolites Are Regionally Detected in Suicidal Behaviors”), can over-activate the NMDAR, generating abnormally high levels of intracellular Ca2+, toxic free radicals and disrupted ATP production that ultimately contribute to loss of neuronal cell function and cell death, a process formally known as glutamate excitotoxicity (Olloquequi et al., 2018). QUIN-mediated glutamate excitotoxicity could be another mechanism by which microglia participate in SB (Suzuki et al., 2019; Baharikhoob and Kolla, 2020; Figure 1). Correspondingly, several clinical trials with ketamine, a NMDAR antagonist, found a significant and rapid reduction in suicidal cognition, a reason why ketamine therapy is currently being evaluated for the treatment of suicidal ideation (Ballard et al., 2021). Ketamine was shown to rescue MDD-like behavior, decreasing brain IL6, TNF and QUIN protein production, as well as CX3CR1-positive cell area, whilst increasing CX3CR1-positive cell arborization in the PFC of adult male mice exposed to LPS (Verdonk et al., 2019). Partial depletion of microglia by the CSF1R inhibitor PLX3397 blocked the effects of R-ketamine, a more potent and longer-lasting enantiomer of (R,S)-ketamine in adult male mice susceptible to CSDS (Zhang K. et al., 2020). Hence, ketamine affects microglia in a manner that is partially required to achieve its anti-depressant effects. It is, however, unknown if similar mechanisms underlie the effects of ketamine in SB.
Despite positive results in the context of MDD, an inverse response to ketamine is found in SCZ, where it produces psychosis-like responses in healthy subjects and can temporarily worsen positive symptoms in individuals previously diagnosed with SCZ (Lahti et al., 2001). This indicates that NMDAR antagonism is to some degree involved in SCZ pathology (Stone et al., 2007; Straub et al., 2007; Nakazawa et al., 2012; Steiner et al., 2012) and that ketamine may possibly induce disorder-specific adaptations in microglia. It is postulated that hypofunctional NMDAR located on GABAergic inhibitory interneurons disinhibit excitatory pyramidal neurons, the principal neurons of the cerebral cortex, leading to a paradoxical increase in glutamatergic activity that contributes to SCZ (Stone et al., 2007; Nakazawa et al., 2012). Based on cortical post-mortem results of asphyxiation suicide deaths, cytokines such as IL6 affecting NOX2 expression in GABAergic neurons, can contribute to oxidative stress that causes the loss of inhibitory tone on glutamatergic neurons and increases glutamatergic excitotoxicity (Schiavone et al., 2016; Supplementary Table 1). Cortical glutamate excitotoxicity is hypothesized to elevate synaptic apoptosis, a sub-lethal form of apoptosis in terminal neurites and individual synapses that triggers synaptic elimination in the absence of cell death, in line with the reduced cortical volume in individuals with SCZ without associated pyramidal cell loss (Bennett, 2011; Schobel et al., 2013; Parellada and Gassó, 2021). While in the DLPFC and HIP apoptosis and neuronal death pathways were significantly correlated with suicide death (Zeng et al., 2020; Glavan et al., 2021), further studies are required to evaluate whether these changes reflect cellular or synaptic events. It is suggested that synaptic apoptosis causes exposure of internal phosphatidylserine and accumulation of complement on the synaptic membrane, such as C1q, which stimulate microglial chemotaxis and synaptic pruning (Nonaka and Nakanishi, 2019; Parellada and Gassó, 2021). Minocycline successfully dampened the aberrant synaptic engulfment of SCZ patient-derived microglial cells in vitro (Sellgren et al., 2019) and may be used for similar outcomes in SB.
Glutamate excitotoxicity elevates extracellular levels of ATP in HIP slices of adult female and male mice (Dissing-Olesen et al., 2014), similar to what is observed in male rats exposed to CUS, which can develop MDD behaviors upon chronic ATP administration (Yue et al., 2017). In mice, cortical P2RY12 binds to extracellular nucleotides, like ATP, and is required for postnatal synaptic plasticity (Sipe et al., 2016), in addition to microglial chemotaxis and phagocytosis (Davalos et al., 2005; Haynes et al., 2006; Dissing-Olesen et al., 2014), as well as BBB closure (Lou et al., 2016), upon injury in adult males. Elevated P2ry12 transcripts are found across the ACC of individuals with SCZ who died by suicide compared to individuals with SCZ who died by other causes (Zhang L. et al., 2020; Supplementary Table 1). Correspondingly, a single-cell microglial study of medicated individuals with MDD has found elevations in P2RY12 protein expression in the frontal lobe, temporal lobe, TLM and SZ, in parallel with a decrease in HLA-DR and CD68 compared to age-, sex- and PMI-matched controls (Böttcher et al., 2020). Moreover, decreased mRNA P2ry12 expression was detected in the ACC of individuals with MDD who died by natural causes but not suicide, compared to age and PMI-matched controls (Zhang L. et al., 2020). Upregulation of P2RY12 without exacerbated inflammation may indicate increased neuron-microglia communication (Böttcher et al., 2020). It is possible that, after stress, ATP-P2RY12 recruitment attracts microglia toward neurons with an elevated excitatory activity, promoting synaptic pruning to reduce excitability along with psychiatric-like behavior (Bollinger and Wohleb, 2019) and SB. Additional studies are warranted to explore the putative origins and outcomes of purinergic signaling in the context of SB.
Trem2 transcripts were reduced in the ACC of individuals with SCZ, but only in deaths not caused by suicide (Zhang L. et al., 2020; Supplementary Table 1). In opposition, mRNA Trem2 expression was not significantly different in the ACC or DLPFC of individuals with MDD who died by natural causes and suicide, compared to age and PMI-matched controls (Zhang L. et al., 2021). TREM2 is a cell surface protein binding to phospholipids, phosphatidylserine, sulfatides, LPS and DNA, which is involved in amyloid β and apoptotic neuron clearance, as well as AD risk (Guerreiro et al., 2013; Jonsson et al., 2013; Wang et al., 2015; Krasemann et al., 2017). MDD risk genes were involved in the pathology of AD (Ni et al., 2018), but it is unclear whether TREM2 signaling is affected (Santos et al., 2016). Balanced TREM2 levels could be a protective factor against the apoptotic effects of glutamate excitotoxicity (Ren et al., 2021) in SB, although requiring more substantial research. Studies with TREM2 knockout mice suggest this protein can reflect microglial proliferation, survival around amyloid β and migration toward injury throughout life (Ulland and Colonna, 2018). Moreover, TREM2 absence impairs postnatal microglial synaptic pruning, consequently increasing HIP excitatory neuronal activity and stereotypic behavior in adult female and male mice (Filipello et al., 2018). Accordingly, elevated microglial TREM2 protein expression reduced microglial production of the pro-inflammatory cytokines TNF and IL1β, but increased phagocytosis in vitro (Takahashi et al., 2005). Additional studies are needed to clarify the outcomes of reduced TREM2 expression in psychiatric or SB risk.
Microglia could additionally influence plasticity in SB by altering the release of neurotrophins, such as BDNF (Dwivedi, 2009). Individuals with a history of suicide attempts and MDD present reduced levels of plasma BDNF compared to control groups (Dawood et al., 2007; Deveci et al., 2007; Kim et al., 2007; Lee et al., 2007), although a larger study found a lack of effect (Eisen et al., 2016). Moreover, decreased BDNF protein expression was detected in the PFC and HIP of individuals who died by suicide, compared to healthy controls (Dwivedi et al., 2003; Banerjee et al., 2013). Similarly, BDNF protein level was lower in the ACC, but not the DLPFC, in suicide decedents with a history ELA compared to controls without reported adversity or suicide death (Youssef et al., 2018). In vivo and in vitro data from adult male rats indicates microglial BDNF can cause neuronal hyperexcitability in the spinal cord relevant to pain disorders (Coull et al., 2005). In adult mice, abrogation of microglial BDNF is enough to recapitulate the effects of CX3CR1 cells depletion in motor learning tasks and cortical dendritic spine remodeling (Parkhurst et al., 2013). Moreover, susceptibility to CUMS in adult male mice was associated with reduced HIP arginase-positive microglial IL4 signaling and decreased microglial production and secretion of BDNF (Zhang J. et al., 2021). Dysfunctional microglial BDNF production and synaptic remodeling activity as a result of susceptibility to stress could help explain changes in neurotransmitter binding and dendritic spine density observed in individuals with SB (Kang et al., 2012; Underwood et al., 2012, 2020; Dean et al., 2016; Holmes et al., 2019). Altogether, these results emphasize the intricate and multidimensional microglial activities putatively involved in SB, encouraging further studies in this area.
Recent research has uncovered that the widespread and diverse functions of microglia are fulfilled by a spectrum of cellular states with variable morphology, proteome, metabolome and ultrastructure (Stratoulias et al., 2019). Microglial diversity is drawn by cues from their microenvironment, which differ according to the species, age, sex, CNS region, particular context of health or disease, as well as lifestyle habits of the organism (Hanamsagar and Bilbo, 2017; Bordeleau et al., 2019; Masuda et al., 2020). Context-dependent microglial diversity challenges the entangling of suicide-specific microglial mechanisms. As a primary constraint, results from animal models of SB risk factors may not completely translate to humans. For example, despite sharing a generally similar transcriptome with mouse microglia, human microglia isolated from surgical procedures presented a species-specific expression bias for several transcripts, including those associated with complement proteins, crucial for synaptic turnover (Gosselin et al., 2017). Secondly, great variability in the distribution of sex, age, PMI and psychiatric diagnoses is present among samples examined thus far (Supplementary Table 1), straining inter- and intra-studies comparisons. For example, the PMI between studies fluctuates from 7 to 40 h (Supplementary Table 1) and was shown to correlate with some of the gene expression and morphological alterations detected in microglia (Steiner et al., 2006; Gos et al., 2014; Torres-Platas et al., 2014b). Acutely tuned to environmental cues, microglia appear to change from the time of death until tissue fixation or cell isolation. Their dynamic processes still respond to axonal lesions of the spinal cord up to 10 h after the death of adult mice (Dibaj et al., 2010). Yet, post-mortem microglial responses are not expected to last longer than this given the depletion of immediate energy sources such as ATP (Dibaj et al., 2010), oxygen and glucose (Eyo and Dailey, 2012). Moreover, it is predicted that, even in the best scenarios of body storage at 4°C, some RNA, DNA and protein degradation occurs, thus, hindering multi-omic approaches and immunohistochemistry assays (Ferrer et al., 2008). Evidence from a mouse and human study, however, suggests that it is still possible to obtain viable microglia and high-quality RNA after 12 h PMI, with relatively few transcriptional changes compared to shorter PMIs (Heng et al., 2021). Correspondingly, one adult male mouse brain harvested and fixed after a PMI of 43 h mostly displayed similar numbers of ramified IBA1-positive cells in the ACC compared to brains immediately harvested after death (Torres-Platas et al., 2014a). Method of death could similarly create acute brain changes related to hypoxia and oxidative stress. To discriminate SB-specific changes, previous post-mortem studies investigating microglia have suggested matching suicide deaths groups with controls who died under stressful or inflammatory conditions, including homicide and death related to cardiovascular disease (Steiner et al., 2008; Clark et al., 2016). In addition to PMI or method of death, inter-individual differences in coping strategies, risk factor exposure, for instance, ELA, as well as factors such as sex, CNS region and age could help explain the heterogenous results concerning microglial gene and protein expression, as well as morphology found across studies in SB (Supplementary Table 1). Notably, significant efforts are already present in the literature to control for confounders using age-, sex-, tissue pH-, PMI-, and psychiatric diagnoses-matched cohorts (Steiner et al., 2006, 2008, 2011; Gos et al., 2014; Torres-Platas et al., 2014a; Busse et al., 2015; Schneider et al., 2015; Pantazatos et al., 2017; Petrasch-Parwez et al., 2020; Snijders et al., 2020; Glavan et al., 2021). It will be crucial that prospective studies continue to strive to contextualize in space and time their analyses of microglia.
Suicide has an important neurobiological component predisposed by cumulative risk factors throughout life, namely stress and psychiatric disorders. Abundant and diverse evidence posit microglia as mechanistically involved in the neurobiological etiology of SB and its risk factors. In this Review, we summarized various promising microglial pathways deriving from stress-induced inflammation, oxidative stress and trophic support that could be further investigated in the context of SB. Our discussion centered around putative outcomes on neuronal activity but also included BBB function, and other immune populations infiltrating the CNS. Moreover, we outlined how diversity in microglial states, functions and features, influenced by factors such as region, sex, age and lifestyle, is a key component to explore in future SB research. According to current data, it is likely that microglia participate in mechanisms that contribute to both the resistance and susceptibility to SB after stress. Selective modulation of processes that boost resistance to neuropathology encountered in SB could help prevent suicide deaths.
EGA, FGI, and M-ÈT conceptualized the manuscript. EGA prepared the tables and figure with input from FGI and M-ÈT. All authors contributed to the writing and editing of the manuscript.
This research was supported by Natural Sciences and Engineering Research Council (NSERC), Canadian Institutes of Health Research (CIHR) of Canada, and ERA-NET Neuron, Synaptic Dysfunction in Disorders of the Central Nervous System (MicroSynDep) grants awarded to M-ÈT. EGA was supported by a Graduate Entrance Award from the University of Victoria. FGI was supported by a scholarship from the Mexican Council of Science and Technology (CONACYT). M-ÈT is a Canada Research Chair (Tier II) in Neurobiology of Aging and Cognition.
The authors declare that the research was conducted in the absence of any commercial or financial relationships that could be construed as a potential conflict of interest.
All claims expressed in this article are solely those of the authors and do not necessarily represent those of their affiliated organizations, or those of the publisher, the editors and the reviewers. Any product that may be evaluated in this article, or claim that may be made by its manufacturer, is not guaranteed or endorsed by the publisher.
We acknowledge and respect that the University of Victoria is located on the territory of the ləkwəŋən peoples and that the Songhees, Esquimalt, and WSÁNEĆ peoples have relationships to this land. We are grateful to Benneth Ben-Azu for his careful revision of the manuscript.
The Supplementary Material for this article can be found online at: https://www.frontiersin.org/articles/10.3389/fncel.2022.839396/full#supplementary-material
Achtyes, E., Keaton, S. A., Smart, L., Burmeister, A. R., Heilman, P. L., Krzyzanowski, S., et al. (2020). Inflammation and kynurenine pathway dysregulation in post-partum women with severe and suicidal depression. Brain Behav. Immun. 83, 239–247. doi: 10.1016/j.bbi.2019.10.017
Aguilar-Valles, A., Aguliar-Valles, A., Kim, J., Jung, S., Woodside, B., and Luheshi, G. N. (2014). Role of brain transmigrating neutrophils in depression-like behavior during systemic infection. Mol. Psychiatry 19, 599–606. doi: 10.1038/mp.2013.137
Alberati-Giani, D., Ricciardi-Castagnoli, P., Köhler, C., and Cesura, A. M. (1996). Regulation of the kynurenine pathway by IFN-gamma in murine cloned macrophages and microglial cells. Adv. Exp. Med. Biol. 398, 171–175. doi: 10.1007/978-1-4613-0381-7_28
Alboni, S., Poggini, S., Garofalo, S., Milior, G., El Hajj, H., Lecours, C., et al. (2016). Fluoxetine treatment affects the inflammatory response and microglial function according to the quality of the living environment. Brain Behav. Immun. 58, 261–271. doi: 10.1016/j.bbi.2016.07.155
Arsenault-Lapierre, G., Kim, C., and Turecki, G. (2004). Psychiatric diagnoses in 3275 suicides: a meta-analysis. BMC Psychiatry 4:37. doi: 10.1186/1471-244X-4-37
Bachmann, S. (2018). Epidemiology of suicide and the psychiatric perspective. Int. J. Environ. Res. Public Health 15:E1425. doi: 10.3390/ijerph15071425
Bachstetter, A. D., Morganti, J. M., Jernberg, J., Schlunk, A., Mitchell, S. H., Brewster, K. W., et al. (2011). Fractalkine and CX3CR1 regulate hippocampal neurogenesis in adult and aged rats. Neurobiol. Aging 32, 2030–2044. doi: 10.1016/j.neurobiolaging.2009.11.022
Baharikhoob, P., and Kolla, N. J. (2020). Microglial dysregulation and suicidality: a stress-diathesis perspective. Front. Psychiatry 11:781. doi: 10.3389/fpsyt.2020.00781
Bakunina, N., Pariante, C. M., and Zunszain, P. A. (2015). Immune mechanisms linked to depression via oxidative stress and neuroprogression. Immunology 144, 365–373. doi: 10.1111/imm.12443
Ballard, E. D., Fields, J., Farmer, C. A., and Zarate, C. A. (2021). Clinical trials for rapid changes in suicidal ideation: lessons from ketamine. Suicide Life Threat Behav. 51, 27–35. doi: 10.1111/sltb.12663
Banerjee, R., Ghosh, A. K., Ghosh, B., Bhattacharyya, S., and Mondal, A. C. (2013). Decreased mRNA and protein expression of BDNF, NGF, and their receptors in the hippocampus from suicide: an analysis in human postmortem brain. Clin. Med. Insights Pathol. 6:Cath.S12530. doi: 10.4137/CPath.S12530
Bayard-Burfield, L., Alling, C., Blennow, K., Jönsson, S., and Träskman-Bendz, L. (1996). Impairment of the blood-CSF barrier in suicide attempters. Eur. Neuropsychopharmacol. 6, 195–199. doi: 10.1016/0924-977X(96)00020-X
Bennett, M. R. (2011). Schizophrenia: susceptibility genes, dendritic-spine pathology and gray matter loss. Prog. Neurobiol. 95, 275–300. doi: 10.1016/j.pneurobio.2011.08.003
Berardelli, I., Serafini, G., Cortese, N., Fiaschè, F., O’Connor, R. C., and Pompili, M. (2020). The involvement of hypothalamus–pituitary–adrenal (HPA) axis in suicide risk. Brain Sci. 10:653. doi: 10.3390/brainsci10090653
Biber, K., Neumann, H., Inoue, K., and Boddeke, H. W. G. M. (2007). Neuronal “On” and “Off” signals control microglia. Trends Neurosci. 30, 596–602. doi: 10.1016/j.tins.2007.08.007
Bisht, K., Sharma, K. P., Lecours, C., Sánchez, M. G., El Hajj, H., Milior, G., et al. (2016). Dark microglia: a new phenotype predominantly associated with pathological states. Glia 64, 826–839. doi: 10.1002/glia.22966
Black, C. N., Bot, M., Scheffer, P. G., Cuijpers, P., and Penninx, B. W. J. H. (2015). Is depression associated with increased oxidative stress? A systematic review and meta-analysis. Psychoneuroendocrinology 51, 164–175. doi: 10.1016/j.psyneuen.2014.09.025
Block, M. L., and Hong, J.-S. (2007). Chronic microglial activation and progressive dopaminergic neurotoxicity. Biochem. Soc. Trans. 35, 1127–1132. doi: 10.1042/BST0351127
Bollinger, J. L., and Wohleb, E. S. (2019). The formative role of microglia in stress-induced synaptic deficits and associated behavioral consequences. Neurosci. Lett. 711:134369. doi: 10.1016/j.neulet.2019.134369
Bordeleau, M., Carrier, M., Luheshi, G. N., and Tremblay, M. -È (2019). Microglia along sex lines: from brain colonization, maturation and function, to implication in neurodevelopmental disorders. Semin. Cell Dev. Biol. 94, 152–163. doi: 10.1016/j.semcdb.2019.06.001
Bordeleau, M., Fernández de Cossío, L., Lacabanne, C., Savage, J. C., Vernoux, N., Chakravarty, M., et al. (2021). Maternal high-fat diet modifies myelin organization, microglial interactions, and results in social memory and sensorimotor gating deficits in adolescent mouse offspring. Brain Behav. Immunity Health 15:100281. doi: 10.1016/j.bbih.2021.100281
Bordeleau, M., Lacabanne, C., Fernández de Cossío, L., Vernoux, N., Savage, J. C., González-Ibáñez, F., et al. (2020). Microglial and peripheral immune priming is partially sexually dimorphic in adolescent mouse offspring exposed to maternal high-fat diet. J. Neuroinflammation 17:264. doi: 10.1186/s12974-020-01914-1
Böttcher, C., Fernández-Zapata, C., Snijders, G. J. L., Schlickeiser, S., Sneeboer, M. A. M., Kunkel, D., et al. (2020). Single-cell mass cytometry of microglia in major depressive disorder reveals a non-inflammatory phenotype with increased homeostatic marker expression. Transl. Psychiatry 10:310. doi: 10.1038/s41398-020-00992-2
Brevet, M., Kojima, H., Asakawa, A., Atsuchi, K., Ushikai, M., Ataka, K., et al. (2010). Chronic foot-shock stress potentiates the influx of bone marrow-derived microglia into hippocampus. J. Neurosci. Res. 88, 1890–1897. doi: 10.1002/jnr.22362
Brisch, R., Steiner, J., Mawrin, C., Krzyñanowska, M., Jankowski, Z., and Gos, T. (2017). Microglia in the dorsal raphe nucleus plays a potential role in both suicide facilitation and prevention in affective disorders. Eur. Arch. Psychiatry Clin. Neurosci. 267, 403–415. doi: 10.1007/s00406-017-0774-1
Brodsky, B. S., Oquendo, M., Ellis, S. P., Haas, G. L., Malone, K. M., and Mann, J. J. (2001). The relationship of childhood abuse to impulsivity and suicidal behavior in adults with major depression. Am. J. Psychiatry 158, 1871–1877. doi: 10.1176/appi.ajp.158.11.1871
Brown, G. L., Ebert, M. H., Goyer, P. F., Jimerson, D. C., Klein, W. J., Bunney, W. E., et al. (1982). Aggression, suicide, and serotonin: relationships to CSF amine metabolites. Am. J. Psychiatry 139, 741–746. doi: 10.1176/ajp.139.6.741
Brundin, L., Bryleva, E. Y., and Thirtamara Rajamani, K. (2017). Role of inflammation in suicide: from mechanisms to treatment. Neuropsychopharmacology 42, 271–283. doi: 10.1038/npp.2016.116
Busse, M., Busse, S., Myint, A. M., Gos, T., Dobrowolny, H., Müller, U. J., et al. (2015). Decreased quinolinic acid in the hippocampus of depressive patients: evidence for local anti-inflammatory and neuroprotective responses? Eur. Arch. Psychiatry Clin. Neurosci. 265, 321–329. doi: 10.1007/s00406-014-0562-0
Cao, P., Chen, C., Liu, A., Shan, Q., Zhu, X., Jia, C., et al. (2021). Early-life inflammation promotes depressive symptoms in adolescence via microglial engulfment of dendritic spines. Neuron 109, 2573–2589.e9. doi: 10.1016/j.neuron.2021.06.012
Catale, C., Bussone, S., Lo Iacono, L., Viscomi, M. T., Palacios, D., Troisi, A., et al. (2020). Exposure to different early-life stress experiences results in differentially altered DNA methylation in the brain and immune system. Neurobiol. Stress 13:100249. doi: 10.1016/j.ynstr.2020.100249
Chastain, L. G., Franklin, T., Gangisetty, O., Cabrera, M. A., Mukherjee, S., Shrivastava, P., et al. (2019). Early life alcohol exposure primes hypothalamic microglia to later-life hypersensitivity to immune stress: possible epigenetic mechanism. Neuropsychopharmacology 44, 1579–1588. doi: 10.1038/s41386-019-0326-7
Chaudhari, N., Talwar, P., Parimisetty, A., Lefebvre d’Hellencourt, C., and Ravanan, P. (2014). A molecular web: endoplasmic reticulum stress, inflammation, and oxidative stress. Front. Cell. Neurosci. 8:213. doi: 10.3389/fncel.2014.00213
Che, Y., Wang, J.-F., Shao, L., and Young, L. T. (2010). Oxidative damage to RNA but not DNA in the hippocampus of patients with major mental illness. J. Psychiatry Neurosci. 35, 296–302. doi: 10.1503/jpn.090083
Choudhury, M. E., Miyanishi, K., Takeda, H., Islam, A., Matsuoka, N., Kubo, M., et al. (2020). Phagocytic elimination of synapses by microglia during sleep. Glia 68, 44–59. doi: 10.1002/glia.23698
Clark, S. M., Pocivavsek, A., Nicholson, J. D., Notarangelo, F. M., Langenberg, P., McMahon, R. P., et al. (2016). Reduced kynurenine pathway metabolism and cytokine expression in the prefrontal cortex of depressed individuals. J. Psychiatry Neurosci. 41, 386–394. doi: 10.1503/jpn.150226
Coccaro, E. (2006). Association of C-reactive protein elevation with trait aggression and hostility in personality disordered subjects: a pilot study. J. Psychiatric Res. 40, 460–465. doi: 10.1016/j.jpsychires.2005.04.005
Coccaro, E. F., Lee, R., and Coussons-Read, M. (2014). Elevated plasma inflammatory markers in individuals with intermittent explosive disorder and correlation with aggression in humans. JAMA Psychiatry 71:158. doi: 10.1001/jamapsychiatry.2013.3297
Conejero, I., Olié, E., Courtet, P., and Calati, R. (2018). Suicide in older adults: current perspectives. Clin. Interv. Aging 13, 691–699. doi: 10.2147/CIA.S130670
Coull, J. A. M., Beggs, S., Boudreau, D., Boivin, D., Tsuda, M., Inoue, K., et al. (2005). BDNF from microglia causes the shift in neuronal anion gradient underlying neuropathic pain. Nature 438, 1017–1021. doi: 10.1038/nature04223
Cuddapah, V. A., Zhang, S. L., and Sehgal, A. (2019). Regulation of the blood-brain barrier by circadian rhythms and sleep. Trends Neurosci. 42, 500–510. doi: 10.1016/j.tins.2019.05.001
Dantzer, R., Cohen, S., Russo, S. J., and Dinan, T. G. (2018). Resilience and immunity. Brain Behav. Immun. 74, 28–42. doi: 10.1016/j.bbi.2018.08.010
Davalos, D., Grutzendler, J., Yang, G., Kim, J. V., Zuo, Y., Jung, S., et al. (2005). ATP mediates rapid microglial response to local brain injury in vivo. Nat. Neurosci. 8, 752–758. doi: 10.1038/nn1472
Dawood, T., Anderson, J., Barton, D., Lambert, E., Esler, M., Hotchkin, E., et al. (2007). Reduced overflow of BDNF from the brain is linked with suicide risk in depressive illness. Mol. Psychiatry 12, 981–983. doi: 10.1038/sj.mp.4002059
de Kloet, E. R., Joëls, M., and Holsboer, F. (2005). Stress and the brain: from adaptation to disease. Nat. Rev. Neurosci. 6, 463–475. doi: 10.1038/nrn1683
Dean, B., Gibbons, A. S., Boer, S., Uezato, A., Meador-Woodruff, J., Scarr, E., et al. (2016). Changes in cortical N-methyl-d-aspartate receptors and post-synaptic density protein 95 in schizophrenia, mood disorders and suicide. Aust. N. Z. J. Psychiatry 50, 275–283. doi: 10.1177/0004867415586601
Deveci, A., Aydemir, O., Taskin, O., Taneli, F., and Esen-Danaci, A. (2007). Serum BDNF levels in suicide attempters related to psychosocial stressors: a comparative study with depression. NPS 56, 93–97. doi: 10.1159/000111539
Dibaj, P., Steffens, H., Nadrigny, F., Neusch, C., Kirchhoff, F., and Schomburg, E. D. (2010). Long-lasting post-mortem activity of spinal microglia in situ in mice. J. Neurosci. Res. 88, 2431–2440. doi: 10.1002/jnr.22402
Dissing-Olesen, L., LeDue, J. M., Rungta, R. L., Hefendehl, J. K., Choi, H. B., and MacVicar, B. A. (2014). Activation of neuronal NMDA receptors triggers transient ATP-mediated microglial process outgrowth. J. Neurosci. 34, 10511–10527. doi: 10.1523/JNEUROSCI.0405-14.2014
Dudek, K. A., Dion-Albert, L., Lebel, M., LeClair, K., Labrecque, S., Tuck, E., et al. (2020). Molecular adaptations of the blood–brain barrier promote stress resilience vs. depression. Proc. Natl. Acad. Sci. U.S.A. 117, 3326–3336. doi: 10.1073/pnas.1914655117
Duman, R. S., Aghajanian, G. K., Sanacora, G., and Krystal, J. H. (2016). Synaptic plasticity and depression: new insights from stress and rapid-acting antidepressants. Nat. Med. 22, 238–249. doi: 10.1038/nm.4050
Dungrawala, H., Manukyan, A., and Schneider, B. L. (2010). Gene regulation: global transcription rates scale with size. Curr. Biol. 20, R979–R981. doi: 10.1016/j.cub.2010.09.064
Dwivedi, Y. (2009). Brain-derived neurotrophic factor: role in depression and suicide. NDT 5, 433–449. doi: 10.2147/NDT.S5700
Dwivedi, Y., Rizavi, H. S., Conley, R. R., Roberts, R. C., Tamminga, C. A., and Pandey, G. N. (2003). Altered gene expression of brain-derived neurotrophic factor and receptor tyrosine kinase b in postmortem brain of suicide subjects. Arch. Gen. Psychiatry 60, 804–815. doi: 10.1001/archpsyc.60.8.804
Eisen, R. B., Perera, S., Bawor, M., Dennis, B. B., El-Sheikh, W., DeJesus, J., et al. (2016). Exploring the association between serum BDNF and attempted suicide. Sci. Rep. 6:25229. doi: 10.1038/srep25229
Erhardt, S., Lim, C. K., Linderholm, K. R., Janelidze, S., Lindqvist, D., Samuelsson, M., et al. (2013). Connecting inflammation with glutamate agonism in suicidality. Neuropsychopharmacology 38, 743–752. doi: 10.1038/npp.2012.248
Espey, M. G., Chernyshev, O. N., Reinhard, J. F., Namboodiri, M. A., and Colton, C. A. (1997). Activated human microglia produce the excitotoxin quinolinic acid. Neuroreport 8, 431–434. doi: 10.1097/00001756-199701200-00011
Eyo, U., and Dailey, M. E. (2012). Effects of oxygen-glucose deprivation on microglial mobility and viability in developing mouse hippocampal tissues. Glia 60, 1747–1760. doi: 10.1002/glia.22394
Falcone, T., Fazio, V., Lee, C., Simon, B., Franco, K., Marchi, N., et al. (2010). Serum S100B: a potential biomarker for suicidality in adolescents? PLoS One 5:e11089. doi: 10.1371/journal.pone.0011089
Ferrer, I., Martinez, A., Boluda, S., Parchi, P., and Barrachina, M. (2008). Brain banks: benefits, limitations and cautions concerning the use of post-mortem brain tissue for molecular studies. Cell Tissue Banking 9, 181–194. doi: 10.1007/s10561-008-9077-0
Filipello, F., Morini, R., Corradini, I., Zerbi, V., Canzi, A., Michalski, B., et al. (2018). The microglial innate immune receptor TREM2 is required for synapse elimination and normal brain connectivity. Immunity 48, 979–991.e8. doi: 10.1016/j.immuni.2018.04.016
Fujigaki, H., Yamamoto, Y., and Saito, K. (2017). L-Tryptophan-kynurenine pathway enzymes are therapeutic target for neuropsychiatric diseases: focus on cell type differences. Neuropharmacology 112, 264–274. doi: 10.1016/j.neuropharm.2016.01.011
Ginhoux, F., Greter, M., Leboeuf, M., Nandi, S., See, P., Gokhan, S., et al. (2010). Fate mapping analysis reveals that adult microglia derive from primitive macrophages. Science 330, 841–845. doi: 10.1126/science.1194637
Glavan, D., Gheorman, V., Gresita, A., Hermann, D. M., Udristoiu, I., and Popa-Wagner, A. (2021). Identification of transcriptome alterations in the prefrontal cortex, hippocampus, amygdala and hippocampus of suicide victims. Sci. Rep. 11:18853. doi: 10.1038/s41598-021-98210-6
Gong, Y., Chai, Y., Ding, J.-H., Sun, X.-L., and Hu, G. (2011). Chronic mild stress damages mitochondrial ultrastructure and function in mouse brain. Neurosci. Lett. 488, 76–80. doi: 10.1016/j.neulet.2010.11.006
Goodfellow, B., Kõlves, K., and Leo, D. (2019). Contemporary definitions of suicidal behavior: a systematic literature review. Suicide Life Threat Behav. 49, 488–504. doi: 10.1111/sltb.12457
Gos, T., Myint, A.-M., Schiltz, K., Meyer-Lotz, G., Dobrowolny, H., Busse, S., et al. (2014). Reduced microglial immunoreactivity for endogenous NMDA receptor agonist quinolinic acid in the hippocampus of schizophrenia patients. Brain Behav. Immun. 41, 59–64. doi: 10.1016/j.bbi.2014.05.012
Gosselin, D., Skola, D., Coufal, N. G., Holtman, I. R., Schlachetzki, J. C. M., Sajti, E., et al. (2017). An environment-dependent transcriptional network specifies human microglia identity. Science 356:eaal3222. doi: 10.1126/science.aal3222
Guerreiro, R., Wojtas, A., Bras, J., Carrasquillo, M., Rogaeva, E., Majounie, E., et al. (2013). TREM2 variants in Alzheimer’s disease. N. Engl. J. Med. 368, 117–127. doi: 10.1056/NEJMoa1211851
Györffy, B. A., Kun, J., Török, G., Bulyáki, É, Borhegyi, Z., Gulyássy, P., et al. (2018). Local apoptotic-like mechanisms underlie complement-mediated synaptic pruning. Proc. Natl. Acad. Sci. U.S.A. 115, 6303–6308. doi: 10.1073/pnas.1722613115
Hagemeyer, N., Hanft, K.-M., Akriditou, M.-A., Unger, N., Park, E. S., Stanley, E. R., et al. (2017). Microglia contribute to normal myelinogenesis and to oligodendrocyte progenitor maintenance during adulthood. Acta Neuropathol. 134, 441–458. doi: 10.1007/s00401-017-1747-1
Hanamsagar, R., and Bilbo, S. D. (2017). Environment matters: microglia function and dysfunction in a changing world. Curr. Opin. Neurobiol. 47, 146–155. doi: 10.1016/j.conb.2017.10.007
Harris, L. M., Huang, X., Linthicum, K. P., Bryen, C. P., and Ribeiro, J. D. (2020). Sleep disturbances as risk factors for suicidal thoughts and behaviours: a meta-analysis of longitudinal studies. Sci. Rep. 10:13888. doi: 10.1038/s41598-020-70866-6
Harrison, J. K., Jiang, Y., Chen, S., Xia, Y., Maciejewski, D., McNamara, R. K., et al. (1998). Role for neuronally derived fractalkine in mediating interactions between neurons and CX3CR1-expressing microglia. Proc. Natl. Acad. Sci. U.S.A. 95, 10896–10901. doi: 10.1073/pnas.95.18.10896
Haruwaka, K., Ikegami, A., Tachibana, Y., Ohno, N., Konishi, H., Hashimoto, A., et al. (2019). Dual microglia effects on blood brain barrier permeability induced by systemic inflammation. Nat. Commun. 10:5816. doi: 10.1038/s41467-019-13812-z
Haynes, S. E., Hollopeter, G., Yang, G., Kurpius, D., Dailey, M. E., Gan, W.-B., et al. (2006). The P2Y12 receptor regulates microglial activation by extracellular nucleotides. Nat. Neurosci. 9, 1512–1519. doi: 10.1038/nn1805
He, J., Hsuchou, H., He, Y., Kastin, A. J., Wang, Y., and Pan, W. (2014). Sleep restriction impairs blood-brain barrier function. J. Neurosci. 34, 14697–14706. doi: 10.1523/JNEUROSCI.2111-14.2014
Hefendehl, J. K., Neher, J. J., Sühs, R. B., Kohsaka, S., Skodras, A., and Jucker, M. (2014). Homeostatic and injury-induced microglia behavior in the aging brain. Aging Cell 13, 60–69. doi: 10.1111/acel.12149
Hellwig, S., Brioschi, S., Dieni, S., Frings, L., Masuch, A., Blank, T., et al. (2016). Altered microglia morphology and higher resilience to stress-induced depression-like behavior in CX3CR1-deficient mice. Brain Behav. Immun. 55, 126–137. doi: 10.1016/j.bbi.2015.11.008
Heng, Y., Dubbelaar, M. L., Marie, S. K. N., Boddeke, E. W. G. M., and Eggen, B. J. L. (2021). The effects of postmortem delay on mouse and human microglia gene expression. Glia 69, 1053–1060. doi: 10.1002/glia.23948
Heyes, M. P., Achim, C. L., Wiley, C. A., Major, E. O., Saito, K., and Markey, S. P. (1996). Human microglia convert l-tryptophan into the neurotoxin quinolinic acid. Biochem. J. 320, 595–597. doi: 10.1042/bj3200595
Heyes, M. P., Saito, K., and Markey, S. P. (1992). Human macrophages convert L-tryptophan into the neurotoxin quinolinic acid. Biochem. J. 283, 633–635. doi: 10.1042/bj2830633
Hinwood, M., Morandini, J., Day, T. A., and Walker, F. R. (2012). Evidence that microglia mediate the neurobiological effects of chronic psychological stress on the medial prefrontal cortex. Cereb. Cortex 22, 1442–1454. doi: 10.1093/cercor/bhr229
Hodes, G. E., Pfau, M. L., Leboeuf, M., Golden, S. A., Christoffel, D. J., Bregman, D., et al. (2014). Individual differences in the peripheral immune system promote resilience versus susceptibility to social stress. Proc. Natl. Acad. Sci. U.S.A. 111, 16136–16141. doi: 10.1073/pnas.1415191111
Hoeffel, G., Chen, J., Lavin, Y., Low, D., Almeida, F. F., See, P., et al. (2015). C-Myb(+) erythro-myeloid progenitor-derived fetal monocytes give rise to adult tissue-resident macrophages. Immunity 42, 665–678. doi: 10.1016/j.immuni.2015.03.011
Holmes, S. E., Hinz, R., Conen, S., Gregory, C. J., Matthews, J. C., Anton-Rodriguez, J. M., et al. (2018). Elevated translocator protein in anterior cingulate in major depression and a role for inflammation in suicidal thinking: a positron emission tomography study. Biol. Psychiatry 83, 61–69. doi: 10.1016/j.biopsych.2017.08.005
Holmes, S. E., Scheinost, D., Finnema, S. J., Naganawa, M., Davis, M. T., DellaGioia, N., et al. (2019). Lower synaptic density is associated with depression severity and network alterations. Nat. Commun. 10:1529. doi: 10.1038/s41467-019-09562-7
Honarpisheh, P., Lee, J., Banerjee, A., Blasco-Conesa, M. P., Honarpisheh, P., d’Aigle, J., et al. (2020). Potential caveats of putative microglia-specific markers for assessment of age-related cerebrovascular neuroinflammation. J. Neuroinflammation 17:366. doi: 10.1186/s12974-020-02019-5
Hoshiko, M., Arnoux, I., Avignone, E., Yamamoto, N., and Audinat, E. (2012). Deficiency of the microglial receptor CX3CR1 impairs postnatal functional development of thalamocortical synapses in the barrel cortex. J. Neurosci. 32, 15106–15111. doi: 10.1523/JNEUROSCI.1167-12.2012
Hughes, A. N., and Appel, B. (2020). Microglia phagocytose myelin sheaths to modify developmental myelination. Nat. Neurosci. 23, 1055–1066. doi: 10.1038/s41593-020-0654-2
Jonsson, T., Stefansson, H., Steinberg, S., Jonsdottir, I., Jonsson, P. V., Snaedal, J., et al. (2013). Variant of TREM2 associated with the risk of Alzheimer’s disease. N. Engl. J. Med. 368, 107–116. doi: 10.1056/NEJMoa1211103
Joost, E., Jordão, M. J. C., Mages, B., Prinz, M., Bechmann, I., and Krueger, M. (2019). Microglia contribute to the glia limitans around arteries, capillaries and veins under physiological conditions, in a model of neuroinflammation and in human brain tissue. Brain Struct. Funct. 224, 1301–1314. doi: 10.1007/s00429-019-01834-8
Kang, H. J., Voleti, B., Hajszan, T., Rajkowska, G., Stockmeier, C. A., Licznerski, P., et al. (2012). Decreased expression of synapse-related genes and loss of synapses in major depressive disorder. Nat. Med. 18, 1413–1417. doi: 10.1038/nm.2886
Ke, X., Fu, Q., Majnik, A., Cohen, S., Liu, Q., and Lane, R. (2018). Adverse early life environment induces anxiety-like behavior and increases expression of FKBP5 mRNA splice variants in mouse brain. Physiol. Genomics 50, 973–981. doi: 10.1152/physiolgenomics.00054.2018
Kierdorf, K., Erny, D., Goldmann, T., Sander, V., Schulz, C., Perdiguero, E. G., et al. (2013). Microglia emerge from erythromyeloid precursors via Pu.1- and Irf8-dependent pathways. Nat. Neurosci. 16, 273–280. doi: 10.1038/nn.3318
Kim, K., Kim, S.-W., Myung, W., Han, C. E., Fava, M., Mischoulon, D., et al. (2017). Reduced orbitofrontal-thalamic functional connectivity related to suicidal ideation in patients with major depressive disorder. Sci. Rep. 7:15772. doi: 10.1038/s41598-017-15926-0
Kim, Y.-K., Lee, H.-P., Won, S.-D., Park, E.-Y., Lee, H.-Y., Lee, B.-H., et al. (2007). Low plasma BDNF is associated with suicidal behavior in major depression. Prog. Neuro Psychopharmacol. Biol. Psychiatry 31, 78–85. doi: 10.1016/j.pnpbp.2006.06.024
Koweszko, T., Gierus, J., Zalewska, A., Maciejczyk, M., Waszkiewicz, N., and Szulc, A. (2020). The relationship between suicide and oxidative stress in a group of psychiatric inpatients. JCM 9:3462. doi: 10.3390/jcm9113462
Kozlowski, C., and Weimer, R. M. (2012). An automated method to quantify microglia morphology and application to monitor activation state longitudinally in vivo. PLoS One 7:e31814. doi: 10.1371/journal.pone.0031814
Krabbe, G., Matyash, V., Pannasch, U., Mamer, L., Boddeke, H. W. G. M., and Kettenmann, H. (2012). Activation of serotonin receptors promotes microglial injury-induced motility but attenuates phagocytic activity. Brain Behav. Immun. 26, 419–428. doi: 10.1016/j.bbi.2011.12.002
Krasemann, S., Madore, C., Cialic, R., Baufeld, C., Calcagno, N., El Fatimy, R., et al. (2017). The TREM2-APOE pathway drives the transcriptional phenotype of dysfunctional microglia in neurodegenerative diseases. Immunity 47, 566–581.e9. doi: 10.1016/j.immuni.2017.08.008
Kreisel, T., Frank, M. G., Licht, T., Reshef, R., Ben-Menachem-Zidon, O., Baratta, M. V., et al. (2014). Dynamic microglial alterations underlie stress-induced depressive-like behavior and suppressed neurogenesis. Mol. Psychiatry 19, 699–709. doi: 10.1038/mp.2013.155
Lahti, A. C., Weiler, M. A., Tamara Michaelidis, B. A., Parwani, A., and Tamminga, C. A. (2001). Effects of ketamine in normal and schizophrenic volunteers. Neuropsychopharmacology 25, 455–467. doi: 10.1016/S0893-133X(01)00243-3
Lampron, A., Larochelle, A., Laflamme, N., Préfontaine, P., Plante, M.-M., Sánchez, M. G., et al. (2015). Inefficient clearance of myelin debris by microglia impairs remyelinating processes. J. Exp. Med. 212, 481–495. doi: 10.1084/jem.20141656
Lee, B.-H., Kim, H., Park, S.-H., and Kim, Y.-K. (2007). Decreased plasma BDNF level in depressive patients. J. Affect. Disord. 101, 239–244. doi: 10.1016/j.jad.2006.11.005
Lee, J.-S., Lee, S.-B., Kim, D.-W., Shin, N., Jeong, S.-J., Yang, C.-H., et al. (2021). Social isolation–related depression accelerates ethanol intake via microglia-derived neuroinflammation. Sci. Adv. 7:eabj3400. doi: 10.1126/sciadv.abj3400
Lehmann, M. L., Cooper, H. A., Maric, D., and Herkenham, M. (2016). Social defeat induces depressive-like states and microglial activation without involvement of peripheral macrophages. J. Neuroinflammation 13:224. doi: 10.1186/s12974-016-0672-x
Lehmann, M. L., Weigel, T. K., Cooper, H. A., Elkahloun, A. G., Kigar, S. L., and Herkenham, M. (2018). Decoding microglia responses to psychosocial stress reveals blood-brain barrier breakdown that may drive stress susceptibility. Sci. Rep. 8:11240. doi: 10.1038/s41598-018-28737-8
Lehmann, M. L., Weigel, T. K., Poffenberger, C. N., and Herkenham, M. (2019). The behavioral sequelae of social defeat require microglia and are driven by oxidative stress in mice. J. Neurosci. 39, 5594–5605. doi: 10.1523/JNEUROSCI.0184-19.2019
Linnartz, B., Kopatz, J., Tenner, A. J., and Neumann, H. (2012). Sialic acid on the neuronal glycocalyx prevents complement C1 binding and complement receptor-3-mediated removal by microglia. J. Neurosci. 32, 946–952. doi: 10.1523/JNEUROSCI.3830-11.2012
Lituma, P. J., Woo, E., O’Hara, B. F., Castillo, P. E., Sibinga, N. E. S., and Nandi, S. (2021). Altered synaptic connectivity and brain function in mice lacking microglial adapter protein Iba1. Proc. Natl. Acad. Sci. U.S.A. 118:e2115539118. doi: 10.1073/pnas.2115539118
Liu, X., Nemeth, D. P., McKim, D. B., Zhu, L., DiSabato, D. J., Berdysz, O., et al. (2019). Cell-type-specific interleukin 1 receptor 1 signaling in the brain regulates distinct neuroimmune activities. Immunity 50, 317–333.e6. doi: 10.1016/j.immuni.2018.12.012
Lou, N., Takano, T., Pei, Y., Xavier, A. L., Goldman, S. A., and Nedergaard, M. (2016). Purinergic receptor P2RY12-dependent microglial closure of the injured blood-brain barrier. Proc. Natl. Acad. Sci. U.S.A. 113, 1074–1079. doi: 10.1073/pnas.1520398113
Lucassen, P. J., Pruessner, J., Sousa, N., Almeida, O. F. X., Van Dam, A. M., Rajkowska, G., et al. (2014). Neuropathology of stress. Acta Neuropathol. 127, 109–135. doi: 10.1007/s00401-013-1223-5
Lutz, P.-E., Mechawar, N., and Turecki, G. (2017). Neuropathology of suicide: recent findings and future directions. Mol. Psychiatry 22, 1395–1412. doi: 10.1038/mp.2017.141
Mann, J. J., McBride, P. A., Brown, R. P., Linnoila, M., Leon, A. C., DeMeo, M., et al. (1992). Relationship between central and peripheral serotonin indexes in depressed and suicidal psychiatric inpatients. Arch. Gen. Psychiatry 49, 442–446. doi: 10.1001/archpsyc.1992.01820060022003
Marguerat, S., and Bähler, J. (2012). Coordinating genome expression with cell size. Trends Genet. 28, 560–565. doi: 10.1016/j.tig.2012.07.003
Martins-de-Souza, D., Guest, P. C., Harris, L. W., Vanattou-Saifoudine, N., Webster, M. J., Rahmoune, H., et al. (2012). Identification of proteomic signatures associated with depression and psychotic depression in post-mortem brains from major depression patients. Transl. Psychiatry 2:e87. doi: 10.1038/tp.2012.13
Masuda, T., Sankowski, R., Staszewski, O., and Prinz, M. (2020). Microglia heterogeneity in the single-cell era. Cell Rep. 30, 1271–1281. doi: 10.1016/j.celrep.2020.01.010
McEwen, B. S., and Magarinos, A. M. (2001). Stress and hippocampal plasticity: implications for the pathophysiology of affective disorders. Hum. Psychopharmacol. 16, S7–S19. doi: 10.1002/hup.266
McKim, D. B., Weber, M. D., Niraula, A., Sawicki, C. M., Liu, X., Jarrett, B. L., et al. (2018). Microglial recruitment of IL-1β-producing monocytes to brain endothelium causes stress-induced anxiety. Mol. Psychiatry 23, 1421–1431. doi: 10.1038/mp.2017.64
Ménard, C., Pfau, M. L., Hodes, G. E., and Russo, S. J. (2017). Immune and neuroendocrine mechanisms of stress vulnerability and resilience. Neuropsychopharmacology 42, 62–80. doi: 10.1038/npp.2016.90
Menard, C., Pfau, M. L., Hodes, G. E., Kana, V., Wang, V. X., Bouchard, S., et al. (2017). Social stress induces neurovascular pathology promoting depression. Nat. Neurosci. 20, 1752–1760. doi: 10.1038/s41593-017-0010-3
Menke, A., Lehrieder, D., Fietz, J., Leistner, C., Wurst, C., Stonawski, S., et al. (2018). Childhood trauma dependent anxious depression sensitizes HPA axis function. Psychoneuroendocrinology 98, 22–29. doi: 10.1016/j.psyneuen.2018.07.025
Milior, G., Lecours, C., Samson, L., Bisht, K., Poggini, S., Pagani, F., et al. (2016). Fractalkine receptor deficiency impairs microglial and neuronal responsiveness to chronic stress. Brain Behav. Immun. 55, 114–125. doi: 10.1016/j.bbi.2015.07.024
Miyamoto, A., Wake, H., Ishikawa, A. W., Eto, K., Shibata, K., Murakoshi, H., et al. (2016). Microglia contact induces synapse formation in developing somatosensory cortex. Nat. Commun. 7:12540. doi: 10.1038/ncomms12540
Mondelli, V., Vernon, A. C., Turkheimer, F., Dazzan, P., and Pariante, C. M. (2017). Brain microglia in psychiatric disorders. Lancet Psychiatry 4, 563–572. doi: 10.1016/S2215-0366(17)30101-3
Murai, N., Mitalipova, M., and Jaenisch, R. (2020). Functional analysis of CX3CR1 in human induced pluripotent stem (iPS) cell-derived microglia-like cells. Eur. J. Neurosci. 52, 3667–3678. doi: 10.1111/ejn.14879
Nakazawa, K., Zsiros, V., Jiang, Z., Nakao, K., Kolata, S., Zhang, S., et al. (2012). GABAergic interneuron origin of schizophrenia pathophysiology. Neuropharmacology 62, 1574–1583. doi: 10.1016/j.neuropharm.2011.01.022
Neher, J. J., and Cunningham, C. (2019). Priming microglia for innate immune memory in the brain. Trends Immunol. 40, 358–374. doi: 10.1016/j.it.2019.02.001
Nguyen, P. T., Dorman, L. C., Pan, S., Vainchtein, I. D., Han, R. T., Nakao-Inoue, H., et al. (2020). Microglial remodeling of the extracellular matrix promotes synapse plasticity. Cell 182, 388–403.e15. doi: 10.1016/j.cell.2020.05.050
Ni, H., Xu, M., Zhan, G.-L., Fan, Y., Zhou, H., Jiang, H.-Y., et al. (2018). The GWAS risk genes for depression may be actively involved in Alzheimer’s Disease. J. Alzheimers Dis. 64, 1149–1161. doi: 10.3233/JAD-180276
Nock, M. K. (2014). The Oxford Handbook of Suicide and Self-Injury. Oxford: Oxford University Press.
Nold, V., Richter, N., Hengerer, B., Kolassa, I.-T., and Allers, K. A. (2021). FKBP5 polymorphisms induce differential glucocorticoid responsiveness in primary CNS cells – First insights from novel humanized mice. Eur. J. Neurosci. 53, 402–415. doi: 10.1111/ejn.14999
Nonaka, S., and Nakanishi, H. (2019). Microglial clearance of focal apoptotic synapses. Neurosci. Lett. 707:134317. doi: 10.1016/j.neulet.2019.134317
Nutma, E., Stephenson, J. A., Gorter, R. P., de Bruin, J., Boucherie, D. M., Donat, C. K., et al. (2019). A quantitative neuropathological assessment of translocator protein expression in multiple sclerosis. Brain 142, 3440–3455. doi: 10.1093/brain/awz287
O’Connor, J. C., Lawson, M. A., André, C., Moreau, M., Lestage, J., Castanon, N., et al. (2009). Lipopolysaccharide-induced depressive-like behavior is mediated by indoleamine 2,3-dioxygenase activation in mice. Mol. Psychiatry 14, 511–522. doi: 10.1038/sj.mp.4002148
O’Connor, M. J., Portnoff, L. C., Lebsack-Coleman, M., and Dipple, K. M. (2019). Suicide risk in adolescents with fetal alcohol spectrum disorders. Birth Defects Res. 111, 822–828. doi: 10.1002/bdr2.1465
Ohsawa, K., Imai, Y., Kanazawa, H., Sasaki, Y., and Kohsaka, S. (2000). Involvement of Iba1 in membrane ruffling and phagocytosis of macrophages/microglia. J. Cell Sci. 113(Pt 17), 3073–3084. doi: 10.1242/jcs.113.17.3073
Olloquequi, J., Cornejo-Córdova, E., Verdaguer, E., Soriano, F. X., Binvignat, O., Auladell, C., et al. (2018). Excitotoxicity in the pathogenesis of neurological and psychiatric disorders: therapeutic implications. J. Psychopharmacol. 32, 265–275. doi: 10.1177/0269881118754680
Pan, L. A., Hassel, S., Segreti, A. M., Nau, S. A., Brent, D. A., and Phillips, M. L. (2013). Differential patterns of activity and functional connectivity in emotion processing neural circuitry to angry and happy faces in adolescents with and without suicide attempt. Psychol. Med. 43, 2129–2142. doi: 10.1017/S0033291712002966
Pandey, G. N., Rizavi, H. S., Ren, X., Bhaumik, R., and Dwivedi, Y. (2014). Toll-like receptors in the depressed and suicide brain. J. Psychiatr. Res. 53, 62–68. doi: 10.1016/j.jpsychires.2014.01.021
Pannell, M., Economopoulos, V., Wilson, T. C., Kersemans, V., Isenegger, P. G., Larkin, J. R., et al. (2020). Imaging of translocator protein upregulation is selective for pro-inflammatory polarized astrocytes and microglia. Glia 68, 280–297. doi: 10.1002/glia.23716
Pantazatos, S. P., Huang, Y.-Y., Rosoklija, G. B., Dwork, A. J., Arango, V., and Mann, J. J. (2017). Whole-transcriptome brain expression and exon-usage profiling in major depression and suicide: evidence for altered glial, endothelial and ATPase activity. Mol. Psychiatry 22, 760–773. doi: 10.1038/mp.2016.130
Paolicelli, R. C., Bisht, K., and Tremblay, M. -È (2014). Fractalkine regulation of microglial physiology and consequences on the brain and behavior. Front. Cell Neurosci. 8:129. doi: 10.3389/fncel.2014.00129
Parellada, E., and Gassó, P. (2021). Glutamate and microglia activation as a driver of dendritic apoptosis: a core pathophysiological mechanism to understand schizophrenia. Transl. Psychiatry 11:271. doi: 10.1038/s41398-021-01385-9
Parkhurst, C. N., Yang, G., Ninan, I., Savas, J. N., Yates, J. R., Lafaille, J. J., et al. (2013). Microglia promote learning-dependent synapse formation through brain-derived neurotrophic factor. Cell 155, 1596–1609. doi: 10.1016/j.cell.2013.11.030
Peferoen, L. A. N., Vogel, D. Y. S., Ummenthum, K., Breur, M., Heijnen, P. D. A. M., Gerritsen, W. H., et al. (2015). Activation status of human microglia is dependent on lesion formation stage and remyelination in multiple sclerosis. J. Neuropathol. Exp. Neurol. 74, 48–63. doi: 10.1097/NEN.0000000000000149
Petrasch-Parwez, E., Schöbel, A., Benali, A., Moinfar, Z., Förster, E., Brüne, M., et al. (2020). Lateralization of increased density of Iba1-immunopositive microglial cells in the anterior midcingulate cortex of schizophrenia and bipolar disorder. Eur. Arch. Psychiatry Clin. Neurosci. 270, 819–828. doi: 10.1007/s00406-020-01107-0
Picard, K., St-Pierre, M.-K., Vecchiarelli, H. A., Bordeleau, M., and Tremblay, M. -È (2021). Neuroendocrine, neuroinflammatory and pathological outcomes of chronic stress: a story of microglial remodeling. Neurochem. Int. 145:104987. doi: 10.1016/j.neuint.2021.104987
Prinz, M., Masuda, T., Wheeler, M. A., and Quintana, F. J. (2021). Microglia and central nervous system-associated macrophages-from origin to disease modulation. Annu. Rev. Immunol. 39, 251–277. doi: 10.1146/annurev-immunol-093019-110159
Qiao, H., Li, M.-X., Xu, C., Chen, H.-B., An, S.-C., and Ma, X.-M. (2016). Dendritic spines in depression: what we learned from animal models. Neural Plast. 2016:8056370. doi: 10.1155/2016/8056370
Raison, C. L., Capuron, L., and Miller, A. H. (2006). Cytokines sing the blues: inflammation and the pathogenesis of depression. Trends Immunol. 27, 24–31. doi: 10.1016/j.it.2005.11.006
Ren, S., Breuillaud, L., Yao, W., Yin, T., Norris, K. A., Zehntner, S. P., et al. (2021). TNF-α–mediated reduction in inhibitory neurotransmission precedes sporadic Alzheimer’s disease pathology in young Trem2 rats. J. Biol. Chem. 296:100089. doi: 10.1074/jbc.RA120.016395
Réus, G. Z., Fries, G. R., Stertz, L., Badawy, M., Passos, I. C., Barichello, T., et al. (2015). The role of inflammation and microglial activation in the pathophysiology of psychiatric disorders. Neuroscience 300, 141–154. doi: 10.1016/j.neuroscience.2015.05.018
Rimmerman, N., Schottlender, N., Reshef, R., Dan-Goor, N., and Yirmiya, R. (2017). The hippocampal transcriptomic signature of stress resilience in mice with microglial fractalkine receptor (CX3CR1) deficiency. Brain Behav. Immun. 61, 184–196. doi: 10.1016/j.bbi.2016.11.023
Robertson, K. D. (2002). DNA methylation and chromatin – unraveling the tangled web. Oncogene 21, 5361–5379. doi: 10.1038/sj.onc.1205609
Rogers, J. T., Morganti, J. M., Bachstetter, A. D., Hudson, C. E., Peters, M. M., Grimmig, B. A., et al. (2011). CX3CR1 deficiency leads to impairment of hippocampal cognitive function and synaptic plasticity. J. Neurosci. 31, 16241–16250. doi: 10.1523/JNEUROSCI.3667-11.2011
Roy, B., Shelton, R. C., and Dwivedi, Y. (2017). DNA methylation and expression of stress related genes in PBMC of MDD patients with and without serious suicidal ideation. J. Psychiatr. Res. 89, 115–124. doi: 10.1016/j.jpsychires.2017.02.005
Russo, S. J., Murrough, J. W., Han, M.-H., Charney, D. S., and Nestler, E. J. (2012). Neurobiology of resilience. Nat. Neurosci. 15, 1475–1484. doi: 10.1038/nn.3234
Santos, L. E., Beckman, D., and Ferreira, S. T. (2016). Microglial dysfunction connects depression and Alzheimer’s disease. Brain Behav. Immun. 55, 151–165. doi: 10.1016/j.bbi.2015.11.011
Savage, J. C., Carrier, M., and Tremblay, M. -È (2019). Morphology of microglia across contexts of health and disease. Methods Mol. Biol. 2034, 13–26. doi: 10.1007/978-1-4939-9658-2_2
Schafer, D. P., Lehrman, E. K., Kautzman, A. G., Koyama, R., Mardinly, A. R., Yamasaki, R., et al. (2012). Microglia sculpt postnatal neural circuits in an activity and complement-dependent manner. Neuron 74, 691–705. doi: 10.1016/j.neuron.2012.03.026
Schiavone, S., Neri, M., Mhillaj, E., Morgese, M. G., Cantatore, S., Bove, M., et al. (2016). The NADPH oxidase NOX2 as a novel biomarker for suicidality: evidence from human post mortem brain samples. Transl. Psychiatry 6:e813. doi: 10.1038/tp.2016.76
Schmaal, L., van Harmelen, A.-L., Chatzi, V., Lippard, E. T. C., Toenders, Y. J., Averill, L. A., et al. (2020). Imaging suicidal thoughts and behaviors: a comprehensive review of 2 decades of neuroimaging studies. Mol. Psychiatry 25, 408–427. doi: 10.1038/s41380-019-0587-x
Schneider, E., El Hajj, N., Müller, F., Navarro, B., and Haaf, T. (2015). Epigenetic dysregulation in the prefrontal cortex of suicide completers. Cytogenet. Genome Res. 146, 19–27. doi: 10.1159/000435778
Schnieder, T. P., Trencevska, I., Rosoklija, G., Stankov, A., Mann, J. J., Smiley, J., et al. (2014). Microglia of prefrontal white matter in suicide. J. Neuropathol. Exp. Neurol. 73, 880–890. doi: 10.1097/NEN.0000000000000107
Schnieder, T. P., Zhou Qin, I. D., Trencevska-Ivanovska, I., Rosoklija, G., Stankov, A., Pavlovski, G., et al. (2019). Blood vessels and perivascular phagocytes of prefrontal white and gray matter in suicide. J. Neuropathol. Exp. Neurol. 78, 15–30. doi: 10.1093/jnen/nly103
Schobel, S. A., Chaudhury, N. H., Khan, U. A., Paniagua, B., Styner, M. A., Asllani, I., et al. (2013). Imaging patients with psychosis and a mouse model establishes a spreading pattern of hippocampal dysfunction and implicates glutamate as a driver. Neuron 78, 81–93. doi: 10.1016/j.neuron.2013.02.011
Seidler, Z. E., Wilson, M. J., Oliffe, J. L., Kealy, D., Toogood, N., Ogrodniczuk, J. S., et al. (2021). “Eventually, I Admitted, ‘I Cannot Do This Alone”’: exploring experiences of suicidality and help-seeking drivers among australian men. Front. Sociol. 6:727069. doi: 10.3389/fsoc.2021.727069
Sellgren, C. M., Gracias, J., Watmuff, B., Biag, J. D., Thanos, J. M., Whittredge, P. B., et al. (2019). Increased synapse elimination by microglia in schizophrenia patient-derived models of synaptic pruning. Nat. Neurosci. 22, 374–385. doi: 10.1038/s41593-018-0334-7
Serafini, G., Parisi, V. M., Aguglia, A., Amerio, A., Sampogna, G., Fiorillo, A., et al. (2020). A specific inflammatory profile underlying suicide risk? systematic review of the main literature findings. IJERPH 17:2393. doi: 10.3390/ijerph17072393
Setiawan, E., Wilson, A. A., Mizrahi, R., Rusjan, P. M., Miler, L., Rajkowska, G., et al. (2015). Role of translocator protein density, a marker of neuroinflammation, in the brain during major depressive episodes. JAMA Psychiatry 72, 268–275. doi: 10.1001/jamapsychiatry.2014.2427
Sierra, A., Encinas, J. M., Deudero, J. J. P., Chancey, J. H., Enikolopov, G., Overstreet-Wadiche, L. S., et al. (2010). Microglia shape adult hippocampal neurogenesis through apoptosis-coupled phagocytosis. Cell Stem Cell 7, 483–495. doi: 10.1016/j.stem.2010.08.014
Šimončičová, E., Gonçalves de Andrade, E., Vecchiarelli, H. A., Awogbindin, I. O., Delage, C. I, and Tremblay, M. -È (2022). Present and future of microglial pharmacology. Trends Pharmacol. Sci. doi: 10.1016/j.tips.2021.11.006 [Epub ahead of print].
Sipe, G. O., Lowery, R. L., Tremblay, M. -È, Kelly, E. A., Lamantia, C. E., and Majewska, A. K. (2016). Microglial P2Y12 is necessary for synaptic plasticity in mouse visual cortex. Nat. Commun. 7:10905. doi: 10.1038/ncomms10905
Smith, A. K., Simon, J. S., Gustafson, E. L., Noviello, S., Cubells, J. F., Epstein, M. P., et al. (2012). Association of a polymorphism in the indoleamine- 2,3-dioxygenase gene and interferon-α-induced depression in patients with chronic hepatitis C. Mol. Psychiatry 17, 781–789. doi: 10.1038/mp.2011.67
Smith, K. E., and Pollak, S. D. (2020). Early life stress and development: potential mechanisms for adverse outcomes. J. Neurodevelop. Disord. 12:34. doi: 10.1186/s11689-020-09337-y
Snijders, G. J. L. J., Sneeboer, M. A. M., Fernández-Andreu, A., Udine, E., Boks, M. P., Ormel, P. R., et al. (2020). Distinct non-inflammatory signature of microglia in post-mortem brain tissue of patients with major depressive disorder. Mol. Psychiatry 26, 3336–3349. doi: 10.1038/s41380-020-00896-z
Stanley, M., and Mann, J. J. (1983). Increased serotonin-2 binding sites in frontal cortex of suicide victims. Lancet 1, 214–216. doi: 10.1016/s0140-6736(83)92590-4
Stanley, M., Virgilio, J., and Gershon, S. (1982). Tritiated imipramine binding sites are decreased in the frontal cortex of suicides. Science 216, 1337–1339. doi: 10.1126/science.7079769
Steiner, J., Bielau, H., Brisch, R., Danos, P., Ullrich, O., Mawrin, C., et al. (2008). Immunological aspects in the neurobiology of suicide: elevated microglial density in schizophrenia and depression is associated with suicide. J. Psychiatr. Res. 42, 151–157. doi: 10.1016/j.jpsychires.2006.10.013
Steiner, J., Bogerts, B., Sarnyai, Z., Walter, M., Gos, T., Bernstein, H.-G., et al. (2012). Bridging the gap between the immune and glutamate hypotheses of schizophrenia and major depression: Potential role of glial NMDA receptor modulators and impaired blood-brain barrier integrity. World J. Biol. Psychiatry 13, 482–492. doi: 10.3109/15622975.2011.583941
Steiner, J., Mawrin, C., Ziegeler, A., Bielau, H., Ullrich, O., Bernstein, H.-G., et al. (2006). Distribution of HLA-DR-positive microglia in schizophrenia reflects impaired cerebral lateralization. Acta Neuropathol. 112, 305–316. doi: 10.1007/s00401-006-0090-8
Steiner, J., Walter, M., Gos, T., Guillemin, G. J., Bernstein, H.-G., Sarnyai, Z., et al. (2011). Severe depression is associated with increased microglial quinolinic acid in subregions of the anterior cingulate gyrus: evidence for an immune-modulated glutamatergic neurotransmission? J. Neuroinflammation 8:94. doi: 10.1186/1742-2094-8-94
Stone, J. M., Morrison, P. D., and Pilowsky, L. S. (2007). Glutamate and dopamine dysregulation in schizophrenia–a synthesis and selective review. J. Psychopharmacol. 21, 440–452. doi: 10.1177/0269881106073126
Stowell, R. D., Wong, E. L., Batchelor, H. N., Mendes, M. S., Lamantia, C. E., Whitelaw, B. S., et al. (2018). Cerebellar microglia are dynamically unique and survey Purkinje neurons in vivo. Dev. Neurobiol. 78, 627–644. doi: 10.1002/dneu.22572
St-Pierre, M.-K., Šimončičová, E., Bögi, E., and Tremblay, M. -È (2020). Shedding light on the dark side of the microglia. ASN Neuro 12:1759091420925335. doi: 10.1177/1759091420925335
Stratoulias, V., Venero, J. L., and Tremblay, M. -È, and Joseph, B. (2019). Microglial subtypes: diversity within the microglial community. EMBO J. 38:e101997. doi: 10.15252/embj.2019101997
Straub, R. E., Lipska, B. K., Egan, M. F., Goldberg, T. E., Callicott, J. H., Mayhew, M. B., et al. (2007). Allelic variation in GAD1 (GAD67) is associated with schizophrenia and influences cortical function and gene expression. Mol. Psychiatry 12, 854–869. doi: 10.1038/sj.mp.4001988
Stremmel, C., Schuchert, R., Wagner, F., Thaler, R., Weinberger, T., Pick, R., et al. (2018). Yolk sac macrophage progenitors traffic to the embryo during defined stages of development. Nat. Commun. 9:75. doi: 10.1038/s41467-017-02492-2
Suzuki, H., Ohgidani, M., Kuwano, N., Chrétien, F., Lorin de la Grandmaison, G., Onaya, M., et al. (2019). Suicide and microglia: recent findings and future perspectives based on human studies. Front. Cell. Neurosci. 13:31. doi: 10.3389/fncel.2019.00031
Swanson, M. E. V., Murray, H. C., Ryan, B., Faull, R. L. M., Dragunow, M., and Curtis, M. A. (2020). Quantitative immunohistochemical analysis of myeloid cell marker expression in human cortex captures microglia heterogeneity with anatomical context. Sci. Rep. 10:11693. doi: 10.1038/s41598-020-68086-z
Takahashi, K., Rochford, C. D. P., and Neumann, H. (2005). Clearance of apoptotic neurons without inflammation by microglial triggering receptor expressed on myeloid cells-2. J. Exp. Med. 201, 647–657. doi: 10.1084/jem.20041611
Tan, Y.-L., Yuan, Y., and Tian, L. (2020). Microglial regional heterogeneity and its role in the brain. Mol. Psychiatry 25, 351–367. doi: 10.1038/s41380-019-0609-8
Tay, T. L., Béchade, C., D’Andrea, I., St-Pierre, M.-K., Henry, M. S., Roumier, A., et al. (2018). Microglia gone rogue: impacts on psychiatric disorders across the lifespan. Front. Mol. Neurosci. 10:421. doi: 10.3389/fnmol.2017.00421
Tay, T. L., Savage, J. C., Hui, C. W., Bisht, K., and Tremblay, M. -È (2017). Microglia across the lifespan: from origin to function in brain development, plasticity and cognition. J. Physiol. 595, 1929–1945. doi: 10.1113/JP272134
Torres-Platas, S. G., Cruceanu, C., Chen, G. G., Turecki, G., and Mechawar, N. (2014b). Evidence for increased microglial priming and macrophage recruitment in the dorsal anterior cingulate white matter of depressed suicides. Brain Behav. Immunity 42, 50–59. doi: 10.1016/j.bbi.2014.05.007
Torres-Platas, S. G., Comeau, S., Rachalski, A., Bo, G. D., Cruceanu, C., Turecki, G., et al. (2014a). Morphometric characterization of microglial phenotypes in human cerebral cortex. J. Neuroinflammation 11:12. doi: 10.1186/1742-2094-11-12
Trapp, B. D., Wujek, J. R., Criste, G. A., Jalabi, W., Yin, X., Kidd, G. J., et al. (2007). Evidence for synaptic stripping by cortical microglia. Glia 55, 360–368. doi: 10.1002/glia.20462
Tremblay, M. È (2021). Microglial functional alteration and increased diversity in the challenged brain: Insights into novel targets for intervention. Brain Behav. Immunity Health 16:100301. doi: 10.1016/j.bbih.2021.100301
Tsirigotis, K., Gruszczynski, W., and Tsirigotis, M. (2011). Gender differentiation in methods of suicide attempts. Med. Sci. Monit. 17, H65–H70. doi: 10.12659/msm.881887
Turecki, G., Brent, D. A., Gunnell, D., O’Connor, R. C., Oquendo, M. A., Pirkis, J., et al. (2019). Suicide and suicide risk. Nat. Rev. Dis. Primers 5:74. doi: 10.1038/s41572-019-0121-0
Turecki, G., Ota, V. K., Belangero, S. I., Jackowski, A., and Kaufman, J. (2014). Early life adversity, genomic plasticity, and psychopathology. Lancet Psychiatry 1, 461–466. doi: 10.1016/S2215-0366(14)00022-4
Turkin, A., Tuchina, O., and Klempin, F. (2021). Microglia function on precursor cells in the adult hippocampus and their responsiveness to serotonin signaling. Front. Cell Dev. Biol. 9:665739. doi: 10.3389/fcell.2021.665739
Tynan, R. J., Naicker, S., Hinwood, M., Nalivaiko, E., Buller, K. M., Pow, D. V., et al. (2010). Chronic stress alters the density and morphology of microglia in a subset of stress-responsive brain regions. Brain Behav. Immun. 24, 1058–1068. doi: 10.1016/j.bbi.2010.02.001
Ulland, T. K., and Colonna, M. (2018). TREM2 – a key player in microglial biology and Alzheimer disease. Nat. Rev. Neurol. 14, 667–675. doi: 10.1038/s41582-018-0072-1
Underwood, M. D., and Arango, V. (2011). Evidence for neurodegeneration and neuroplasticity as part of the neurobiology of suicide. Biol. Psychiatry 70, 306–307. doi: 10.1016/j.biopsych.2011.06.004
Underwood, M. D., Bakalian, M. J., Johnson, V. L., Kassir, S. A., Ellis, S. P., Mann, J. J., et al. (2020). Less NMDA receptor binding in dorsolateral prefrontal cortex and anterior cingulate cortex associated with reported early-life adversity but not suicide. Int. J. Neuropsychopharmacol. 23, 311–318. doi: 10.1093/ijnp/pyaa009
Underwood, M. D., Kassir, S. A., Bakalian, M. J., Galfalvy, H., Mann, J. J., and Arango, V. (2012). Neuron density and serotonin receptor binding in prefrontal cortex in suicide. Int. J. Neuropsychopharmacol. 15, 435–447. doi: 10.1017/S1461145711000691
Uranova, N. A., Vikhreva, O. V., Rakhmanova, V. I., and Orlovskaya, D. D. (2018). Ultrastructural pathology of oligodendrocytes adjacent to microglia in prefrontal white matter in schizophrenia. NPJ Schizophr. 4:26. doi: 10.1038/s41537-018-0068-2
Vainchtein, I. D., and Molofsky, A. V. (2020). Astrocytes and microglia: in sickness and in health. Trends Neurosci. 43, 144–154. doi: 10.1016/j.tins.2020.01.003
Ventorp, F., Barzilay, R., Erhardt, S., Samuelsson, M., Träskman-Bendz, L., Janelidze, S., et al. (2016). The CD44 ligand hyaluronic acid is elevated in the cerebrospinal fluid of suicide attempters and is associated with increased blood–brain barrier permeability. J. Affect. Disord. 193, 349–354. doi: 10.1016/j.jad.2015.12.069
Verdonk, F., Petit, A.-C., Abdel-Ahad, P., Vinckier, F., Jouvion, G., de Maricourt, P., et al. (2019). Microglial production of quinolinic acid as a target and a biomarker of the antidepressant effect of ketamine. Brain Behav. Immunity 81, 361–373. doi: 10.1016/j.bbi.2019.06.033
Verdonk, F., Roux, P., Flamant, P., Fiette, L., Bozza, F. A., Simard, S., et al. (2016). Phenotypic clustering: a novel method for microglial morphology analysis. J. Neuroinflammation 13:153. doi: 10.1186/s12974-016-0614-7
Walker, D. G., and Lue, L.-F. (2015). Immune phenotypes of microglia in human neurodegenerative disease: challenges to detecting microglial polarization in human brains. Alzheimers Res. Ther. 7:56. doi: 10.1186/s13195-015-0139-9
Wang, H.-T., Huang, F.-L., Hu, Z.-L., Zhang, W.-J., Qiao, X.-Q., Huang, Y.-Q., et al. (2017). Early-life social isolation-induced depressive-like behavior in rats results in microglial activation and neuronal histone methylation that are mitigated by minocycline. Neurotox Res. 31, 505–520. doi: 10.1007/s12640-016-9696-3
Wang, Q., Roy, B., Turecki, G., Shelton, R. C., and Dwivedi, Y. (2018). Role of complex epigenetic switching in tumor necrosis factor-α upregulation in the prefrontal cortex of suicide subjects. AJP 175, 262–274. doi: 10.1176/appi.ajp.2017.16070759
Wang, Y., Cella, M., Mallinson, K., Ulrich, J. D., Young, K. L., Robinette, M. L., et al. (2015). TREM2 lipid sensing sustains the microglial response in an Alzheimer’s disease model. Cell 160, 1061–1071. doi: 10.1016/j.cell.2015.01.049
Weber, M. D., McKim, D. B., Niraula, A., Witcher, K. G., Yin, W., Sobol, C. G., et al. (2019). The influence of microglial elimination and repopulation on stress sensitization induced by repeated social defeat. Biol. Psychiatry 85, 667–678. doi: 10.1016/j.biopsych.2018.10.009
Weinhard, L., di Bartolomei, G., Bolasco, G., Machado, P., Schieber, N. L., Neniskyte, U., et al. (2018). Microglia remodel synapses by presynaptic trogocytosis and spine head filopodia induction. Nat. Commun. 9:1228. doi: 10.1038/s41467-018-03566-5
Wilkinson, B. L., and Landreth, G. E. (2006). The microglial NADPH oxidase complex as a source of oxidative stress in Alzheimer’s disease. J. Neuroinflammation 3:30. doi: 10.1186/1742-2094-3-30
Winkler, Z., Kuti, D., Ferenczi, S., Gulyás, K., and Polyák, Á, and Kovács, K. J. (2017). Impaired microglia fractalkine signaling affects stress reaction and coping style in mice. Behav. Brain Res. 334, 119–128. doi: 10.1016/j.bbr.2017.07.023
Wohleb, E. S., Franklin, T., Iwata, M., and Duman, R. S. (2016). Integrating neuroimmune systems in the neurobiology of depression. Nat. Rev. Neurosci. 17, 497–511. doi: 10.1038/nrn.2016.69
Wohleb, E. S., Hanke, M. L., Corona, A. W., Powell, N. D., Stiner, L. M., Bailey, M. T., et al. (2011). Adrenergic receptor antagonism prevents anxiety-like behavior and microglial reactivity induced by repeated social defeat. J. Neurosci. 31, 6277–6288. doi: 10.1523/JNEUROSCI.0450-11.2011
Wohleb, E. S., Powell, N. D., Godbout, J. P., and Sheridan, J. F. (2013). Stress-induced recruitment of bone marrow-derived monocytes to the brain promotes anxiety-like behavior. J. Neurosci. 33, 13820–13833. doi: 10.1523/JNEUROSCI.1671-13.2013
Wohleb, E. S., Terwilliger, R., Duman, C. H., and Duman, R. S. (2018). Stress-induced neuronal colony stimulating factor 1 provokes microglia-mediated neuronal remodeling and depressive-like behavior. Biol. Psychiatry 83, 38–49. doi: 10.1016/j.biopsych.2017.05.026
Wolf, Y., Shemer, A., Levy-Efrati, L., Gross, M., Kim, J.-S., Engel, A., et al. (2018). Microglial MHC class II is dispensable for experimental autoimmune encephalomyelitis and cuprizone-induced demyelination. Eur. J. Immunol. 48, 1308–1318. doi: 10.1002/eji.201847540
Wood, S. K., Wood, C. S., Lombard, C. M., Lee, C. S., Zhang, X.-Y., Finnell, J. E., et al. (2015). Inflammatory factors mediate vulnerability to a social stress-induced depressive-like phenotype in passive coping rats. Biol. Psychiatry 78, 38–48. doi: 10.1016/j.biopsych.2014.10.026
Wu, W., Nicolazzo, J. A., Wen, L., Chung, R., Stankovic, R., Bao, S. S., et al. (2013). Expression of Tryptophan 2,3-dioxygenase and production of kynurenine pathway metabolites in triple transgenic mice and human Alzheimer’s Disease brain. PLoS One 8:e59749. doi: 10.1371/journal.pone.0059749
Wulff, K., Gatti, S., Wettstein, J. G., and Foster, R. G. (2010). Sleep and circadian rhythm disruption in psychiatric and neurodegenerative disease. Nat. Rev. Neurosci. 11, 589–599. doi: 10.1038/nrn2868
Yin, W., Gallagher, N. R., Sawicki, C. M., McKim, D. B., Godbout, J. P., and Sheridan, J. F. (2019). Repeated social defeat in female mice induces anxiety-like behavior associated with enhanced myelopoiesis and increased monocyte accumulation in the brain. Brain Behav. Immunity 78, 131–142. doi: 10.1016/j.bbi.2019.01.015
Youssef, M. M., Underwood, M. D., Huang, Y.-Y., Hsiung, S., Liu, Y., Simpson, N. R., et al. (2018). Association of BDNF Val66Met polymorphism and brain BDNF levels with major depression and suicide. Int. J. Neuropsychopharmacol. 21, 528–538. doi: 10.1093/ijnp/pyy008
Yue, N., Huang, H., Zhu, X., Han, Q., Wang, Y., Li, B., et al. (2017). Activation of P2X7 receptor and NLRP3 inflammasome assembly in hippocampal glial cells mediates chronic stress-induced depressive-like behaviors. J. Neuroinflammation 14:102. doi: 10.1186/s12974-017-0865-y
Zeng, D., He, S., Ma, C., Wen, Y., Song, W., Xu, Q., et al. (2020). Network-based approach to identify molecular signatures in the brains of depressed suicides. Psychiatry Res. 294:113513. doi: 10.1016/j.psychres.2020.113513
Zhang, J., Rong, P., Zhang, L., He, H., Zhou, T., Fan, Y., et al. (2021). IL4-driven microglia modulate stress resilience through BDNF-dependent neurogenesis. Sci. Adv. 7:eabb9888. doi: 10.1126/sciadv.abb9888
Zhang, K., Yang, C., Chang, L., Sakamoto, A., Suzuki, T., Fujita, Y., et al. (2020). Essential role of microglial transforming growth factor-β1 in antidepressant actions of (R)-ketamine and the novel antidepressant TGF-β1. Transl. Psychiatry 10:32. doi: 10.1038/s41398-020-0733-x
Zhang, L., Verwer, R. W. H., Lucassen, P. J., Huitinga, I., and Swaab, D. F. (2020). Prefrontal cortex alterations in glia gene expression in schizophrenia with and without suicide. J. Psychiatr. Res. 121, 31–38. doi: 10.1016/j.jpsychires.2019.11.002
Zhang, L., Verwer, R. W. H., Zhao, J., Huitinga, I., Lucassen, P. J., and Swaab, D. F. (2021). Changes in glial gene expression in the prefrontal cortex in relation to major depressive disorder, suicide and psychotic features. J. Affect. Disord. 295, 893–903. doi: 10.1016/j.jad.2021.08.098
Zhang, S. L., Lahens, N. F., Yue, Z., Arnold, D. M., Pakstis, P. P., Schwarz, J. E., et al. (2021). A circadian clock regulates efflux by the blood-brain barrier in mice and human cells. Nat. Commun. 12:617. doi: 10.1038/s41467-020-20795-9
Zhang, S. L., Yue, Z., Arnold, D. M., Artiushin, G., and Sehgal, A. (2018). A circadian clock in the blood-brain barrier regulates xenobiotic efflux. Cell 173, 130–139.e10. doi: 10.1016/j.cell.2018.02.017
Keywords: microglia, suicide, stress, epigenetics, inflammation, oxidative stress, neuronal support, synaptic plasticity
Citation: Gonçalves de Andrade E, González Ibáñez F and Tremblay M-È (2022) Microglia as a Hub for Suicide Neuropathology: Future Investigation and Prevention Targets. Front. Cell. Neurosci. 16:839396. doi: 10.3389/fncel.2022.839396
Received: 19 December 2021; Accepted: 22 February 2022;
Published: 18 May 2022.
Edited by:
Dirk M. Hermann, University of Duisburg-Essen, GermanyReviewed by:
Laetitia Weinhard, New York University, United StatesCopyright © 2022 Gonçalves de Andrade, González Ibáñez and Tremblay. This is an open-access article distributed under the terms of the Creative Commons Attribution License (CC BY). The use, distribution or reproduction in other forums is permitted, provided the original author(s) and the copyright owner(s) are credited and that the original publication in this journal is cited, in accordance with accepted academic practice. No use, distribution or reproduction is permitted which does not comply with these terms.
*Correspondence: Marie-Ève Tremblay, ZXZldHJlbWJsYXlAdXZpYy5jYQ==
Disclaimer: All claims expressed in this article are solely those of the authors and do not necessarily represent those of their affiliated organizations, or those of the publisher, the editors and the reviewers. Any product that may be evaluated in this article or claim that may be made by its manufacturer is not guaranteed or endorsed by the publisher.
Research integrity at Frontiers
Learn more about the work of our research integrity team to safeguard the quality of each article we publish.