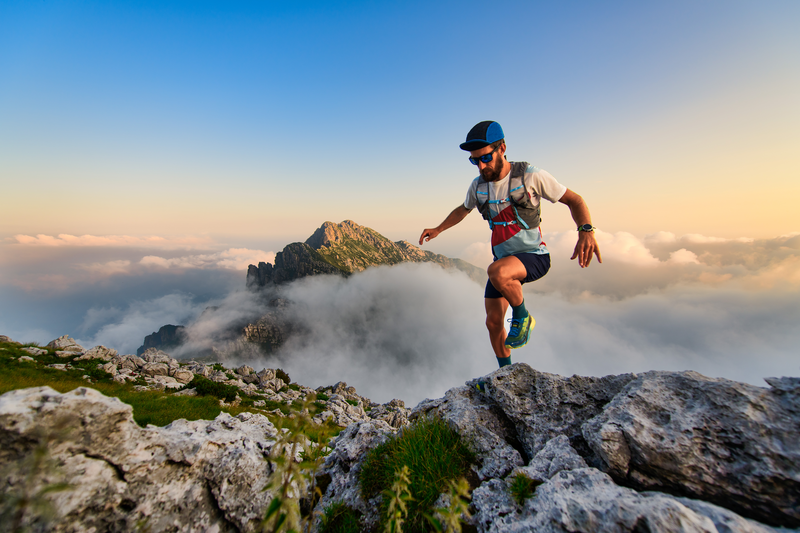
95% of researchers rate our articles as excellent or good
Learn more about the work of our research integrity team to safeguard the quality of each article we publish.
Find out more
ORIGINAL RESEARCH article
Front. Cell. Neurosci. , 31 March 2022
Sec. Cellular Neuropathology
Volume 16 - 2022 | https://doi.org/10.3389/fncel.2022.774297
This article is part of the Research Topic Brain Injury and Repair Following Cerebrovascular Diseases: From Bench to Bedside View all 14 articles
Ferroptosis, an iron-dependent form of non-apoptotic cell death, plays important roles in cerebral ischemia. Previously we have found that L-F001, a novel fasudil-lipoic acid dimer with good pharmacokinetic characters has good neuroprotection against toxin-induced cell death in vitro and in vivo. Here, we investigated the protective effects of L-F001 against a Glutathione peroxidase 4 (GPX4) inhibitor Ras-selective lethality 3 (RSL3) -induced ferroptosis in HT22 cells. We performed MTT, Transmission Electron Microscope (TEM), Western blot, and immunofluorescence analyses to determine the protective effects of L-F001 treatment. RSL3 treatment significantly reduced HT22 cell viability and L-F001 significantly protected RSL3-induced cell death in a concentration-dependent manner and significantly attenuated Mitochondrial shrinkage observed by TEM. Meanwhile, L-F001 significantly decreased RSL3-induced ROS and lipid peroxidation levels in HT22 cells. Moreover L-F001could restore GPX4 and glutamate-cysteine ligase modifier subunit (GCLM) levels, and significantly deceased Cyclooxygenase (COX-2) levels to rescue the lipid peroxidation imbalance. In addition, FerroOrange fluorescent probe and Western blot analysis revealed that L-F001 treatment decreased the total number of intracellular Fe2+ and restore Ferritin heavy chain 1 (FTH1) level in RSL3-induced HT22 cells. Finally, L-F001 could reduce RSL3-induced c-Jun N-terminal kinase (JNK) activation, which might be a potential drug target for LF-001. Considering that L-F001 has a good anti-ferroptosis effect, our results showed that L-F001 might be a multi-target agent for the therapy of ferroptosis-related diseases, such as cerebral ischemia.
Cerebral ischemia, occurring as the result of cardiac arrest, brain injury, shock, and age-related diseases, and then leading to neuronal death and neurological deficits, is a leading cause of disability and death worldwide (Madl and Holzer, 2004). Clinically, there is almost no effective therapy except to restore the blood flow either by pharmacological or mechanical thrombolysis till now (Sarkar et al., 2019). However, only less than 10% of cerebral ischemia patients are eligible for tissue plasminogen activator therapy, and half of those patients fail to demonstrate clinical improvement (DeSai and Hays Shapshak, 2021). So, discovering new drugs against cerebral ischemia is still an urgent issue.
Ferroptosis, a newly discovered iron-associated programmed cell death was reported the play important role in cerebral ischemia. In terms of morphology, the density of mitochondria in ferroptosis is lower, and mitochondrial crests are reduced or disappeared and the outer mitochondrial membrane is ruptured (Dixon et al., 2012; Xie et al., 2016). The biochemical signs of ferroptosis are lipid peroxidation and intracellular elevated Fe2+ level (Xie et al., 2016). Meanwhile, the reduced Glutathione (GSH), Glutathione peroxidase 4 (GPX4) inactivation and COX-2 over activation also predict the occurrence of ferroptosis (Cao and Dixon, 2016).
Growing evidence indicates that iron is a risk factor in the development of cerebral ischemia and ferroptosis is involved in the cerebral ischemia (She et al., 2020). The intracellular elevated Fe2+ level is increased in ischemic brains and iron chelation reduces ischemia-induced brain injury (Abdul et al., 2021). Moreover, several studies elucidated that the activation of ferroptosis following excitatory toxicity in cerebral ischemia induced by the lipid peroxidation mediated by the degradation of GPX4 and overexpression of Acyl-CoA Synthetase Long-Chain Family Member 4 (She et al., 2020; Cui et al., 2021). Meanwhile, the pathology of cerebral ischemia can be effectively alleviated by lipophilic ferroptosis inhibitors such as ferrostatin-1 and liproxstatin-1 (Alim et al., 2019; Guan et al., 2021). In addition, iron metabolism plays an important role in ferroptosis’s pathogenesis, which is also a potential target for cerebral ischemia and ischemic stroke (Dixon and Stockwell, 2014; Doll and Conrad, 2017; Chen et al., 2021). When iron homeostasis is broken, the iron redox couple (Fe3+/Fe2+) could trigger a Fenton reaction which causes overwhelming lipid peroxidation and definitively induces ferroptosis. And using the iron-chelating agent deferoxamine pretreatment can protect against both ferroptosis and cerebral ischemia (Li et al., 2008). Additionally, c-Jun N-terminal kinase (JNK), one member of the mitogen-activated protein kinase (MAPK) plays important role in regulating iron homeostasis through JNK/Sp1 and Stat4/Sp1 signal (Qiu et al., 2020).
L-F001 (Figure 1A), a fasudil-lipoic acid derivative synthesized by our lab has neuroprotection with multifunctional effects such as Rho-associated kinase (ROCK) inhibition. Our previous studies confirmed that L-F001 could prevent cell death induced by paraquat through alleviating endoplasmic reticulum stress and mitochondrial dysfunction in PC12 cells (Shen et al., 2015). In addition, L-F001 also could effectively reduce the cytotoxicity induced by 6-Hydroxydopamine hydrobromide (6-OHDA) in vitro and 1-methyl-4-phenyl-1,2,3,6-tetrahydropyridine (MPTP)-induced dopamine neuron toxicity in mice and improve the levels of p-Akt (Ser473), p-GSK3β(Ser9) to exert a neuroprotective effect (Luo et al., 2017). Moreover, L-F001 can significantly inhibit neuroinflammation induced by LPS and reduce microglial activation in vitro and in vivo (Chen et al., 2017). Considering ferroptosis plays important roles in these PD models (Tian et al., 2020; Mahoney-Sánchez et al., 2021) and L-F001 has anti-PD effects, we hypothesized that L-F001 might be benefit for ferroptosis to rescue other diseases, which might be a target for the therapy of cerebral ischemia. RSL3 is a GPX4 inhibitor which can block the ability for GPX4 that catalyzes GSH to oxidized glutathione (GSSG) and then reduces toxic peroxide to nontoxic hydroxyl compound to indirectly inhibits lipid peroxidation (Yang et al., 2014). Here we investigate the effects of L-F001 on RSL-3-induced ferroptosis in HT22 cells, a mice hippocampal cell line. Our data showed that L-F001 could inhibit RSL3-induced lipid peroxidation, maintain iron homeostasis, and inhibit the JNK pathway to attenuate ferroptosis. And L-F001 might be a multi-target agent for the therapy of ferroptosis-related diseases, such as cerebral ischemia.
Figure 1. L-F001 reduces RSL3-induced cytotoxicity in HT22 cells. (A) Chemical structure of L-F001. (B) HT22 cells were treated with 0.1 μM RSL3 alone, and then added L-F001 (1–10 μM), fasudil (10 μM), and LA (10 μM) at 37°C for 24 h. MTT assays were used to determine cell viability (n = 5). (C) The morphology of HT22 cells (scale bar = 25 μm). (D) Transmission electron microscopy pictures of mitochondrial in cells from control, RSL3, and RSL3 + L-F001 group (Scale bar = 1,200 nm). ###P < 0.001 compared with the control group. *P < 0.05, **P < 0.01, and ***P < 0.001 compared with the RSL3-induced group.
L-F001 (purity>98%) was synthesized before, and the chemical characterization (mass spectroscopy, purity, etc) could be found over there (Chen et al., 2014). Dulbecco’s Modified Eagle’s Medium (DMEM) was from Gibco-BRL (NY, USA). Fetal bovine serum (FBS) was from Hyclone (Logan, UT, USA). A BCA protein assay kit was purchased from Thermo (Waltham, MA, USA). DMSO was obtained from Sigma-Aldrich Inc. (St Louis, MO, USA). Anti-GPX4 antibody, anti-FTH1antibody from Abcam (Cambridge, USA). Anti-COX-2, anti-JNK/p-JNK antibody from Cell Signaling Technology (Woburn, USA). Anti- GCLM antibody form ABclonal (Wuhan, Hubei, P.R.C.).
The sources of the cells are described in previous articles (Lu et al., 2020). HT22 cells were cultured in DMEM (10% FBS) under 5% CO2 and 37°C. And cells were subcultured every 2 days. Morphological change of induced cells was observed by phase-contrast microscopy (Olympus, Tokyo, Japan).
MTT assay was prepared according to our previous article to determine cell viability (Peng et al., 2021). HT22 cells were cultured in 96-well plates at 5 × 103 cells per well and cultured 24 h before administration. Ten microliter of MTT (5 mg/ml) was then added to each well, and the mixture was incubated for 2 h at 37°C. MTT reagent was then carefully replaced with DMSO (100 μl per well) to dissolve formazan crystals. After the mixture was shaken at room temperature for 10 min, absorbance was determined at 490 nm using a microplate reader (Bio-Tek, USA). Cell survival assays were performed in triplicate.
Transmission electron microscope protocol was performed as previously described (Peng et al., 2021). HT22 cells were grown on a 60 mm dish at 6 × 105 cells per dish and cultured 24 h before administration. Then cells were treated with N2L and RSL3. A transmission electron microscope observed the ultrastructure of mitochondria. Briefly, HT22 cells were fixed following drug treatment with 4°C pre-cooled 2.5% glutaraldehyde for 24 h and with 1% osmium tetraoxide for 1 h. The cells were then dehydrated for 15 min at a series of acetone concentrations (50%, 70%, 80%, 90%, and 100%) and embedded in resin. The samples were sliced and double-stained with uranyl acetate and lead citrate, and representative images were obtained using a JEM-1400 electron microscope (JEOL Ltd., Japan).
Measurement of ROS was performed as previously described (Peng et al., 2021). The DHE probes were diluted 1:1,000 with serum-free medium to a final concentration of 10 μM. The serum-free cell culture medium was removed, and an appropriate volume of diluted DHE was added. The appropriate volume to cover the cells was added and the cells were incubated for 20 min in a 37°C cell incubator. The cells were washed three times with serum-free cell culture to adequately remove DHE that did not enter the cells. The high-content screening (HCS) system (Thermo, Waltham, MA, USA) was used to take fluorescence images and analyze the data (excitation wavelengths = 549 nm).
Measurement of lipid oxidation level was using BODIPY 581/591 C11 probe (Glpbio, Guangzhou, China). HT22 cells were grown on a 20 mm glass-bottom dish at 5 × 104 cells per dish and cultured 24 h before administration. Cells were cultured with 2.5 μM C11-BODIPY581/591 for 30 min after drug treatment. The excitation and emission band of oxidized type is pass of 460–495 nm and 510–550 nm, respectively. But the excitation and emission band of reduced type is pass of 565–581 nm and 585–591 nm, respectively. Then photos were taken under a laser confocal microscope (Olympus, Tokyo, Japan).
Rho-Bob is a gift from Prof. Fang Liu from Guangzhou University of Chinese Medicine, which detected the endogenous hydroxyl radicals in cells and the measurement was performed as previously described (Peng et al., 2021). Cells were cultured with 5 μM Rho-Bob for 30 min and observed under a confocal microscope. The excitation and emission band of the oxidized type is 532 nm. And the excitation and emission band of the reduced type is pass of 580–600 nm and 640–660 nm, respectively. Then photos were taken under a laser confocal microscope (Olympus, Tokyo, Japan).
Cells were cultured with 1 μM FerroOrange for 30 min and observed under a confocal microscope (Ex/Em: 561 nm/570–620 nm).To facilitate the observation and analysis, the fluorescence signal from FerroOrange was marked as orange. And cell images were taken using a laser confocal microscope (Olympus, Tokyo, Japan).
Measurement of Immunofluorescence was performed as previously described (Chen et al., 2014). The cells were washed three times with PBS. After being fixed and blocked, the cells were stained overnight at 4°C with anti-COX-2 antibody (CST, 12282, 1:250 dilution) and anti-GPX4 antibody (Abcam, ab125066, 1:500 dilution), followed by Fluorescent secondary antibody (Abcam, ab150080, ab150077, 1:500). Finally, DAPI was used for nuclear staining and cell images were taken using a laser confocal microscope (Olympus, Tokyo, Japan).
Western blotting analysis was performed as previously described (Chen et al., 2014). The primary antibodies are described in “Materials” Section.
Data were shown as the mean ± S.E.M. for 3–5 times independent experiments. Western blotting and Fluorescence intensity was quantified through Image J software. Differences between groups were tested using one-way analysis of variance (ANOVA), followed by a Tukey-Kramer test as post-hoc comparison using the software Prism 8 (Chicago, USA). Differences were considered statistically significant if *P < 0.05.
In our previous research, L-F001 could prevent 6-OHDA-induced cytotoxicity, and 6-OHDAwas a good ferroptosis inducer that could degrade ferritin and damage the iron homeostasis (Sun et al., 2020; Tian et al., 2020). L-F001 (1–10 μM) was incubated with RSL3 in HT22 cells to evaluate protective effects. Our results show that L-F001 reduced RSL3-induced HT22 cells’ cytotoxicity in a dose-dependent manner, and among them, 10 μM L-F001 could almost completely reverse RSL-3-induced HT22 cell injury and restore cell morphology. lipoic acid (LA) had a similar protective effect, but fasudil (Fas) had a slight protective effect (Figures 1B,C). Therefore, the ROCK inhibition of L-F001 was hardly related to the anti-ferroptosis effect. And LA group, which has the function of resisting ferroptosis (Zhang et al., 2018; Liu et al., 2020), plays an important role in the anti-ferroptosis of L-F001. Electron microscope investigation showed the shrunken mitochondria and absence of mitochondria cristae in RSL3-treated HT22 cells, and obvious improvement of mitochondrial morphology could be observed after treatment with L-F001 (Figure 1D).
We measured the intracellular ROS and lipid oxidation levels by dihydroethidium and C11-BODIPY 581/591 probes. ROS and lipid oxidation levels in HT22 cells dramatically increased under the RSL3 treatment. Pretreatment with 10 μM L-F001 significantly decreased ROS (Figures 2A,B) and lipid peroxidation levels in RSL3-induced HT22 cells (Figure 2C). Those results showed L-F001 could effectively improve the lipid metabolism process in ferroptosis.
Figure 2. L-F001 reduces RSL3-induced ROS production and lipid peroxidation in HT22 cells. (A) HT22 cells were treated with 0.1 μM RSL3 alone, and then added L-F001 (1–10 μM) and LA (10 μM) at 37°C for 24 h. ROS formation was measured with a fluorescence microscope (scale bar = 50 μm). (B) ROS levels were measured with a fluorescence plate reader (n = 3). (C) The lipid ROS formation was monitored by a laser confocal microscope using a C11-BODIPY581/591 probe (Scale bar = 25 μm). #P < 0.05 compared with the control group. *P < 0.05, **P < 0.01 compared with the RSL3-induced group.
RSL3 pretreatment resulted in obvious shifts of lipid peroxidation-related proteins in HT22 cells, including cyclooxygenase-2 (COX-2; Yang et al., 2014), GCLM, and GPX4 protein levels (Seibt et al., 2019) 3 and 10 μM L-F001 pretreatment resulted in obviously reduced levels of COX-2 and restored levels of GPX4 and GCLM inRSL3-induced HT22 cells (Figure 3A). Immunofluorescence results confirmed those changes (Figures 3B,C). These data suggested that L-F001 could restore GCLM and GPX4 levels to inhibit ferroptosis in HT22 cells.
Figure 3. L-F001 affects the levels of lipid peroxidation-related protein proteins in HT22 cells. HT22 cells were treated with 0.1 μM RSL3 alone, and then added L-F001 (1–10 μM) at 37°C for 24 h. (A) The levels of COX-2, GCLM, and GPX4 were measured by Western blot, and the amount of COX-2, GCLM, and GPX4 were estimated by densitometric analysis of each protein band (n = 3). (B,C) Cells were immunofluorescence stained with anti-COX-2 and GPX4 antibodies. Nuclear counterstaining was done with DAPI (Scale bar = 100 μm). #P < 0.05 and ##P < 0.01 compared with the control group. *P < 0.05 and ***P < 0.001 compared with the RSL3 group.
Excess intracellular Fe2+ is a marker of ferroptosis, which triggers Fenton’s reaction to lead to membrane lipid peroxidation. We determined the Fe2+ by FerroOrange fluorescent probe and found that 10 μM L-F001 significantly reduced Fe2+ levels under RSL3 treatment in HT22 cells (Figure 4A). Meanwhile, hydroxyl radicals which were produced in Fenton’s reaction dramatically increased under the RSL3 treatment in HT22 cells. Pretreatment with 10 μM L-F001 significantly decreased hydroxyl radicals’ production in RSL3-induced HT22 cells (Figure 4B). To measure the iron storage function, we detected the level of FTH1. And Pretreatment with 10 μM L-F001 could significantly restore the FTH1 level under RSL3 treatment in HT22 cells (Figure 4C).
Figure 4. L-F001 reduces RSL3-induced impairment of iron homeostasis in HT22 cells. HT22 cells were treated with 0.1 μM RSL3 alone, and then added L-F001(10 μM) at 37°C for 24 h. (A) Intracellular Fe2+ level in HT22 cells were detected by FerroOrange probe (scale bar = 25 μm). The fluorescence intensity of the CT group was defined as 100% (n = 5). (B) Endogenous hydroxyl radicals’ level in HT22 cells were detected by Rho-Bob probe (Scale bar = 25 μm). (C) Protein level and analysis of FTH1 in cells (n = 3). ##P < 0.01 and ###P < 0.001 compared with the control group. **P < 0.01 compared with the RSL3-induced group.
Lipid peroxidation also induces phosphorylation of JNK, which is involved in cellular responses to environmental stresses. To explore the potential mechanism of L-F001 inhibiting RSL3-induced ferroptosis, we detected p-JNK/JNK protein levels. p-JNK/JNK increased upon the treatment with RSL3 in HT22 cells, and 10 μM L-F001 pretreatment hindered the increase of JNK phosphorylation level (Figure 5).
Figure 5. L-F001 inhibits RSL3-induced JNK activation in HT22 cells. HT22 cells were treated with 0.1 μM RSL3 alone, and then added L-F001(1–10 μM) at 37°C for 24 h. The levels of P-JNK and JNK were measured by Western blot, and the amount of P-JNK and JNK were estimated by densitometric analysis of each protein band (n = 3). ###P < 0.001 compared with the control group. *P < 0.05 and **P < 0.01 compared with the RSL3 group.
Ferroptosis, a new type of programmed cell death is associated with iron ions and plays an important role in cerebral ischemia (Datta et al., 2020; Liu et al., 2022). L-F001 is a good neuroprotective agent with multifunctional effects such as anti-inflammation and anti-oxidative stress (Shen et al., 2015; Chen et al., 2017; Luo et al., 2017). We found that L-F001 could reduce the lipid peroxidation, impairment of iron homeostasis, and JNK activation in the RSL3-induced HT22 hippocampal neuronal cell line, providing a potential strategy apart from ROCK inhibition for treating ferroptosis-related cerebral ischemia.
MTT assay was used to evaluate cell viability in our previous studies on ferroptosis (Peng et al., 2021) and the neuroprotective effect of L-F001. L-F001 significantly rescued cell viability and showed a similar effect with LA in the RSL3-induced HT22 cells. Still, fasudil had little effect to protect RSL3-induced cell death, indicating that the protection of L-F001 was almost related to the LA group (Figures 1B,C). LA belongs to a group of B vitamins, which plays the role of coenzyme in the oxidative respiratory chain and has antioxidant activities. In recent years, it has been found that lipoic acid has an anti-ferroptosis effect, which can not only alleviate the ferroptosis of PC12 cells induced by MPP+ by activating PI3K/Akt/Nrf2 (Liu et al., 2021), but also alleviate the ferroptosis induced by cobalt nanoparticles (Liu et al., 2020). At the animal level, lipoic acid can significantly reduce iron overload and lipid peroxidation in P301S Tau transgenic mice (Zhang et al., 2018). Compared to LA, L-F001 has a good function of ROCK inhibition and anti-inflammation effect which were often therapeutic targets for cerebral ischemia. At the same time, L-F001 significantly recovered mitochondrial damage (Figure 1D). These findings indicated L-F001 could inhibit ferroptosis and the effect of L-F001 on ferroptosis is largely attributed to the lipoic acid group.
Lipid peroxidation is a bridge between ferroptosis and cerebral ischemia. Ferroptosis is characterized by lipid peroxidation, and the levels of lipid hydroperoxides were significantly higher in ischemic stroke patients (Zeiger et al., 2009; Yang and Stockwell, 2016). L-F001 not only reduced ROS production and lipid peroxidation (Figures 2A,B) but also affects the levels of lipid peroxidation-related protein. Meanwhile, we have found that the ROS production and cell viability after L-F001 treatment is similar with LA in RSL3-induced HT22 cells, and there was no statistical difference between the two groups in 10 μM. Therefore, we thought the LA group is the functional part of L-F001 to resist ferroptosis. And LA has been proved that it had a good 2,2-Diphenyl-1-picrylhydrazyl (DPPH) scavenging ability which represented direct scavenging ability on free radicals (Zhao and Liu, 2011). For this reason, L-F001, as a derivative of LA, might be inferred that it would also have good DPPH scavenging ability same as LA. COX-2 is a key enzyme of lipid peroxidation involved in synthesizing prostaglandins and studies have shown that COX-2 is markedly upregulated during ferroptosis, which is a downstream and suitable marker for the lipid peroxidation that occurs during GPX4-regulated ferroptosis (Yang et al., 2014). Knocking out COX-2 can reduce neuron ferroptosis and lipid peroxidation (Xiao et al., 2019). Interestingly, we found that L-F001 could decrease the level of COX-2, proving that lipid peroxidation has gone (Figures 3A,B). GCLM is a part of the first rate-limiting enzyme of glutathione synthesis, which can produce GSH, a substrate of GPX4, to resist ferroptosis (Kang et al., 2021). GPX4 is a selenium-containing membrane lipid repair enzyme, which can suppress the activation of lipoxygenase and cyclooxygenase at the nucleus and endoplasmic reticulum by reducing fatty acid hydroperoxide as activators of lipoxygenase and cyclooxygenase (Imai et al., 2017). The GPX4 inhibitor RSL3 promotes lipid peroxidation and ferroptosis (Franklin et al., 2009), which leads to GPX4 and GCLM degradation. L-F001 pretreatment could rescue RSL3-induced decrease of GPX4 and GCLM levels (Figures 3A,C). These results confirmed that L-F001 could reduce lipid peroxidation to play an anti-ferroptosis role.
Iron homeostasis is a complex process and relies on the coordination of multiple iron metabolism proteins, including the heavy and light subunit of ferritin (FTH1 and FTL), which is a protein complex that safely concentrates intracellular iron in a mineralized, redox-inactive form; transferrin, an iron-binding serum protein; ferroportin, the only known cellular iron efflux pump (Bogdan et al., 2016). Excessive Fe2+ is a hallmark of ferroptosis, which reacts with H2O2 to produce many hydroxyl radicals (OH•), and OH• promotes the oxidation of polyunsaturated fatty acids to hydroperoxide derivatives of lipids (LOOH) on the cell membrane (Li et al., 2008). FTH1 plays a vital role in the efflux and storage of Fe3+/Fe2+ in cells. Several previous studies elucidated that treating iron chelator deferoxamine (Van der Loo et al., 2020; Abdul et al., 2021) and promoting iron export could prevent ferroptosis damage after ischemic stroke. In the RSL3-treated HT22 cells, we observed the excessive Fe2+ and the downregulation of FTH1, and L-F001 can reduce excessive Fe2+ and restored FTH1 levels (Figures 4A–C). These results indicated that L-F001 could maintain intracellular iron homeostasis and decrease Fenton’s reaction to playing an anti-ferroptosis role.
The activation of the MAPK pathway is one of the important signs of ferroptosis (Stockwell et al., 2017), in which JNK is an important member. JNK responds to environmental stress, and cerebral ischemia can also induce the robust activation of JNK cascades, ultimately resulting in neuron death (Nozaki et al., 2001). In ferroptosis, excess lipid peroxidation products produced during the oxidation of the plasma membrane can remain active in the JNK and enhanced phosphorylation (Nagase et al., 2020; Qiu et al., 2020). And using JNK specific pharmacological inhibitors can alleviate not only ferroptosis (Fuhrmann et al., 2020) and cerebral ischemia (Sun et al., 2015; Zheng et al., 2020). L-F001 significantly reduced JNK phosphorylation (Figure 5), whose effect is reduced intracellular ROS, thus reducing the cell damage caused by JNK hyperphosphorylation. As for the other two MAPKs signaling (ERK and P38), they can all be activated by the same stimulation factors such as cytokines, neurotransmitters, hormones, cellular stress, and so on, and undergo the same changes. Many articles related to ferroptosis only detect one of them to characterize the change of ERK and P38 (Yang et al., 2021; Wang et al., 2022). In this study, we focused on the protective effects of L-F001 on ferroptosis and tentatively evaluated the JNK pathway. On the other side, our previous results indicated that L-F001 could activate Nrf-2/HO-1 signaling (Luo et al., 2017), we also found that Nrf2/HO-1 signaling is activation under RSL-3 treatment rather than downregulation (Peng et al., 2021). Furthermore, some research demonstrated that many active compounds exhibit protection through further upregulation of Nrf2/HO-1 signaling (Gou et al., 2020; Wei et al., 2021; Fu et al., 2022), compared to the already increased Nrf2 signaling. Therefore Nrf2/HO-1 signaling activation might be an important part in L-F001 anti-ferroptosis role. And in a future study, we will deeply explore the signaling pathway mechanism of L-F001 against ferroptosis such as Nrf2/HO-1, ERK, and P38.
In conclusion, due to the lipoic acid group, L-F001 is a good antioxidant and ferroptosis inhibitor, which can significantly restore RSL3-induced broken iron homeostasis, reduce lipid peroxidation, and JNK overactivation in HT22 cells. Consequently, because of its anti-ferroptosis and ROCK inhibition effect, L-F001 can potentially treat ferroptosis-related cerebral ischemia without the hypotensive response of fasudil. However, there are multiple programmed cell deaths involved in cerebral ischemia apart from ferroptosis, and the animals and clinical application need to be further verified.
The original contributions presented in the study are included in the article/Supplementary Material, further inquiries can be directed to the corresponding author.
WP: investigation, writing—original draft, and formal analysis. SW and JH: investigation and formal analysis. ZZ: investigation and writing—review. YY: investigation. RZ: formal analysis and validation. RP: writing—review and editing. YO: conceptualization, project administration, and supervision. All authors contributed to the article and approved the submitted version.
This work was supported by YO from Guangzhou People’s Livelihood Science and Technology Project (No. 201903010079), Natural Science Foundation of Guangdong Province (No. 2021A1515010323), and Rural Science and Technology Commissioner Program of Guangdong Province (No. KTP2020323).
The authors declare that the research was conducted in the absence of any commercial or financial relationships that could be construed as a potential conflict of interest.
All claims expressed in this article are solely those of the authors and do not necessarily represent those of their affiliated organizations, or those of the publisher, the editors and the reviewers. Any product that may be evaluated in this article, or claim that may be made by its manufacturer, is not guaranteed or endorsed by the publisher.
The Supplementary Material for this article can be found online at: https://www.frontiersin.org/articles/10.3389/fncel.2022.774297/full#supplementary-material.
Abdul, Y., Li, W., Ward, R., Abdelsaid, M., Hafez, S., Dong, G., et al. (2021). Deferoxamine treatment prevents post-stroke vasoregression and neurovascular unit remodeling leading to improved functional outcomes in type 2 male diabetic rats: role of endothelial ferroptosis. Transl. Stroke Res. 12, 615–630. doi: 10.1007/s12975-020-00844-7
Alim, I., Caulfield, J. T., Chen, Y., Swarup, V., Geschwind, D. H., Ivanova, E., et al. (2019). Selenium drives a transcriptional adaptive program to block ferroptosis and reat stroke. Cell 177, 1262–1279.e25. doi: 10.1016/j.cell.2019.03.032
Bogdan, A. R., Miyazawa, M., Hashimoto, K., and Tsuji, Y. (2016). Regulators of iron homeostasis: new players in metabolism, cell death and disease. Trends Biochem. Sci. 41, 274–286. doi: 10.1016/j.tibs.2015.11.012
Cao, J. Y., and Dixon, S. J. (2016). Mechanisms of ferroptosis. Cell Mol. Life Sci. 73, 2195–2209. doi: 10.1007/s00018-016-2194-1
Chen, W., Jiang, L., Hu, Y., Tang, N., Liang, N., Li, X. F., et al. (2021). Ferritin reduction is essential for cerebral ischemia-induced hippocampal neuronal death through p53/SLC7A11-mediated ferroptosis. Brain Res. 1752:147216. doi: 10.1016/j.brainres.2020.147216
Chen, M., Liu, Q., Liu, A., Tan, M., Xie, Z., Uri, A., et al. (2014). Simply combining fasudil and lipoic acid in a novel multitargeted chemical entity potentially useful in central nervous system disorders. RSC Adv. 4, 37266–37269doi: 10.1039/C4RA07337A
Chen, J., Yin, W., Tu, Y., Wang, S., Yang, X., Chen, Q., et al. (2017). L-F001, a novel multifunctional ROCK inhibitor, suppresses neuroinflammation in vitro and in vivo: involvement of NF-κB inhibition and Nrf2 pathway activation. Eur. J. Pharmacol. 806, 1–9. doi: 10.1016/j.ejphar.2017.03.025
Cui, Y., Zhang, Y., Zhao, X., Shao, L., Liu, G., Sun, C., et al. (2021). ACSL4 exacerbates ischemic stroke by promoting ferroptosis-induced brain injury and neuroinflammation. Brain Behav. Immun. 93, 312–321. doi: 10.1016/j.bbi.2021.01.003
Datta, A., Sarmah, D., Mounica, L., Kaur, H., Kesharwani, R., Verma, G., et al. (2020). Cell death pathways in ischemic stroke and targeted pharmacotherapy. Transl. Stroke Res. 11, 1185–1202. doi: 10.1007/s12975-020-00806-z
DeSai, C., and Hays Shapshak, A. (2021). “Cerebral ischemia,” in StatPearls [Internet]. Treasure Island, FL: StatPearls Publishing.
Dixon, S. J., Lemberg, K. M., Lamprecht, M. R., Skouta, R., Zaitsev, E. M., Gleason, C. E., et al. (2012). Ferroptosis: an iron-dependent form of nonapoptotic cell death. Cell 149, 1060–1072. doi: 10.1016/j.cell.2012.03.042
Dixon, S. J., and Stockwell, B. R. (2014). The role of iron and reactive oxygen species in cell death. Nat. Chem. Biol. 10, 9–17. doi: 10.1038/nchembio.1416
Doll, S., and Conrad, M. (2017). Iron and ferroptosis: a still ill-defined liaison. IUBMB Life 69, 423–434. doi: 10.1002/iub.1616
Franklin, C. C., Backos, D. S., Mohar, I., White, C. C., Forman, H. J., and Kavanagh, T. J. (2009). Structure, function and post-translational regulation of the catalytic and modifier subunits of glutamate cysteine ligase. Mol. Aspects Med. 30, 86–98. doi: 10.1016/j.mam.2008.08.009
Fu, C., Wu, Y., Liu, S., Luo, C., Lu, Y., Liu, M., et al. (2022). Rehmannioside A improves cognitive impairment and alleviates ferroptosis via activating PI3K/AKT/Nrf2 and SLC7A11/GPX4 signaling pathway after ischemia. J. Ethnopharmacol. 289:115021. doi: 10.1016/j.jep.2022.115021
Fuhrmann, D. C., Mondorf, A., Beifuß, J., Jung, M., and Brüne, B. (2020). Hypoxia inhibits ferritinophagy, increases mitochondrial ferritin and protects from ferroptosis. Redox Biol. 36:101670. doi: 10.1016/j.redox.2020.101670
Gou, Z., Su, X., Hu, X., Zhou, Y., Huang, L., Fan, Y., et al. (2020). Melatonin improves hypoxic-ischemic brain damage through the Akt/Nrf2/Gpx4 signaling pathway. Brain Res. Bull. 163, 40–48. doi: 10.1016/j.brainresbull.2020.07.011
Guan, X., Li, Z., Zhu, S., Cheng, M., Ju, Y., Ren, L., et al. (2021). Galangin attenuated cerebral ischemia-reperfusion injury by inhibition of ferroptosis through activating the SLC7A11/GPX4 axis in gerbils. Life Sci. 264:118660. doi: 10.1016/j.lfs.2020.118660
Imai, H., Matsuoka, M., Kumagai, T., Sakamoto, T., and Koumura, T. (2017). Lipid peroxidation-dependent cell death regulated by GPx4 and ferroptosis. Curr. Top. Microbiol. Immunol. 403, 143–170. doi: 10.1007/82_2016_508
Kang, Y. P., Mockabee-Macias, A., Jiang, C., Falzone, A., Prieto-Farigua, N., Stone, E., et al. (2021). Non-canonical glutamate-cysteine ligase activity protects against ferroptosis. Cell Metab. 33, 174–189.e7. doi: 10.1016/j.cmet.2020.12.007
Li, Y. X., Ding, S. J., Xiao, L., Guo, W., and Zhan, Q. (2008). Desferoxamine preconditioning protects against cerebral ischemia in rats by inducing expressions of hypoxia inducible factor 1α and erythropoietin. Neurosci. Bull. 24, 89–95. doi: 10.1007/s12264-008-0089-3
Liu, Y., Fang, Y., Zhang, Z., Luo, Y., Zhang, A., Lenahan, C., et al. (2022). Ferroptosis: an emerging therapeutic target in stroke. J. Neurochem. 160, 64–73. doi: 10.1111/jnc.15351
Liu, L., Yang, S., and Wang, H. (2021). α-Lipoic acid alleviates ferroptosis in the MPP+-induced PC12 cells via activating the PI3K/Akt/Nrf2 pathway. Cell Biol. Int. 45, 422–431. doi: 10.1002/cbin.11505
Liu, Y., Zhu, W., Ni, D., Zhou, Z., Gu, J. H., Zhang, W., et al. (2020). Alpha lipoic acid antagonizes cytotoxicity of cobalt nanoparticles by inhibiting ferroptosis-like cell death. J. Nanobiotechnology 18:141. doi: 10.1186/s12951-020-00700-8
Lu, J., Chen, C., Deng, X., Mak, M. S., Zhu, Z., He, X., et al. (2020). Design, synthesis and biological evaluation of novel multifunctional rolipram-tranilast hybrids as potential treatment for traumatic brain injury. ACS Chem. Neurosci. 11, 2348–2360. doi: 10.1021/acschemneuro.0c00339
Luo, L., Chen, J., Su, D., Chen, M., Luo, B., Pi, R., et al. (2017). L-F001, a multifunction ROCK inhibitor prevents 6-OHDA induced cell death through activating Akt/GSK-3beta and Nrf2/HO-1 signaling pathway in PC12 cells and attenuates MPTP-induced dopamine neuron toxicity in mice. Neurochem. Res. 42, 615–624. doi: 10.1007/s11064-016-2117-4
Madl, C., and Holzer, M. (2004). Brain function after resuscitation from cardiac arrest. Curr. Opin. Crit. Care 10, 213–217. doi: 10.1097/01.ccx.0000127542.32890.fa
Mahoney-Sánchez, L., Bouchaoui, H., Ayton, S., Devos, D., Duce, J. A., and Devedjian, J. C. (2021). Ferroptosis and its potential role in the physiopathology of Parkinson’s disease. Prog. Neurobiol. 196:101890. doi: 10.1016/j.pneurobio.2020.101890
Nagase, H., Katagiri, Y., Oh-Hashi, K., Geller, H. M., and Hirata, Y. (2020). Reduced sulfation enhanced oxytosis and ferroptosis in mouse hippocampal HT22 cells. Biomolecules 10:92. doi: 10.3390/biom10010092
Nozaki, K., Nishimura, M., and Hashimoto, N. (2001). Mitogen-activated protein kinases and cerebral ischemia. Mol. Neurobiol. 23, 1–19. doi: 10.1385/MN:23:1:01
Peng, W., Zhu, Z., Yang, Y., Hou, J., Lu, J., Chen, C., et al. (2021). N2L, a novel lipoic acid-niacin dimer, attenuates ferroptosis and decreases lipid peroxidation in HT22 cells. Brain Res. Bull. 174, 250–259. doi: 10.1016/j.brainresbull.2021.06.014
Qiu, L., Ge, L., and Hu, Q. (2020). Dexmedetomidine protects SK-N-SH nerve cells from oxidative injury by maintaining iron homeostasis. Biol. Pharm. Bull. 43, 424–431. doi: 10.1248/bpb.b19-00711
Sarkar, S., Chakraborty, D., Bhowmik, A., and Ghosh, M. K. (2019). Cerebral ischemic stroke: cellular fate and therapeutic opportunities. Front. Biosci. (Landmark Ed) 24, 435–450. doi: 10.2741/4727
Seibt, T. M., Proneth, B., and Conrad, M. (2019). Role of GPX4 in ferroptosis and its pharmacological implication. Free Radic. Biol Med. 133, 144–152. doi: 10.1016/j.freeradbiomed.2018.09.014
She, X., Lan, B., Tian, H., and Tang, B. (2020). Cross talk between ferroptosis and cerebral ischemia. Front. Neurosci. 14:776. doi: 10.3389/fnins.2020.00776
Shen, W., Wang, L., Pi, R., Li, Z., and Wang, R. (2015). L-F001, a multifunctional ROCK inhibitor prevents paraquat-induced cell death through attenuating ER stress and mitochondrial dysfunction in PC12 cells. Biochem. Biophys. Res. Commun. 464, 794–799. doi: 10.1016/j.bbrc.2015.07.035
Stockwell, B. R., Friedmann Angeli, J. P., Bayir, H., Bush, A. I., Conrad, M., Dixon, S. J., et al. (2017). Ferroptosis: a regulated cell death nexus linking metabolism, redox biology and disease. Cell 171, 273–285. doi: 10.1016/j.cell.2017.09.021
Sun, K., Fan, J., and Han, J. (2015). Ameliorating effects of traditional Chinese medicine preparation, Chinese materia medica and active compounds on ischemia/reperfusion-induced cerebral microcirculatory disturbances and neuron damage. Acta Pharm. Sin. B 5, 8–24. doi: 10.1016/j.apsb.2014.11.002
Sun, Y., He, L., Wang, T., Hua, W., Qin, H., Wang, J., et al. (2020). Activation of p62-keap1-Nrf2 pathway protects 6-Hydroxydopamine-induced ferroptosis in dopaminergic cells. Mol. Neurobiol. 57, 4628–4641. doi: 10.1007/s12035-020-02049-3
Tian, Y., Lu, J., Hao, X., Li, H., Zhang, G., Liu, X., et al. (2020). FTH1 inhibits ferroptosis through ferritinophagy in the 6-OHDA model of Parkinson’s disease. Neurotherapeutics 17, 1796–1812. doi: 10.1007/s13311-020-00929-z
Van der Loo, L. E., Aquarius, R., Teernstra, O., Klijn, K., Menovsky, T., van Dijk, J., et al. (2020). Iron chelators for acute stroke. Cochrane Database Syst. Rev. 11:CD009280. doi: 10.1002/14651858.CD009280.pub3
Wang, X., Zhang, C., Zou, N., Chen, Q., Wang, C., Zhou, X., et al. (2022). Lipocalin-2 silencing suppresses inflammation and oxidative stress of acute respiratory distress syndrome by ferroptosis via inhibition of MAPK/ERK pathway in neonatal mice. Bioengineered 13, 508–520. doi: 10.1080/21655979.2021.2009970
Wei, N., Lu, T., Yang, L., Dong, Y., and Liu, X. (2021). Lipoxin A4 protects primary spinal cord neurons from Erastin-induced ferroptosis by activating the Akt/Nrf2/HO-1 signaling pathway. FEBS Open Bio 11, 2118–2126. doi: 10.1002/2211-5463.13203
Xiao, X., Jiang, Y., Liang, W., Wang, Y., Cao, S., Yan, H., et al. (2019). miR-212–5p attenuates ferroptotic neuronal death after traumatic brain injury by targeting Ptgs2. Mol. Brain 12:78. doi: 10.1186/s13041-019-0501-0
Xie, Y., Hou, W., Song, X., Yu, Y., Huang, J., Sun, X., et al. (2016). Ferroptosis: process and function. Cell Death Differ. 23, 369–379. doi: 10.1038/cdd.2015.158
Yang, J., Mo, J., Dai, J., Ye, C., Cen, W., Zheng, X., et al. (2021). Cetuximab promotes RSL3-induced ferroptosis by suppressing the Nrf2/HO-1 signalling pathway in KRAS mutant colorectal cancer. Cell Death Dis. 12:1079. doi: 10.1038/s41419-021-04367-3
Yang, W. S., SriRamaratnam, R., Welsch, M. E., Shimada, K., Skouta, R., Viswanathan, V. S., et al. (2014). Regulation of ferroptotic cancer cell death by GPX4. Cell 156, 317–331. doi: 10.1016/j.cell.2013.12.010
Yang, W. S., and Stockwell, B. R. (2016). Ferroptosis: death by lipid peroxidation. Trends Cell Biol. 26, 165–176. doi: 10.1016/j.tcb.2015.10.014
Zeiger, S. L., Musiek, E. S., Zanoni, G., Vidari, G., Morrow, J. D., Milne, G. J., et al. (2009). Neurotoxic lipid peroxidation species formed by ischemic stroke increase injury. Free Radic. Biol. Med. 47, 1422–1431. doi: 10.1016/j.freeradbiomed.2009.08.011
Zhang, Y. H., Wang, D. W., Xu, S. F., Zhang, S., Fan, Y. G., Yang, Y. Y., et al. (2018). α-lipoic acid improves abnormal behavior by mitigation of oxidative stress, inflammation, ferroptosis and tauopathy in P301S tau transgenic mice. Redox Biol. 14, 535–548. doi: 10.1016/j.redox.2017.11.001
Zhao, F., and Liu, Z. Q. (2011). Comparison of antioxidant effectiveness of lipoic acid and dihydrolipoic acid. J. Biochem. Mol. Toxicol. 25, 216–223. doi: 10.1002/jbt.20378
Keywords: L-F001, ferroptosis, iron homeostasis, lipid peroxidation, c-Jun N-terminal kinase
Citation: Peng W, Ouyang Y, Wang S, Hou J, Zhu Z, Yang Y, Zhou R and Pi R (2022) L-F001, a Multifunctional Fasudil-Lipoic Acid Dimer Prevents RSL3-Induced Ferroptosis via Maintaining Iron Homeostasis and Inhibiting JNK in HT22 Cells. Front. Cell. Neurosci. 16:774297. doi: 10.3389/fncel.2022.774297
Received: 15 October 2021; Accepted: 09 March 2022;
Published: 31 March 2022.
Edited by:
Gaiqing Wang, The Third People’s Hospital of Hainan Province, ChinaReviewed by:
Tarun Keswani, Massachusetts General Hospital and Harvard Medical School, United StatesCopyright © 2022 Peng, Ouyang, Wang, Hou, Zhu, Yang, Zhou and Pi. This is an open-access article distributed under the terms of the Creative Commons Attribution License (CC BY). The use, distribution or reproduction in other forums is permitted, provided the original author(s) and the copyright owner(s) are credited and that the original publication in this journal is cited, in accordance with accepted academic practice. No use, distribution or reproduction is permitted which does not comply with these terms.
*Correspondence: Ying Ouyang, b3V5eUBtYWlsLnN5c3UuZWR1LmNu
† These authors have contributed equally to this work
Disclaimer: All claims expressed in this article are solely those of the authors and do not necessarily represent those of their affiliated organizations, or those of the publisher, the editors and the reviewers. Any product that may be evaluated in this article or claim that may be made by its manufacturer is not guaranteed or endorsed by the publisher.
Research integrity at Frontiers
Learn more about the work of our research integrity team to safeguard the quality of each article we publish.