- 1Department of Neurology, Tongji Hospital, Tongji Medical College, Huazhong University of Science and Technology, Wuhan, China
- 2Department of Radiology, Tongji Hospital, Tongji Medical College, Huazhong University of Science and Technology, Wuhan, China
Sphingosine-1-phosphate (S1P) signaling is being increasingly recognized as a strong modulator of immune cell migration and endothelial function. Fingolimod and other S1P modulators in ischemic stroke treatment have shown promise in emerging experimental models and small-scale clinical trials. In this article, we will review the current knowledge of the role of S1P signaling in brain ischemia from the aspects of inflammation and immune interventions, sustaining endothelial functions, regulation of blood-brain barrier integrity, and functional recovery. We will then discuss the current and future therapeutic perspectives of targeting S1P for the treatment of ischemic stroke. Mechanism studies would help to bridge the gap between preclinical studies and clinical practice. Future success of bench-to-bedside translation shall be based on in depth understanding of S1P signaling during stroke and on the ability to have a fine temporal and spatial regulation of the signal pathway.
Introduction
As the second largest cause of death and a leading cause of disability (Johnson et al., 2019), stroke produces immense health and economic burdens globally (Virani et al., 2020). With an aging population, the prevalence of stroke can be predicted to increase. More worrisome is the fact that the incidence of stroke in young adults has increased in recent years, which causes a profound socioeconomic impact due to high health-care expenditure and compromised labor productivity (Ekker et al., 2018). Most stroke survivors are unable to live independently and have greater risks of recurrence and other long-term disabling sequelae such as dementia (Levine et al., 2015).
Although the primary health goal is a decrease in stroke incidence as prevention is always better than cure. The increasing global stroke burden indicates that primary prevention strategies may not be sufficiently effective (Johnson et al., 2019), which calls for effective therapies in the acute phase and long-term follow-up rehabilitation for people who have developed stroke. About 87% of all strokes are ischemic (Virani et al., 2020). Despite a series of clinical trials with neuroprotective drugs, current treatment options remain limited to thrombolysis and mechanical recanalization for the acute phase of ischemic stroke (Powers, 2019). While effective, the treatments can only be applied to less than 10% of patients for a narrow treatment time window and strict exclusion criteria (Rinaldo et al., 2019). Moreover, even a successful recanalization may lead to enlarged infarction due to ischemic-reperfusion (IR) injury (Mizuma et al., 2018). Novel safe and effective treatment strategies are therefore needed.
Sphingosine-1-phosphate (S1P) is a terminal breakdown product of sphingolipid metabolism discovered in the 1960s (Cartier and Hla, 2019). The degradation of plasma membrane sphingomyelin produces ceramide (Merrill, 2011). S1P is then produced through the metabolism of ceramide by ceramidase and two types of sphingosine kinases (SphK1 and −2). S1P can also be recycled back to ceramide or irreversibly degraded by S1P lyse (Figure 1; Hannun and Obeid, 2018). Many tissues keep a low intracellular S1P level by a rapid degradation of S1P. By contrast, S1P can be transported extracellularly allowing its extracellular action (Proia and Hla, 2015). Erythrocytes and endothelial cells are the major producers of S1P, while substantial amounts of S1P stored in platelets can only be released upon activation (Gazit et al., 2016). In circulation, S1P is bound mainly to high-density lipoproteins and albumin (Proia and Hla, 2015). These protein chaperones of S1P enable its solubility and specific biological activity. Outside the cell, S1P binds to S1P receptors (S1PR) to exert its biological actions. Vertebrates possess five S1PR (S1PR1-5) which are G-protein coupled receptors (Proia and Hla, 2015). These widely expressed S1PRs couple to key intracellular signaling pathways (Chun et al., 2010; Proia and Hla, 2015), thus coupling phospholipid metabolism with intercellular communication (Cartier and Hla, 2019). In the central nervous system, neuronal lineages express all S1PR (Soliven et al., 2011). Astrocytes and microglia express predominantly S1PR1 and S1PR3 (Benarroch, 2021). S1PR5 is mainly expressed in oligodendrocytes (di Nuzzo et al., 2014). As for peripheral immune cells, T cells are known to express S1PR1 (Baeyens et al., 2021), S1PR2 (Baeyens et al., 2015), and S1PR4 (Xiong et al., 2019). Human B cells express S1PR1, S1PR2, and S1PR4 at different levels by different B-cell subtypes but not S1PR3 (Sic et al., 2014). S1P signaling participates in many processes of growth and development and pathological conditions (Proia and Hla, 2015). It is essential for neural and vascular development (Mizugishi et al., 2005). S1P is in relatively high concentrations in circulation compared with tissue parenchyma. This concentration gradient, formed by the interplay of S1P synthetic and degradative enzymes as well as S1P exporters, is fundamental to S1P biology such as regulating lymphocyte migration (Cyster and Schwab, 2012) and supporting endothelial barrier function (Camerer et al., 2009).
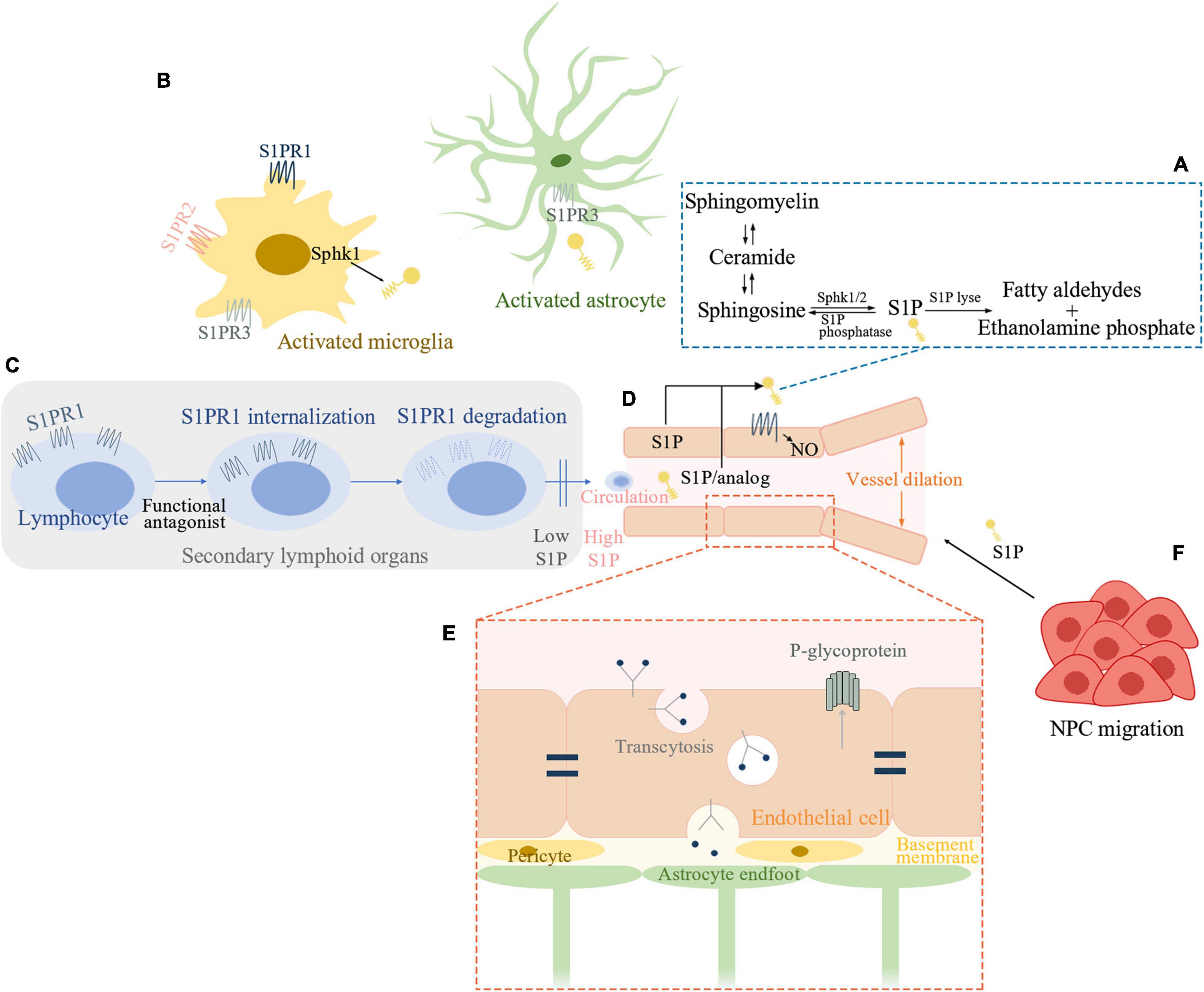
Figure 1. Sphingosine1-phosphate (S1P) signaling pathway involved in ischemic stroke. (A) Schematic outline of S1P metabolism. (B) S1P signaling in post-ischemic brain inflammation. Sphk1 level is elevated in microglia and S1P production is enhanced in activated microglia. S1PR1, S1PR2, and S1PR3 influence microglial activation. Astrocytes interact extensively with microglia. S1P can activate astrocytes while S1PR3 is known to promote astrogliosis in ischemic brain. (C) S1P and recruitment of peripheral lymphocytes. Functional antagonist of S1PR1 can induce receptor internalization and degradation and thereby inhibits peripheral lymphocytes’ recruitment. (D) S1P signaling in sustaining endothelial functions. S1P-S1PR1-nitric oxide regulates vessel dilation to flow. S1PR1 distributes to the abluminal surface of the endothelial cells. Either cell-autonomous S1P or system S1P/analog which penetrates the blood-brain barrier (BBB) can activate endothelial S1PR1 and sustains endothelial function. (E) S1P inhibits the function of P-glycoprotein at the BBB. Brain endothelial S1PR1 may help maintain BBB function by sustaining a proper distribution of tight junction proteins. Endothelial S1PR1 may participate in regulating vesicular transport in the early phase of BBB opening after ischemic stroke. (F) S1P is a chemoattractant for neural progenitor cells (NPC) toward the infarcted area thus facilitating neurogenesis. Sphk, sphingosine kinase; S1P, sphingosine-1-phosphate; S1PR, S1P receptor; NO, nitric oxide; NPC, neural progenitor cells.
The observation that Rag1–/– mice, that were mice devoid of lymphocytes, developed smaller infarct volume than that of wild-type mice (Yilmaz et al., 2006), pioneering a new field of immunomodulating therapies in ischemic stroke, especially those that have been used for the treatment of multiple sclerosis (MS) (Dreikorn et al., 2018). One of the best-studied drugs is fingolimod (FTY720), a S1P modulator that causes rapid induction of lymphopenia (Cyster and Schwab, 2012) and is approved for the treatment of relapsing-remitting multiple sclerosis (RRMS). Moreover, recent studies suggest that the function of S1P signaling in ischemic stroke includes but goes far beyond immunomodulating (Li et al., 2020; Chua et al., 2021; Nitzsche et al., 2021). Emerging experimental models and small-scale clinical trials have shown promise of S1P modulator for the treatment of stroke (Cyster and Schwab, 2012; Kraft et al., 2013; Fu et al., 2014; Qin et al., 2017). Herein, we highlight S1P signaling pathway in ischemic stroke and the translation from biomedical research basis into clinical stroke applications.
S1P in Cerebral Ischemia
We attempt to have a brief summary from the aspects of brain inflammation and immune interventions, sustaining endothelial functions, regulation of blood-brain barrier (BBB), and functional recovery (Figure 1).
S1P Signaling in Post-ischemic Brain Inflammation and Immune Interventions
Inflammatory reactions and immune responses have long been recognized as important elements during ischemic stroke (Fu et al., 2015). Neural cell death after ischemia releases damage-associated molecular patterns (DAMPs) and triggers immune responses including activation of microglia and astrocytes, recruitment of resident and peripheral immune cells (Shi et al., 2019). Inflammation can be both detrimental and beneficial at certain stages after stroke (Lambertsen et al., 2018).
Microglia are among the very first cell types to be activated and recruited to the site of ischemia (Ma et al., 2017). Upon activation, microglia produce numerous mediators such as cytokines and chemokines, growth and trophic factors (Ma et al., 2017). Studies showed that Sphk1 level was elevated in microglia and S1P production was enhanced mainly in activated microglia after ischemia (Kimura et al., 2008; Zheng et al., 2015). The production of S1P on the other hand enhanced the release of proinflammatory mediators from microglia (Nayak et al., 2010; Moon et al., 2015). In addition, S1PR1, S1PR2, and S1PR3 were shown to influence microglial activation and inflammation (Gaire et al., 2018b,2019; Sapkota et al., 2019; Gaire and Choi, 2021). S1PR1 knock-down reduced microglial activation and microglial proliferation after ischemia (Gaire et al., 2018a). Therefore, the pathogenic role of S1P signaling may have a close relationship with microglia activation after ischemic stroke.
As the most numerous cells in the brain, astrocytes interact extensively with microglia (Liddelow et al., 2020) and help recruit immune cells (Li M. et al., 2017). S1P was also shown to activate astrocytes (Sorensen et al., 2003) while S1PR3 could promote astrogliosis after ischemic stroke (Gaire et al., 2018a,b). An antagonist of S1PR3, CAY10444 was found to attenuate astrocyte activation after transient middle cerebral artery occlusion (tMCAO) (Gaire et al., 2018b). Furthermore, S1PR3 deletion could attenuate S1P-induced inflammatory responses in astrocytes (Dusaban et al., 2017).
Invasion of peripheral lymphocytes drives the progression of inflammation (Shi et al., 2019). During IR injury, T cells interact with platelets and formulate a complex process called thrombo-inflammation which leads to infarct expansion (Stoll and Nieswandt, 2019). Experiments on a series of ischemic stroke mouse models indicated that the protective effect of S1P modulators such as fingolimod could be largely attributed to impairment of lymphocyte trafficking and thereby, lymphocyte-driven thrombo-inflammation (Kraft et al., 2013). A marked decrease in infarct volume and improvement of functional outcome were found after fingolimod administration in wild type but not lymphocyte-deficient Rag1–/– mice after tMCAO, highlighting the key mechanism of lymphocytopenia in its protective effect (Kraft et al., 2013). Lymphocytopenia could then attenuate thrombo-inflammation in microvasculature and increase cerebral blood flow (CBF) after tMCAO (Kraft et al., 2013). However, in another permanent occlusion of the middle cerebral artery (pMCAO) mouse model, infarct volume and behavioral dysfunction were not reduced by fingolimod (Liesz et al., 2011). Similar results were found in pan-hematopoietic S1pr1 knockout (KO) mice. Hematopoietic S1PR1 deficiency induced lymphopenia and exerted some neuroprotection after tMCAO, but not pMCAO (Nitzsche et al., 2021). These unexpected negative findings in pMCAO can be explained by the difference in the contribution of neuroinflammation in transient and permanent ischemia (Stoll and Nieswandt, 2019). In contrast to tMCAO where recanalization occurs, and the function of T cells is well established as contributing to IR injury in an antigen-independent fashion, in pMCAO, the contribution of T cells is less clear and more complex as secondary phenomena such as gut microbiome mediated systemic immunomodulation and stroke-related immunodepression syndrome may participate in the process (Stoll and Nieswandt, 2019).
S1P Signaling in Sustaining Endothelial Functions
Cerebral endothelial dysfunction contributes to stroke-induced brain injury. Despite successful recanalization, as many as 25–50% of patients still have undesirable long-term outcomes (Meinel et al., 2020). A main reason for this futile recanalization is poor reperfusion in the microvasculature downstream of an occlusion (Tian et al., 2018). In addition, to counteract the expansion of the infarct core, adequate and timely perfusion is needed to rescue the ischemic penumbra, the area surrounding the necrotic core, where CBF is still sufficient to keep neurons alive (Manning et al., 2014). Not only the number and size but also the dilatory capacity, integrity, and patency of collateral anastomose are important for successful reperfusion (Bonnin et al., 2019). Ischemia impairs endothelium-dependent vasodilation (Hu et al., 2017) and induces a proinflammatory endothelial phenotype (Ishikawa et al., 2003) which may promote thrombus formation and reduce blood flow. Sustaining endothelial functions in ischemic stroke may therefore constitute a therapeutic opportunity (Shuaib et al., 2011).
S1PR1, S1PR2, and S1PR3 are found in endothelial cells and S1P signaling help sustain endothelial functions (Proia and Hla, 2015). S1P is believed to act on endothelial S1PR1 to reduce vascular leakage (Camerer et al., 2009) and improve endothelial barrier (Huwiler and Zangemeister-Wittke, 2018). S1P-S1PR1-nitric oxide signaling was found to be a new regulatory pathway of vessel dilation to flow (Cantalupo et al., 2017). As for endothelial S1PR1 in the brain, a recent study demonstrated that after the formation of BBB, S1PR1 distributed to the abluminal surface of the endothelial cells which shielded them from ligands in circulation (Nitzsche et al., 2021). Therefore, cell-autonomous S1P is required to activate these endothelial S1PR1. While BBB penetration is needed for synthetic ligands to reach these receptors (Nitzsche et al., 2021). S1PR1 maintained endothelial function during cerebral ischemia as endothelial cell S1pr1 knockout (S1pr1ECKO) mice showed impaired microvascular perfusion after pMCAO (Nitzsche et al., 2021). Moreover, failure of collateral formation was found in S1pr1ECKO mice after pMCAO (Nitzsche et al., 2021). Based on these findings, the study showed that a S1PR1-selective agonist which can readily penetrate BBB targeting at endothelial S1PR1 receptor pool provided protection against ischemic injury (Nitzsche et al., 2021). And this beneficial effect was independent of reperfusion, which was in contrast to that of lymphocytopenia.
S1P Regulation of BBB Integrity
Lying between peripheral circulation and the brain parenchyma, BBB serves as both structural and metabolic barriers that restrict the access of many compounds while keeping the transport of nutrients and oxygen to the brain, thus maintaining the extracellular environment (Profaci et al., 2020). BBB permeability is controlled by cerebral endothelial cells along with pericytes and astrocytes through the presence of endothelial tight junctions (TJ), efflux and solute transporters, and low levels of transcytosis (Obermeier et al., 2013). During reperfusion, oxidative stress-induced BBB disruption causes vascular leakage which leads to vasogenic edema and aggravated brain damage. Moreover, extreme barrier disruption will result in intracerebral hemorrhage (Jickling et al., 2014). In experimental stroke studies, BBB opening is biphasic. A partial recovery was found between the initial and second increase in BBB permeability (Pillai et al., 2009). Two different mechanisms participated in this process, beginning with upregulation of endothelial transcytosis (6 h after tMCAO). Dynamic remodeling of TJ complexes forming gaps or protrusions is not obvious until in the late phase (24–48 h after tMCAO) (Knowland et al., 2014).
S1P has been reported to inhibit the function of P-glycoprotein at the BBB (Cannon et al., 2012). Since P-glycoprotein is an efflux pump for small-molecule drugs, S1P might therefore enhance drug transportation across the BBB (Cannon et al., 2012). S1pr1ECKO mice showed a size-selective BBB disruption and different subcellular distribution of tight junctional proteins in brain microvessels (Yanagida et al., 2017). Thus, brain endothelial S1PR1 may help maintain BBB function by sustaining a proper distribution of tight junction proteins. During ischemia, endothelial cell S1P signaling also plays important roles. Profound edema can be seen in S1pr1ECKO mice shortly after tMCAO by MRI (Nitzsche et al., 2021), which suggests that endothelial S1PR1 may participate in regulating vesicular transport in the early phase of BBB opening. In line with this, in brain arterioles, ApoM-S1P regulates vesicular transport through S1PR1 signaling (Janiurek et al., 2019). In contrast, S1PR2 was shown to induce cerebrovascular permeability in tMCAO mice by experiments using genetic approaches and a S1PR2 antagonist (Kim et al., 2015).
S1P Signaling in Functional Recovery Following an Ischemic Stroke
A series of events such as neurogenesis, synaptogenesis, angiogenesis, and white matter remodeling (Sommer and Schabitz, 2021) happen after ischemic stroke that contribute to neural repair and functional recovery (Overman and Carmichael, 2014). S1P signaling could also be involved in these events. The migration of neural progenitor cells (NPC) is an important step in neurogenesis (Koh and Park, 2017). S1P has been shown as a chemoattractant for NPCs released from infarction zone and S1PR2 antagonism enhanced the migration of NPCs toward the infarcted area thus facilitating neurogenesis (Kimura et al., 2008). Besides, fingolimod was found to enhance angiogenesis in a photothrombotic stroke model (Shang et al., 2020). In another photothrombotic stroke model, fingolimod significantly decreased astrogliosis and increased post-synaptic densities up to a month after the onset of ischemia (Brunkhorst et al., 2013). Other roles of S1P signaling in functional recovery following an ischemic stroke are poorly understood and shall constitute a promising direction for future studies.
The contributions of S1P signaling in ischemic stroke are primarily through diverse mechanisms as mentioned above. Additional mechanisms warrant further exploration. Some researches supported a possible direct action of S1P signaling on neuronal function after ischemic stroke (Hasegawa et al., 2010, 2013), while others proposed that such influence might be limited. As fingolimod, an analog of sphingosine, penetrated the blood-brain barrier, but it was not primarily located in neurons (Miron et al., 2008). Besides, in neuronal cell cultures under hypoxic conditions, fingolimod did not reduce cell death (Kraft et al., 2013). With more studies being undertaken, joint targeting of diverse mechanisms is suggested.
Insights Into Current and Future Therapeutic Perspectives
Fingolimod
Fingolimod (Gilenya™, Novartis), is an analog of sphingosine and was the first oral treatment approved by the United States Food and Drug Administration for RRMS (Brinkmann et al., 2010). Fingolimod is phosphorylated by Sphk2 to fingolimod-phosphate (fingolimod-P) (Zheng et al., 2015), which is an agonist for four S1PR (S1PR1, 3, 4, and 5) (Brinkmann et al., 2002). Fingolimod-P activates S1P receptors with very high potency and efficacy. The over-activation leads to rapid receptor internalization and degradation, especially for S1PR1 (Cyster and Schwab, 2012). Thus, fingolimod serves as a functional antagonist of S1PR1. While S1PR3, 4, 5 are also internalized but can come back to the cell surface without being degraded (Huwiler and Zangemeister-Wittke, 2018).
The mechanism of action of fingolimod in ischemic stroke is mainly mediated by the functional antagonism and agonism of S1P receptors (Wang et al., 2020). Fingolimod binds to S1PR1 on lymphocytes and leads to transient receptor degradation, thereby preventing lymphocytes from releasing to the bloodstream (Brinkmann, 2007). As discussed above, this decreases post-stroke lymphocyte infiltration and therefore thrombo-inflammation formation and inflammatory response. Besides its role in immunomodulation, fingolimod can also enhance the endothelial barrier function (Peng et al., 2004; Dudek et al., 2007). However, this effect is likely to be dose-dependent, as higher concentrations or prolonged fingolimod treatment increase endothelial permeability and vascular leakage instead (Shea et al., 2010; Muller et al., 2011). Fingolimod is highly lipophilic and can cross the BBB so it may exert a direct effect on CNS (Fu et al., 2015).
Based on previous studies that fingolimod reduced IR-induced tissue injury in the kidney (Troncoso et al., 2001) and liver (Anselmo et al., 2002), animal models of stroke were performed (Table 1). Most results indicated a beneficial role of fingolimod in tMCAO (Czech et al., 2009; Shichita et al., 2009; Wacker et al., 2009; Hasegawa et al., 2010; Pfeilschifter et al., 2011a,b; Wei et al., 2011; Kraft et al., 2013), thromboembolic stroke model (Campos et al., 2013) and photothrombotic stroke model (Brunkhorst et al., 2013; Li X. et al., 2017; Shang et al., 2020). In contrast, a few negative results were found in a pMCAO model (Liesz et al., 2011) and a large hemispheric stroke model when co-administration with rt-PA (Cai et al., 2013).
Encouraged by favorable preclinical data, clinical trials on fingolimod in patients with ischemic stroke have also been conducted. In an open-label pilot trial of patients presented beyond the 4.5 h time window for thrombolytic therapy with anterior circulation infarction, oral fingolimod was given 0.5 mg daily for 3 consecutive days (Fu et al., 2014). Results showed that from baseline to 7 days, enlargement of infarct volume was more restricted and microvascular permeability was reduced in patients who received fingolimod plus standard treatment than in patients receiving standard treatment alone (Fu et al., 2014). Fingolimod treatment was also associated with better neurological function recovery (Fu et al., 2014). In a multi-center trial of patients with anterior or middle cerebral artery occlusion, alteplase plus oral fingolimod 0.5 mg daily for 3 consecutive days were given (Zhu et al., 2015). Patients who received the combination therapy had smaller lesion volume at day 1 and better clinical outcomes at day 90 than patients who received solely alteplase (Zhu et al., 2015). In extended time windows from 4.5–6 h post stroke, patients receiving fingolimod along with alteplase also had a favorable shift of 90-day modified Rankin Scale score (Tian et al., 2018). No adverse effect was reported in the clinical trials mentioned above. More studies aiming to determine whether fingolimod enhances the action of endovascular treatment (NCT04629872) and a combination of fingolimod with alteplase in conjunction with thrombectomy (NCT04675762) are underway. Although the available clinical data are promising, it is noteworthy that current trials were not double-blinded and the number of participants was small and included mainly Asian patients. Therefore, it is still too early to confirm the role of fingolimod in the treatment of ischemic stroke. Additional large-scale, well-designed experiments are warranted.
Other S1PR Modulators
As potential substitutes to fingolimod, other S1PR modulators with better specificity, improved pharmacokinetic properties are under study. SEW2871 and LASW1238 are selective agonists of S1PR1 and were shown to reduce infarct volume after tMCAO (Hasegawa et al., 2010; Brait et al., 2016). CYM-5442 is another S1PR1-selective agonist reported to have preferential distribution to the brain after systemic administration (Gonzalez-Cabrera et al., 2012). Besides, CYM-5442 is shown to induce a shorter duration of lymphopenia than fingolimod (Gonzalez-Cabrera et al., 2012). In a recent experiment of a pMCAO model, CYM-5442 reduced 24-hour infarct size when administered 0-6 hours after occlusion and the beneficial effect was demonstrated to depend on endothelial S1PR1. In contrast, in another tMCAO model, siponimod, a S1PR modulator of S1PR1 and S1PR5, did not reduce stroke size in middle-aged mice despite significant lymphopenia. More basic and clinical researches are in progress to dig deeper into the therapeutic potential of other S1PR modulators for ischemic stroke.
Future Therapeutic Perspectives
The Underlying Mechanisms of S1P Signaling in Ischemic Stroke Need Further Study
S1P modulators have shown promise in experimental models and some small-scale clinical trials of stroke. Yet their mechanisms of action are not fully understood. Optimal targeting strategies remain to be defined. A recent study revealed the pivotal role of endothelial S1P signaling in supporting BBB and maintaining perfusion in the penumbra area of ischemic stroke (Nitzsche et al., 2021). This argues against the application of functional S1PR1 antagonists, which may also block endothelial S1P signaling. Since most S1PR1 agonists also induce lymphopenia (Brait et al., 2016; Nitzsche et al., 2021), joint targeting of lymphocyte and endothelial cell receptors with S1PR1 agonists is suggested. Future application of S1P modulation in ischemic stroke treatment depends on an in-depth understanding of the mechanism of action, and on the ability to have a fine temporal and spatial regulation of the signal pathway. With more studies being undertaken, strategies for developing new-generation drugs with superior attributes will be provided.
To Develop More Specific S1PR Modulators and Seek Other Targets in S1P Signaling Pathway in Ischemic Stroke Treatment
Fingolimod, the S1PR modulator used in most preclinical and clinical studies so far, is non-specific and desensitizes brain endothelial S1PR1 at high doses as discussed above. Moreover, it induces long-lasting lymphopenia (Gonzalez-Cabrera et al., 2012). As the contribution of different S1PR on ischemic stroke is not fully elucidated (Brait et al., 2016), drugs with more specificity could be not only safer but also more efficacious. Besides S1PR, S1P signaling is providing more therapeutic targets such as S1P transporters and metabolic enzymes. New biased S1PR agonists have been developed that do not cause receptor desensitization and do not induce lymphopenia (Poirier et al., 2020). ApoM-Fc is a soluble carrier for S1P, which can specifically activate endothelial S1P receptors but do not influence circulating lymphocyte numbers (Swendeman et al., 2017). With genetic approaches being applied, the roles of SphK and other enzymes in S1P signaling shall be further elucidated (Gaire and Choi, 2021). New pharmacological advances can be expected.
Timing, Drug Formulation Shall Be Considered and Catering to the Therapeutic Strategy of Ischemic Stroke
The appropriate timing for drug administration is vital for ischemic stroke treatment, especially when considering the narrow time window for thrombolytic therapy and dramatically different series of events occurring at different time points after stroke. Medications for stroke treatment therefore shall be fast-acting. As for fingolimod, lymphopenia is induced within 6 h of the first dose and the lymphocyte count readily returns to baseline within 72 h of the last dose (Fu et al., 2015). Treatment duration is another problem in need of attention. A study showed that S1P1 agonist LASW1238 reduced infarct size in a tMCAO mouse model only when the lymphopenia state was induced for 24 hours. Defining the best dose and duration of drugs to ensure sufficient but not excessive lymphopenia is thus critical (Brait et al., 2016). Besides, the optimal route of drug delivery should also be considered. In cases of severe stroke, intravenous administration is preferable to oral administration for the impaired ability of swallowing and absorption in these patients (Fu et al., 2015).
To Reveal the Role of S1P Signaling in Functional Recovery and Post-stroke Sequelae
As mentioned above, the role of S1P signaling in neurogenesis, synaptogenesis, angiogenesis, white matter remodeling, and other processes of functional recovery after stroke is poorly studied. Besides, due to a reduced mortality of ischemic stroke, stroke prevalence is on the rise. Other than sensorimotor deficits, neuropsychiatric sequelae such as depression dramatically reduce the quality of life. In light of this, assessing the role of S1P signaling in post-stroke sequelae is promising.
Methodological Quality of Preclinical Trials Shall Be Improved and Close Cooperation Between Preclinical and Clinical Studies Are Called For
Although there are dozens of preclinical trials on S1P modulators in ischemic stroke treatment as described above, it should be noted that these experiments are heterogeneous in design. The ischemic stroke models, occlusion times, doses of drug, timing, and duration of treatment, route of administration are different (Table 1). Methodological problems might limit the effective translation from bench to bedside. In light of this, preclinical studies should be better designed to incorporate sex, age, and comorbidity factors. On the other hand, problems encountered in clinical trials can be brought back to mechanism research. The close cooperation between preclinical and clinical studies would be valuable to bring new insight into S1P signaling modulation in ischemic stroke treatment.
Conclusion
Numerous preclinical and clinical studies suggest that S1P signaling pathway is actively engaged in ischemic stroke pathology. Several small-scale clinical trials investigating the effect of fingolimod in ischemic stroke patients have shown promising results. Other S1PR modulators with better specificity and pharmacokinetic property are under development. Synergistic interaction between preclinical and clinical studies would help to achieve new pharmacological advances in ischemia stroke treatment.
Author Contributions
S-QZ drafted the initial version of the manuscript. JX, MC, L-QZ, and KS collected information and edited the manuscript. CQ and D-ST directed the work, reviewed and edited the manuscript. All authors contributed to the article and approved it for publication.
Funding
This work was supported by the National Natural Science Foundation of China (Grants: 82071380, 81873743, and 81801223), and Tongji Hospital (HUST) Foundation for Excellent Young Scientist (Grant No. 2020YQ06).
Conflict of Interest
The authors declare that the research was conducted in the absence of any commercial or financial relationships that could be construed as a potential conflict of interest.
Publisher’s Note
All claims expressed in this article are solely those of the authors and do not necessarily represent those of their affiliated organizations, or those of the publisher, the editors and the reviewers. Any product that may be evaluated in this article, or claim that may be made by its manufacturer, is not guaranteed or endorsed by the publisher.
References
Anselmo, D., Amersi, F. F., Shen, X. D., Gao, F., Katori, M., Ke, B., et al. (2002). FTY720: a novel approach to the treatment of hepatic ischemia-reperfusion injury. Transplant Proc. 34, 1467–1468. doi: 10.1016/s0041-1345(02)02933-0
Baeyens, A., Bracero, S., Chaluvadi, V. S., Khodadadi-Jamayran, A., Cammer, M., and Schwab, S. R. (2021). Monocyte-derived S1P in the lymph node regulates immune responses. Nature 592, 290–295.
Baeyens, A., Fang, V., Chen, C., and Schwab, S. R. (2015). Exit strategies: S1P signaling and T cell migration. Trends Immunol. 36, 778–787. doi: 10.1016/j.it.2015.10.005
Benarroch, E. E. (2021). What is the role of Sphingosine-1-Phosphate receptors in pain? Neurology 96, 525–528.
Bonnin, P., Mazighi, M., Charriaut-Marlangue, C., and Kubis, N. (2019). Early collateral recruitment after stroke in infants and adults mechanisms and therapeutic opportunities. Stroke 50, 2604–2611.
Brait, V. H., Tarrason, G., Gavalda, A., Godessart, N., and Planas, A. M. (2016). Selective Sphingosine 1-Phosphate receptor 1 agonist is protective against ischemia/reperfusion in mice. Stroke 47, 3053–3056.
Brinkmann, V. (2007). Sphingosine 1-phosphate receptors in health and disease: mechanistic insights from gene deletion studies and reverse pharmacology. Pharmacol. Ther. 115, 84–105. doi: 10.1016/j.pharmthera.2007.04.006
Brinkmann, V., Billich, A., Baumruker, T., Heining, P., Schmouder, R., Francis, G., et al. (2010). Fingolimod (FTY720): discovery and development of an oral drug to treat multiple sclerosis. Nat. Rev. Drug Discov. 9, 883–897.
Brinkmann, V., Davis, M. D., Heise, C. E., Albert, R., Cottens, S., Hof, R., et al. (2002). The immune modulator FTY720 targets sphingosine 1-phosphate receptors. J. Biol. Chem. 277, 21453–21457.
Brunkhorst, R., Kanaan, N., Koch, A., Ferreiros, N., Mirceska, A., Zeiner, P., et al. (2013). FTY720 treatment in the convalescence period improves functional recovery and reduces reactive astrogliosis in photothrombotic stroke. PLoS One 8:e70124. doi: 10.1371/journal.pone.0070124
Cai, A., Schlunk, F., Bohmann, F., Kashefiolasl, S., Brunkhorst, R., Foerch, C., et al. (2013). Coadministration of FTY720 and rt-PA in an experimental model of large hemispheric stroke-no influence on functional outcome and blood-brain barrier disruption. Exp. Transl. Stroke Med. 5:11. doi: 10.1186/2040-7378-5-11
Camerer, E., Regard, J. B., Cornelissen, I., Srinivasan, Y., Duong, D. N., Palmer, D., et al. (2009). Sphingosine-1-phosphate in the plasma compartment regulates basal and inflammation-induced vascular leak in mice. J. Clin. Investig. 119, 1871–1879. doi: 10.1172/jci38575
Campos, F., Qin, T., Castillo, J., Seo, J. H., Arai, K., Lo, E. H., et al. (2013). Fingolimod reduces hemorrhagic transformation associated with delayed tissue plasminogen activator treatment in a mouse thromboembolic model. Stroke 44, 505–511. doi: 10.1161/STROKEAHA.112.679043
Cannon, R. E., Peart, J. C., Hawkins, B. T., Campos, C. R., and Miller, D. S. (2012). Targeting blood-brain barrier sphingolipid signaling reduces basal P-glycoprotein activity and improves drug delivery to the brain. Proc. Natl. Acad. Sci. USA 109, 15930–15935. doi: 10.1073/pnas.1203534109
Cantalupo, A., Gargiulo, A., Dautaj, E., Liu, C., Zhang, Y., Hla, T., et al. (2017). S1PR1 (Sphingosine-1-Phosphate Receptor 1) signaling regulates blood flow and pressure. Hypertension 70, 426–434. doi: 10.1161/HYPERTENSIONAHA.117.09088
Cartier, A., and Hla, T. (2019). Sphingosine 1-phosphate: lipid signaling in pathology and therapy. Science 366:eaar5551.
Chua, X. Y., Ho, L. T. Y., Xiang, P., Chew, W. S., Lam, B. W. S., Chen, C. P., et al. (2021). Preclinical and clinical evidence for the involvement of Sphingosine 1-phosphate signaling in the pathophysiology of vascular cognitive impairment. Neuromolcular Med. 23, 47–67. doi: 10.1007/s12017-020-08632-0
Chun, J., Hla, T., Lynch, K. R., Spiegel, S., and Moolenaar, W. H. (2010). International union of basic and clinical pharmacology. LXXVIII. Lysophospholipid Receptor Nomenclature. Pharmacol. Rev. 62, 579–587. doi: 10.1124/pr.110.003111
Cyster, J. G., and Schwab, S. R. (2012). Sphingosine-1-Phosphate and lymphocyte egress from lymphoid organs. Annu. Rev. Immunol. 30, 69–94.
Czech, B., Pfeilschifter, W., Mazaheri-Omrani, N., Strobel, M. A., Kahles, T., Neumann-Haefelin, T., et al. (2009). The immunomodulatory sphingosine 1-phosphate analog FTY720 reduces lesion size and improves neurological outcome in a mouse model of cerebral ischemia. Biochem. Biophys. Res. Commun. 389, 251–256. doi: 10.1016/j.bbrc.2009.08.142
di Nuzzo, L., Orlando, R., Nasca, C., and Nicoletti, F. (2014). Molecular pharmacodynamics of new oral drugs used in the treatment of multiple sclerosis. Drug Des. Devel Ther. 8, 555–568.
Dreikorn, M., Milacic, Z., Pavlovic, V., Meuth, S. G., Kleinschnitz, C., and Kraft, P. (2018). Immunotherapy of experimental and human stroke with agents approved for multiple sclerosis: a systematic review. Ther. Adv. Neurol. Disord. 11:1756286418770626. doi: 10.1177/1756286418770626
Dudek, S. M., Camp, S. M., Chiang, E. T., Singleton, P. A., Usatyuk, P. V., Zhao, Y., et al. (2007). Pulmonary endothelial cell barrier enhancement by FTY720 does not require the S1P1 receptor. Cell. Signal. 19, 1754–1764.
Dusaban, S. S., Chun, J., Rosen, H., Purcell, N. H., and Brown, J. H. (2017). Sphingosine 1-phosphate receptor 3 and RhoA signaling mediate inflammatory gene expression in astrocytes. J. Neuroinflamm. 14:111.
Ekker, M. S., Boot, E. M., Singhal, A. B., Tan, K. S., Debette, S., Tuladhar, A. M., et al. (2018). Epidemiology, aetiology, and management of ischaemic stroke in young adults. Lancet Neurol. 17, 790–801.
Fu, Y., Liu, Q., Anrather, J., and Shi, F. D. (2015). Immune interventions in stroke. Nat. Rev. Neurol. 11, 524–535.
Fu, Y., Zhang, N., Ren, L., Yan, Y. P., Sun, N., Li, Y. J., et al. (2014). Impact of an immune modulator fingolimod on acute ischemic stroke. Proc. Natl. Acad. Sci. USA 111, 18315–18320. doi: 10.1073/pnas.1416166111
Gaire, B. P., and Choi, J. W. (2021). Sphingosine 1-Phosphate receptors in cerebral ischemia. Neuromolecular Med. 23, 211–223.
Gaire, B. P., Bae, Y. J., and Choi, J. W. (2019). S1P1 regulates M1/M2 polarization toward brain injury after transient focal cerebral ischemia. Biomol. Ther. 27, 522–529. doi: 10.4062/biomolther.2019.005
Gaire, B. P., Song, M. R., and Choi, J. W. (2018b). Sphingosine 1-phosphate receptor subtype 3 (S1P3) contributes to brain injury after transient focal cerebral ischemia via modulating microglial activation and their M1 polarization. J. Neuroinflamm. 15:284. doi: 10.1186/s12974-018-1323-1
Gaire, B. P., Lee, C. H., Sapkota, A., Lee, S. Y., Chun, J., Cho, H. J., et al. (2018a). Identification of Sphingosine 1-Phosphate Receptor Subtype 1 (S1P1) as a pathogenic factor in transient focal cerebral ischemia. Mol. Neurobiol. 55, 2320–2332. doi: 10.1007/s12035-017-0468-8
Gazit, S. L., Mariko, B., Therond, P., Decouture, B., Xiong, Y. Q., Couty, L., et al. (2016). Platelet and erythrocyte sources of S1P are redundant for vascular development and homeostasis, but both rendered essential after plasma s1p depletion in anaphylactic shock. Circ. Res. 119, E110–E126. doi: 10.1161/CIRCRESAHA.116.308929
Gonzalez-Cabrera, P. J., Cahalan, S. M., Nguyen, N., Sarkisyan, G., Leaf, N. B., Cameron, M. D., et al. (2012). S1P(1) receptor modulation with cyclical recovery from lymphopenia ameliorates mouse model of multiple sclerosis. Mol. Pharmacol. 81, 166–174. doi: 10.1124/mol.111.076109
Hannun, Y. A., and Obeid, L. M. (2018). Sphingolipids and their metabolism in physiology and disease. Nat. Rev. Mol. Cell Biol. 19, 175–191.
Hasegawa, Y., Suzuki, H., Altay, O., Rolland, W., and Zhang, J. H. (2013). Role of the sphingosine metabolism pathway on neurons against experimental cerebral ischemia in rats. Transl. Stroke Res. 4, 524–532. doi: 10.1007/s12975-013-0260-7
Hasegawa, Y., Suzuki, H., Sozen, T., Rolland, W., and Zhang, J. H. (2010). Activation of sphingosine 1-phosphate receptor-1 by FTY720 is neuroprotective after ischemic stroke in rats. Stroke 41, 368–374.
Hu, X. M., De Silva, T. M., Chen, J., and Faraci, F. M. (2017). Cerebral Vascular Disease and neurovascular injury in ischemic stroke. Circ. Res. 120, 449–471.
Huwiler, A., and Zangemeister-Wittke, U. (2018). The sphingosine 1-phosphate receptor modulator fingolimod as a therapeutic agent: recent findings and new perspectives. Pharmacol. Ther. 185, 34–49. doi: 10.1016/j.pharmthera.2017.11.001
Ishikawa, M., Cooper, D., Russell, J., Salter, J. W., Zhang, J. H., Nanda, A., et al. (2003). Molecular determinants of the prothrombogenic and inflammatory phenotype assumed by the postischemic cerebral microcirculation. Stroke 34, 1777–1782. doi: 10.1161/01.STR.0000074921.17767.F2
Janiurek, M. M., Soylu-Kucharz, R., Christoffersen, C., Kucharz, K., and Lauritzen, M. (2019). Apolipoprotein M-bound sphingosine-1-phosphate regulates blood-brain barrier paracellular permeability and transcytosis. Elife 8:e49405. doi: 10.7554/eLife.49405
Ji, J., Wang, J., Yang, J., Wang, X. P., Huang, J. J., Xue, T. F., et al. (2019). The Intra-nuclear SphK2-S1P axis facilitates M1-to-M2 shift of microglia via suppressing HDAC1-Mediated KLF4 Deacetylation. Front. Immunol. 10:1241. doi: 10.3389/fimmu.2019.01241
Jickling, G. C., Liu, D. Z., Stamova, B., Ander, B. P., Zhan, X. H., Lu, A. G., et al. (2014). Hemorrhagic transformation after ischemic stroke in animals and humans. J. Cereb. Blood Flow Metab. 34, 185–199.
Johnson, C. O., Nguyen, M., Roth, G. A., Nichols, E., Alam, T., Abate, D., et al. (2019). Global, regional, and national burden of stroke, 1990-2016: a systematic analysis for the Global Burden of Disease Study 2016. Lancet Neurol. 18, 439–458.
Kim, G. S., Yang, L., Zhang, G. Q., Zhao, H. G., Selim, M., McCullough, L. D., et al. (2015). Critical role of sphingosine-1-phosphate receptor-2 in the disruption of cerebrovascular integrity in experimental stroke. Nat. Commun. 6:7893. doi: 10.1038/ncomms8893
Kimura, A., Ohmori, T., Kashiwakura, Y., Ohkawa, R., Madoiwa, S., Mimuro, J., et al. (2008). Antagonism of Sphingosine 1-Phosphate Receptor-2 enhances migration of neural progenitor cells toward an area of brain infarction. Stroke 39, 3411–3417. doi: 10.1161/STROKEAHA.108.514612
Knowland, D., Arac, A., Sekiguchi, K. J., Hsu, M., Lutz, S. E., Perrino, J., et al. (2014). Stepwise recruitment of transcellular and paracellular pathways underlies blood-brain barrier breakdown in stroke. Neuron 82, 603–617. doi: 10.1016/j.neuron.2014.03.003
Kraft, P., Gob, E., Schuhmann, M. K., Gobel, K., Deppermann, C., Thielmann, I., et al. (2013). FTY720 ameliorates acute ischemic stroke in mice by reducing thrombo-inflammation but not by direct neuroprotection. Stroke 44, 3202–3210. doi: 10.1161/STROKEAHA.113.002880
Lambertsen, K. L., Finsen, B., and Clausen, B. H. (2018). Post-stroke inflammation—target or tool for therapy? Acta Neuropathol. 137, 693–714.
Levine, D. A., Galecki, A. T., Langa, K. M., Unverzagt, F. W., Kabeto, M. U., Giordani, B., et al. (2015). Trajectory of cognitive decline after incident stroke. JAMA 314, 41–51.
Li, M., Li, Z., Yao, Y., Jin, W.-N., Wood, K., Liu, Q., et al. (2017). Astrocyte-derived interleukin-15 exacerbates ischemic brain injury via propagation of cellular immunity. Proc. Natl. Acad. Sci. U.S.A. 114, E396–E405. doi: 10.1073/pnas.1612930114
Li, X., Wang, M. H., Qin, C., Fan, W. H., Tian, D. S., and Liu, J. L. (2017). Fingolimod suppresses neuronal autophagy through the mTOR/p70S6K pathway and alleviates ischemic brain damage in mice. PLoS One 12:e0188748. doi: 10.1371/journal.pone.0188748
Li, Y. J., Shi, S. X., Liu, Q., Shi, F. D., and Gonzales, R. J. (2020). Targeted role for sphingosine-1-phosphate receptor 1 in cerebrovascular integrity and inflammation during acute ischemic stroke. Neurosci. Lett. 735:135160. doi: 10.1016/j.neulet.2020.135160
Liddelow, S. A., Marsh, S. E., and Stevens, B. (2020). Microglia and astrocytes in disease: dynamic duo or partners in crime? Trends Immunol. 41, 820–835.
Liesz, A., Sun, L., Zhou, W., Schwarting, S., Mracsko, E., Zorn, M., et al. (2011). FTY720 reduces post-ischemic brain lymphocyte influx but does not improve outcome in permanent murine cerebral ischemia. PLoS One 6:e21312. doi: 10.1371/journal.pone.0021312
Ma, Y., Wang, J., Wang, Y., and Yang, G. Y. (2017). The biphasic function of microglia in ischemic stroke. Prog. Neurobiol. 157, 247–272.
Manning, N. W., Campbell, B. C. V., Oxley, T. J., and Chapot, R. (2014). Acute ischemic stroke time. penumbra, and reperfusion. Stroke 45, 640–644.
Meinel, T. R., Kaesmacher, J., Mosimann, P. J., Seiffge, D., Jung, S., Mordasini, P., et al. (2020). Association of initial imaging modality and futile recanalization after thrombectomy. Neurology 95, e2331–e2342.
Merrill, A. H. (2011). Sphingolipid and glycosphingolipid metabolic pathways in the Era of Sphingolipidomics. Chem. Rev. 111, 6387–6422. doi: 10.1021/cr2002917
Miron, V. E., Schubart, A., and Antel, J. P. (2008). Central nervous system-directed effects of FTY720 (fingolimod). J. Neurol. Sci. 274, 13–17. doi: 10.1016/j.jns.2008.06.031
Mizugishi, K., Yamashita, T., Olivera, A., Miller, G. F., Spiegel, S., and Proia, R. L. (2005). Essential role for sphingosine kinases in neural and vascular development. Mol. Cell. Biol. 25, 11113–11121. doi: 10.1128/MCB.25.24.11113-11121.2005
Mizuma, A., You, J. S., and Yenari, M. A. (2018). Targeting reperfusion injury in the age of mechanical thrombectomy. Stroke 49, 1796–1802. doi: 10.1161/STROKEAHA.117.017286
Moon, E., Han, J. E., Jeon, S., Ryu, J. H., Choi, J. W., and Chun, J. (2015). Exogenous S1P exposure potentiates ischemic stroke damage that is reduced possibly by inhibiting S1P receptor signaling. Mediators Inflamm. 2015:492659. doi: 10.1155/2015/492659
Muller, H. C., Hocke, A. C., Hellwig, K., Gutbier, B., Peters, H., Schonrock, S. M., et al. (2011). The Sphingosine-1 Phosphate receptor agonist FTY720 dose dependently affected endothelial integrity in vitro and aggravated ventilator-induced lung injury in mice. Pulm. Pharmacol. Ther. 24, 377–385. doi: 10.1016/j.pupt.2011.01.017
Nakagawa, S., and Aruga, J. (2020). Sphingosine 1-Phosphate signaling is involved in impaired blood-brain barrier function in ischemia-reperfusion injury. Mol. Neurobiol. 57, 1594–1606. doi: 10.1007/s12035-019-01844-x
Nayak, D., Huo, Y., Kwang, W. X., Pushparaj, P. N., Kumar, S. D., Ling, E. A., et al. (2010). Sphingosine kinase 1 regulates the expression of proinflammatory cytokines and nitric oxide in activated microglia. Neuroscience 166, 132–144.
Nazari, M., Keshavarz, S., Rafati, A., Namavar, M. R., and Haghani, M. (2016). Fingolimod (FTY720) improves hippocampal synaptic plasticity and memory deficit in rats following focal cerebral ischemia. Brain Res. Bull. 124, 95–102. doi: 10.1016/j.brainresbull.2016.04.004
Nitzsche, A., Poittevin, M., Benarab, A., Bonnin, P., Faraco, G., Uchida, H., et al. (2021). Endothelial S1P1 signaling counteracts infarct expansion in ischemic stroke. Circ. Res. 128, 363–382. doi: 10.1161/CIRCRESAHA.120.316711
Obermeier, B., Daneman, R., and Ransohoff, R. M. (2013). Development, maintenance and disruption of the blood-brain barrier. Nat. Med. 19, 1584–1596.
Overman, J. J., and Carmichael, S. T. (2014). Plasticity in the injured brain: more than molecules matter. Neuroscientist 20, 15–28. doi: 10.1177/1073858413491146
Peng, X., Hassoun, P. M., Sammani, S., McVerry, B. J., Burne, M. J., Rabb, H., et al. (2004). Protective effects of sphingosine 1-phosphate in murine endotoxin-induced inflammatory lung injury. Am. J. Respir. Crit. Care Med. 169, 1245–1251. doi: 10.1164/rccm.200309-1258OC
Pfeilschifter, W., Czech-Zechmeister, B., Sujak, M., Foerch, C., Wichelhaus, T. A., and Pfeilschifter, J. (2011a). Treatment with the immunomodulator FTY720 does not promote spontaneous bacterial infections after experimental stroke in mice. Exp. Transl. Stroke Med. 3:2.
Pfeilschifter, W., Czech-Zechmeister, B., Sujak, M., Mirceska, A., Koch, A., Rami, A., et al. (2011b). Activation of sphingosine kinase 2 is an endogenous protective mechanism in cerebral ischemia. Biochem. Biophys. Res. Commun. 413, 212–217.
Pillai, D. R., Dittmar, M. S., Baldaranov, D., Heidemann, R. M., Henning, E. C., Schuierer, G., et al. (2009). Cerebral ischemia-reperfusion injury in rats-A 3 T MRI study on biphasic blood-brain barrier opening and the dynamics of edema formation. J. Cereb. Blood Flow Metab. 29, 1846–1855. doi: 10.1038/jcbfm.2009.106
Poirier, B., Briand, V., Kadereit, D., Schafer, M., Wohlfart, P., Philippo, M. C., et al. (2020). A G protein-biased S1P1 agonist. SAR247799, protects endothelial cells without affecting lymphocyte numbers. Sci. Signal. 13:eaax8050. doi: 10.1126/scisignal.aax8050
Powers (2019). Guidelines for the early management of patients with acute ischemic stroke: 2019 Update to the 2018 guidelines for the early management of acute ischemic stroke: a guideline for healthcare professionals from the American Heart Association/American Stroke Association. Stroke 50, E440–E441.
Profaci, C. P., Munji, R. N., Pulido, R. S., and Daneman, R. (2020). The blood-brain barrier in health and disease: important unanswered questions. J. Exp. Med. 217:e20190062. doi: 10.1084/jem.20190062
Proia, R. L., and Hla, T. (2015). Emerging biology of sphingosine-1-phosphate: its role in pathogenesis and therapy. J. Clin. Investig. 125, 1379–1387.
Qin, C., Fan, W. H., Liu, Q., Shang, K., Murugan, M., Wu, L. J., et al. (2017). Fingolimod protects against ischemic white matter damage by modulating microglia toward M2 polarization via STAT3 pathway. Stroke 48, 3336–3346. doi: 10.1161/STROKEAHA.117.018505
Rinaldo, L., Rabinstein, A. A., Cloft, H., Knudsen, J. M., Castilla, L. R., and Brinjikji, W. (2019). Racial and ethnic disparities in the utilization of thrombectomy for acute stroke analysis of data from 2016 to 2018. Stroke 50, 2428–2432.
Salas-Perdomo, A., Miro-Mur, F., Gallizioli, M., Brait, V. H., Justicia, C., Meissner, A., et al. (2019). Role of the S1P pathway and inhibition by fingolimod in preventing hemorrhagic transformation after stroke. Sci. Rep. 9:8309. doi: 10.1038/s41598-019-44845-5
Sapkota, A., Gaire, B. P., Kang, M. G., and Choi, J. W. (2019). S1P2 contributes to microglial activation and M1 polarization following cerebral ischemia through ERK1/2 and JNK. Sci. Rep. 9:12106. doi: 10.1038/s41598-019-48609-z
Shang, K., He, J., Zou, J., Qin, C., Lin, L., Zhou, L. Q., et al. (2020). Fingolimod promotes angiogenesis and attenuates ischemic brain damage via modulating microglial polarization. Brain Res. 1726:146509. doi: 10.1016/j.brainres.2019.146509
Shea, B. S., Brooks, S. F., Fontaine, B. A., Chun, J., Luster, A. D., and Tager, A. M. (2010). Prolonged exposure to sphingosine 1-phosphate receptor-1 agonists exacerbates vascular leak, fibrosis, and mortality after lung injury. Am. J. Respir. Cell Mol. Biol. 43, 662–673. doi: 10.1165/rcmb.2009-0345OC
Shi, K., Tian, D.-C., Li, Z.-G., Ducruet, A. F., Lawton, M. T., and Shi, F.-D. (2019). Global brain inflammation in stroke. Lancet Neurol. 18, 1058–1066.
Shichita, T., Sugiyama, Y., Ooboshi, H., Sugimori, H., Nakagawa, R., Takada, I., et al. (2009). Pivotal role of cerebral interleukin-17-producing gammadeltaT cells in the delayed phase of ischemic brain injury. Nat. Med. 15, 946–950. doi: 10.1038/nm.1999
Shuaib, A., Butcher, K., Mohammad, A. A., Saqqur, M., and Liebeskind, D. S. (2011). Collateral blood vessels in acute ischaemic stroke: a potential therapeutic target. Lancet Neurol. 10, 909–921.
Sic, H., Kraus, H., Madl, J., Flittner, K. A., von Munchow, A. L., Pieper, K., et al. (2014). Sphingosine-1-phosphate receptors control B-cell migration through signaling components associated with primary immunodeficiencies, chronic lymphocytic leukemia, and multiple sclerosis. J. Allergy Clin. Immunol. 134, 420–428. doi: 10.1016/j.jaci.2014.01.037
Soliven, B., Miron, V., and Chun, J. (2011). The neurobiology of sphingosine 1-phosphate signaling and sphingosine 1-phosphate receptor modulators. Neurology 76(8 Suppl. 3), S9–S14.
Sommer, C. J., and Schabitz, W. R. (2021). Principles and requirements for stroke recovery science. J. Cereb. Blood Flow Metab. 41, 471–485.
Sorensen, S. D., Nicole, O., Peavy, R. D., Montoya, L. M., Lee, C. J., Murphy, T. J., et al. (2003). Common signaling pathways link activation of murine PAR-1. LPA, and S1P receptors to proliferation of astrocytes. Mol. Pharmacol. 64, 1199–1209. doi: 10.1124/mol.64.5.1199
Stoll, G., and Nieswandt, B. (2019). Thrombo-inflammation in acute ischaemic stroke - implications for treatment. Nat. Rev. Neurol. 15, 473–481. doi: 10.1038/s41582-019-0221-1
Swendeman, S. L., Xiong, Y., Cantalupo, A., Yuan, H., Burg, N., Hisano, Y., et al. (2017). An engineered S1P chaperone attenuates hypertension and ischemic injury. Sci. Signal. 10:eaal2722. doi: 10.1126/scisignal.aal2722
Tian, D. C., Shi, K., Zhu, Z., Yao, J., Yang, X., Su, L., et al. (2018). Fingolimod enhances the efficacy of delayed alteplase administration in acute ischemic stroke by promoting anterograde reperfusion and retrograde collateral flow. Ann. Neurol. 84, 717–728. doi: 10.1002/ana.25352
Troncoso, P., Ortiz, M., Martinez, L., and Kahan, B. D. (2001). FTY 720 prevents ischemic reperfusion damage in rat kidneys. Transplant Proc. 33, 857–859.
Virani, S. S., Alonso, A., Benjamin, E. J., Bittencourt, M. S., Callaway, C. W., Carson, A. P., et al. (2020). Heart disease and stroke statistics-2020 update: a report from the american heart association. Circulation 141, E139–E596. doi: 10.1161/CIR.0000000000000757
Wacker, B. K., Park, T. S., and Gidday, J. M. (2009). Hypoxic preconditioning-induced cerebral ischemic tolerance: role of microvascular sphingosine kinase 2. Stroke 40, 3342–3348. doi: 10.1161/STROKEAHA.109.560714
Wang, Z., Kawabori, M., and Houkin, K. (2020). FTY720 (Fingolimod) ameliorates brain injury through multiple mechanisms and is a strong candidate for stroke treatment. Curr. Med. Chem. 27, 2979–2993. doi: 10.2174/0929867326666190308133732
Wei, Y., Yemisci, M., Kim, H. H., Yung, L. M., Shin, H. K., Hwang, S. K., et al. (2011). Fingolimod provides long-term protection in rodent models of cerebral ischemia. Ann. Neurol. 69, 119–129.
Xiong, Y., Piao, W., Brinkman, C. C., Li, L., Kulinski, J. M., Olivera, A., et al. (2019). CD4 T cell sphingosine 1-phosphate receptor (S1PR)1 and S1PR4 and endothelial S1PR2 regulate afferent lymphatic migration. Sci. Immunol. 4:eaav1263. doi: 10.1126/sciimmunol.aav1263
Yanagida, K., Liu, C. H., Faraco, G., Galvani, S., Smith, H. K., Burg, N., et al. (2017). Size-selective opening of the blood–brain barrier by targeting endothelial sphingosine 1–phosphate receptor 1. Proc. Natl. Acad. Sci. U.S.A. 114, 4531–4536. doi: 10.1073/pnas.1618659114
Yilmaz, G., Arumugam, T. V., Stokes, K. Y., and Granger, D. N. (2006). Role of T lymphocytes and interferon-gamma in ischemic stroke. Circulation 113, 2105–2112.
Zheng, S., Wei, S., Wang, X., Xu, Y., Xiao, Y., Liu, H., et al. (2015). Sphingosine kinase 1 mediates neuroinflammation following cerebral ischemia. Exp. Neurol. 272, 160–169. doi: 10.1016/j.expneurol.2015.03.012
Keywords: sphingosine-1-phosphate, ischemic stroke, fingolimod, neuroprotective agent, translational research
Citation: Zhang S-Q, Xiao J, Chen M, Zhou L-Q, Shang K, Qin C and Tian D-S (2021) Sphingosine-1-Phosphate Signaling in Ischemic Stroke: From Bench to Bedside and Beyond. Front. Cell. Neurosci. 15:781098. doi: 10.3389/fncel.2021.781098
Received: 22 September 2021; Accepted: 08 November 2021;
Published: 30 November 2021.
Edited by:
Gaiqing Wang, The Third People’s Hospital of Hainan Province, ChinaCopyright © 2021 Zhang, Xiao, Chen, Zhou, Shang, Qin and Tian. This is an open-access article distributed under the terms of the Creative Commons Attribution License (CC BY). The use, distribution or reproduction in other forums is permitted, provided the original author(s) and the copyright owner(s) are credited and that the original publication in this journal is cited, in accordance with accepted academic practice. No use, distribution or reproduction is permitted which does not comply with these terms.
*Correspondence: Chuan Qin, Y2h1YW5xaW5AdGpoLnRqbXUuZWR1LmNu; Dai-Shi Tian, dGlhbmRzQHRqaC50am11LmVkdS5jbg==