- Translational and Functional Genomics Branch, National Human Genome Research Institute, National Institutes of Health, Bethesda, MD, United States
Millions of Americans experience hearing or balance disorders due to loss of hair cells in the inner ear. The hair cells are mechanosensory receptors used in the auditory and vestibular organs of all vertebrates as well as the lateral line systems of aquatic vertebrates. In zebrafish and other non-mammalian vertebrates, hair cells turnover during homeostasis and regenerate completely after being destroyed or damaged by acoustic or chemical exposure. However, in mammals, destroying or damaging hair cells results in permanent impairments to hearing or balance. We sought an improved method for studying hair cell damage and regeneration in adult aquatic vertebrates by generating a transgenic zebrafish with the capacity for targeted and inducible hair cell ablation in vivo. This model expresses the human diphtheria toxin receptor (hDTR) gene under the control of the myo6b promoter, resulting in hDTR expressed only in hair cells. Cell ablation is achieved by an intraperitoneal injection of diphtheria toxin (DT) in adult zebrafish or DT dissolved in the water for larvae. In the lateral line of 5 days post fertilization (dpf) zebrafish, ablation of hair cells by DT treatment occurred within 2 days in a dose-dependent manner. Similarly, in adult utricles and saccules, a single intraperitoneal injection of 0.05 ng DT caused complete loss of hair cells in the utricle and saccule by 5 days post-injection. Full hair cell regeneration was observed for the lateral line and the inner ear tissues. This study introduces a new method for efficient conditional hair cell ablation in adult zebrafish inner ear sensory epithelia (utricles and saccules) and demonstrates that zebrafish hair cells will regenerate in vivo after this treatment.
Introduction
Loss of hearing or balance can be debilitating and imposes a significant personal, societal, and economic burden upon individuals, their families, and communities. Approximately 37.5 million Americans report some degree of hearing loss with incidences increasing with age. About 33.4 million adults reported a problem during the past 12 months with balance, unsteadiness, or blurred vision after moving their head. Hearing and balance disorders are most often attributed to loss or damage to the sensory hair cells of the auditory and vestibular organs. The hair cells are mechanosensory receptors that receive signals from our environment and transmit them to the brain. These hair cells reside in the sensory epithelia of auditory and vestibular organs in all vertebrates as well as in the lateral line systems of aquatic vertebrates (Popper and Fay, 1993; Bever and Fekete, 2002; Nicolson, 2005). In zebrafish and non-mammalian vertebrates, hair cells turnover during homeostasis and regenerate completely after being damaged or destroyed by acoustic or chemical exposure, while in mammals, destroying or damaging hair cells results in permanent impairments to hearing and balance. Mammalian hair cell regeneration has been observed but in a very limited fashion in the auditory and vestibular organs of embryonic and newborn mice and mature adults (Burns et al., 2012; Golub et al., 2012; Bucks et al., 2017). Since the majority of hearing and balance disorders in humans are due to the loss or damage of hair cells, understanding how to stimulate the hair cell regeneration process in the mammalian inner ear represents a direct solution to hearing loss or vestibular problems. The zebrafish is an excellent genetic model to understand hair cell regeneration and inner ear function. Yet, the majority of zebrafish hair cell regeneration and inner ear research to date has focused on the larval lateral line due to its relatively simple structure and accessibility. Lateral line regeneration occurs through support cell proliferation and differentiation. Lateral line hair cells frequently undergo apoptosis, constant turnover, and are renewed by peripheral supporting cell division. Research on the zebrafish lateral line has identified candidate genes, pathways, and cell populations involved in hair cell regeneration at single-cell resolution (Baek et al., 2021). Less is known about the mechanisms underlying hair cell regeneration within the inner ear sensory epithelium of zebrafish. The inner ear sensory epithelium is composed of sensory hair cells organized into “orientation groups” that are surrounded by supporting cells and are innervated by fibers from the eighth cranial nerve (Popper, 2020; Popper and Hawkins, 2021). In the adult inner ear, the sensory epithelium continues to expand for the first 10 months of life and subsequently have a low but measurable turnover of hair cells (Lombarte and Popper, 1994; Higgs et al., 2002).
To uncover detailed mechanisms of hair cell regeneration in animals that possess the capacity to regenerate hearing, frequently used models for experimental hair cell destruction in adult fish include acoustic overstimulation/sound exposure (Smith et al., 2004; Schuck and Smith, 2009; Liang et al., 2012), blast wave exposure (Wang et al., 2019), and aminoglycoside antibiotics (Song et al., 1995; Uribe et al., 2013). However, sound exposure experiments achieved ≤75% hair cell ablation and only in the auditory organs while blast wave exposure elicited more serious hearing loss phenotypes, but also caused brain injury with increased cell apoptosis and decreased neurogenesis. Aminoglycoside administration using a high dose of gentamicin induced only a 15% reduction in sensory hair cell loss across the entire saccule and utricle. Adult zebrafish experiments that use aminoglycosides, laser ablation, or sound exposure to induce hair cell death show that supporting cells repopulate the inner ear within 1–7 days post hair cell death (Schuck and Smith, 2009; Liang et al., 2012; Uribe et al., 2013). Despite similarities between the lateral line and inner ear sensory epithelium, such as the shared type I- and type II-like hair cell types (Song et al., 1995), it is unclear whether the adult inner ear regeneration pathways are distinct in some way from the lateral line.
In order to investigate the mechanism of hair cell regeneration in adult auditory and vestibular organs, we sought to establish a robust new model for hearing loss and regeneration research by generating a transgenic zebrafish with the capacity for targeted and inducible hair cell ablation in vivo. The gene encoding the human diphtheria toxin receptor (hDTR) was placed downstream of the zebrafish myo6b promoter whose expression is limited to differentiated hair cells in zebrafish. Since the orthologous zebrafish receptor has a significantly lower affinity to diphtheria toxin (DT) than the human one, treatment with DT resulted in hair cell specific ablation with minimal systemic side effects. Here we show that a single, low concentration, intraperitoneal injection of DT in the Tg(myo6b:hDTR) transgenic background caused complete loss of hair cells in the adult zebrafish utricle and saccule and that over time the hair cells regenerated. We also show that DT exposure ablated larval lateral line hair cells in a dose-dependent manner. This ablation approach could also be used in other tissues where cell-specific ablation is desirable in zebrafish.
Materials and Methods
Experimental Animals
TAB5 wild-type (WT) zebrafish were used in this study. Fish were randomly selected and represented roughly equal numbers of males and females. All animal experiments were performed under an approved Animal Study Protocol (G-01-3) and animals were handled in compliance with the NHGRI Animal Care and Use Committee’s written guidance and the Shared Zebrafish Facility User Standards and Operating Procedures.
Generation of myo6b-DTR Zebrafish
We targeted expression of the hDTR (heparin-binding epidermal growth factor precursor; proHB-EGF) to zebrafish hair cells by using the hair cell-specific myo6b promoter (Kindt et al., 2012). We generated the Tg(myo6b:hDTR) construct as follows. The full coding region of the hDTR gene (Genscript Clone ID OHu26607D) was PCR amplified with the following 5′ adaptor (attB) sequences to increase the specificity of cloning orientation (Forward primer adds attB1 site 5′-GGGGACAAGTTTGTACAAAAAAGCAGGCTTAACCCAC TGCTTACTGGCTTA-3′ and Reverse Primer adds attB2 site and a stop codon 5′ - GGGGACCACTTTGTACAAGAAAGCTGGG TACTAACTAGAAGGCACAGTCGAGG-3′. A middle entry clone was generated by performing a BP recombination between the attB hDTR PCR product with the pDONR 221 clone. The hDTR-pDONR221 middle entry clone was verified by restriction digest and sequencing. To create a Gateway expression clone, an LR reaction was performed using a 5′ Entry myo6b clone (gift from Dr. Katie Kindt), hDTR middle entry clone, 3′ Entry PolyA clone, and destination vector pDestTol2CG2. The myo6b:hDTR construct was verified by sequencing and injected into TAB5 1-cell stage embryos with mRNA encoding the Tol2 transposase (Kawakami et al., 2000). All experimental zebrafish used in this study were heterozygous, Tg(myo6b:hDTR).
In situ Hybridization Chain Reaction
Three dpf embryos were fixed in a 4% formaldehyde solution and stored overnight in 100% methanol. Embryos were rehydrated with a series of graded 1 mL methanol/PBST washes (75, 50, and 25% methanol) for 5 min and then rinsed five times in PBST (0.1% Tween-20) for 5 min. Embryos were treated with 1 mL of proteinase K (2 mg/mL) for 10 min at room temperature followed by two washes with PBST without incubation. Embryos were then postfixed with a 4% formaldehyde solution for 20 min at room temperature. Following fixation, embryos were washed five times for 5 min with PBST. Embryos were hybridized with in situ hybridization chain reaction (HCR) probes that were purchased commercially (Molecular Instruments, Inc.) and targeted hDTR (HBEGF, NM_001945.3) and myo6b (NM_001004110.1). Detection and amplification was performed in accordance with the HCR v3.0 protocol for whole-mount zebrafish embryos and larvae (Choi et al., 2016). Embryos were mounted in 0.8% low melting agarose and imaged on a Zeiss LSM 880 confocal microscope. Maximum intensity projections of z-stacks were generated in Image J/Fiji software (Schindelin et al., 2012).
RNA Isolation From Adult Inner Ear Tissues
Saccules and utricles were dissected from adult WT and Tg(myo6b:hDTR) zebrafish and homogenized in 0.7 mL TRIzol Reagent (Thermo Fisher Scientific, United States) with a power homogenizer. RNA was isolated from aqueous phase after TRIzol/chloroform extraction and treated with DNase I. RNA was purified using the RNA Clean & Concentrator-5 (Zymo Research) and measured (Nanodrop One).
Quantitative Real-Time PCR Analysis on Adult Inner Ear Tissues
RNA was transcribed into cDNA according to manufacturer’s instructions (SuperScript III RT, Thermo Fisher Scientific, United States). Quantitative Real-Time PCR Analysis (RT-qPCR) was performed in technical replicates using 1:1 cDNA in each reaction and a primer concentration of 0.5 μM. PowerUp SYBR Green Master Mix (Thermo Fisher Scientific Cat, #4344463, United States) and self-designed primers were used (Eurofins, Luxembourg). Primers were designed by using Primer3 followed by a UCSC in silico PCR to search the zebrafish sequence database. hDTR was amplified using the forward primer 5′-GACCCTCCCACTGTATCCAC-3′ and the reverse primer 5′-GCTCCTCCTTGTTTGGTGTG-3′. myo6b was amplified using the forward primer 5′-ATTAAGAGCTATCAGGGACGC-3′ and the reverse primer 5′-GCTCATCTTCAGAACCCTCAT-3′. ef1alpha was used as a housekeeping gene and was amplified using the forward primer 5′-CGACAAGAGAACCATCGAGAAGTT-3′ and the reverse primer 5′-CCAGGCGTACTTGAAGGA-3′.
Larval Zebrafish Diphtheria Toxin Treatments
Diphtheria toxin was purchased from Sigma-Aldrich (D0564). Five dpf transgenic larvae were exposed for 3–12 h in various concentrations of DT dissolved in 1X Holtfreter’s medium, washed and then maintained in 1X Holtfreter’s for up to 3 days. Eight dpf zebrafish were treated with 8 μM YO-PRO-1 dye (Y3603, Molecular Probes, OR, United States) dissolved in 1X Holtfreter’s medium for 1 h at 28.5°C. After washing, fish were lightly anesthetized with 0.01% tricaine and placed in 96-well, glass bottomed plates for observation. Stained neuromasts in the lateral trunk region were visualized and quantified with an inverted Leica stereomicroscope using a 10X objective. For time-course experiments, larvae were returned to 1X Holtfreter’s medium for recovery.
Adult Zebrafish Diphtheria Toxin Administration
Diphtheria toxin was dissolved in 1XPBS. Six to ten month-old WT (TAB5) and transgenic adult zebrafish of mixed sex were injected one time with DT into the abdominal cavity, posterior to the pelvic girdle, using a microsyringe for nanoliter injection with a 35G beveled needle. Concentration ranges from 0.01 to 50 ng per fish were tested. Before intraperitoneal injection, fish were fasted for 24 h and then lightly anesthetized with buffered MS-222. Immediately after injection, fish recovered in fresh system water and maintained off system for up to 14 days. Tank density was limited to 25 adult zebrafish in a 6 L tank. Fish were fed and water changed once daily. Health and water quality inspections were completed twice daily. Injection experiments required approximately 150 adult zebrafish total per genotype.
Histological Methods
Adult zebrafish were euthanized using buffered MS-222. The heads were dissected and fixed in 4% formaldehyde overnight at 4°C. Inner ears were dissected as previously described in Liang and Burgess (2009).
Hair Cell Labeling
Alexa Fluor 488 phalloidin was used to visualize and quantify F-actin in stereocilia of zebrafish. Utricles and saccules were dissected and stained using Alexa Fluor 488 phalloidin as previously described in Liang and Burgess (2009)Liang and Burgess (2012). Proteins were detected in whole-mount utricles and saccules using standard immunofluorescence labeling methods.
Following overnight fixation, inner ear sensory epithelia were rinsed several times in PBTX (PBS plus 0.1% Triton X-100) and blocked for 1 h in BBTX at room temperature (PBS plus 0.5% BSA, 2% NGS, and 0.1% Triton X-100). Inner ear sensory epithelia were incubated overnight at 4°C with primary antibodies. Inner ears were washed three times for 10 min in PBTX and then incubated overnight at 4°C with secondary antibodies in BBTX. After washing three times for 10 min in PBTX, inner ear sensory epithelia were incubated for 45 min at room temperature with Alexa Flour 488 phalloidin in PBS (Thermo Fisher Scientific, #A12379, United States, 1:1,000-dilution). Following three washes in PBS for 10 min, saccules (with or without lagena) and utricles were mounted in Vectashield with DAPI. Primary and secondary antibodies used include the rabbit myosin VI and myosin VIIa antibodies (Proteus Biosciences 25-6790 and 25-6791, 1:300-dilution), rabbit cleaved Caspase-3 (Cell Signaling #9661, 1:300-dilution), Alexa Fluor 568 goat anti-rabbit IgG (1:1,000-dilution).
Cellular Imaging and Analysis
Confocal images were acquired with a Zeiss LSM 880 confocal microscope. Confocal Z stacks of the entire saccule and utricle were projected into a single image to capture all phalloidin positive cells from different planes of focus for hair cell counting. Counts of phalloidin labeled hair cell bundles were obtained from preselected 50 μm × 50 μm digital boxes along the rostral-caudal axis of the saccule or the medial-striola planes of the utricle. Normal hair cells were quantified as hair cell bundles with intact stereocilia using Image J/Fiji software (Schindelin et al., 2012). Cleaved caspase-3 positive cells were counted with Image J/Fiji software from the entire saccule and utricle whole mount.
Results
Generation of myo6b:hDTR Zebrafish
To create a model that would allow for hair cell specific ablation, we utilized the human DTR gene, which has previously been used to effectively ablate hair cells in the mouse utricle (Golub et al., 2012). In order to drive expression of hDTR only in hair cells, we use the zebrafish hair cell specific promoter myo6b which is expressed in auditory, vestibular, and lateral line hair cells in zebrafish (Figure 1A; Obholzer et al., 2008; Matern et al., 2018). The construct was cloned using the Gateway System into a Tol2 transposon vector and was injected with Tol2 transposase mRNA (Kawakami et al., 2000) into zebrafish embryos at the one-cell stage to create a stable transgenic line. Single-copy F1 fish were identified by PCR. hDTR expression was verified by qRT-PCR in adult zebrafish inner ear auditory (saccule) and vestibular (utricle) sensory epithelia of stable transgenic lines carrying a single Tg(myo6b:hDTR) transgene (Figure 1B). Considering hDTR mRNA levels were robustly expressed in adult inner ear sensory epithelia heterozygous for the Tg(myo6b:hDTR) allele, we reasoned that heterozygous expression of the transgene would suffice for the hair cell ablation response to DT. Therefore, all hair cell ablation experiments were performed on animals carrying a single Tg(myo6b:hDTR) transgene in larvae and adult zebrafish. To confirm Tg(myo6b:hDTR) was expressed in hair cells, we performed HCR on WT and Tg(myo6b:hDTR) 3 dpf embryos using a probe targeting the hDTR. As expected, the hDTR fluorescent signal was present in lateral line hair cells and in the anterior macula of Tg(myo6b:hDTR) fish, but absent in WT controls. As a control, we simultaneously hybridized and detected a probe for myo6b in lateral line hair cells and in the anterior macula of the inner ear of Tg(myo6b:hDTR) and WT fish (Figures 1C,D). All hair cells can be seen expressing both genes simultaneously.
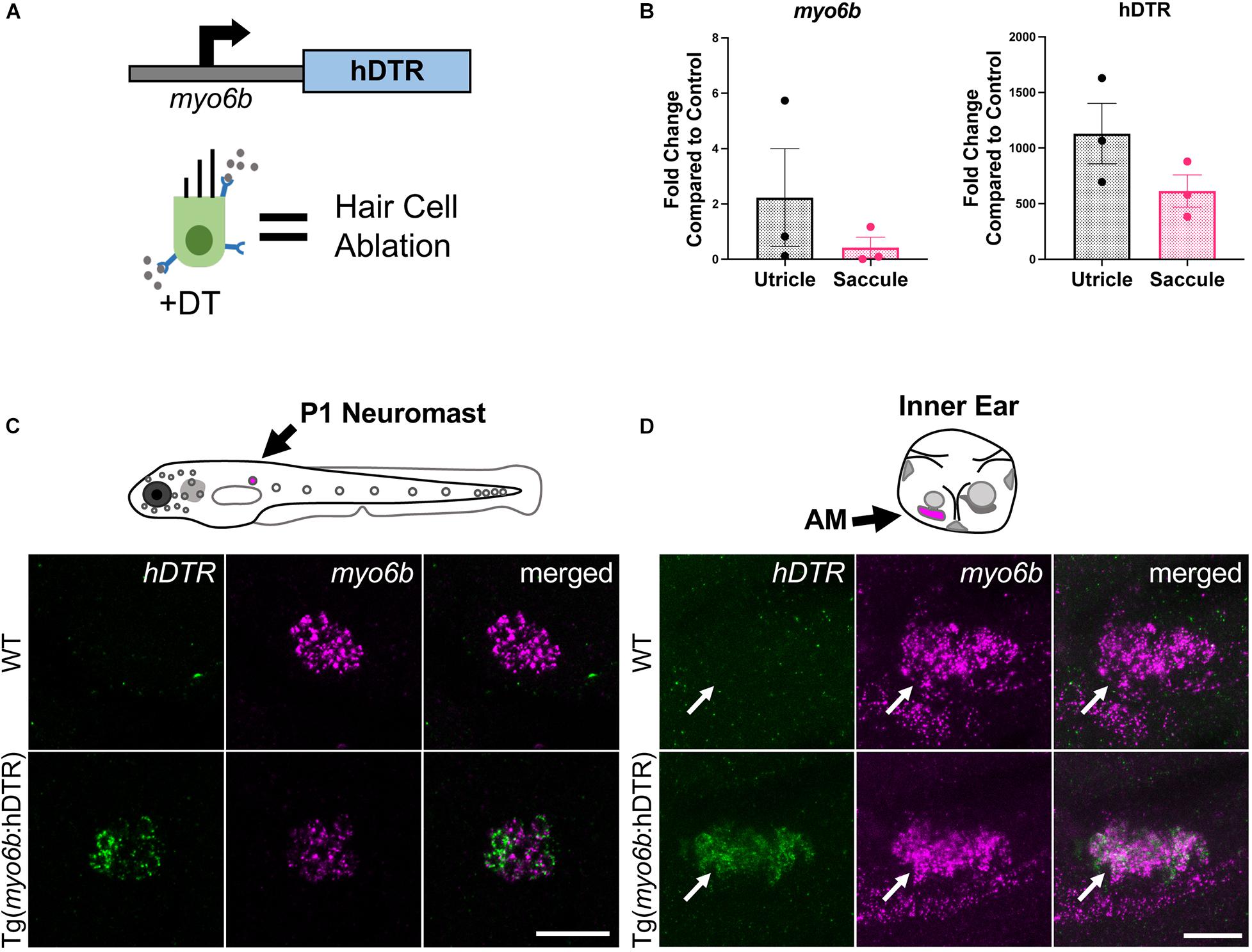
Figure 1. The Tg(myo6b:hDTR) zebrafish. (A) Schematic representation of the human diphtheria toxin receptor (hDTR) construct driven by the hair cell-specific myo6b promoter and representation of the hair cell-specific ablation approach. Diphtheria toxin (DT) is internalized in cells specifically expressing the human version of the DT receptor, triggering cell death. (B) qRT-PCR analysis on untreated Tg(myo6b:hDTR) zebrafish saccule and utricle showing expression of hDTR and myo6b relative to wild-type (WT) animals. The values are represented as the mean ± SEM from three independent samples. (C) Schematic depicts a lateral view of a whole zebrafish larva with neuromasts (gray circles). The P1 neuromast examined for imaging is indicated by magenta fill. In situ HCR using probes targeting hDTR and myo6b in hair cells of a single P1 neuromast of 3 dpf WT and Tg(myo6b:hDTR) embryos is shown. Brightness and contrast adjusted 50 and 25%, respectively. Scale bar 20 μm. (D) Schematic depicts the left ear of a larval zebrafish with anterior macula indicated by magenta fill. Lateral views of inner ears are shown following HCR in situ with probes targeting hDTR and myo6b in 3 dpf WT and Tg(myo6b:hDTR) embryos. Brightness and contrast adjusted 65 and 35%, respectively. Scale bar 20 μm.
Diphtheria Toxin Induced Hair Cell Death and Regeneration in the Larval Lateral Line
Figure 2A shows the experimental regimen for the larval neuromast hair cell ablation studies. To determine whether DT was able to ablate hair cells of the lateral line of zebrafish expressing hDTR in hair cells, we performed a dose-response assay using 5 dpf larvae that were WT or heterozygous for the Tg(myo6b:hDTR) allele. We treated 5 dpf larvae with concentrations of dissolved DT ranging from 0.5 to 1.5 μg/mL and continuous exposure times ranging from 3 to 12 h. After rinsing the larvae three times in fresh medium, the number of YO-PRO-1 labeled cells in each of the four identified neuromasts (P1, P2, P3, and P4) was determined for the different exposure concentrations and timepoints following DT treatment. YO-PRO-1 labeled hair cell loss and recovery was analyzed at 0, 24, 48, and 72 h post-DT treatment. Hair cells were assessed by fluorescence microscopy and counted for approximately eight fish per group.
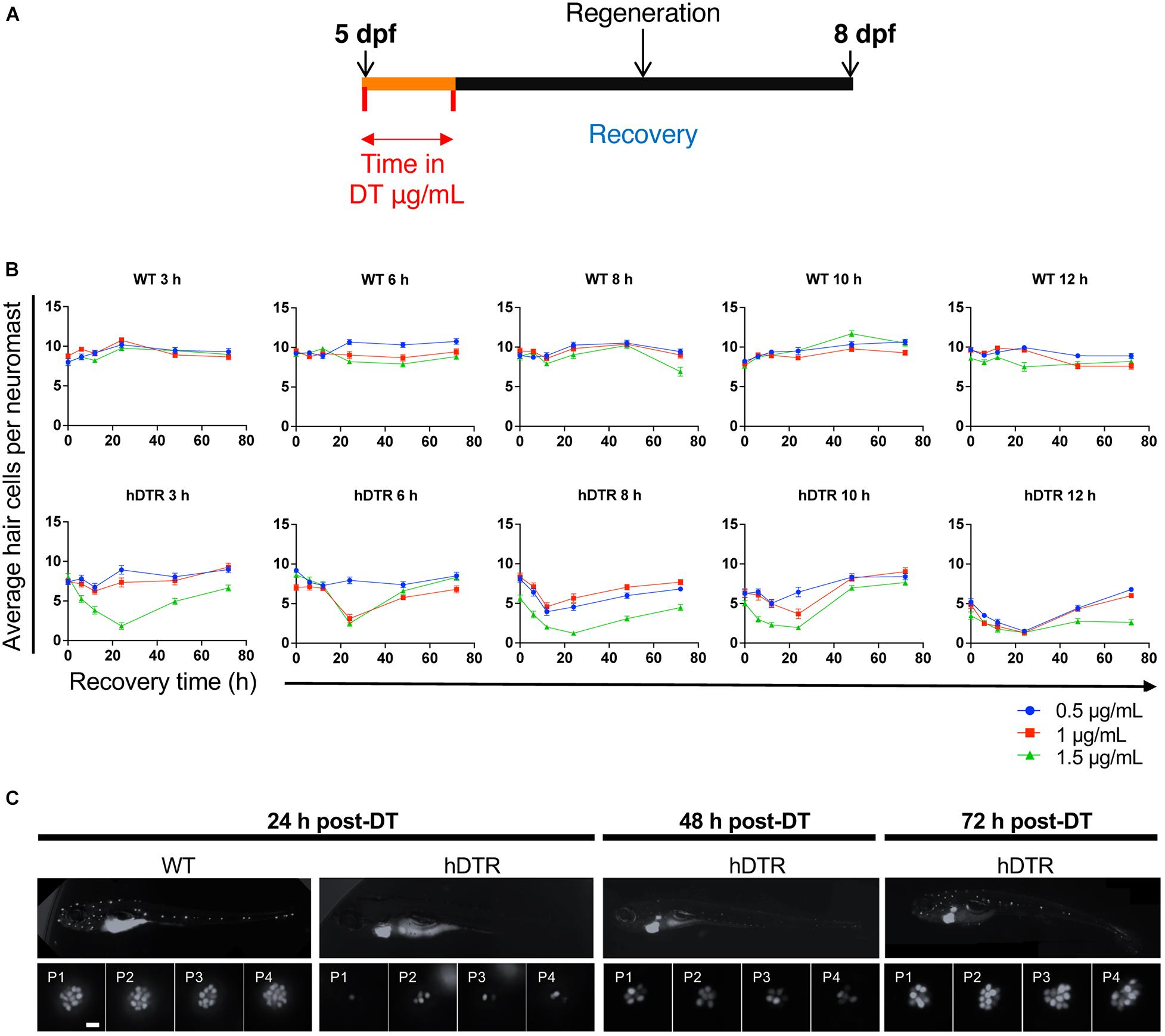
Figure 2. Tg(myo6b:hDTR) larval zebrafish show hair cell loss and regeneration in lateral line neuromasts after in vivo DT treatment. (A) Schematic representation of the larval DT exposure treatment. (B) Wild-type (WT) and Tg(myo6b:hDTR) (hDTR) larvae were exposed to three concentrations of DT (0.5, 1, and 1.5 μg/mL) for various durations (3, 6, 8, 10, and 12 h). Neuromast viability was monitored daily by YO-PRO-1 labeling 0 to 72 h post-incubation. Shown is the quantification of YO-PRO-1 labeled hair cells. The values are represented as the mean ± SEM from eight fish. (C) Regeneration time-course showing P1–P4 over three days of recovery. Larvae were exposed to 1 μg/mL of DT for 6 h. Scale bar 100 μm.
Diphtheria toxin had no effect on YO-PRO-1 staining in WT 5 dpf zebrafish at all concentrations and durations examined (Figure 2B). However, DT reduced the number of YO-PRO-1 labeled neuromast hair cells in 5 dpf Tg(myo6b:hDTR) fish in a dose-dependent manner with no general toxicity or mortality to the larvae at any of the doses or exposure times. At the higher exposure concentrations and longer exposure times, the YO-PRO-1 staining in the neuromasts was absent (Figure 2B). We observe a delayed onset of death and cumulative effect of DT on hair cells which is likely a result of diphtheria toxin’s mechanism of action. The dose and time of exposure influenced the amount of hair cell ablation, but synchronous hair cell loss in Tg(myo6b:hDTR) continued even after withdrawal of DT. Lower doses and longer exposure times were as effective at ablating hair cells as high doses for shorter times. For example, at 3 h incubation with 1.5 μg/mL of DT (the highest concentration of toxin tested on larvae), hair cells are progressively lost until 24 h post treatment, but no significant hair cell loss is observed immediately after treatment at 0 h (Figure 2B). These data show a relationship between DT concentration, exposure time, and hair cell death.
Lateral line hair cells regenerate following acute chemical injury. In Tg(myo6b:hDTR) larvae, hair cell regeneration (defined as recovery in YO-PRO-1 labeling) was evident within 2 days post DT exposure for all concentrations and exposure durations tested (Figure 2B). At 72 h following DT exposure, the mean number of hair cells per neuromast was not significantly different from the unexposed larvae, indicating that recovery was complete at intermediate concentrations (0.5 and 1 μg/mL). Larvae exposed to DT at the highest (1.5 μg/mL) and longest duration (12 h) showed only partial hair cell regeneration by 72 h post-DT.
Figure 2C shows a representative WT zebrafish neuromast 24 h post exposure to 1 μg/mL of DT for 6 h and demonstrates individual hair cells in each of the four neuromasts (P1, P2, P3, and P4) brightly stained with YO-PRO-1. By comparison, Tg(myo6b:hDTR) larvae exposed to 1 μg/mL of DT for 6 h showed a clear reduction in the number of YO-PRO-1 labeled hair cells in all four neuromasts 24 h post exposure. Supplementary Figure 1 shows the progression of cell death from 0 to 24 h post DT treatment (A, B) and the subsequent hair cell regeneration (C).
Diphtheria Toxin Induced Hair Cell Death and Regeneration in the Adult Inner Ear
Using fluorescently-tagged phalloidin to visualize adult inner ear hair cell bundles and myosin VI/VIIa antibodies for hair cell bodies, we assessed auditory (saccule) and vestibular (utricle) hair cells in untreated adult zebrafish that were WT or heterozygous for the Tg(myo6b:hDTR) allele (Supplementary Figure 2). Fluorescent phalloidin, a highly specific F-actin stain, was used to visualize hair cells (Supplementary Figure 2, green channel). Hair cell bodies were labeled with myosin VI/VIIa (Supplementary Figure 2, red channel), which labels the cytoplasm of hair cells to confirm cell death (as opposed to hair cell bundle damage). Figure 3 illustrates the overall appearance of a phalloidin stained utricle and saccule (Figure 3B). The average hair cell densities of the untreated Tg(myo6b:hDTR) utricle and saccule macule are 85 and 161 per 2.5 mm2 area, respectively. Myosin VI/VIIa was present in the cytoplasm of all hair cells examined in untreated WT, DT treated WT, and untreated DTR fish (Supplementary Figure 2, red channel). The pattern of phalloidin and myosin VI/VIIa labeling in the adult inner ear was consistent in treated and untreated WT controls and untreated Tg(myo6b:hDTR) sensory epithelia, although there was some variability in fluorescence intensity that was not quantified (Figure 4 red channel; Supplementary Figure 2; Coffin et al., 2007). The number of hair cells per utricle and saccule did not differ significantly between these two groups and no differences were observed with respect to hair cell appearance. These observations suggested that inner ear hair cell development and maintenance were not affected in adult Tg(myo6b:hDTR) zebrafish.
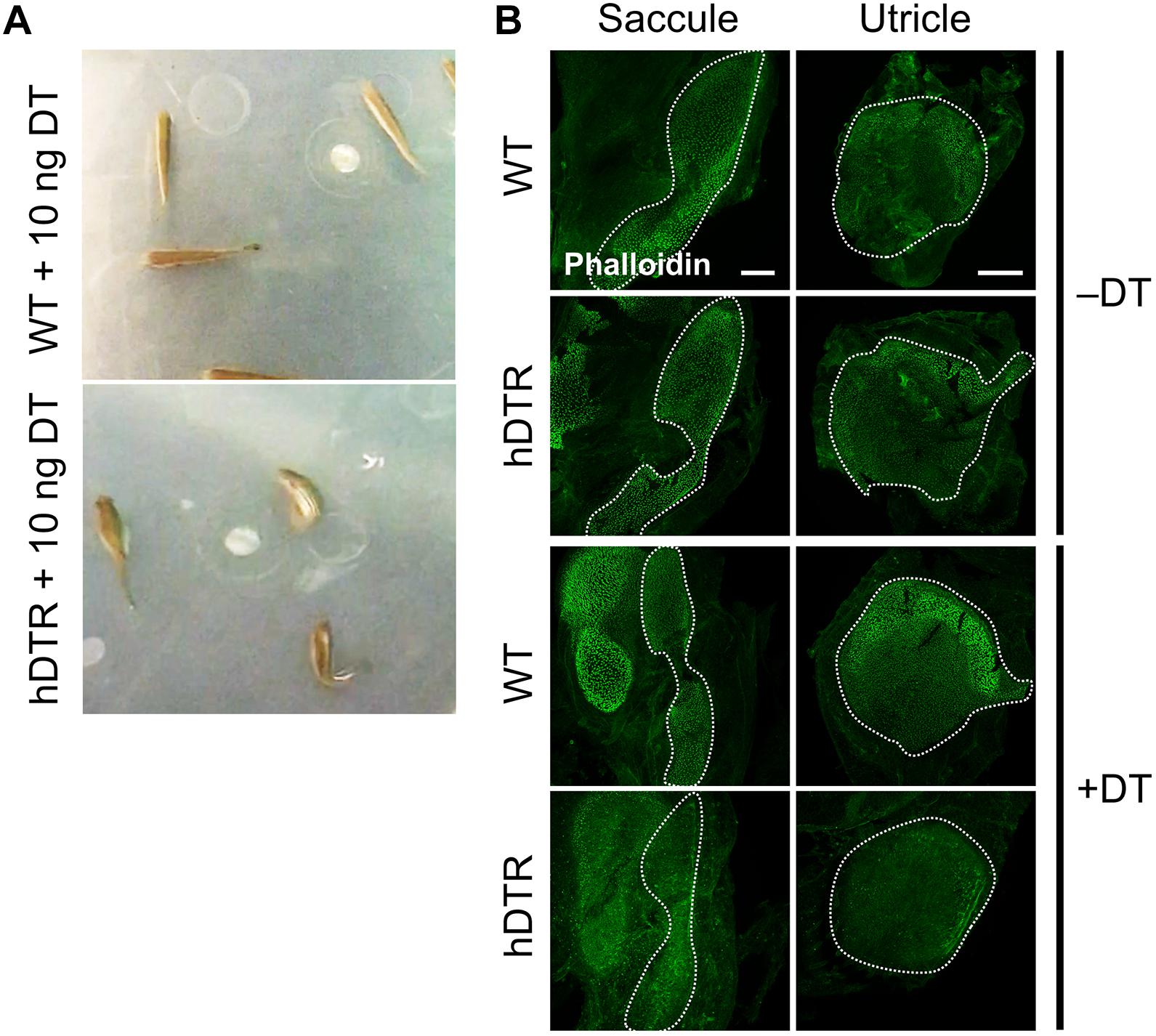
Figure 3. Adult Tg(myo6b:hDTR) injected fish exhibit spatial disorientation and impaired balance. (A) Injected WT fish (WT), (top) and Tg(myo6b:hDTR) (hDTR), (bottom) with 10 ng of DT 3 days-post injection. (B) Saccule and utricle from untreated (–DT) or treated (+DT) WT and Tg(myo6b:hDTR) fish. Saccule and utricle from treated (+DT) WT and Tg(myo6b:hDTR) fish were isolated 3 days post-DT. Scale bar 100 μm.
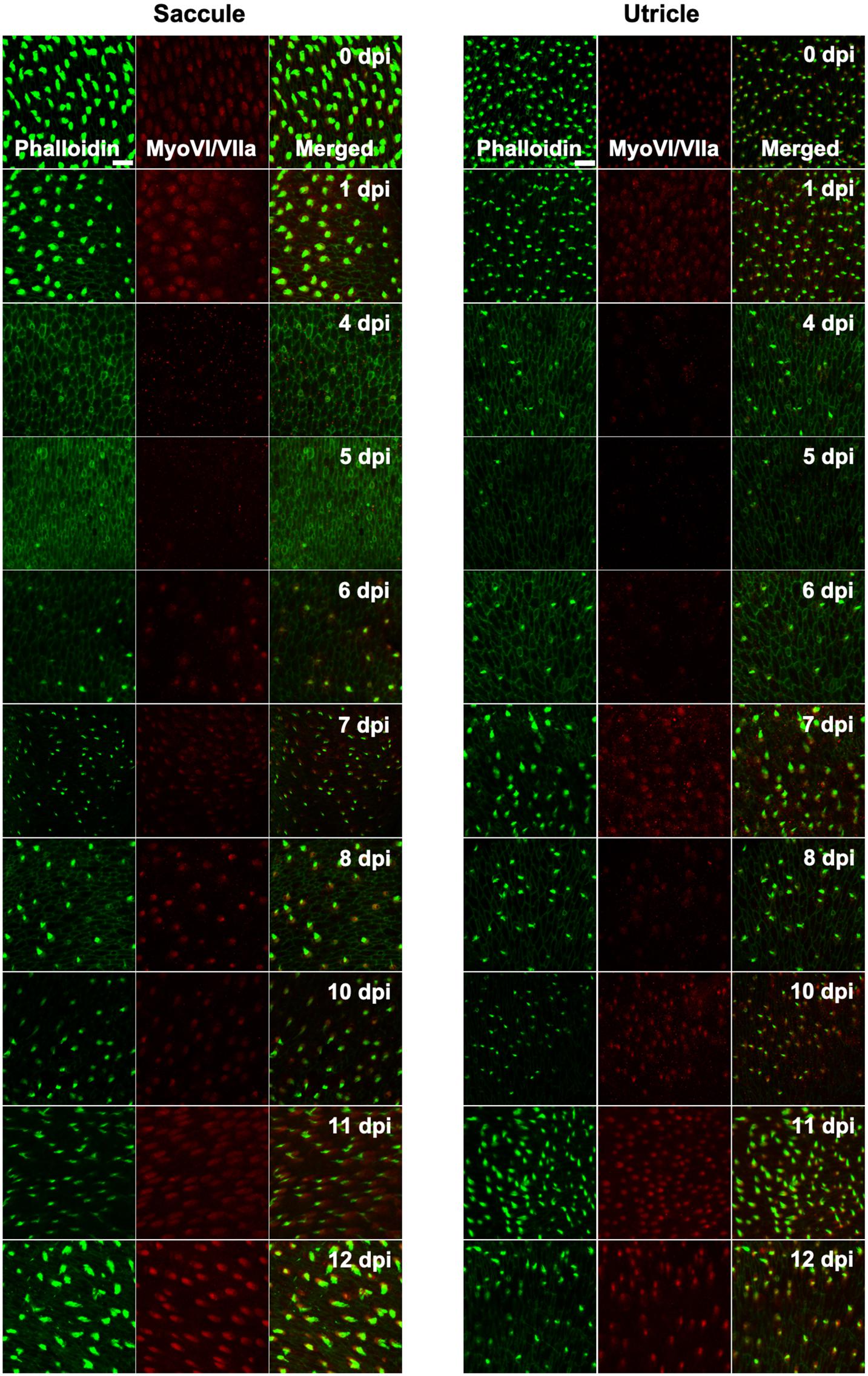
Figure 4. Tg(myo6b:hDTR) adult zebrafish hair cell loss and regeneration in the inner ear after DT treatment. Saccule and utricle were isolated at specified timepoints following DT administration in Tg(myo6b:hDTR) fish. Close examination of hair cells with phalloidin (green channel) and hair cell bodies with anti-myosin VI/VIIa (red channel). Scale bar 100 μm. For qualitative purposes, brightness was increased 10% and contrast by 20% across all images.
In order to determine an appropriate dose of DT to use for downstream ablation experiments in adult zebrafish, we evaluated the effect of various concentrations of injected DT (ranging from 0.01 to 10 ng per fish) on adult fish that were WT or heterozygous for the Tg(myo6b:hDTR) allele. No to minimal defects in swimming behaviors were observed in Tg(myo6b:hDTR) fish injected with 0.01–0.05 ng of DT. At concentrations above 1 ng per fish (such as 10 ng), Tg(myo6b:hDTR) fish exhibited consistent swimming defects that come out most strongly when the water was agitated (Supplementary Video 1). The behavior of injected Tg(myo6b:hDTR) fish with 10 ng of DT consisted of somersaulting and random lateral looping (Figure 3A). Tg(myo6b:hDTR) injected fish mimic the swimming behavior of fish under microgravity conditions (Von Baumgarten et al., 1975), fish that have undergone laceration or removal of the utricle (Pfeiffer, 1964), and the sputnik and orbiter adult circler mutants with mutations affecting vestibular function (Nicolson et al., 1998). The onset of swimming defects correlated to when the hair cells in the inner ear were maximally ablated (Figure 3). Similarly, recovery in swimming behavior correlated to when the hair cells in the inner ear regenerated.
We characterized the time-course of hair cell loss and the minimum dose capable of completely ablating hair cells to help maximize animal viability and reduce excess stress. Similar to our observations in larval zebrafish treated with DT, we observed a delay and cumulative effect of DT on hair cell death in adult zebrafish. The dose of exposure influenced when hair cell loss was first observed and the time it took for hair cells to return. We determined a low DT dose of 0.05 ng per fish was sufficient for nearly complete hair cell ablation throughout the utricle, saccule and even lagena followed by hair cell recovery within a 13-day window, although we were only able to detect very subtle behavioral phenotypes at this concentration, suggesting looping behaviors may require complete loss of hair cell function to be observed.
Adult fish received a single intraperitoneal injection of 1 μL of 0.05 ng DT. Immediately following injection, fish were allowed to recover for up to 14 days in static tanks. Adult inner ears (utricle and saccule) were harvested following timepoints ranging from 0 to 13 days post injection. DT did not affect hair cells in injected WT zebrafish at any time point examined. In contrast, significant hair cell loss was observed between 4 and 5 days after DT treatment in adult Tg(myo6b:hDTR) zebrafish (Figure 4). We confirmed elimination of hair cells (as opposed to hair cell bundle damage) by co-labeling sensory epithelia with phalloidin and myosin VI/VIIa antibodies. Phalloidin staining revealed structural changes that occurred at the epithelial surface 4 and 5 days after DT treatment such as putative lesions and bundle-less cuticular plates in the region of stereociliary loss over the time course of hair cell death. The lack of phalloidin in stereocilia and bundle-less cuticular plates correlated with the absence of myosin VI/VIIa labeling (Figure 4). We conclude that complete hair cell loss is achieved 5 days post-DT treatment in adult Tg(myo6b:hDTR) zebrafish.
Hair cells re-emerged as indicated by the appearance of short phalloidin positive hair cell bundles and myosin VI/VIIa positive cell bodies by day 6 post-DT treatment. On day 7 post-DT, saccules and utricles exhibited an increase in short, immature-like bundles, and an increase in hair cell bundle density. The increase in phalloidin labeled stereocilia corresponded to an increase in myosin VI/VIIa positive cell bodies (Figure 4). The pattern of hair cell bundle density and intensity using phalloidin labeling remained consistent after day 8 in the saccule and utricle, although there was some variability in fluorescence intensity using myosin VI/VIIa labeling. Quantifying the hair cell density of randomly selected regions of the utricle and saccule, we could see that the hair cells return to control levels by 13 days post-DT (Figure 5).
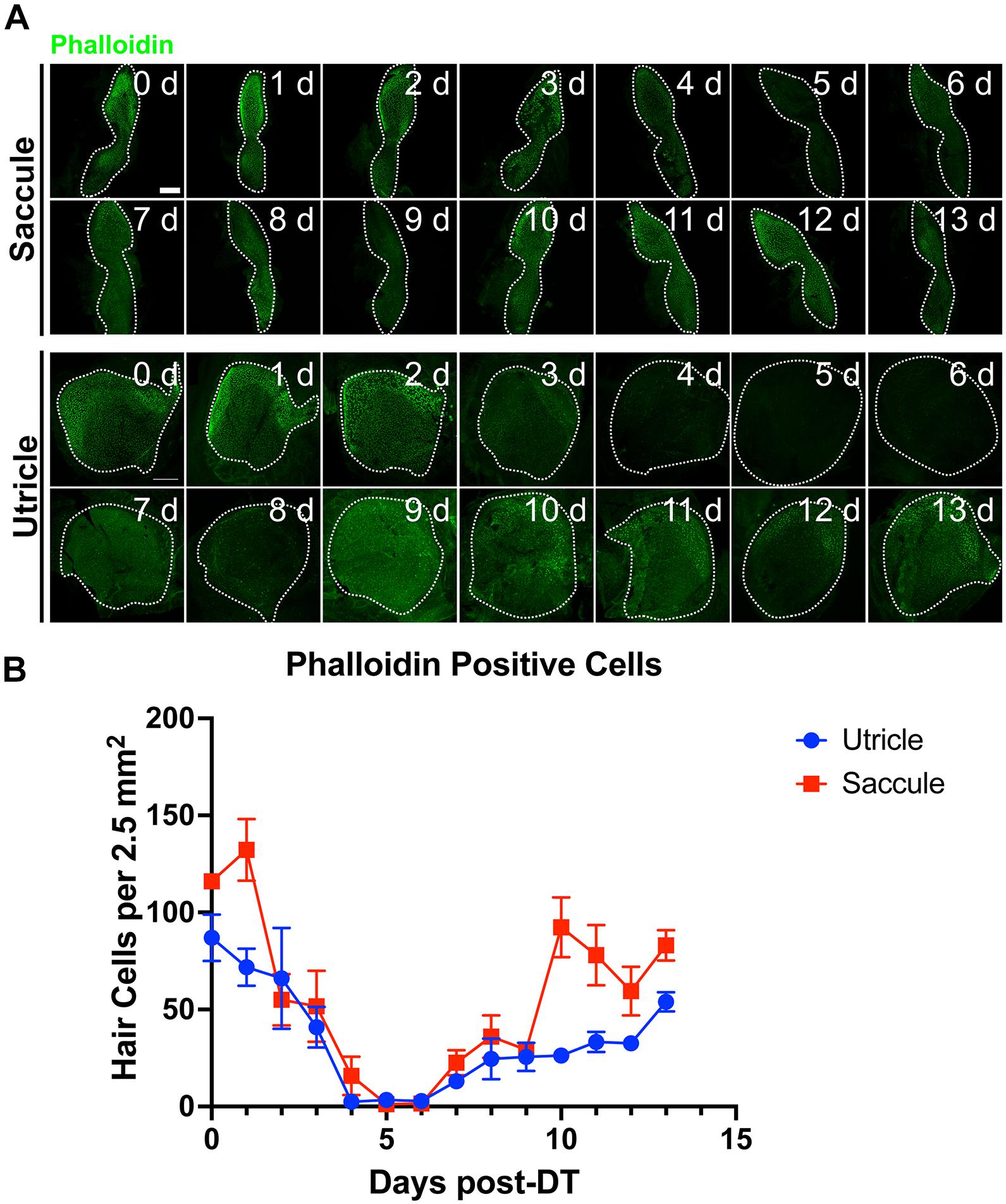
Figure 5. Tg(myo6b:hDTR) adult zebrafish hair cell loss and regeneration in the inner ear after DT treatment. (A) Tg(myo6b:hDTR) saccule and utricle used for quantification were isolated at specified timepoints following DT administration and hair cells were labeled with phalloidin (green channel). Scale bar 100 μm. (B) Quantification of phalloidin positive hair cell number after DT injection. Error bars demonstrate the mean ± SEM.
Hair Cell Apoptosis Is Triggered by DT Treatment in hDTR Transgenic Fish
Apoptosis results in cleavage of caspase-3. Therefore, to test whether hair cells in treated Tg(myo6b:hDTR) fish were undergoing apoptosis, we stained for cleaved caspase-3 on DT treated fish. In the utricle and saccule of DT treated Tg(myo6b:hDTR) fish, we observed cleaved caspase-3-positive cells 1 day post-DT and the number of cleaved caspase-3 positive cells significantly increased by day 2 post-DT (Figure 6). The number of cleaved caspase-3 positive cells declined on day 3 post-DT and each day thereafter examined (Figure 6B). The number of caspase positive cells did appear to be lower than the number of hair cells ablated so we conclude that at least some but maybe not all of the hair cell death is due to apoptosis and cell death is initiated as early as 24 h post DT treatment.
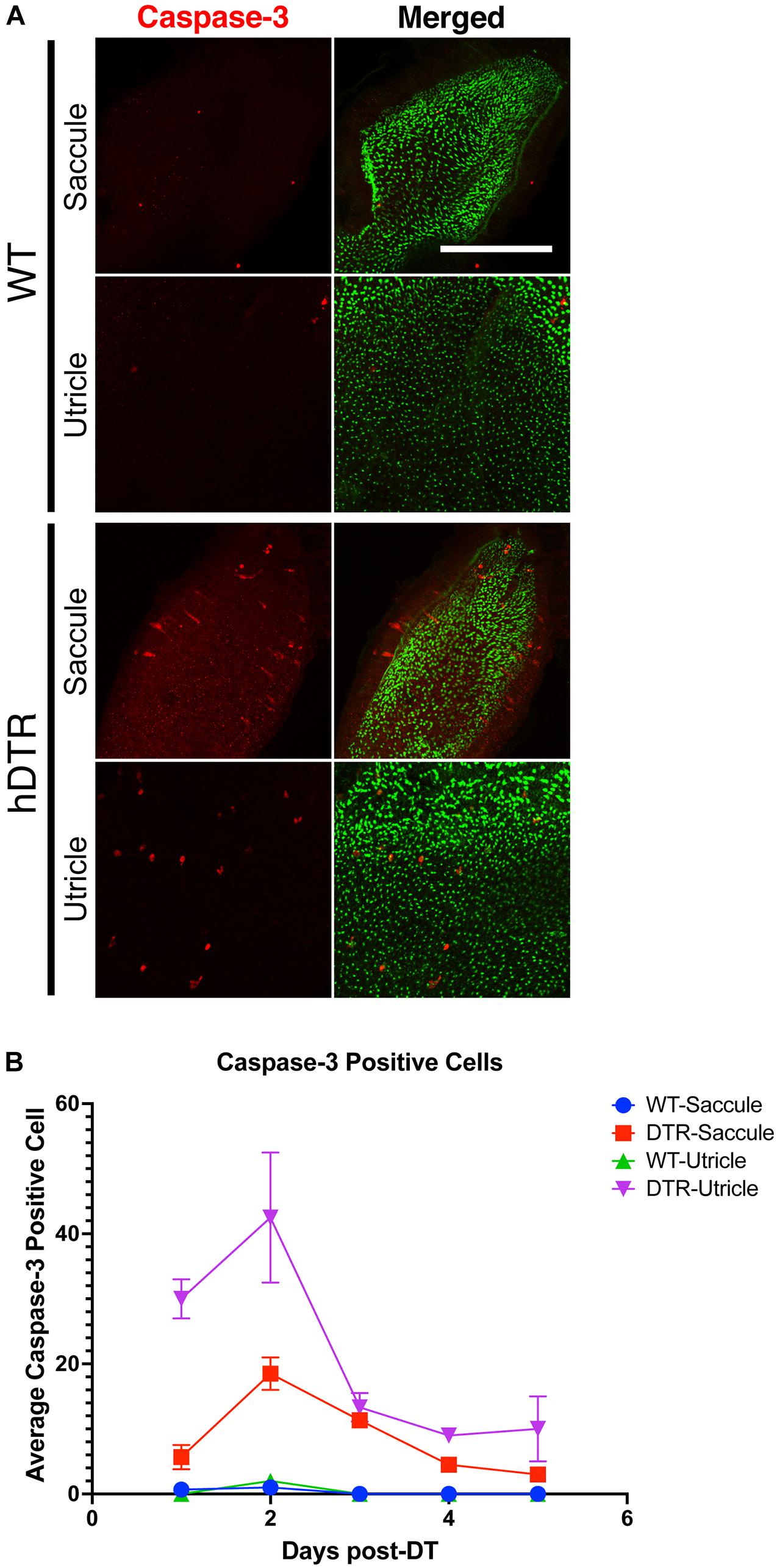
Figure 6. Diphtheria toxin induced hair cell death is primarily due to apoptosis. (A) Saccule and utricle from WT and Tg(myo6b:hDTR) (hDTR) inner ear tissues 2 days post-DT. Hair cells are labeled with phalloidin (green channel) and cleaved caspase-3 (red channel) positive cells were detected 2 days post-DT. (B) Quantification of cleaved caspase-3 positive cells in saccule and utricle dissected on days 1–5 following DT injection. Scale bar 100 μm. For qualitative purposes, brightness and contrast increased by 20%.
Discussion
Hair Cell Regeneration
Efforts have been made to manipulate a small number of factors in the mammalian inner ear to reactivate hair cell regeneration. There has been some limited success inducing hair cell differentiation in a mature mammalian auditory organ by overexpressing the Atoh1 transcription factor an inducer of hair cell fate in all vertebrates (Groves et al., 2013). However, more work is required to identify if there are other factors involved in hair cell regeneration. Our understanding and potentially rescuing hair cell regeneration in mammals requires a comprehensive investigation of how regeneration successfully occurs in other vertebrates, such as the zebrafish. A long term motivation is to investigate the transcriptomic and epigenomic regulation of zebrafish inner ear hair cell regeneration with spatio-temporal resolution. Results from these studies could shed light on the gene regulatory responses that occur as a consequence of hair cell regeneration and provide insight on how supporting cells contribute new hair cells in an adult vertebrate inner ear.
A New Model of Hair Cell Loss
We show that a stable zebrafish line expressing the hDTR in a tissue-specific fashion, can be successfully applied to the analysis of hair cell regeneration in the zebrafish adult inner ear and larval lateral line systems. Environmental exposure of larvae to DT or adult injection of DT was utilized to achieve hair cell specific ablation in zebrafish expressing hDTR under the control of the myo6b promoter. With this technique, we observed extensive, targeted cell death after a delay, which was likely due to the mechanism of action for DT. DT binds to the toxin receptor, it internalizes, and the catalytic A subunit acts as an inhibitor of protein synthesis and arrests RNA synthesis, which ultimately leads to apoptosis or cell death (Collier, 1975). A potential drawback of the system is the extreme potency of the toxin which could cause “off-site injuries” by binding to the lower affinity endogenous receptors. Studies which express diphtheria toxin A fragment (DTA) in zebrafish embryos in the retina (Kurita et al., 2003) or in the germline (Slanchev et al., 2005) resulted in undesired ablation of other cells and even death of the organism due to DT toxicity. However, we find that cell specific expression of the hDTR in hair cells using the myo6b promoter causes no morphological defects in comparison to WT fish. Moreover, DT can be administered at low enough doses to cause cell specific ablation with limited to no toxicity in other cells and no noticeable toxicity to the animal.
Comparison With Other Adult Zebrafish Models for Hair Cell Ablation
At least three additional zebrafish models have been used to ablate hair cells in the adult inner ear. Immediately following cessation of sound exposure (to 100 Hz pure tone at 179 dB re 1 μPa RMS) for 36 h results in a 75% reduction in stereocilia density of the auditory hair cells in saccules. These sound exposure experiments significantly decrease hair cells to 43% in the caudal region and 75% of the total distance from the rostral tip. Specifically, sound exposure produces hair bundle loss only in the caudal region of the saccule and then 2 days later noticeable bundle loss is seen in the central portion of the rostral region (25%) 0 and 2 days post sound exposure (Schuck and Smith, 2009). Similar to our observations using DTR-fish treated with DT, auditory hair cell regeneration was observed 2 days post sound exposure. Aminoglycoside administration using a single intraperitoneal injection of a high dose of gentamicin induced a noticeable but limited reduction in sensory hair cell loss across the entire saccule and utricle, accompanied by shifts in auditory thresholds (Uribe et al., 2013). It was not shown whether hair cells replenished following gentamicin exposure. In contrast to both acoustic and ototoxic exposure, we observed near complete hair cell loss throughout the auditory and vestibular sensory epithelia followed by regeneration of hair cells. The Tg(myo6b:hDTR) zebrafish and DT system represents an ideal method for hair cell ablation in a regeneration study as the method induces synchronous destruction of all hair cells with negligible effects on neighboring inner ear cells. Moreover, this system is mild enough to allow subsequent hair cell regeneration. It was interesting to note that while 0.05 ng DT injections ablated essentially all but a few residual hair cells, we typically did not observe significant circling behavior in those fish. It suggests to us that overt vestibular behaviors are perhaps only observable in the most severe cases of functional hair cell deficiency in zebrafish.
Most hair cell regeneration studies have been implemented using the larval lateral line systems. However, the larval lateral line system and inner ear hair cells are not identical and examination of adult inner ears could have significant differences relevant to potential therapeutic treatments. The Tg(myo6b:hDTR) fish will enable examination of adult behaviors associated with auditory dysfunction and equilibrium orientation defects. DT treated Tg(myo6b:hDTR) adult zebrafish have defects similar to those seen in human hereditary and environmentally induced forms of deafness which may serve as a model for such disorders since zebrafish are accessible to a wide range of analyses. This method could facilitate uncovering roles of specific tissues during development, homeostasis, and aging of the ear. Analysis of the recovery after cell ablation may also reveal novel cellular and molecular mechanisms underlying the regenerative processes, thus bringing insights to the field of regenerative medicine.
Data Availability Statement
The original contributions presented in the study are included in the article/Supplementary Material, further inquiries can be directed to the corresponding author.
Ethics Statement
The animal study was reviewed and approved by the National Human Genome Research Animal Care and Use Committee.
Author Contributions
EJ and SB designed the experiments and wrote the manuscript. EJ, CS, and LC-C performed the experiments. All authors contributed to the article and approved the submitted version.
Funding
This research was supported by the Intramural Research Program of the National Human Genome Research Institute (ZIAHG200386-06).
Conflict of Interest
The authors declare that the research was conducted in the absence of any commercial or financial relationships that could be construed as a potential conflict of interest.
Publisher’s Note
All claims expressed in this article are solely those of the authors and do not necessarily represent those of their affiliated organizations, or those of the publisher, the editors and the reviewers. Any product that may be evaluated in this article, or claim that may be made by its manufacturer, is not guaranteed or endorsed by the publisher.
Acknowledgments
We thank Katie Kindt for generously donating the 5′ entry myo6b clone; Blake Carrington and Raman Sood for assistance with cell injections; Stephen Frederickson and Tannia Clark, and Charles River for zebrafish care; and the members of the Burgess laboratory for helpful discussion.
Supplementary Material
The Supplementary Material for this article can be found online at: https://www.frontiersin.org/articles/10.3389/fncel.2021.721950/full#supplementary-material
References
Baek, S., Tran, N. T. T., Diaz, D. C., Tsai, Y.-Y., Acedo, J. N., Lush, M. E., et al. (2021). High-resolution single cell transcriptome analysis of zebrafish sensory hair cell regeneration. bioRxiv [Preprint]. doi: 10.1101/2021.07.15.452338
Bever, M. M., and Fekete, D. M. (2002). Atlas of the developing inner ear in zebrafish. Dev. Dyn. 223, 536–543. doi: 10.1002/dvdy.10062
Bucks, S. A., Cox, B. C., Vlosich, B. A., Manning, J. P., Nguyen, T. B., and Stone, J. S. (2017). Supporting cells remove and replace sensory receptor hair cells in a balance organ of adult mice. Elife 6:e18128. doi: 10.7554/eLife.18128
Burns, J. C., Cox, B. C., Thiede, B. R., Zuo, J., and Corwin, J. T. (2012). In vivo proliferative regeneration of balance hair cells in newborn mice. J. Neurosci. 32, 6570–6577. doi: 10.1523/JNEUROSCI.6274-11.2012
Choi, H. M., Calvert, C. R., Husain, N., Huss, D., Barsi, J. C., Deverman, B. E., et al. (2016). Mapping a multiplexed zoo of mRNA expression. Development 143, 3632–3637. doi: 10.1242/dev.140137
Coffin, A. B., Dabdoub, A., Kelley, M. W., and Popper, A. N. (2007). Myosin VI and VIIa distribution among inner ear epithelia in diverse fishes. Hear. Res. 224, 15–26. doi: 10.1016/j.heares.2006.11.004
Collier, R. J. (1975). Diphtheria toxin: mode of action and structure. Bacteriol. Rev. 39, 54–85. doi: 10.1128/br.39.1.54-85.1975
Golub, J. S., Tong, L., Ngyuen, T. B., Hume, C. R., Palmiter, R. D., Rubel, E. W., et al. (2012). Hair cell replacement in adult mouse utricles after targeted ablation of hair cells with diphtheria toxin. J. Neurosci. 32, 15093–15105. doi: 10.1523/JNEUROSCI.1709-12.2012
Groves, A. K., Zhang, K. D., and Fekete, D. M. (2013). The genetics of hair cell development and regeneration. Annu. Rev. Neurosci. 8, 361–381. doi: 10.1146/annurev-neuro-062012-170309
Higgs, D. M., Souza, M. J., Wilkins, H. R., Presson, J. C., and Popper, A. N. (2002). Age- and size-related changes in the inner ear and hearing ability of the adult zebrafish (Danio rerio). J. Assoc. Res. Otolaryngol. 3, 174–184. doi: 10.1007/s101620020035
Kawakami, K., Shima, A., and Kawakami, N. (2000). Identification of a functional transposase of the Tol2 element, an Ac-like element from the Japanese medaka fish, and its transposition in the zebrafish germ lineage. Proc. Natl. Acad. Sci. U.S.A. 97, 11403–11408. doi: 10.1073/pnas.97.21.11403
Kindt, K. S., Finch, G., and Nicolson, T. (2012). Kinocilia mediate mechanosensitivity in developing zebrafish hair cells. Dev. Cell 23, 329–341. doi: 10.1016/j.devcel.2012.05.022
Kurita, R., Sagara, H., Aoki, Y., Link, B. A., Arai, K., and Watanabe, S. (2003). Suppression of lens growth by alphaA-crystallin promoter-driven expression of diphtheria toxin results in disruption of retinal cell organization in zebrafish. Dev. Biol. 255, 113–127. doi: 10.1016/s0012-1606(02)00079-9
Liang, J., and Burgess, S. M. (2009). Gross and fine dissection of inner ear sensory epithelia in adult zebrafish (Danio rerio). J. Vis. Exp. 27:1211. doi: 10.3791/1211
Liang, J., Wang, D., Renaud, G., Wolfsberg, T. G., Wilson, A. F., and Burgess, S. M. (2012). The stat3/socs3a pathway is a key regulator of hair cell regeneration in zebrafish. [corrected]. J. Neurosci. 32, 10662–10673. doi: 10.1523/JNEUROSCI.5785-10.2012
Lombarte, A., and Popper, A. N. (1994). Quantitative analyses of postembryonic hair cell addition in the otolithic endorgans of the inner ear of the European hake, Merluccius merluccius (Gadiformes, Teleostei). J. Comp. Neurol. 345, 419–428. doi: 10.1002/cne.903450308
Matern, M. S., Beirl, A., Ogawa, Y., Song, Y., Paladugu, N., Kindt, K. S., et al. (2018). Transcriptomic profiling of zebrafish hair cells using ribotag. Front. Cell Dev. Biol. 6:47. doi: 10.3389/fcell.2018.00047
Nicolson, T. (2005). The genetics of hearing and balance in zebrafish. Annu. Rev. Genet. 39, 9–22. doi: 10.1146/annurev.genet.39.073003.105049
Nicolson, T., Rusch, A., Friedrich, R. W., Granato, M., Ruppersberg, J. P., and Nusslein-Volhard, C. (1998). Genetic analysis of vertebrate sensory hair cell mechanosensation: the zebrafish circler mutants. Neuron 20, 271–283. doi: 10.1016/s0896-6273(00)80455-9
Obholzer, N., Wolfson, S., Trapani, J. G., Mo, W., Nechiporuk, A., Busch-Nentwich, E., et al. (2008). Vesicular glutamate transporter 3 is required for synaptic transmission in zebrafish hair cells. J. Neurosci. 28, 2110–2118. doi: 10.1523/JNEUROSCI.5230-07.2008
Pfeiffer, W. (1964). “Equilibrium orientation in fish1 1in memoriam Prof. Dr. Erich von Hoist,” in International Review of General and Experimental Zoology, Vol. 1, eds W. J. L. Felts and R. J. Harrison (Amsterdam: Elsevier), 77–111. doi: 10.1016/B978-1-4831-9977-1.50008-0
Popper, A. N. (2020). Colleagues as friends. ICES J. Mar. Sci. 77, 2033–2042. doi: 10.1093/icesjms/fsaa097
Popper, A. N., and Fay, R. R. (1993). Sound detection and processing by fish: critical review and major research questions. Brain Behav. Evol. 41, 14–38. doi: 10.1159/000113821
Popper, A. N., and Hawkins, A. D. (2021). “Hearing,” in The Physiology of Fishes, 5th Edn, eds S. Currie and D. H. Evans (Boca Raton, FL: CRC Press), 143–157. doi: 10.1201/9781003036401
Schindelin, J., Arganda-Carreras, I., Frise, E., Kaynig, V., Longair, M., Pietzsch, T., et al. (2012). Fiji: an open-source platform for biological-image analysis. Nat. Methods 9, 676–682. doi: 10.1038/nmeth.2019
Schuck, J. B., and Smith, M. E. (2009). Cell proliferation follows acoustically-induced hair cell bundle loss in the zebrafish saccule. Hear. Res. 253, 67–76. doi: 10.1016/j.heares.2009.03.008
Slanchev, K., Stebler, J., de la Cueva-Mendez, G., and Raz, E. (2005). Development without germ cells: the role of the germ line in zebrafish sex differentiation. Proc. Natl. Acad. Sci. U.S.A. 102, 4074–4079. doi: 10.1073/pnas.0407475102
Smith, M. E., Kane, A. S., and Popper, A. N. (2004). Noise-induced stress response and hearing loss in goldfish (Carassius auratus). J. Exp. Biol. 207(Pt 3), 427–435. doi: 10.1242/jeb.00755
Song, J., Yan, H. Y., and Popper, A. N. (1995). Damage and recovery of hair cells in fish canal (but not superficial) neuromasts after gentamicin exposure. Hear. Res. 91, 63–71. doi: 10.1016/0378-5955(95)00170-0
Uribe, P. M., Sun, H., Wang, K., Asuncion, J. D., Wang, Q., Chen, C. W., et al. (2013). Aminoglycoside-induced hair cell death of inner ear organs causes functional deficits in adult zebrafish (Danio rerio). PLoS One 8:e58755. doi: 10.1371/journal.pone.0058755
Von Baumgarten, R. J., Baldrighi, G., Shillinger, G. L. Jr., Harth, O., and Thuemler, R. (1975). Vestibular function in the space environment. Acta Astronaut. 2, 49–58. doi: 10.1016/0094-5765(75)90042-9
Keywords: cell ablation, hair cell regeneration, zebrafish, diphtheria toxin, inner ear, hearing, hair cell loss
Citation: Jimenez E, Slevin CC, Colón-Cruz L and Burgess SM (2021) Vestibular and Auditory Hair Cell Regeneration Following Targeted Ablation of Hair Cells With Diphtheria Toxin in Zebrafish. Front. Cell. Neurosci. 15:721950. doi: 10.3389/fncel.2021.721950
Received: 07 June 2021; Accepted: 30 July 2021;
Published: 19 August 2021.
Edited by:
Bernd Fritzsch, The University of Iowa, United StatesReviewed by:
Daniela Vallone, Karlsruhe Institute of Technology (KIT), GermanyWalace Gomes-Leal, Federal University of Western Pará, Brazil
Copyright © 2021 Jimenez, Slevin, Colón-Cruz and Burgess. This is an open-access article distributed under the terms of the Creative Commons Attribution License (CC BY). The use, distribution or reproduction in other forums is permitted, provided the original author(s) and the copyright owner(s) are credited and that the original publication in this journal is cited, in accordance with accepted academic practice. No use, distribution or reproduction is permitted which does not comply with these terms.
*Correspondence: Shawn M. Burgess, YnVyZ2Vzc0BtYWlsLm5paC5nb3Y=