- Department of Medical Biotechnology, Dongguk University-Gyeongju, Gyeongju, South Korea
Glioblastoma (GBM) is the most common and malignant form of primary brain tumor with a median survival time of 14–16 months in GBM patients. Surgical treatment with chemotherapy and radiotherapy may help increase survival by removing GBM from the brain. However, complete surgical resection to eliminate GBM is almost impossible due to its high invasiveness. When GBM cells migrate to the brain, they interact with various cells, including astrocytes, neurons, endothelial cells, and the extracellular matrix (ECM). They can also make their cell body shrink to infiltrate into narrow spaces in the brain; thereby, they can invade regions of the brain and escape from surgery. Brain tumor cells create an appropriate microenvironment for migration and invasion by modifying and degrading the ECM. During those processes, the Ca2+ signaling pathway and other signaling cascades mediated by various ion channels contribute mainly to gene expression, motility, and invasion of GBM cells. Furthermore, GBM cells release glutamate, affecting migration via activation of ionotropic glutamate receptors in an autocrine manner. This review focuses on the cellular mechanisms of glioblastoma invasion and motility related to ECM, Ca2+ signaling, and glutamate. Finally, we discuss possible therapeutic interventions to inhibit invasion by GBM cells.
Introduction
Gliomas are a common type of primary central nervous system tumors derived from non-neuronal glial cells and include astrocytomas, oligodendrogliomas, and ependymomas. Gliomas are classified into grades I–IV by the World Health Organization (WHO) based on specific pathological features, treatment strategies, and malignancy state (Louis et al., 2007). The higher the WHO grade, the more aggressive the tumor. Glioblastoma (GBM) is a grade IV glioma and the most aggressive and deadliest of primary brain cancers; it arises from astrocytes. The main symptoms of GBM are advancing neurological deficits, persistent headaches, loss of appetite, double or blurred vision, vomiting, and seizures. Of the patients diagnosed with glioma and analyzed in the United States between 2000 and 2014, 61.5% had glioblastomas (Ostrom et al., 2018). The overall survival rate of GBM patients is 39.7% at 1 year and 5.5% at 5 years (Ostrom et al., 2017). Even with medical treatments such as radiation, temozolomide administration, and surgery, the median survival for patients with diagnosed GBM is still only 12–18 months (Stupp et al., 2005; Wen and Kesari, 2008). The highly infiltrative behavior of GBM cells makes it impossible to completely remove the tumor by surgical intervention, causing treatments to be less effective. Furthermore, the invasive nature of GBM results in the destruction of normal brain structures and functions.
Less than 2% of glioblastoma cells migrate beyond the brain (Beauchesne, 2011; Lun et al., 2011; Hamilton et al., 2014), with most GBM cells infiltrating into healthy brain tissue through the perivascular space around blood vessels and the brain parenchyma space that contains neuron and glial cells (Cuddapah et al., 2014). For glioma cells to penetrate, changes in several key factors are needed: energy metabolism (Horing et al., 2012; Kathagen-Buhmann et al., 2016), ion channels (Thompson and Sontheimer, 2016), neurotransmitters, proteases (Demuth and Berens, 2004), cytoskeleton, cell adhesion, and remodeling of the extracellular matrix (ECM) (Cuddapah et al., 2014). The brain ECM is critically involved in various cellular processes, including migration and invasion of glioma cells associated with altering microenvironmental composition (Giese and Westphal, 1996; Charles et al., 2012). Furthermore, intracellular Ca2+ signaling through inositol 1,4,5- triphosphate receptors (IP3Rs), store-operated channels (SOCs), transient receptor potential (TRP) channels, voltage-gated Ca2+ channels (VGCCs), P2 × 7 receptors, and ionotropic glutamate receptors contribute to the motility of glioma cells. This review describes a cellular invasion mechanism of glioblastoma cells that is associated with the ECM and Ca2+ signaling.
The Interaction Between the Extracellular Matrix and Binding Proteins for Migration
The brain ECM forms a physical barrier but actively interacts with the environment through the signaling behavior of a variety of ligands (Miyata and Kitagawa, 2017). Cell migration requires a coordinated process including adhesion of the cell and attachment to and detachment from the ECM (Ridley et al., 2003). The ECM is a highly organized structural network providing structural support and allowing cellular growth, survival, maturation, differentiation, and migration (Theocharis et al., 2016). Glioblastoma cells continuously interact with the ECM, experiencing remodeling for migration and infiltration. Several of the ECM molecules involved in migration are proteoglycans and their binding partners, including hyaluronan, tenascins, glycoproteins, galectins, laminin, and fibrous proteins.
Proteoglycans are heavily glycosylated proteins consisting of core proteins covalently linked to glycosaminoglycan (GAG). Proteoglycans such as heparin sulfate proteoglycan (HSPG) and chondroitin sulfate proteoglycan (CSPG) regulate cellular movement through a variety of signaling pathways. It has been reported that proteoglycan mRNA expression in human GBM is altered compared to that in normal human brain tissue. A subset of GBM highly express CD44, PTPRZ1, and CSPG4/NG2 of the membrane-associated proteoglycans (Wade et al., 2013). CD44 is up-regulated in a GBM dependent on variant form six of the CD44/AKT signaling pathway (Jijiwa et al., 2011). Overexpression of NG2 produces properties similar to those of GBM patients, and knockdown of NG2 using shRNA reduces tumor growth and angiogenesis (Wang et al., 2011). Blocking of PTPRZ1 expression suppresses GBM growth in vivo (Ulbricht et al., 2006). One of the most up-regulated ECM constituents is Versican (VCAN), a member of a family of large aggregating CSPGs. The expression level of the VCAN isoform is altered in brain tumor and has been associated with metastasis of gliomas (Paulus et al., 1996). VCAN mediates glioma migration via TGF-β2 signaling, which induces the malignant property of brain tumors (Arslan et al., 2007). Also, VCAN was substantially up-regulated in a cerebral cortex lesion (Asher et al., 2002). Brevican, a major proteoglycan in the adult human brain, is overexpressed in glioma cells and correlates with late-stage tumor metastasis (Dwyer et al., 2014). Brevican promotes glioma cell motility after proteolytic cleavage by ADAMTS4 and the up-regulation of integrin (Held-Feindt et al., 2006; Hu et al., 2008; Lu et al., 2012).
Hyaluronan (HA) is the principal GAG of brain ECM and contains glucuronic acid and N-acetyl glucosamine (Park et al., 2008; Amorim et al., 2021). Hyaluronan concentration is high in invasive cancer cells and mediates tumor cell proliferation, migration, and invasion by interacting with CD44 and Receptor for HA-Mediated Motility (RHAMM) receptors (Lokeshwar et al., 1997; Toole, 2004; Lokeshwar et al., 2014). Hyaluronan also facilitates invasion of human glioma cells and the secretion of matrix metalloproteinase (MMP) and plasminogen activator (PA) while not affecting proliferation (Nakagawa et al., 1996). MMP is responsible for invasion and progression through the degradation of ECM components, and MMP-2, MMP-9, MT-MMP levels are significantly increased in human gliomas (Chintala et al., 1999). One of the HA receptors, CD44, binds HA in the extracellular space and cytoskeletal components in the intracellular area, acting as a cell membrane glycoprotein and is involved in numerous cellular processes (Tsukita et al., 1994; Misra et al., 2015; Naor, 2016). CD44 expression is increased in GBM, and it elevates the invasion and proliferation of GBM (Kuppner et al., 1992; Breyer et al., 2000; Anido et al., 2010). CD44-specific antisense oligonucleotide, which prevents CD44 expression, substantially inhibits invasion of glioma cells (Merzak et al., 1994). Inhibition of the interaction between HA and CD44 reduces glioblastoma invasion in hydrogels lacking matrix-bound HA, suggesting of production of HA in GBM cells (Chen et al., 2017). Hyaluronan is synthesized in extracellular region and degraded into different size. Glioblastoma invasiveness is directly affected by HA polymer molecular weight within a methacrylamide-functionalized gelatin (GelMA) hydrogel (Chen et al., 2018). Consistent with these results, the CD44 expression level is higher in severe grade glioma cells, and the anti-CD44 antibody effectively reduces the migration of GBM (Yoshida et al., 2012). Epidermal growth factor stimulation promotes CD44 mRNA expression, which results in glioma cell invasion (Monaghan et al., 2000). The other HA-binding protein is Receptor for HA-Mediated Motility (RHAMM), which has increased expression in higher grade glioma cells, and RHAMM soluble peptide suppresses cell proliferation (Akiyama et al., 2001). Overall, HA interactions with CD44 and RHAMM are critical for tumorigenesis and brain tumor invasion.
Tenascins are large multimeric glycoproteins that are differentially expressed in adults, during embryonic development, and in normal and cancer cells (Latijnhouwers et al., 2000; Orend and Chiquet-Ehrismann, 2006). They are thought to have important roles in the migration and invasion of glioma. Tenascin-C (TN-C) near blood vessels is more highly concentrated in glioblastomas than in low-grade astrocytic tumors (Kim et al., 2000) and is expressed in human glioma in vivo (Brosicke et al., 2013). Overexpression of interleukin-33, induced by inflammation, increases invasion of GBM and is associated with elevated TN-C expression via the PI3K and NF-κB signaling pathways (Zhang J. F. et al., 2019). Moreover, TN-C triggers glioma invasiveness through MMP-12 (Sarkar et al., 2006). Additionally, TNIIIA2, a synthetic TN-C peptide, positively regulates the adhesion and migration of cells interacting with integrin (Saito et al., 2007). In the tumor microenvironment, TN-C increases glioblastoma invasion and negatively regulates proliferation (Xia et al., 2016). Tenascin-C also promotes invasion of brain tumor-initiating cells, which is regulated by metalloproteinase ADAM-9 (Sarkar et al., 2015). Consistently, shRNA designed for targeting TN-C impairs glioma cell motility in wound-scratch assay (Angel et al., 2020). It has been suggested that Tenascin-R (TN-R) and Tenascin-W (TN-W), as members of the tenascin family, may be associated with progression and malignancy of glioma cells; however, that hypothesis remains to be elucidated.
Integrins, binding proteins with functions in the infiltration of glioma, stimulate cell adhesion and interact with the ECM as transmembrane heterodimeric receptors composed of α and β chains. In particular, specific antibodies against the αv and β1 integrins (Friedlander et al., 1996) and the treatment of integrin inhibitors (Ishida et al., 2014) suppress glioma cell migration. Inconsistently, it has been suggested that blocking the αv integrin subunit could enhance invasion of glioma in non-migratory glioma (Treasurywala and Berens, 1998). To diagnosis GBM, integrin αvβ3 can be targeted by using hydrocyanines to detect the presence of reactive oxygen species (Zhang L. et al., 2019). Moreover, α3β1 integrins, specific receptors of laminin-5, are highly expressed and have a critical role in the motility and invasion of glioma cells (Fukushima et al., 1998; Zhou et al., 2015). It has also been reported that α3 integrins are critical for the invasiveness of glioma stem-like cells and act through the ERK1/2 pathway (Nakada et al., 2013). Furthermore, signaling mediated by integrins can modulate the activities of MMP and PA to degrade the ECM and allow glioma to invade. Taken together, through their interactions with the ECM, integrins are critically involved in glioma pathogenesis, migration, and infiltration (Figure 1A).
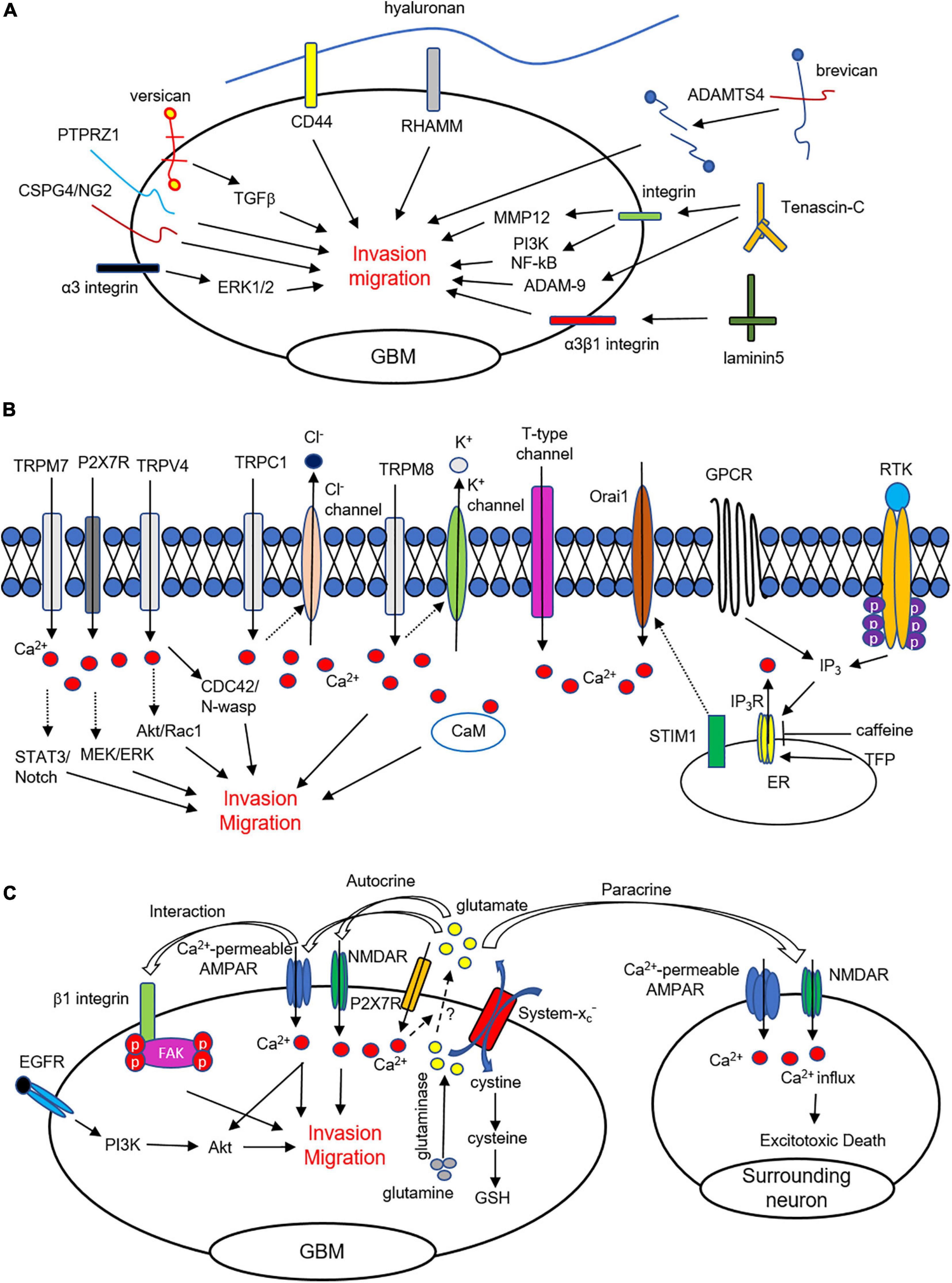
Figure 1. ECM, Ca2+ signaling, and glutamate mediates invasion and migration in glioma cells. (A) Extracellular matrix and their binding partners regulate invasion and motility in GBM cells. (B) Intracellular Ca2+ elevation from ER and extracellular region affects glioma cell migration and invasion. Ca2+ influx through TRP channels, P2 × 7Rs, and t-type Ca2+ channels are critically involved in glioma cell infiltration. (C) Glioma cells release glutamate through a cysteine-glutamate exchanger (system xC–). Released glutamate from glioma cells activates Ca2+-permeable AMPARs and NMDARs and affects migration and invasion. Sustained Ca2+ influx causes excitotoxic death of surrounding cells to make microenvironment for invasion.
Glioma cells infiltrate the brain area using cell-ECM interactions and the associated dynamics. Proteoglycans, hyaluronan, and tenascins have major roles in the brain ECM during invasion, and the associated binding partners, including CD44, RHAMM, and integrins, also have critical roles. Furthermore, ECM molecules including laminins, reelin, heparin-binding growth-associated molecule, tenascin-R, tenascin-C, and CSPG have been suggested in synaptic plasticity and neuronal activity (Dityatev and Schachner, 2003). Interaction of various ECM components with cell surface recognition molecules, receptors, and ion channels affects synaptic plasticity by regulating Ca2 + influx, signaling in endocytic zones, small GTPases, and ECM remodeling (Dityatev and Schachner, 2003). In C6 glioma cell implanted rats, synaptic plasticity is impaired and neuronal activity is abnormally changed (Wang et al., 2011). Probably, abnormal interaction between ECM and its binding partners could cause long-term memory deficit, one of the symptoms in GBM patients, which could be further tested.
Calcium Signaling in Glioblastoma Is Related to Migration and Invasion
An accumulation of evidence demonstrates that Ca2+ is critical for tumorigenesis in GBM and is related to proliferation, motility, and invasiveness. GBM cells express various receptor tyrosine kinase (RTK) and G protein-coupled receptors (GPCRs) that contribute to Ca2+ release from the endoplasmic reticulum (ER). Various agonists for RTK and GPCR increase Ca2+ release from the ER via the activation of inositol 1,4,5-trisphosphate receptors (IP3Rs) in GBM cells (Kang et al., 2010). Blocking of IP3R-mediated Ca2+ release by caffeine was shown to inhibit GBM invasion and extend survival in a skin xenograft model injected with GBM cells (Kang et al., 2010). On the other hand, trifluoperazine (TFP) inhibits proliferation, motility, and invasion by largely increasing IP3R-mediated Ca2+ from the ER in GBM cells (Kang et al., 2017). These results suggest that abnormal responses the either increase or decrease Ca2+ release can suppress glioma cell migration and invasion. Overall, appropriate Ca2+ dynamics mediated by IP3Rs are responsible for the inhibition of metastasis of GBM cells (Figure 1B).
In addition, Ca2+ entry from the extracellular region is associated with a cytosolic Ca2+ increase, which leads to diverse intracellular signaling and motility in glioma. Store-operated channels (SOCs), Ca2+-permeable transient receptor potential (TRP) channels, voltage-gated Ca2+ channels (VGCCs), and P2 × 7 receptors mainly contribute to Ca2+ influx in glioma cells. SOCs are activated by depleting Ca2+ from the ER, sensed by STIM1, and SOC inhibition reduces proliferation and increases apoptosis of GBM cells (Liu et al., 2011). Furthermore, the expression levels of STIM1 and Orai1 are significantly higher in GBM cells than in primary astrocytes, and silencing of STIM1 and Orai1 decreases GBM invasion (Motiani et al., 2013). Besides, the expression of Orai2 is elevated in high-grade glioma, which is an indication of poor survival in GBM patients (Yuan et al., 2019). Consistently, suppression of Ca2+ entry by SOCs inhibits glioma cell motility through the actions of proline-rich tyrosine kinase 2 (Pyk2) (Zhu et al., 2014; Zhu et al., 2020), suggesting that Ca2+ entry is significantly associated with the metastatic characteristics of GBM cells (Figure 1B).
Previously, it has been reported that TRP channels including TRPC1, TRPC6, TRPM2, TRPM3, TRPM7, TRPM8, TRPV1, and TRPV2 are overexpressed in GBM patients, implying the contribution of TRP channels in the progression of GBM (Alptekin et al., 2015). TRP-canonical1 (TRPC1) is associated with cell proliferation, tumor size, and regulation of Cl– channels during changes to the volume of glioma cells for migration (Bomben and Sontheimer, 2010; Cuddapah et al., 2013). TRPC6, another TRPC family member, regulates hypoxylation and stability of hypoxia-inducible factor-1 (HIF-1) in human glioma cells under hypoxic conditions (Li et al., 2015). In human glioma cells, attenuation of TRPC6 activity inhibits cell growth and arrests the cell cycle (Ding et al., 2010). Additionally, TRPC4 activation results in a large Ca2+ influx and enhancement of cell migration in medulloblastoma, a brain cancer arising from cerebellar precursor cells (Wei et al., 2017).
Another family of TRP channels is also directly associated with glioma progression, including migration and invasion of GBM cells. TRP-melastatin7 (TRPM7) promotes migration and invasion of glioma via activation of STAT3 and Notch signaling pathways (Liu et al., 2014), while glioma invasion was reduced in cells transfected with a TRPM7 mutant (Wan et al., 2019). Pharmacological inhibition or siRNA for TRPM7 also reduces migration and invasion in human glioma cells (Leng et al., 2015) and antagonist for TRPM7 reduces various cellular functions such as proliferation, viability, migration, and invasion in both U251/U87 cells (Wong et al., 2020). Furthermore, a TRPM8 agonist increases cytosolic Ca2+, subsequently leading to activation of Ca2+-activated K+ channels that induce glioma cell migration (Wondergem and Bartley, 2009). Consistently, it has been suggested that blocking of TRPM8 can reduce migration and survival in GBM cells (Klumpp et al., 2017). The expression level of TRPM8 is markedly correlated with the invasiveness of glioma cells (Zeng et al., 2019). TRP-vanilloid 4 (TRPV4), another TRP channel subfamily, is abnormally up-regulated in glioma, promoting migration and invasion through AKT/Rac1 signaling (Ou-Yang et al., 2018). Stimulation of TRPV4 increases migration and invasion through the Cdc42/N-wasp axis by regulating cellular protrusions (Yang et al., 2020). Study of various proteins interacting with TRPV2 in GBM may provide possible biomarkers for GBM diagnosis and lead to novel therapeutics (Donate-Macian et al., 2018). Overall, Ca2+ entry through TRP channels is associated with cell survival, proliferation, migration, and invasion of brain tumor cells (Figure 1B).
Voltage-gated Ca2+ channels (VGCCs) are classified as having high voltage-activated (P/Q-, N-, L-type), intermediate voltage-activated (R-type), or low voltage-activated (T-type) channels. Blocking of T-type VGCCs by endostatin reduces cell proliferation and migration in human GBM cells (Zhang et al., 2012). Another pharmacological blockade using mibefradil and siRNA-mediated knockdown of T-type VGCC reduces cell survival and induces apoptosis through the mTOR/Akt pathway in GBM (Valerie et al., 2013) and GBM stem-like cells (Zhang et al., 2017). In another study, decreased expression of T-type VGCC reduced the proliferation of glioma cells (Panner et al., 2005). More recently, it was shown that mibefradil treatment and silencing of T-type VGCC by shRNA reduces GBM cell survival by apoptosis (Visa et al., 2019). Blocking of P/Q- and N-type VGCC affects glioma progression by inhibiting proliferation and viability in glioma cell lines and increasing astrocytes and microglia in the near tumor region in vivo and in vitro (Nicoletti et al., 2017) (Figure 1B).
P2 × 7 receptor (P2 × 7R) is associated with ligand-gated cation channels activated by extracellular ATP binding and leading to intracellular Ca2+ mobilization. Ca2+ signaling mediated by P2 × 7R is related to the malignant characteristics of glioma cells. P2 × 7R is functionally expressed in rat C6 glioma cells, and its activation increases pro-inflammatory factors and cell mobility (Wei et al., 2008). In brains injected with glioma cells, immunostaining of P2 × 7R shows co-localization with tumor cells and microglia, and in a scratch-wound assay, C6 glioma cell migration elevated by P2 × 7R activation is completely blocked by an antagonist for the receptor (Ryu et al., 2011). Consistent with those results, other groups have reported that an agonist for P2 × 7R increases glioma cell migration and proliferation, which is mediated by the MEK/ERK signaling pathway (Ji et al., 2018). Furthermore, activation of P2 × 7R enhances cell death in radiosensitive M059J human glioma cells (Gehring et al., 2012) and GL261 mouse glioma (Tamajusuku et al., 2010). Intriguingly, suppressing P2 × 7R with an antagonist or shRNA promotes cell growth through the up-regulation of EGFR, p-EGFR, HIF-1α, and VEGF in glioma cells (Fang et al., 2013).
Intracellular Ca2+ increase via various ion channels regulates motility and invasion through Ca2+-activated K+ channels upregulated in malignant glioma (Turner et al., 2014). TRAM-34, a specific inhibitor for Ca2+-activated K+ channels, reduces tumor infiltration of GBM cells implanted in the brain tissue of mice (Ruggieri et al., 2012). Furthermore, calmodulin (CaM) as a regulator of intracellular Ca2+ signaling affects GBM invasion by invadopodia formation (Li et al., 2018).
Most of the research in the field of Ca2+ signaling of brain tumor cells related to invasion and migration has been conducted only in the past few years, suggesting that there are still many questions to be solved. Ca2+ signaling, remodeling of Ca2+ signaling, and Ca2+-transporting proteins of GBM cells could be all considered in future investigation.
Glutamate Signaling for Invasion in Glioma Cells
Glutamate, a major excitatory neurotransmitter in the nervous system, has significant roles in the proliferation, growth, and movement of brain tumor cells. Glioma cells have been shown to produce a large amount of glutamate by glutaminase, an enzyme that converts glutamate from glutamine (Yao et al., 2014), and result in a P2 × 7Rs-mediated intracellular Ca2+ increase (Strong et al., 2018). Glutamate is released from glioma cells through system xC–, a cysteine-glutamate exchanger, whose expression level is strongly correlated with brain tumor metastasis (de Groot and Sontheimer, 2011; Takeuchi et al., 2013). Rather than glutamate uptake, glioma cells release large amounts of glutamate, subsequently elevating the extracellular glutamate concentration, leading to excitotoxic death in surrounding neurons; thereby generating a space for cell motility (Ye and Sontheimer, 1999; Chung et al., 2005; Sontheimer, 2008; Noch and Khalili, 2009). Furthermore, inhibition of glutamate release using sulfasalazine, a system xC– blocker, reduces glioma invasion and tumor growth in vivo (Lyons et al., 2007).
Glutamate released from glioma cells activates the Ca2+-permeable AMPA receptors (AMPARs) expressed in the same cell or neighboring cells, and such releases can induce Ca2+ oscillations that are important for cell movement (Lyons et al., 2007). AMPA receptors control glioma cell’s motility through Akt activation and Ca2+ signaling, and activation of the Glutamate-AMPAR-Akt pathway contributes to the invasive growth characteristic of glioma (Ishiuchi et al., 2007). Another study has shown that interactions between AMPARs and β1 integrin induce focal adhesion kinase (FAK) autophosphorylation and Rac activation, thereby facilitating glioma migration and invasion (Piao et al., 2009). In addition, blocking of Ca2+-permeable AMPARs inhibits migration, while overexpression of Ca2+-permeable AMPARs promotes an increase in the number of migratory cells (Ishiuchi et al., 2002). Propofol, a widely used anesthetic, inhibits invasiveness by increasing surface expression of GluA2-containing AMPARs and downregulating system xC– expression (Wang et al., 2017). Some researchers have suggested that NMDARs are not functionally expressed in glioblastoma cells (Lyons et al., 2007; Stepulak et al., 2009). However, several studies recently demonstrated that stimulation of NMDARs enhances invasion, whereas MK-801 treatment, an activity-dependent antagonist for NMDARs, reduces invasion in human GBM cells (Muller-Langle et al., 2019; Nandakumar et al., 2019). Kynurenic acid, a non-selective antagonist of all ionotropic glutamate receptors, reduces the migration of human GBM cells (Walczak et al., 2014). Even though metabotropic glutamate receptors 3 and 5 (mGluR3 and mGluR5) are expressed in glioma cells (Condorelli et al., 1997; Stepulak et al., 2009), their roles in cell mobility have not been elucidated. In addition to invasion and migration, glutamate stimulates tumor growth, proliferation, and survival of glioma cells through the EGFR-phospho-Akt and PI3K/AKT pathways (Schunemann et al., 2010; Prickett and Samuels, 2012). Overall, glioma cells release glutamate, which directly affects migration and invasion through the functional expressions of AMPARs and NMDARs in the cell (Figure 1C).
Glutamate and its receptors might be a therapeutic target for the inhibition of GBM invasion through the interaction between tumor cells and nearby cells. GBM cells release not only glutamate but also various molecules including cytokines, metabolites, and nucleic acids contributing to tumor cell progression (Almiron Bonnin et al., 2018). Therefore, targeting the secretory mechanisms could potentially develop therapeutics to reduce GBM invasion.
Conclusion
Invasion is the main characteristic of malignant glioma and one of the obstacles to radiotherapeutic, chemotherapeutic, and surgical treatments. This review provides an overview of ECM, Ca2+ signaling, and glutamate release and their associations with glioma cell invasion and migration. These components are linked with each other for invasion and migration in GBM cells (Figure 2). Glutamate released from GBM cells activates Ca2+-permeable AMPAR in an autocrine manner contributing to intracellular Ca2+ increase for invasion and migration (Figure 2).
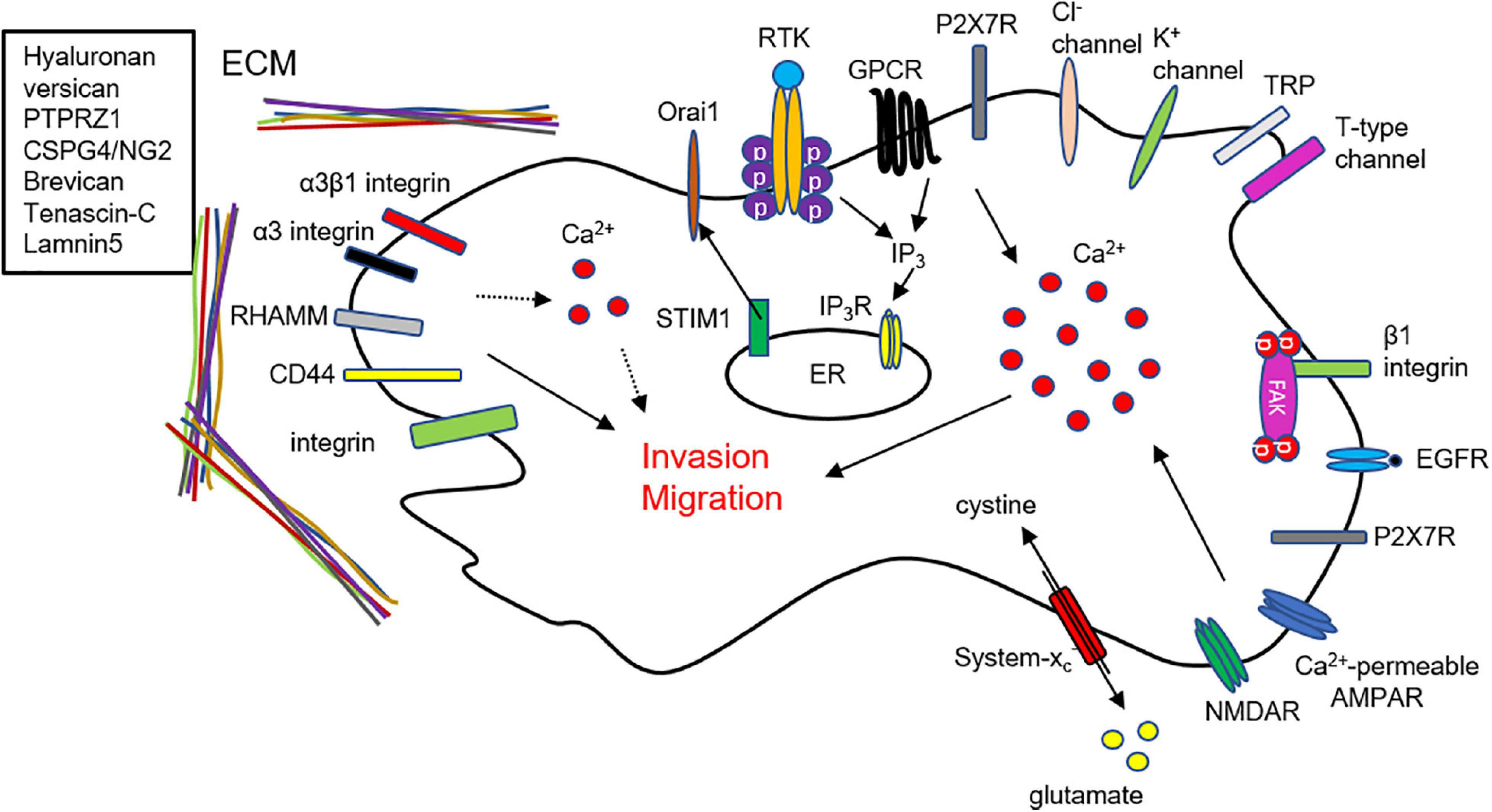
Figure 2. Schematic linking mechanisms of ECM, Ca2+ signaling, and glutamate in GBM invasion. Summary of mechanisms of glioma invasion related to ECM, Ca2+ signaling, and glutamate. Glutamate release from GBM cells activates Ca2+ permeable AMPARs and NMDARs in an autocrine manner, thereby cause an intracellular Ca2+ increase in GBM cells. ECM and their binding partners affect GBM invasion by regulating Ca2+ signaling.
To date, current treatment for GBM patients is the surgery to remove the brain tumor, followed by a combination of radiotherapy and temozolomide treatment (Stupp et al., 2005). Other agent bevacizumab that received approval for GBM treatment has shown only moderate effect (Kreisl et al., 2009). In addition, therapeutic interventions targeting ECM, Ca2+ signaling, and glutamate and aimed at blocking invasion have not been very successful in GBM patients. Mibefradil, a selective T-type Ca2+ channel blocker, followed by temozolomide was given to high-grade glioma patients (Holdhoff et al., 2017). A combination of radiation therapy with cilengitide, an inhibitor for α V integrins, was tested in GBM patients (Eisele et al., 2014). In preclinical studies, matrix metalloproteinase (MMP) inhibitors were effective in the reduction of glioma invasion, but not in clinical trials (Tonn et al., 1999; Koutroulis et al., 2008). Several drugs targeting the PI3K/Akt pathway were partially effective in preclinical studies (Drappatz et al., 2009). Talampanel, the allosteric inhibitor of AMPARs, is used in phase II trial with chemotherapy or radiotherapy (Grossman et al., 2009; Iwamoto et al., 2010).
However, these kinds of therapeutic approaches moderately increase survival and tumor still recur in all cases. A combination of those pharmacological approaches could be worthwhile to attempt, but it remains a great challenge. Glioblastoma can move into normal brain tissue escaping from surgery and radiotherapy due to its high invasiveness. Therefore, better understanding the cellular mechanism of GBM invasion could help to develop a new treatment that suppresses invasion and migration. Furthermore, the therapeutics based on mechanisms that only focus on glioma cells still have limitations. Invasion and migration can be achieved by the complex interplay between glioblastoma and surrounding cells. Therefore, future studies should focus not only on glioma cells but also on the interaction between brain tumor cells and other surrounding cells, including neurons and glial cells.
Author Contributions
J-SS and K-SH wrote the manuscript and contributed to editing and proofreading the manuscript. HK wrote the glutamate part of the manuscript and prepared the figures. All authors contributed to the article and approved the submitted version.
Funding
This work was supported by the 2020 Dongguk University Research Fund.
Conflict of Interest
The authors declare that the research was conducted in the absence of any commercial or financial relationships that could be construed as a potential conflict of interest.
References
Akiyama, Y., Jung, S., Salhia, B., Lee, S., Hubbard, S., Taylor, M., et al. (2001). Hyaluronate receptors mediating glioma cell migration and proliferation. J. Neurooncol. 53, 115–127.
Almiron Bonnin, D. A., Havrda, M. C., and Israel, M. A. (2018). Glioma Cell secretion: a driver of tumor progression and a potential therapeutic target. Cancer Res. 78, 6031–6039. doi: 10.1158/0008-5472.can-18-0345
Alptekin, M., Eroglu, S., Tutar, E., Sencan, S., Geyik, M. A., Ulasli, M., et al. (2015). Gene expressions of TRP channels in glioblastoma multiforme and relation with survival. Tumour Biol. 36, 9209–9213. doi: 10.1007/s13277-015-3577-x
Amorim, S., Reis, C. A., Reis, R. L., and Pires, R. A. (2021). Extracellular matrix mimics using hyaluronan-based biomaterials. Trends Biotechnol. 39, 90–104. doi: 10.1016/j.tibtech.2020.06.003
Angel, I., Pilo Kerman, O., Rousso-Noori, L., and Friedmann-Morvinski, D. (2020). Tenascin C promotes cancer cell plasticity in mesenchymal glioblastoma. Oncogene 39, 6990–7004. doi: 10.1038/s41388-020-01506-6
Anido, J., Saez-Borderias, A., Gonzalez-Junca, A., Rodon, L., Folch, G., Carmona, M. A., et al. (2010). TGF-beta Receptor Inhibitors Target the CD44(high)/Id1(high) Glioma-Initiating Cell Population in Human Glioblastoma. Cancer Cell 18, 655–668. doi: 10.1016/j.ccr.2010.10.023
Arslan, F., Bosserhoff, A. K., Nickl-Jockschat, T., Doerfelt, A., Bogdahn, U., and Hau, P. (2007). The role of versican isoforms V0/V1 in glioma migration mediated by transforming growth factor-beta2. Br. J. Cancer 96, 1560–1568. doi: 10.1038/sj.bjc.6603766
Asher, R. A., Morgenstern, D. A., Shearer, M. C., Adcock, K. H., Pesheva, P., and Fawcett, J. W. (2002). Versican is upregulated in CNS injury and is a product of oligodendrocyte lineage cells. J. Neurosci. 22, 2225–2236. doi: 10.1523/jneurosci.22-06-02225.2002
Beauchesne, P. (2011). Extra-neural metastases of malignant gliomas: myth or reality?”. Cancers 3, 461–477. doi: 10.3390/cancers3010461
Bomben, V. C., and Sontheimer, H. (2010). Disruption of transient receptor potential canonical channel 1 causes incomplete cytokinesis and slows the growth of human malignant gliomas. Glia 58, 1145–1156. doi: 10.1002/glia.20994
Breyer, R., Hussein, S., Radu, D. L., Putz, K. M., Gunia, S., Hecker, H., et al. (2000). Disruption of intracerebral progression of C6 rat glioblastoma by in vivo treatment with anti-CD44 monoclonal antibody. J. Neurosurg. 92, 140–149. doi: 10.3171/jns.2000.92.1.0140
Brosicke, N., van Landeghem, F. K., Scheffler, B., and Faissner, A. (2013). Tenascin-C is expressed by human glioma in vivo and shows a strong association with tumor blood vessels. Cell Tissue Res. 354, 409–430. doi: 10.1007/s00441-013-1704-9
Charles, N. A., Holland, E. C., Gilbertson, R., Glass, R., and Kettenmann, H. (2012). The brain tumor microenvironment. Glia 60, 502–514.
Chen, J. E., Pedron, S., and Harley, B. A. C. (2017). The combined influence of hydrogel stiffness and matrix-bound hyaluronic acid content on glioblastoma invasion. Macromol. Biosci. 17:10.1002/mabi.201700018.
Chen, J. E., Pedron, S., Shyu, P., Hu, Y., Sarkaria, J. N., and Harley, B. A. C. (2018). Influence of hyaluronic acid transitions in tumor microenvironment on glioblastoma malignancy and invasive behavior. Front. Mater. 5:39. doi: 10.3389/fmats.2018.00039
Chintala, S. K., Tonn, J. C., and Rao, J. S. (1999). Matrix metalloproteinases and their biological function in human gliomas. Int. J. Dev. Neurosci. 17, 495–502. doi: 10.1016/s0736-5748(99)00010-6
Chung, W. J., Lyons, S. A., Nelson, G. M., Hamza, H., Gladson, C. L., Gillespie, G. Y., et al. (2005). Inhibition of cystine uptake disrupts the growth of primary brain tumors. J. Neurosci. 25, 7101–7110. doi: 10.1523/jneurosci.5258-04.2005
Condorelli, D. F., Dell’Albani, P., Corsaro, M., Giuffrida, R., Caruso, A., Trovato Salinaro, A., et al. (1997). Metabotropic glutamate receptor expression in cultured rat astrocytes and human gliomas. Neurochem. Res. 22, 1127–1133.
Cuddapah, V. A., Robel, S., Watkins, S., and Sontheimer, H. (2014). A neurocentric perspective on glioma invasion. Nat. Rev. Neurosci. 15, 455–465. doi: 10.1038/nrn3765
Cuddapah, V. A., Turner, K. L., and Sontheimer, H. (2013). Calcium entry via TRPC1 channels activates chloride currents in human glioma cells. Cell Calcium 53, 187–194. doi: 10.1016/j.ceca.2012.11.013
de Groot, J., and Sontheimer, H. (2011). Glutamate and the biology of gliomas. Glia 59, 1181–1189. doi: 10.1002/glia.21113
Demuth, T., and Berens, M. E. (2004). Molecular mechanisms of glioma cell migration and invasion. J. Neurooncol. 70, 217–228. doi: 10.1007/s11060-004-2751-6
Ding, X., He, Z., Zhou, K., Cheng, J., Yao, H., Lu, D., et al. (2010). Essential role of TRPC6 channels in G2/M phase transition and development of human glioma. J. Natl. Cancer Inst. 102, 1052–1068. doi: 10.1093/jnci/djq217
Dityatev, A., and Schachner, M. (2003). Extracellular matrix molecules and synaptic plasticity. Nat. Rev. Neurosci. 4, 456–468. doi: 10.1038/nrn1115
Donate-Macian, P., Gomez, A., Degano, I. R., and Peralvarez-Marin, A. (2018). A TRPV2 interactome-based signature for prognosis in glioblastoma patients. Oncotarget 9, 18400–18409. doi: 10.18632/oncotarget.24843
Drappatz, J., Norden, A. D., and Wen, P. Y. (2009). Therapeutic strategies for inhibiting invasion in glioblastoma. Expert Rev. Neurother. 9, 519–534. doi: 10.1586/ern.09.10
Dwyer, C. A., Bi, W. L., Viapiano, M. S., and Matthews, R. T. (2014). Brevican knockdown reduces late-stage glioma tumor aggressiveness. J. Neurooncol. 120, 63–72. doi: 10.1007/s11060-014-1541-z
Eisele, G., Wick, A., Eisele, A. C., Clement, P. M., Tonn, J., Tabatabai, G., et al. (2014). Cilengitide treatment of newly diagnosed glioblastoma patients does not alter patterns of progression. J. Neurooncol. 117, 141–145. doi: 10.1007/s11060-014-1365-x
Fang, J., Chen, X., Zhang, L., Chen, J., Liang, Y., Li, X., et al. (2013). P2X7R suppression promotes glioma growth through epidermal growth factor receptor signal pathway. Int. J. Biochem. Cell Biol. 45, 1109–1120. doi: 10.1016/j.biocel.2013.03.005
Friedlander, D. R., Zagzag, D., Shiff, B., Cohen, H., Allen, J. C., Kelly, P. J., et al. (1996). Migration of brain tumor cells on extracellular matrix proteins in vitro correlates with tumor type and grade and involves alphaV and beta1 integrins. Cancer Res. 56, 1939–1947.
Fukushima, Y., Ohnishi, T., Arita, N., Hayakawa, T., and Sekiguchi, K. (1998). Integrin alpha3beta1-mediated interaction with laminin-5 stimulates adhesion, migration and invasion of malignant glioma cells. Int. J. Cancer 76, 63–72. doi: 10.1002/(sici)1097-0215(19980330)76:1<63::aid-ijc11>3.0.co;2-h
Gehring, M. P., Pereira, T. C., Zanin, R. F., Borges, M. C., Braga Filho, A., Battastini, A. M., et al. (2012). P2X7 receptor activation leads to increased cell death in a radiosensitive human glioma cell line. Purinergic Signal. 8, 729–739. doi: 10.1007/s11302-012-9319-2
Giese, A., and Westphal, M. (1996). Glioma invasion in the central nervous system. Neurosurgery 39, 235–250. doi: 10.1097/00006123-199608000-00001
Grossman, S. A., Ye, X., Chamberlain, M., Mikkelsen, T., Batchelor, T., Desideri, S., et al. (2009). Talampanel with standard radiation and temozolomide in patients with newly diagnosed glioblastoma: a multicenter phase II trial. J. Clin. Oncol. 27, 4155–4161. doi: 10.1200/jco.2008.21.6895
Hamilton, J. D., Rapp, M., Schneiderhan, T., Sabel, M., Hayman, A., Scherer, A., et al. (2014). Glioblastoma multiforme metastasis outside the CNS: three case reports and possible mechanisms of escape. J. Clin. Oncol. 32, e80–e84.
Held-Feindt, J., Paredes, E. B., Blomer, U., Seidenbecher, C., Stark, A. M., Mehdorn, H. M., et al. (2006). Matrix-degrading proteases ADAMTS4 and ADAMTS5 (disintegrins and metalloproteinases with thrombospondin motifs 4 and 5) are expressed in human glioblastomas. Int. J. Cancer 118, 55–61. doi: 10.1002/ijc.21258
Holdhoff, M., Ye, X., Supko, J. G., Nabors, L. B., Desai, A. S., Walbert, T., et al. (2017). Timed sequential therapy of the selective T-type calcium channel blocker mibefradil and temozolomide in patients with recurrent high-grade gliomas. Neuro Oncol. 19, 845–852. doi: 10.1093/neuonc/nox020
Horing, E., Harter, P. N., Seznec, J., Schittenhelm, J., Buhring, H. J., Bhattacharyya, S., et al. (2012). The “go or grow” potential of gliomas is linked to the neuropeptide processing enzyme carboxypeptidase E and mediated by metabolic stress. Acta Neuropathol. 124, 83–97. doi: 10.1007/s00401-011-0940-x
Hu, B., Kong, L. L., Matthews, R. T., and Viapiano, M. S. (2008). The proteoglycan brevican binds to fibronectin after proteolytic cleavage and promotes glioma cell motility. J. Biol. Chem. 283, 24848–24859. doi: 10.1074/jbc.m801433200
Ishida, J., Onishi, M., Kurozumi, K., Ichikawa, T., Fujii, K., Shimazu, Y., et al. (2014). Integrin inhibitor suppresses bevacizumab-induced glioma invasion. Transl. Oncol. 7, 292–302.e1.
Ishiuchi, S., Tsuzuki, K., Yoshida, Y., Yamada, N., Hagimura, N., Okado, H., et al. (2002). Blockage of Ca(2+)-permeable AMPA receptors suppresses migration and induces apoptosis in human glioblastoma cells. Nat. Med. 8, 971–978. doi: 10.1038/nm746
Ishiuchi, S., Yoshida, Y., Sugawara, K., Aihara, M., Ohtani, T., Watanabe, T., et al. (2007). Ca2+-permeable AMPA receptors regulate growth of human glioblastoma via Akt activation. J. Neurosci. 27, 7987–8001. doi: 10.1523/jneurosci.2180-07.2007
Iwamoto, F. M., Kreisl, T. N., Kim, L., Duic, J. P., Butman, J. A., Albert, P. S., et al. (2010). Phase 2 trial of talampanel, a glutamate receptor inhibitor, for adults with recurrent malignant gliomas. Cancer 116, 1776–1782. doi: 10.1002/cncr.24957
Ji, Z., Xie, Y., Guan, Y., Zhang, Y., Cho, K. S., Ji, M., et al. (2018). Involvement of P2X7 Receptor in Proliferation and Migration of Human Glioma Cells. Biomed Res. Int. 2018:8591397.
Jijiwa, M., Demir, H., Gupta, S., Leung, C., Joshi, K., Orozco, N., et al. (2011). CD44v6 regulates growth of brain tumor stem cells partially through the AKT-mediated pathway. PLoS One 6:e24217. doi: 10.1371/journal.pone.0024217
Kang, S. S., Han, K. S., Ku, B. M., Lee, Y. K., Hong, J., Shin, H. Y., et al. (2010). Caffeine-mediated inhibition of calcium release channel inositol 1,4,5-trisphosphate receptor subtype 3 blocks glioblastoma invasion and extends survival. Cancer Res. 70, 1173–1183. doi: 10.1158/0008-5472.can-09-2886
Kang, S., Hong, J., Lee, J. M., Moon, H. E., Jeon, B., Choi, J., et al. (2017). Trifluoperazine, a well-known antipsychotic, inhibits glioblastoma invasion by binding to calmodulin and disinhibiting calcium release channel IP3R. Mol. Cancer Ther. 16, 217–227. doi: 10.1158/1535-7163.mct-16-0169-t
Kathagen-Buhmann, A., Schulte, A., Weller, J., Holz, M., Herold-Mende, C., Glass, R., et al. (2016). Glycolysis and the pentose phosphate pathway are differentially associated with the dichotomous regulation of glioblastoma cell migration versus proliferation. Neuro Oncol. 18, 1219–1229. doi: 10.1093/neuonc/now024
Kim, C. H., Bak, K. H., Kim, Y. S., Kim, J. M., Ko, Y., Oh, S. J., et al. (2000). Expression of tenascin-C in astrocytic tumors: its relevance to proliferation and angiogenesis. Surg. Neurol. 54, 235–240. doi: 10.1016/s0090-3019(00)00307-4
Klumpp, D., Frank, S. C., Klumpp, L., Sezgin, E. C., Eckert, M., Edalat, L., et al. (2017). TRPM8 is required for survival and radioresistance of glioblastoma cells. Oncotarget 8, 95896–95913. doi: 10.18632/oncotarget.21436
Koutroulis, I., Zarros, A., and Theocharis, S. (2008). The role of matrix metalloproteinases in the pathophysiology and progression of human nervous system malignancies: a chance for the development of targeted therapeutic approaches?”. Expert Opin. Ther. Targets 12, 1577–1586. doi: 10.1517/14728220802560307
Kreisl, T. N., Kim, L., Moore, K., Duic, P., Royce, C., Stroud, I., et al. (2009). Phase II trial of single-agent bevacizumab followed by bevacizumab plus irinotecan at tumor progression in recurrent glioblastoma. J. Clin. Oncol. 27, 740–745. doi: 10.1200/jco.2008.16.3055
Kuppner, M. C., Van Meir, E., Gauthier, T., Hamou, M. F., and de Tribolet, N. (1992). Differential expression of the CD44 molecule in human brain tumours. Int. J. Cancer 50, 572–577. doi: 10.1002/ijc.2910500414
Latijnhouwers, M. A., de Jongh, G. J., Bergers, M., de Rooij, M. J., and Schalkwijk, J. (2000). Expression of tenascin-C splice variants by human skin cells. Arch. Dermatol. Res. 292, 446–454. doi: 10.1007/s004030000152
Leng, T. D., Li, M. H., Shen, J. F., Liu, M. L., Li, X. B., Sun, H. W., et al. (2015). Suppression of TRPM7 inhibits proliferation, migration, and invasion of malignant human glioma cells. CNS Neurosci. Ther. 21, 252–261. doi: 10.1111/cns.12354
Li, S., Wang, J., Wei, Y., Liu, Y., Ding, X., Dong, B., et al. (2015). Crucial role of TRPC6 in maintaining the stability of HIF-1alpha in glioma cells under hypoxia. J. Cell Sci. 128, 3317–3329. doi: 10.1242/jcs.173161
Li, T., Yi, L., Hai, L., Ma, H., Tao, Z., Zhang, C., et al. (2018). The interactome and spatial redistribution feature of Ca(2+) receptor protein calmodulin reveals a novel role in invadopodia-mediated invasion. Cell Death Dis. 9:292.
Liu, H., Hughes, J. D., Rollins, S., Chen, B., and Perkins, E. (2011). Calcium entry via ORAI1 regulates glioblastoma cell proliferation and apoptosis. Exp. Mol. Pathol. 91, 753–760. doi: 10.1016/j.yexmp.2011.09.005
Liu, M., Inoue, K., Leng, T., Guo, S., and Xiong, Z. G. (2014). TRPM7 channels regulate glioma stem cell through STAT3 and Notch signaling pathways. Cell. Signal. 26, 2773–2781. doi: 10.1016/j.cellsig.2014.08.020
Lokeshwar, V. B., Mirza, S., and Jordan, A. (2014). Targeting hyaluronic acid family for cancer chemoprevention and therapy. Adv. Cancer Res. 123, 35–65. doi: 10.1016/b978-0-12-800092-2.00002-2
Lokeshwar, V. B., Obek, C., Soloway, M. S., and Block, N. L. (1997). Tumor-associated hyaluronic acid: a new sensitive and specific urine marker for bladder cancer. Cancer Res. 57, 773–777.
Louis, D. N., Ohgaki, H., Wiestler, O. D., Cavenee, W. K., Burger, P. C., Jouvet, A., et al. (2007). The 2007 WHO classification of tumours of the central nervous system. Acta Neuropathol. 114, 97–109. doi: 10.1007/978-94-007-1399-4_10
Lu, R., Wu, C., Guo, L., Liu, Y., Mo, W., Wang, H., et al. (2012). The role of brevican in glioma: promoting tumor cell motility in vitro and in vivo. BMC Cancer 12:607. doi: 10.1186/1471-2407-12-607
Lun, M., Lok, E., Gautam, S., Wu, E., and Wong, E. T. (2011). The natural history of extracranial metastasis from glioblastoma multiforme. J. Neurooncol. 105, 261–273. doi: 10.1007/s11060-011-0575-8
Lyons, S. A., Chung, W. J., Weaver, A. K., Ogunrinu, T., and Sontheimer, H. (2007). Autocrine glutamate signaling promotes glioma cell invasion. Cancer Res. 67, 9463–9471. doi: 10.1158/0008-5472.can-07-2034
Merzak, A., Koocheckpour, S., and Pilkington, G. J. (1994). CD44 mediates human glioma cell adhesion and invasion in vitro. Cancer Res. 54, 3988–3992.
Misra, S., Hascall, V. C., Markwald, R. R., and Ghatak, S. (2015). Interactions between Hyaluronan and Its Receptors (CD44, RHAMM) Regulate the Activities of Inflammation and Cancer. Front. Immunol. 6:201. doi: 10.3389/fimmu.2015.00201
Miyata, S., and Kitagawa, H. (2017). Formation and remodeling of the brain extracellular matrix in neural plasticity: roles of chondroitin sulfate and hyaluronan. Biochim. Biophys. Acta Gen. Subj. 1861, 2420–2434. doi: 10.1016/j.bbagen.2017.06.010
Monaghan, M., Mulligan, K. A., Gillespie, H., Trimble, A., Winter, P., Johnston, P. G., et al. (2000). Epidermal growth factor up-regulates CD44-dependent astrocytoma invasion in vitro. J. Pathol. 192, 519–525. doi: 10.1002/1096-9896(2000)9999:9999<::aid-path784>3.0.co;2-m
Motiani, R. K., Hyzinski-Garcia, M. C., Zhang, X., Henkel, M. M., Abdullaev, I. F., Kuo, Y. H., et al. (2013). STIM1 and Orai1 mediate CRAC channel activity and are essential for human glioblastoma invasion. Pflugers Arch. 465, 1249–1260. doi: 10.1007/s00424-013-1254-8
Muller-Langle, A., Lutz, H., Hehlgans, S., Rodel, F., Rau, K., and Laube, B. (2019). NMDA receptor-mediated signaling pathways enhance radiation resistance, survival and migration in glioblastoma cells-A potential target for adjuvant radiotherapy. Cancers 11:503. doi: 10.3390/cancers11040503
Nakada, M., Nambu, E., Furuyama, N., Yoshida, Y., Takino, T., Hayashi, Y., et al. (2013). Integrin alpha3 is overexpressed in glioma stem-like cells and promotes invasion. Br. J. Cancer 108, 2516–2524. doi: 10.1038/bjc.2013.218
Nakagawa, T., Kubota, T., Kabuto, M., and Kodera, T. (1996). Hyaluronic acid facilitates glioma cell invasion in vitro. Anticancer Res. 16, 2917–2922.
Nandakumar, D. N., Ramaswamy, P., Prasad, C., Srinivas, D., and Goswami, K. (2019). Glioblastoma invasion and NMDA receptors: a novel prospect. Physiol. Int. 106, 250–260. doi: 10.1556/2060.106.2019.22
Naor, D. (2016). Editorial: interaction between hyaluronic acid and its receptors (CD44, RHAMM) regulates the activity of inflammation and cancer. Front. Immunol. 7:39. doi: 10.3389/fimmu.2016.00039
Nicoletti, N. F., Erig, T. C., Zanin, R. F., Roxo, M. R., Ferreira, N. P., Gomez, M. V., et al. (2017). Pre-clinical evaluation of voltage-gated calcium channel blockers derived from the spider P. nigriventer in glioma progression. Toxicon 129, 58–67. doi: 10.1016/j.toxicon.2017.02.001
Noch, E., and Khalili, K. (2009). Molecular mechanisms of necrosis in glioblastoma: the role of glutamate excitotoxicity. Cancer Biol. Ther. 8, 1791–1797. doi: 10.4161/cbt.8.19.9762
Orend, G., and Chiquet-Ehrismann, R. (2006). Tenascin-C induced signaling in cancer. Cancer Lett. 244, 143–163. doi: 10.1016/j.canlet.2006.02.017
Ostrom, Q. T., Cote, D. J., Ascha, M., Kruchko, C., and Barnholtz-Sloan, J. S. (2018). Adult Glioma incidence and survival by race or ethnicity in the United States From 2000 to 2014. JAMA Oncol. 4, 1254–1262. doi: 10.1001/jamaoncol.2018.1789
Ostrom, Q. T., Gittleman, H., Liao, P., Vecchione-Koval, T., Wolinsky, Y., Kruchko, C., et al. (2017). CBTRUS statistical report: primary brain and other central nervous system tumors diagnosed in the United States in 2010-2014. Neuro Oncol. 19(Suppl._5), v1–v88.
Ou-Yang, Q., Li, B., Xu, M., and Liang, H. (2018). TRPV4 promotes the migration and invasion of glioma cells via AKT/Rac1 signaling. Biochem. Biophys. Res. Commun. 503, 876–881. doi: 10.1016/j.bbrc.2018.06.090
Panner, A., Cribbs, L. L., Zainelli, G. M., Origitano, T. C., Singh, S., and Wurster, R. D. (2005). Variation of T-type calcium channel protein expression affects cell division of cultured tumor cells. Cell Calcium 37, 105–119. doi: 10.1016/j.ceca.2004.07.002
Park, J. B., Kwak, H. J., and Lee, S. H. (2008). Role of hyaluronan in glioma invasion. Cell Adh. Migr. 2, 202–207. doi: 10.4161/cam.2.3.6320
Paulus, W., Baur, I., Dours-Zimmermann, M. T., and Zimmermann, D. R. (1996). Differential expression of versican isoforms in brain tumors. J. Neuropathol. Exp. Neurol. 55, 528–533. doi: 10.1097/00005072-199605000-00005
Piao, Y., Lu, L., and de Groot, J. (2009). AMPA receptors promote perivascular glioma invasion via beta1 integrin-dependent adhesion to the extracellular matrix. Neuro Oncol. 11, 260–273. doi: 10.1215/15228517-2008-094
Prickett, T. D., and Samuels, Y. (2012). Molecular pathways: dysregulated glutamatergic signaling pathways in cancer. Clin. Cancer Res. 18, 4240–4246. doi: 10.1158/1078-0432.ccr-11-1217
Ridley, A. J., Schwartz, M. A., Burridge, K., Firtel, R. A., Ginsberg, M. H., Borisy, G., et al. (2003). Cell migration: integrating signals from front to back. Science 302, 1704–1709. doi: 10.1126/science.1092053
Ruggieri, P., Mangino, G., Fioretti, B., Catacuzzeno, L., Puca, R., Ponti, D., et al. (2012). The inhibition of KCa3.1 channels activity reduces cell motility in glioblastoma derived cancer stem cells. PLoS One 7:e47825. doi: 10.1371/journal.pone.0047825
Ryu, J. K., Jantaratnotai, N., Serrano-Perez, M. C., McGeer, P. L., and McLarnon, J. G. (2011). Block of purinergic P2X7R inhibits tumor growth in a C6 glioma brain tumor animal model. J. Neuropathol. Exp. Neurol. 70, 13–22. doi: 10.1097/nen.0b013e318201d4d4
Saito, Y., Imazeki, H., Miura, S., Yoshimura, T., Okutsu, H., Harada, Y., et al. (2007). A peptide derived from tenascin-C induces beta1 integrin activation through syndecan-4. J. Biol. Chem. 282, 34929–34937. doi: 10.1074/jbc.m705608200
Sarkar, S., Nuttall, R. K., Liu, S., Edwards, D. R., and Yong, V. W. (2006). Tenascin-C stimulates glioma cell invasion through matrix metalloproteinase-12. Cancer Res. 66, 11771–11780. doi: 10.1158/0008-5472.can-05-0470
Sarkar, S., Zemp, F. J., Senger, D., Robbins, S. M., and Yong, V. W. (2015). ADAM-9 is a novel mediator of tenascin-C-stimulated invasiveness of brain tumor-initiating cells. Neuro Oncol. 17, 1095–1105. doi: 10.1093/neuonc/nou362
Schunemann, D. P., Grivicich, I., Regner, A., Leal, L. F., de Araujo, D. R., Jotz, G. P., et al. (2010). Glutamate promotes cell growth by EGFR signaling on U-87MG human glioblastoma cell line. Pathol. Oncol. Res. 16, 285–293. doi: 10.1007/s12253-009-9223-4
Sontheimer, H. (2008). A role for glutamate in growth and invasion of primary brain tumors. J. Neurochem. 105, 287–295. doi: 10.1111/j.1471-4159.2008.05301.x
Stepulak, A., Luksch, H., Gebhardt, C., Uckermann, O., Marzahn, J., Sifringer, M., et al. (2009). Expression of glutamate receptor subunits in human cancers. Histochem. Cell Biol. 132, 435–445.
Strong, A. D., Indart, M. C., Hill, N. R., and Daniels, R. L. (2018). GL261 glioma tumor cells respond to ATP with an intracellular calcium rise and glutamate release. Mol. Cell. Biochem. 446, 53–62. doi: 10.1007/s11010-018-3272-5
Stupp, R., Mason, W. P., van den Bent, M. J., Weller, M., Fisher, B., Taphoorn, M. J., et al. (2005). Radiotherapy plus concomitant and adjuvant temozolomide for glioblastoma. N. Engl. J. Med. 352, 987–996.
Takeuchi, S., Wada, K., Toyooka, T., Shinomiya, N., Shimazaki, H., Nakanishi, K., et al. (2013). Increased xCT expression correlates with tumor invasion and outcome in patients with glioblastomas. Neurosurgery 72, 33–41. doi: 10.1227/neu.0b013e318276b2de
Tamajusuku, A. S., Villodre, E. S., Paulus, R., Coutinho-Silva, R., Battasstini, A. M., Wink, M. R., et al. (2010). Characterization of ATP-induced cell death in the GL261 mouse glioma. J. Cell. Biochem. 109, 983–991. doi: 10.1002/jcb.22478
Theocharis, A. D., Skandalis, S. S., Gialeli, C., and Karamanos, N. K. (2016). Extracellular matrix structure. Adv. Drug Deliv. Rev. 97, 4–27.
Thompson, E. G., and Sontheimer, H. (2016). A role for ion channels in perivascular glioma invasion. Eur. Biophys. J. 45, 635–648. doi: 10.1007/s00249-016-1154-x
Tonn, J. C., Kerkau, S., Hanke, A., Bouterfa, H., Mueller, J. G., Wagner, S., et al. (1999). Effect of synthetic matrix-metalloproteinase inhibitors on invasive capacity and proliferation of human malignant gliomas in vitro. Int. J. Cancer 80, 764–772. doi: 10.1002/(sici)1097-0215(19990301)80:5<764::aid-ijc22<3.0.co;2-j
Toole, B. P. (2004). Hyaluronan: from extracellular glue to pericellular cue. Nat. Rev. Cancer 4, 528–539. doi: 10.1038/nrc1391
Treasurywala, S., and Berens, M. E. (1998). Migration arrest in glioma cells is dependent on the alphav integrin subunit. Glia 24, 236–243. doi: 10.1002/(sici)1098-1136(199810)24:2<236::aid-glia8>3.0.co;2-1
Tsukita, S., Oishi, K., Sato, N., Sagara, J., Kawai, A., and Tsukita, S. (1994). ERM family members as molecular linkers between the cell surface glycoprotein CD44 and actin-based cytoskeletons. J. Cell Biol. 126, 391–401. doi: 10.1083/jcb.126.2.391
Turner, K. L., Honasoge, A., Robert, S. M., McFerrin, M. M., and Sontheimer, H. (2014). A proinvasive role for the Ca(2+) -activated K(+) channel KCa3.1 in malignant glioma. Glia 62, 971–981. doi: 10.1002/glia.22655
Ulbricht, U., Eckerich, C., Fillbrandt, R., Westphal, M., and Lamszus, K. (2006). RNA interference targeting protein tyrosine phosphatase zeta/receptor-type protein tyrosine phosphatase beta suppresses glioblastoma growth in vitro and in vivo. J. Neurochem. 98, 1497–1506. doi: 10.1111/j.1471-4159.2006.04022.x
Valerie, N. C., Dziegielewska, B., Hosing, A. S., Augustin, E., Gray, L. S., Brautigan, D. L., et al. (2013). Inhibition of T-type calcium channels disrupts Akt signaling and promotes apoptosis in glioblastoma cells. Biochem. Pharmacol. 85, 888–897. doi: 10.1016/j.bcp.2012.12.017
Visa, A., Sallan, M. C., Maiques, O., Alza, L., Talavera, E., Lopez-Ortega, R., et al. (2019). T-Type Cav3.1 channels mediate progression and chemotherapeutic resistance in glioblastoma. Cancer Res. 79, 1857–1868. doi: 10.1158/0008-5472.can-18-1924
Wade, A., Robinson, A. E., Engler, J. R., Petritsch, C., James, C. D., and Phillips, J. J. (2013). Proteoglycans and their roles in brain cancer. FEBS J. 280, 2399–2417. doi: 10.1111/febs.12109
Walczak, K., Deneka-Hannemann, S., Jarosz, B., Zgrajka, W., Stoma, F., Trojanowski, T., et al. (2014). Kynurenic acid inhibits proliferation and migration of human glioblastoma T98G cells. Pharmacol. Rep. 66, 130–136. doi: 10.1016/j.pharep.2013.06.007
Wan, J., Guo, A. A., Chowdhury, I., Guo, S., Hibbert, J., Wang, G., et al. (2019). TRPM7 induces mechanistic target of Rap1b through the downregulation of miR-28-5p in Glioma proliferation and invasion. Front. Oncol. 9:1413. doi: 10.3389/fonc.2019.01413
Wang, J., Svendsen, A., Kmiecik, J., Immervoll, H., Skaftnesmo, K. O., Planaguma, J., et al. (2011). Targeting the NG2/CSPG4 proteoglycan retards tumour growth and angiogenesis in preclinical models of GBM and melanoma. PLoS One 6:e23062. doi: 10.1371/journal.pone.0023062
Wang, X. Y., Li, Y. L., Wang, H. Y., Zhu, M., Guo, D., Wang, G. L., et al. (2017). Propofol inhibits invasion and proliferation of C6 glioma cells by regulating the Ca(2+) permeable AMPA receptor-system xc(-) pathway. Toxicol. In Vitro 44, 57–65. doi: 10.1016/j.tiv.2017.06.026
Wei, W. C., Huang, W. C., Lin, Y. P., Becker, E. B. E., Ansorge, O., Flockerzi, V., et al. (2017). Functional expression of calcium-permeable canonical transient receptor potential 4-containing channels promotes migration of medulloblastoma cells. J. Physiol. 595, 5525–5544. doi: 10.1113/jp274659
Wei, W., Ryu, J. K., Choi, H. B., and McLarnon, J. G. (2008). Expression and function of the P2X(7) receptor in rat C6 glioma cells. Cancer Lett. 260, 79–87. doi: 10.1016/j.canlet.2007.10.025
Wen, P. Y., and Kesari, S. (2008). Malignant gliomas in adults. N. Engl. J. Med. 359, 492–507. doi: 10.1056/nejmra0708126
Wondergem, R., and Bartley, J. W. (2009). Menthol increases human glioblastoma intracellular Ca2+, BK channel activity and cell migration. J. Biomed. Sci. 16:90. doi: 10.1186/1423-0127-16-90
Wong, R., Gong, H., Alanazi, R., Bondoc, A., Luck, A., Sabha, N., et al. (2020). Inhibition of TRPM7 with waixenicin A reduces glioblastoma cellular functions. Cell Calcium 92:102307. doi: 10.1016/j.ceca.2020.102307
Xia, S., Lal, B., Tung, B., Wang, S., Goodwin, C. R., and Laterra, J. (2016). Tumor microenvironment tenascin-C promotes glioblastoma invasion and negatively regulates tumor proliferation. Neuro Oncol. 18, 507–517. doi: 10.1093/neuonc/nov171
Yang, W., Wu, P. F., Ma, J. X., Liao, M. J., Xu, L. S., and Yi, L. (2020). TRPV4 activates the Cdc42/N-wasp pathway to promote glioblastoma invasion by altering cellular protrusions. Sci. Rep. 10:14151.
Yao, P. S., Kang, D. Z., Lin, R. Y., Ye, B., Wang, W., and Ye, Z. C. (2014). Glutamate/glutamine metabolism coupling between astrocytes and glioma cells: neuroprotection and inhibition of glioma growth. Biochem. Biophys. Res. Commun. 450, 295–299. doi: 10.1016/j.bbrc.2014.05.120
Ye, Z. C., and Sontheimer, H. (1999). Glioma cells release excitotoxic concentrations of glutamate. Cancer Res. 59, 4383–4391.
Yoshida, T., Matsuda, Y., Naito, Z., and Ishiwata, T. (2012). CD44 in human glioma correlates with histopathological grade and cell migration. Pathol. Int. 62, 463–470. doi: 10.1111/j.1440-1827.2012.02823.x
Yuan, F., Yi, L., Hai, L., Wang, Y., Yang, Y., Li, T., et al. (2019). Identification of Key pathways and genes in the Orai2 mediated classical and mesenchymal subtype of glioblastoma by bioinformatic analyses. Dis. Markers 2019:7049294.
Zeng, J., Wu, Y., Zhuang, S., Qin, L., Hua, S., Mungur, R., et al. (2019). Identification of the role of TRPM8 in glioblastoma and its effect on proliferation, apoptosis and invasion of the U251 human glioblastoma cell line. Oncol. Rep. 42, 1517–1526.
Zhang, J. F., Tao, T., Wang, K., Zhang, G. X., Yan, Y., Lin, H. R., et al. (2019). IL-33/ST2 axis promotes glioblastoma cell invasion by accumulating tenascin-C. Sci. Rep. 9:20276.
Zhang, L., Meng, X., Shan, X., Gu, T., Zhang, J., Feng, S., et al. (2019). Integrin alphavbeta3-Specific Hydrocyanine for Cooperative Targeting of Glioblastoma with High Sensitivity and Specificity. Anal. Chem. 91, 12587–12595. doi: 10.1021/acs.analchem.9b03725
Zhang, Y., Cruickshanks, N., Yuan, F., Wang, B., Pahuski, M., Wulfkuhle, J., et al. (2017). Targetable T-type Calcium Channels Drive Glioblastoma. Cancer Res. 77, 3479–3490. doi: 10.1158/0008-5472.can-16-2347
Zhang, Y., Zhang, J., Jiang, D., Zhang, D., Qian, Z., Liu, C., et al. (2012). Inhibition of T-type Ca(2)(+) channels by endostatin attenuates human glioblastoma cell proliferation and migration. Br. J. Pharmacol. 166, 1247–1260. doi: 10.1111/j.1476-5381.2012.01852.x
Zhou, P., Erfani, S., Liu, Z., Jia, C., Chen, Y., Xu, B., et al. (2015). CD151-alpha3beta1 integrin complexes are prognostic markers of glioblastoma and cooperate with EGFR to drive tumor cell motility and invasion. Oncotarget 6, 29675–29693. doi: 10.18632/oncotarget.4896
Zhu, M., Chen, L., Zhao, P., Zhou, H., Zhang, C., Yu, S., et al. (2014). Store-operated Ca(2+) entry regulates glioma cell migration and invasion via modulation of Pyk2 phosphorylation. J. Exp. Clin. Cancer Res. 33:98. doi: 10.1186/preaccept-3101393591453932
Keywords: glioblastoma, invasion, extracellular matrix, Ca2+, glutamate, ion channels
Citation: So J-S, Kim H and Han K-S (2021) Mechanisms of Invasion in Glioblastoma: Extracellular Matrix, Ca2+ Signaling, and Glutamate. Front. Cell. Neurosci. 15:663092. doi: 10.3389/fncel.2021.663092
Received: 02 February 2021; Accepted: 29 April 2021;
Published: 02 June 2021.
Edited by:
Jinsoo Seo, Daegu Gyeongbuk Institute of Science and Technology (DGIST), South KoreaReviewed by:
Andrea Gerbino, University of Bari Aldo Moro, ItalySara Pedron, University of Illinois at Urbana-Champaign, United States
Copyright © 2021 So, Kim and Han. This is an open-access article distributed under the terms of the Creative Commons Attribution License (CC BY). The use, distribution or reproduction in other forums is permitted, provided the original author(s) and the copyright owner(s) are credited and that the original publication in this journal is cited, in accordance with accepted academic practice. No use, distribution or reproduction is permitted which does not comply with these terms.
*Correspondence: Kyung-Seok Han, a3NoYW5AZG9uZ2d1ay5hYy5rcg==