- 1Department of Translational Medical Sciences, University of Naples Federico II, Naples, Italy
- 2Center for Basic and Clinical Immunology Research (CISI), University of Naples Federico II, Naples, Italy
- 3World Allergy Organization Center of Excellence, University of Naples Federico II, Naples, Italy
- 4Center for Experimental Medicine and Rheumatology, William Harvey Research Institute, Barts and The London School of Medicine and Dentistry, Queen Mary University of London, London, United Kingdom
- 5Institute of Experimental Endocrinology and Oncology “Gaetano Salvatore”, National Research Council (CNR), Naples, Italy
Mast cells and their mediators play a role in the control of homeostasis and in the pathogenesis of several disorders. The concept of rodent mast cell heterogeneity, initially established in the mid-1960s has been extended in humans. Human mast cells isolated and purified from different anatomic sites can be activated via aggregation of cell surface high affinity IgE receptors (FcεRI) by antigens, superantigens, anti-IgE, and anti-FcεRI. MAS-related G protein-coupled receptor-X2 (MRGPRX2) is expressed at high level in human skin mast cells (MCs) (HSMCs), synovial MCs (HSyMCs), but not in lung MCs (HLMCs). MRGPX2 can be activated by neuropeptide substance P, several opioids, cationic drugs, and 48/80. Substance P (5 × 10−7 M – 5 × 10−6 M) induced histamine and tryptase release from HSMCs and to a lesser extent from HSyMCs, but not from HLMCs and human cardiac MCs (HHMCs). Morphine (10−5 M – 3 × 10−4 M) selectively induced histamine and tryptase release from HSMCs, but not from HLMCs and HHMCs. SP and morphine were incomplete secretagogues because they did not induce the de novo synthesis of arachidonic acid metabolites from human mast cells. In the same experiments anti-IgE (3 μg/ml) induced the release of histamine and tryptase and the de novo synthesis of prostaglandin D2 (PGD2) from HLMCs, HHMCs, HSyMCs, and HSMCs. By contrast, anti-IgE induced the production of leukotriene C4 (LTC4) from HLMCs, HHMCs, HSyMCs, but not from HSMCs. These results are compatible with the heterogeneous expression and function of MRGPRX2 receptor on primary human mast cells isolated from different anatomic sites.
Introduction
Mast cells arise from stem cell-derived human mast cell progenitors in the bone marrow, circulate and complete their maturation in all vascularized tissues (Galli, 2016; Olivera et al., 2018). Mast cell differentiation, phenotypes and functions in tissues are largely determined by the microenvironment (e.g., cytokines, activating and inhibitory stimuli, chemokines) (Mukai et al., 2018). Mast cells are canonically implicated in allergic disorders (Liccardi et al., 2003; Fujisawa et al., 2014; Bradding and Arthur, 2016; Canonica et al., 2016; Subramanian et al., 2016; Mukai et al., 2018), but also in several non allergic conditions including autoimmune disorders (de Paulis et al., 1996; Brown and Weinberg, 2018; Rivellese et al., 2018; Yu et al., 2018), cardiovascular diseases (Patella et al., 1996, 1998; Theoharides et al., 2011; Shi et al., 2015; Ngkelo et al., 2016), bacterial (Piliponsky and Romani, 2018) and viral diseases (Haidl and Marshall, 2015), neurological disorders (Skaper et al., 2014; Theoharides et al., 2016; Skaper et al., 2017; Conti et al., 2018), and cancer (Galdiero et al., 2016; Varricchi et al., 2017). Increasing evidence supports the role of mast cells and their mediators in neurogenic inflammation leading to pain and itch (Gupta and Harvima, 2018; Steinhoff et al., 2018; Yosipovitch et al., 2018).
Enerbäck first established the concept of mast cell heterogeneity through detailed morphological and histochemical studies (Enerback, 1966a,b,c). Two distinct subpopulations of rodent mast cells, connective tissue mast cells and mucosal mast cells, differ in their location, staining features, mediator content and responsiveness to activating stimuli (Enerback, 1966a,b,c; Tainsh and Pearce, 1992; Varricchi et al., 2016). Mast cells isolated and purified from several human tissues have led to the recognition of histochemical, functional, and immunological differences among these cells in humans (Church et al., 1982; Schwartz et al., 1987; Casolaro et al., 1989; Stellato et al., 1991; Bischoff and Dahinden, 1992). For example, activation of mast cells isolated from human lung (HLMCs) by antigens, anti-IgE and superantigens leads to arachidonic acid metabolism through both the cyclooxygenase (prostaglandin D2, PGD2) and the 5-lipoxygenase pathway (peptide leukotriene C4, LTC4) (Schulman et al., 1982; de Paulis et al., 1991; Stellato et al., 1992a), whereas HSMCs only synthesize PGD2 (Benyon et al., 1987; Stellato et al., 1992b). Based on their protease composition, two types of human mast cells have been proposed: tryptase+ chymase+ cells (MTC), and tryptase+ chymase− (MT), being the prototypes (Schwartz et al., 1987). However, this traditional classification is rather simplistic and mast cells show significant plasticity (Galli et al., 2011; Borriello et al., 2014). Indeed, analysis of human mast cell transcriptome demonstrated considerable greater heterogeneity across tissues than previously appreciated (Motakis et al., 2014; Dwyer et al., 2016). Moreover, recent evidence indicates that each of the two mast cell subsets originates from different precursors through several waves of mast cell differentiation, and that they display distinct surface receptors and mediators (Gentek et al., 2018; Li et al., 2018).
Human mast cells can be activated by the engagement of a plethora of receptors (Varricchi et al., 2018). Aggregation of cell surface FcεRI by antigens, anti-IgE or superantigens leads to the degranulation and the generation of newly synthesized lipid mediators, cytokines, angiogenic, and lymphangiogenic factors (Marone et al., 2006; Detoraki et al., 2009; Theoharides et al., 2010; Taracanova et al., 2018). The identification of MRGPRX2 receptor and its mouse orthologue Mrgprb2 has opened a new research avenue in mast cell biology (Tatemoto et al., 2006; Fujisawa et al., 2014; McNeil et al., 2015). MRGPRX2 can be activated by several ligands such as neuropeptides (e.g., substance P, VIP, etc.), opioids (i.e., morphine), cationic drugs (e.g., atracurium, icatibant), and 48/80 (Tatemoto et al., 2006; McNeil et al., 2015; Ali, 2017). A clinical relevance is emerging for MRGPRX2 because this receptor is implicated in drug reactions (McNeil et al., 2015) and is overexpressed in HSMCs of patients with chronic urticaria (Fujisawa et al., 2014). Gaudenzio et al. have elegantly demonstrated that substance P (SP) and IgE cross-linking (i.e., anti-IgE) induce distinct mast cell degranulation strategies in human primary MC cultures and mouse MCs (Gaudenzio et al., 2016). In this study we compared the patterns of responsiveness to anti-IgE and to MRGPRX2 agonists (SP and morphine) and the mediators synthesized by primary human lung (HLMCs), cardiac (HHMCs), skin (HSMCs), and synovial MCs (HSyMCs).
Materials and Methods
Reagents
HClO4 (Baker Chemical Co., Deventer, Netherlands), BSA, piperazine-N, N′-bis (2-ethanesulfonic acid), hyaluronidase, chymopapain, elastase type I, morphine, substance P, LTC4, and PGD2 (Sigma Chemical Co., St. Louis, MO), collagenase (Worthington Biochemical Co., Freehold, NJ), Hanks’ balanced salt solution and fetal calf serum (FCS; GIBCO, Grand Island, NY), deoxyribonuclease I and pronase (Calbiochem, La Jolla, CA), RPMI 1640 with 25 mM HEPES buffer, Eagle’s minimum essential medium (Flow Laboratories, Irvine, United Kingdom), Percoll (Pharmacia Fine Chemicals, Uppsala, Sweden), (3H)-LCT4 and (3H)-PGD2 (New England Nuclear, Boston, MA) were commercially purchased. CD117 MicroBead kit was purchased from Miltenyi Biotec (Bologna, Italy). Rabbit anti-IgE antibody was kindly donated by Dr. Kimishige Ishizaka (La Jolla Institute for Allergy and Immunology, La Jolla, CA). Rabbit anti-LTC4 antibody was a gift of Dr. Lawrence M. Lichtenstein (The Johns Hopkins University, Baltimore, MD). Tryptase fluoro-enzyme immune assay (FEIA; Phadia Diagnostic AB, Uppsala, Sweden) was kindly donated by Kabi Pharmacia (Milan, Italy).
Buffers
The Pipes buffer used in these experiments was made by 25 mM Pipes, 110 mM NaCl, 5 mM KCl, pH 7.37 and referred to as P buffer. P2CG contains, in addition to P buffer, 2 mM CaCl2 and 1 g/l dextrose (Patella et al., 1996); pH was titrated to 7.4 with sodium bicarbonate. PGMD contains 1 mM MgCl2, 10 mg/l DNase, and 1 g/l gelatin in addition to P buffer, pH 7.37. The Tyrode’s buffer was made by 12 mM NaHCO3, 127 mM NaCl, 5 mM KCl, 0.5 mM NaH2PO4, 1 mM MgCl2, 5 mM glucose, and 10 mM HEPES.
Isolation of HLMCs
The study was approved by the Ethics Committee of the University of Naples Federico II (N. 7/9). Macroscopically normal lung tissue obtained from patients undergoing lung resection for lung cancer was dissected free from pleura, bronchi, and blood vessels, minced into 5- to 10-mm fragments and dispersed into a single cell suspension as previously described (de Paulis et al., 1991; Staiano et al., 2016). Yields with this technique ranged between 0.4 × 105 and 1.5 × 106 mast cells per g of wet tissue and purity between 1 and 8%. Mast cells of enhanced purity (10–65%) were obtained by flotation over discontinuous Percoll gradient. Mast cells were further purified using a CD117 MicroBead kit sorting system according to the manufacturer’s instructions. Mast cell purities using this technique ranged from 85 to 98%.
Isolation of HSMCs
Skin obtained from patients undergoing either mastectomy for breast cancer or elective cosmetic surgery was separated from the subcutaneous fat by blunt dissection. The tissue was cut into 1- to 2-mm fragments dispersed into single cell suspension as previously described (de Paulis et al., 1992). Yields with this technique ranged between 0.1 and 0.9 × 106 mast cells/g of wet tissue and purities were between 4 and 8%. Mast cells were further purified using a CD117 MicroBead kit cell sorting system (Miltenyi Biotec, Bologna, Italy) according to the manufacturer’s instructions. Mast cell purities using this technique ranged from 84 to 96%.
Isolation of HHMCs
The heart tissue used in this study was obtained from patients undergoing heart transplantation at the Deutsches Herzzentrum, Berlin, mostly for cardiomyopathy (Patella et al., 1998). The explanted heart, immediately immersed in cold (4°C) cardioplegic solution, was processed within 5–18 h of removal. Fat tissue, large vessels, and pericardium were removed. The tissue was finely minced into 2- to 5-mm fragments, suspended in P buffer (10 ml/g of wet tissue), and washed by centrifugation 3 times. After each centrifugation, the heart fragments were filtered through a 150-μm pore Nytex cloth (Tetko, Elmsford, NY). Fragments were incubated (15 min, 37°C) under constant stirring in P buffer containing 10 mg collagenase/g of wet tissue. At the end of the incubation the cell suspension was filtered through a 150-μm pore Nytex cloth and three additional cycles of enzymatic digestion were performed. After the last procedure, the cells were centrifuged (150 g, 22°C, 8 min) and filtered through a 60-μm pore Nytex cloth to remove large particles and large cells (mostly myocytes). Lastly, cells were washed twice in PGMD by centrifugation (150 g, 22°C, 8 min). Cell pellets were resuspended in P buffer containing 2% BSA and centrifuged (25 g, 22°C, 2 min) to remove sedimented myocytes. Myocytes (>100 μm long) were pelleted and discarded; supernatants containing endothelial cells, fibroblasts and mast cells were then collected and centrifuged (150 g, 22°C, 8 min). HHMCs were partially purified by flotation through a discontinuous Percoll gradient (Patella et al., 1998). The purity of these populations ranged from 0.1 to 18%. The enzymatic dispersion tissue yielded ≈ 5 × 104 mast cells per gram of heart tissue. HHMCs were further purified using a CD117 MicroBead kit sorting system. Mast cell purities using these techniques ranged from 26 to 58%.
Isolation of HSyMCs
The synovial tissue used in this study was obtained from patients with osteorthrites or rheumatoid arthritis undergoing synoviectomy. Resected joint tissue was immersed in P buffer (4°C) and was processed within 2 h of removal (de Paulis et al., 1996). Fat and fibrous tissue were removed and the tissue was finely minced into 2-5-mm fragments, suspended in P buffer (10 ml/g of wet tissue), and washed twice by centrifugation (150 g, 22°C, 8 min). The minced synovium was incubated (45 min at 37°C) with chymopapain (1 mg/ml) and pronase (0.5 mg/ml) in 1 ml Tyrode’s buffer/g synovial tissue. Remaining tissue was digested for another 45 min at 37°C with collagenase (1 mg/ml). Cell suspensions were filtered twice through 200 μ pore Nytex cloth, centrifuged (200 g, 22°C, 8 min), and washed twice with P buffer. Yields with this technique ranged from 0.2 × 106 to 1.0 × 106 mast cells/g of wet tissue. HSyMCs were purified by discontinuous Percoll gradient (de Paulis et al., 1996). The purity of these populations ranged from 16 to 35%. Mast cells were further purified using a CD117 MicroBead kit sorting system. HSyMCs purities using these techniques ranged from 71 to 94%.
Histamine Release Assay
Cells (≈ 3 × 104 mast cells per tube) were resuspended in P2CG, and 0.3 ml of the cell suspension were placed in 12 × 75 mm polyethylene tubes and warmed to 37°C; 0.2 ml of each prewarmed releasing stimulus (anti-IgE, substance P or morphine) was added, and incubation was continued at 37°C for 45 min (Patella et al., 1990). Cell were centrifuged (1000 g, 22°C, 2 min), and the supernatants were stored at −70°C for subsequent assay of histamine, tryptase, LTC4 and PGD2 content. Experiments with HSMCs were performed at 30°C as previously described (Stellato et al., 1992a). The cell-free supernatants were assayed for histamine with an automated fluorometric technique (Siraganian, 1974). The percent histamine release from mast cells was calculated as previously described (de Paulis et al., 1991; Varricchi et al., 2019). All values are based on means of duplicate determinations which differed by less than 10%.
Immunoassay of Tryptase, PGD2, and LTC4
Tryptase was analyzed by FEIA (Phadia Diagnostic AB, Uppsala, Sweden) (Stellato et al., 1992a). PGD2 and LTC4 were analyzed by radioimmunoassay (Patella et al., 1990; de Paulis et al., 1991). The anti-PGD2 and anti-LTC4 antibodies had less than 1% cross-reactivity to other eicosanoids (Patella et al., 1990; de Paulis et al., 1991). Data were normalized on total cell number.
Statistical Analysis
Values are expressed as means ± SEM. Statistical significance was assessed by using 1-way ANOVA (for data sets with Gaussian distribution) or Kruskal-Wallis test (for data sets without Gaussian distribution), followed by the Dunn multiple correction test. Results were analyzed with GraphPad Prism software (version 7.05: GraphPad Software, La Jolla, CA), and p-values of less than 0.05 were considered significant.
Results
Heterogeneous Effects of Anti-IgE on the Activation of HLMCs, HSMCs, HHMCs, and HSyMCs
As previously reported (Peachell et al., 1988; de Paulis et al., 1996; Genovese et al., 2000; Varricchi et al., 2019), exposure of mast cells isolated from different anatomic sites (lung: HLMCs; skin: HSMCs; heart: HHMCs; synovial tissue: HSyMCs) to anti-IgE (10−1 to 3 μg/ml) resulted in a dose dependent release of histamine (data not shown). The ability of mast cells isolated from different human tissues to secrete histamine when challenged with anti-IgE indicates that they have IgE bound to FcεRI. Figure 1 summarizes the release of preformed (histamine and tryptase) and de novo synthesized mediators (LTC4 and PGD2) from HLMCs, HLSMCs, HHMCs, and HSyMCs when challenged anti-IgE (3 μg/ml). All types of human mast cells examined released the same percent of histamine and tryptase (Figures 1A,B). By contrast, striking differences were found among different types of mast cells when we compared the de novo synthesis of lipid mediators. HSMCs did not produce LTC4 compared to HLMCs (p < 0.01) and to HHMCs and HSyMCs (p < 0.01). Moreover, maximal stimulation of HHMCs and HSyMCs with anti-IgE led to the LTC4 production of 20.2 ± 3.5 and 22.5 ± 4.4 ng/106 mast cells, respectively, which was significantly lower than HLMCs (51.5 ± 8.40 ng/106 cells; p < 0.05). Interestingly, the anti-IgE-mediated production of PGD2 from HLMCs (52.3 ± 6.9 ng/106 mast cells) and HSMCs (39.0 ± 10.0 ng/106 mast cells) did not differ between the two groups. However, only the production of PGD2 from HLMCs, but not HSMCs, was significantly higher than that produced by HHMCs (19.3 ± 4.5 ng/106 mast cells) and HSyMCs (21.3 ± 4.6 ng/106 mast cells) (p < 0.01). Collectively these results identify striking differences with respect to the release of different types of mediators in response to IgE-mediated stimuli among human mast cells isolated from different anatomic sites.
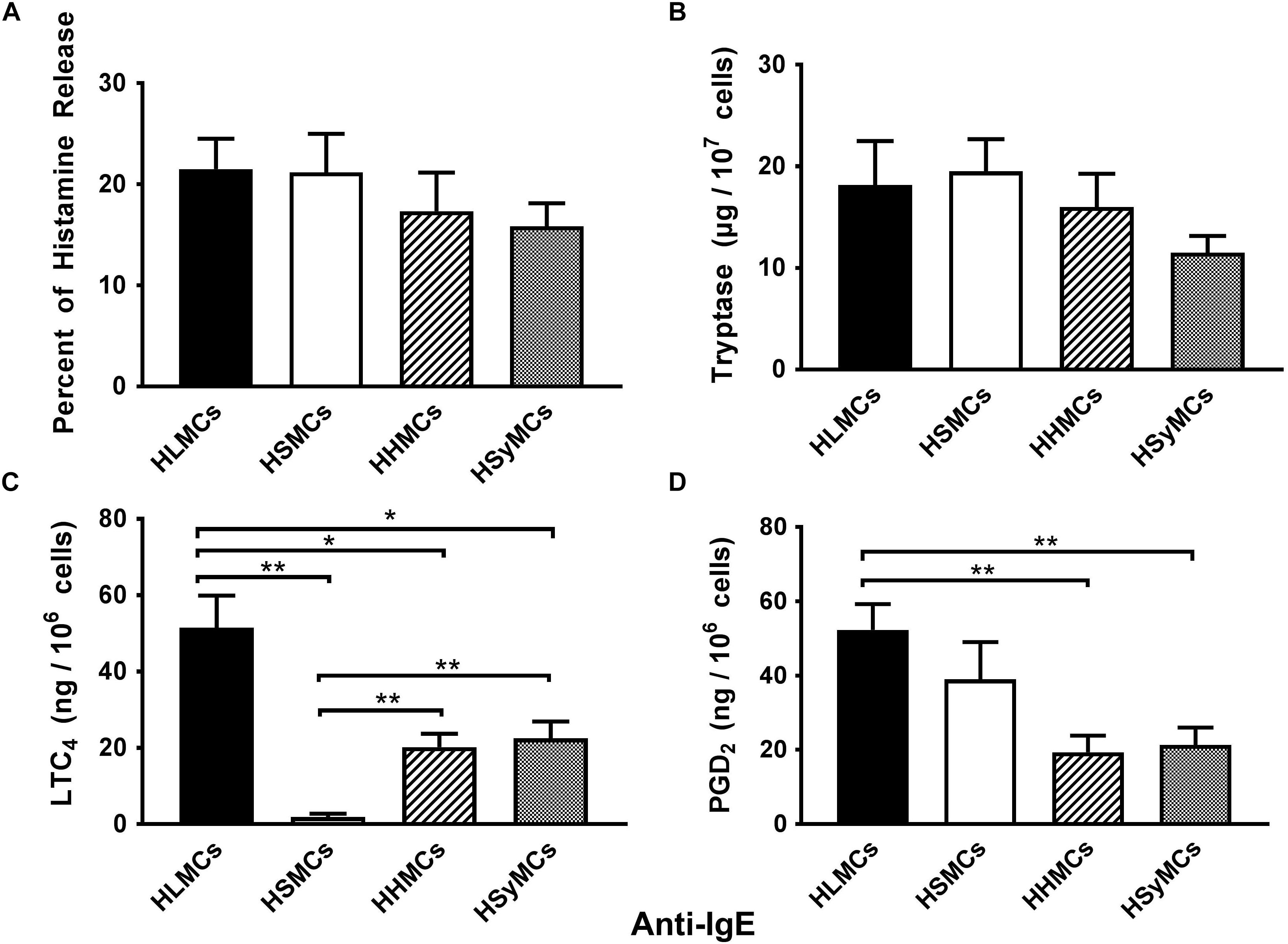
Figure 1. Effects of maximal stimulation of anti-IgE (3 μg/ml) on the release of histamine (A), tryptase (B) and the de novo synthesis of LTC4 (C), and PGD2 (D) from HLMCs (black bars), HSMCs (open bars), HHMCs (dashed bars), and HSyMCs (dot bars). Each point represents the mean ± SEM of six experiments in duplicate. Statistical significance was determined by ∗p < 0.05; ∗∗p < 0.01.
Heterogeneous Effects of Substance P on the Activation of HLMCs, HSMCs, HHMCs, and HSyMCs
Substance P (SP) has long been established as an inflammatory neuropeptide (O’Connor et al., 2004; Mashaghi et al., 2016) and potent endogenous pruritogen in mice and humans (Azimi et al., 2017; Gupta and Harvima, 2018; Yosipovitch et al., 2018). Although the classical receptor for SP is the neurokinin-1 receptor (NK-1R) (Douglas and Leeman, 2011), recent studies have demonstrated that SP activates MRGPRX2 receptor in addition to NK-1R to induce itch (Azimi et al., 2017). There is also evidence that SP can activate adventitial mast cells (Bot et al., 2010). Moreover, SP can be released into joint tissues from sensory nerve fibers (Pereira da Silva and Carmo-Fonseca, 1990; Gronblad et al., 1991) and its concentrations are increased in synovial fluid from patients with rheumatoid arthritis (Devillier et al., 1986). We therefore compared the effects of increasing concentrations (5 × 10−7 to 5 × 10−6 M) of SP on the activation of HLMCs, HSMCs, HHMCs, and HSyMCs. Figure 2 shows that SP caused concentration-dependent histamine and tryptase release from HSMCs whereas it had no effect on both HLMCs and HHMCs. SP caused histamine and tryptase release from HSyMCs only at the higher concentrations (10−6 M and 5 × 10−6 M) examined. The percent histamine release from HSyMCs caused by the latter concentrations of SP was significantly lower (p < 0.001) than that induced from HSMCs. Interestingly, in these experiments SP did not induce the metabolism of arachidonic acid through the 5-lipoxygenase pathway (LTC4) (Figure 2C) and the cyclooxygenase (PGD2) (Figure 2D) in all types of mast cell examined.
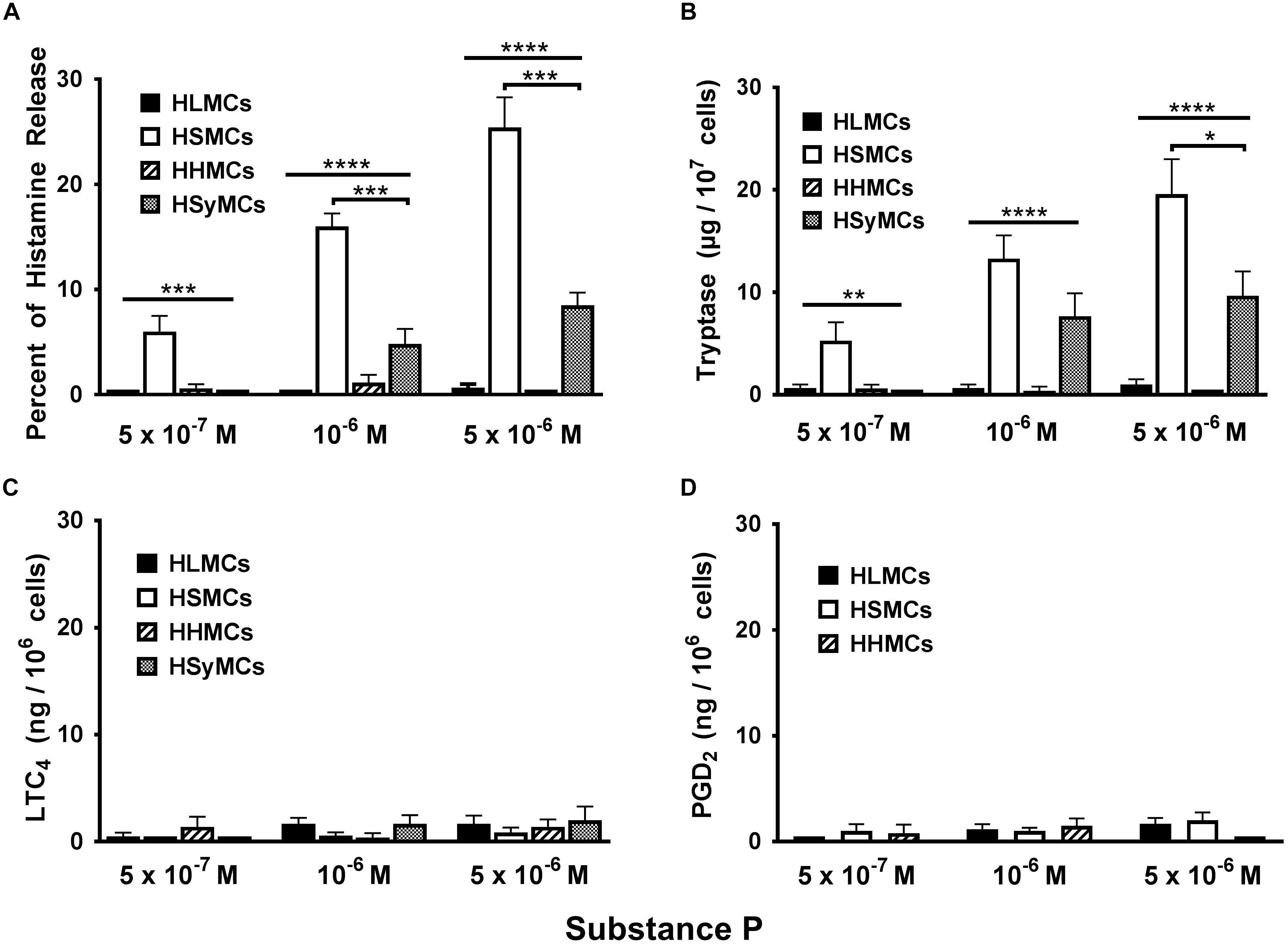
Figure 2. Effects of increasing concentrations of substance P (5 × 10−7 M to 5 × 10−6 M) on the release of histamine (A), tryptase (B), and the de novo synthesis of LTC4 (C), and PGD2 (D) from HLMCs, HSMCs, HHMCs, and HSyMCs. Each point represents the mean ± SEM of six experiments in duplicate. Statistical significance was determined by ∗p < 0.05; ∗∗p < 0.01; ∗∗∗p < 0.001; ∗∗∗∗p < 0.0001.
Heterogeneous Effects of Morphine on the Activation of HLMCs, HSMCs, HHMCs, and HSyMCs
Opioid compounds bind to multiple receptors also present on several cells of innate and adaptive immunity where they exert immunomodulatory effects (Boland and Pockley, 2018; Plein and Rittner, 2018). Recent evidence indicates that several opioid compounds including morphine can activate the human LAD2 mast cell line through MRGPRX2 (Lansu et al., 2017). Figure 3 shows the results of several experiments comparing the effects of increasing concentrations (10−5 to 3 × 10−4 M) of morphine on mediator release from primary HLMCs, HSMCs, and HHMCs. Morphine selectively induced histamine and tryptase release from HSMCs but not from HLMCs and HHMCs. Interestingly, morphine was an incomplete secretagogue because it did not induce the production of both LTC4 and PGD2 from all types of human mast cells.
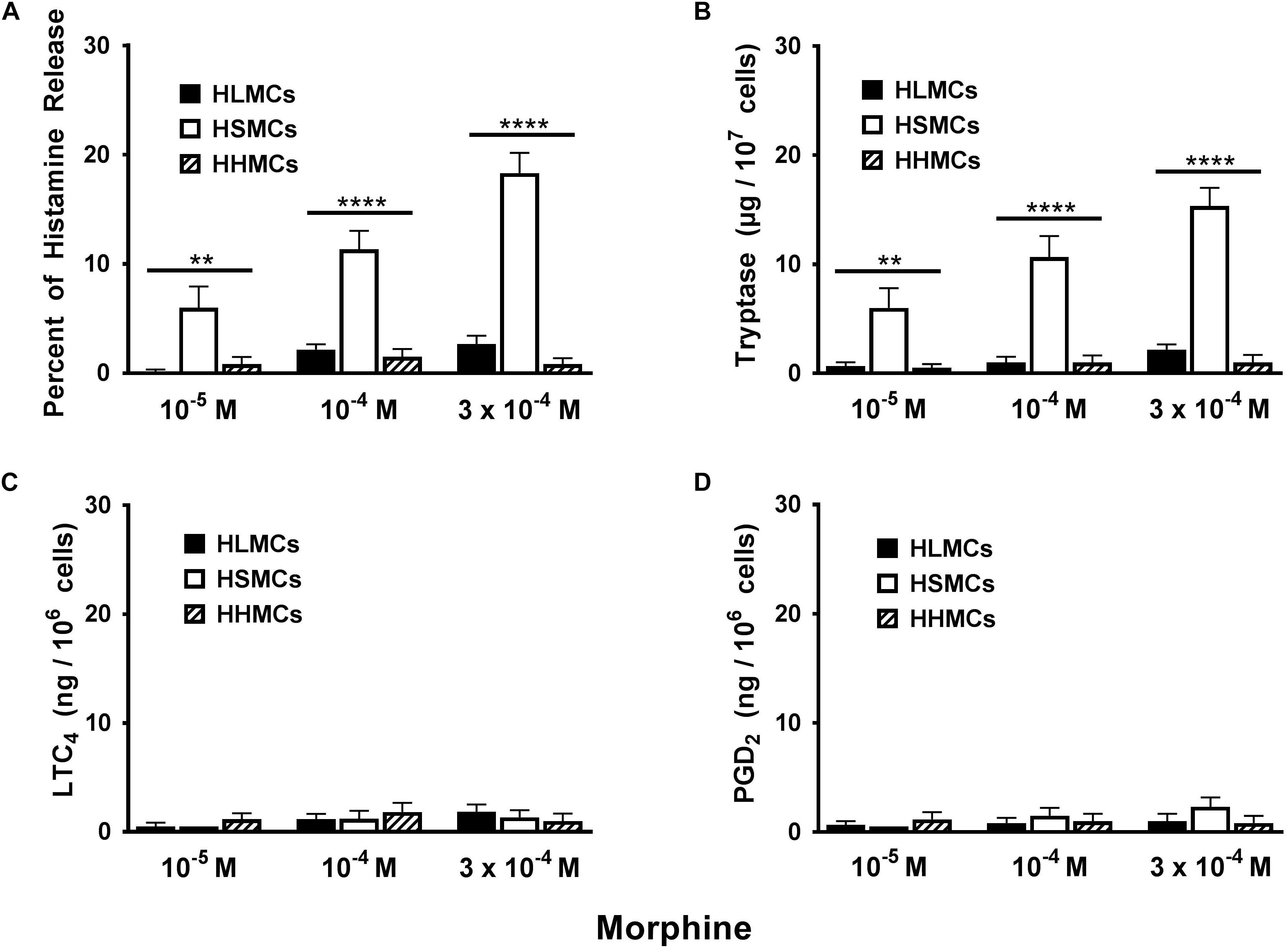
Figure 3. Effects of increasing concentrations of morphine (10−5 M to 3 × 10−4 M) on the release of histamine (A), tryptase (B) and the de novo synthesis of LTC4 (C), and PGD2 (D) from HLMCs, HSMCs and HHMCs. Each point represents the mean ± SEM of six experiments in duplicate. Statistical significance was determined by ∗∗p < 0.01; ∗∗∗∗p < 0.0001.
Discussion
The results of this study extends previous findings demonstrating the functional heterogeneity of human primary mast cells isolated from different anatomic sites with respect to FcεRI-mediated activation (Schwartz et al., 1987; Casolaro et al., 1989; Stellato et al., 1992a; Patella et al., 1998; Galli et al., 2011; Motakis et al., 2014). No differences were found with respect to anti-IgE-mediated release of preformed mediators (histamine and tryptase) from human primary mast cells purified from different anatomic sites. By contrast, striking differences were demonstrated among different types of mast cells with respect to the anti-IgE-induced de novo synthesis of LTC4 and PGD2. Interestingly, LTC4 is not produced by anti-IgE-activated HSMCs whereas these cells synthesize PGD2. Moreover, the IgE-mediated production of both LTC4 and PGD2 from HLMCs was higher than that of HHMCs and HSyMCs. Collectively, these results suggest the existence of profound differences between the biochemical mechanisms that regulate the secretion of preformed mediators and the de novo production of lipid molecules among different types of human primary mast cells.
Tatemoto and coworkers first demonstrated the presence of MRGPRX2 mRNA in human skin and in human cord blood mast cells (CBMCs) (Tatemoto et al., 2006). They also found that several basic secretagogues, including SP, induced mast cell degranulation. They suggested that MRGPRX2 receptor is highly expressed in MCTC compared to MT. Since this initial observation several groups have demonstrated that various neuropeptides (e.g., VIP), endogenous and exogenous opioids (e.g., morphine), cationic drugs (e.g., icatibant, atracurium, ciprofloxacin), and 48/80 can activate human mast cells via the MRGPRX2 receptor (McNeil et al., 2015; Gaudenzio et al., 2016; Lansu et al., 2017; Okamura et al., 2017; Alkanfari et al., 2018). Interestingly the group of Theoharides has demonstrated that SP can synergistically potentiate the production of several cytokines (e.g., TNF-α, VEGF, IL-1β) by LAD2 mast cells (Theoharides et al., 2010; Taracanova et al., 2017, 2018).
The activating property of MRGPRX2 agonists has been evaluated in human LAD2 cell line (Guhl et al., 2005; Kulka et al., 2008; Theoharides et al., 2010; McNeil et al., 2015), human peripheral blood-derived cultured mast cells (PBCMCs) (Gaudenzio et al., 2016), and human CBMCs (Tatemoto et al., 2006). In the present study performed using primary mast cell isolated and purified from different human tissues, we found that two MRGPRX2 agonists, SP and morphine, selectively induce the release of preformed mediators (histamine and tryptase) from HSMCs, but not from HLMCs and HHMCs. These findings are consistent with the observation that the MRGPRX2 receptor is expressed in HSMCs but not in lung mast cells (Fujisawa et al., 2014; Babina et al., 2018). We also found that high concentrations of SP caused small but significant release of histamine and tryptase from HSyMCs. This observation could be of some interest because Okamura et al. have demonstrated that SP activates HSyMCs to release histamine and to produce PGD2 (Lee et al., 2013) through the activation of MRGPRX2 (Okamura et al., 2017). In our study SP caused some release of preformed mediators (i.e., histamine and tryptase) from HSyMCs, but not the de novo synthesis of both PGD2 and LTC4. Several studies have suggested the involvement of SP in experimental arthritis (Levine et al., 1984; Ahmed et al., 1995; Seegers et al., 2003) and in rheumatoid arthritis (Hernanz et al., 1993; Menkes et al., 1993; Miller et al., 2000; Grimsholm et al., 2005; Dirmeier et al., 2008). Further studies are needed to clarify the SP-mediated production of proinflammatory and immunomodulatory mediators from HSyMCs.
There is increasing evidence that cardiac mast cells play a role in several myocardial disorders (Patella et al., 1990, 1996, 1998; Theoharides et al., 2011; Shi et al., 2015; Ngkelo et al., 2016; Marino et al., 2017). It has been reported that SP induces adverse myocardial remodeling (Melendez et al., 2011) and intraplaque hemorrhage in atherosclerosis (Bot et al., 2010) via the activation of mast cells. Azimi and collaborators have implicated mast cell MRGPRX2 in human and experimental cardiometabolic disorders (Azimi et al., 2017). However, the mechanism(s) of SP-mediated vascular and cardiac mast cell activation remains controversial (Shi et al., 2017). In our study SP and morphine failed to induce the release of preformed and de novo synthesized mediators from partially purified HHMCs. Interestingly, we have previously demonstrated by immunoelectron microscopy the presence of both tryptase and chymase in human cardiac mast cells (Patella et al., 1995). Thus, although HHMCs contain both serine proteases, similarly to HSMCs, they differ from the latter in response to MRGPRX2 activators. Several explanations can justify this intriguing observation: first, the possibility of the existence of MRGPRX2 variants expressed in different types of human mast cells (Alkanfari et al., 2018) cannot be excluded; second, the complex enzymatic and mechanical procedure to purify HHMCs might alter the expression and function of MRGPRX2 both at the plasma membrane and intracellular sites (Fujisawa et al., 2014). We are presently investigating the surface and intracellular localization of MRGPRX2 in HHMCs to explain the apparent lack of functional effects of SP and morphine on these cells.
Increasing evidence supports the role of mast cells in neurogenic inflammation (Skaper et al., 2014; Skaper et al., 2017) leading to itch and pain (Vincent et al., 2013; Gupta and Harvima, 2018; Yosipovitch et al., 2018). Nerve fibers release proinflammatory and vasoactive neuropeptides such as SP (Rosa and Fantozzi, 2013; Skaper et al., 2017), which can activate mast cells. These cells release algogenic and pruritogenic mediators such as tryptase and histamine (Yosipovitch et al., 2018), which activate specific nociceptors on sensory nerve fibers (Vergnolle et al., 2001; Rosa and Fantozzi, 2013). There is increasing evidence that SP is linked to itch and pain through activation of MRGPRX2 on mast cells and sensory neurons (Azimi et al., 2016, 2017). We found that SP is a potent activator of the release of both histamine and tryptase from HSMCs that highly express MRGPRX2 (Fujisawa et al., 2014). The role of tryptase is particularly relevant because this protease activates the PAR2 receptor on nerve endings (Vergnolle et al., 2001; Zhang et al., 2012) stimulating the release of SP and other neuropeptides (Steinhoff et al., 2000) that activate nociceptors on nerve terminals as well as mast cells in a paracrine manner. Moreover, in vivo administration of morphine can induce histamine release (Baldo and Pham, 2012; Kumar and Singh, 2013) and itching in humans presumably via MRGPRX2-mediated HSMC activation.
Our study has some limitations which have to be pointed out. It was performed using primary mast cells isolated from several tissues (i.e., lung, heart, synovial, skin) obtained from different patients. Moreover, these mast cells might have different characteristics from cells obtained from healthy donors. Finally, the mechanical and enzymatic procedures to isolate mast cells from different anatomic sites are quite different. We cannot exclude the possibility that the techniques used to isolate and purify mast cells from different tissues might explain, at least in part, their different response to MRGPRX2 activation.
In conclusion, the results of this study demonstrate that there is greater functional heterogeneity of primary human mast cells across tissues than previously appreciated. First, we extend previous findings demonstrating heterogeneity when different types of human mast cells are activated via aggregation of FcεRI by anti-IgE. Second, there is heterogeneity of de novo synthesized mediators produced by different human mast cells activated by IgE-cross-linking. Third, there is heterogeneity of human mast cells with respect to MRGPRX2 activation. Additional studies are needed to examine the intracellular and membrane expression of MRGPRX2 in different types of primary human mast cells.
Data Availability
The datasets for this study will not be made publicly available because Some of the data are part of a patent.
Author Contributions
GV, AG, SL, RP, GM, and GS have conceived and designed the study. GV, AP, SL, RP, FR, and GM performed the experiments. AP performed the statistical analysis of the results. AP and GM elaborated the figures. All the authors contributed intellectually and to the writing of the final version of the manuscript.
Funding
This work was supported partly by grants from the Regione Campania CISI-Lab Project, CRèME Project and TIMING Project.
Conflict of Interest Statement
The authors declare that the research was conducted in the absence of any commercial or financial relationships that could be construed as a potential conflict of interest.
Acknowledgments
GM would like to thank Dr. Lawrence M. Lichtenstein for illuminating advice in the initial studies on human skin and synovial mast cells. Special thanks to Dr. Gjada Criscuolo for critical reading of the manuscript and to the administrative staff (Dr. Roberto Bifulco and Dr. Anna Ferraro), without whom we could not function as an integrated team.
References
Ahmed, M., Bjurholm, A., Schultzberg, M., Theodorsson, E., and Kreicbergs, A. (1995). Increased levels of substance P and calcitonin gene-related peptide in rat adjuvant arthritis. A combined immunohistochemical and radioimmunoassay analysis. Arthritis Rheum. 38, 699–709. doi: 10.1002/art.1780380519
Ali, H. (2017). Emerging roles for MAS-related G protein-coupled receptor-X2 in host defense peptide, opioid, and neuropeptide-mediated inflammatory reactions. Adv. Immunol. 136, 123–162. doi: 10.1016/bs.ai.2017.06.002
Alkanfari, I., Gupta, K., Jahan, T., and Ali, H. (2018). Naturally occurring missense MRGPRX2 variants display loss of function phenotype for mast cell degranulation in response to substance P, Hemokinin-1, Human beta-Defensin-3, and Icatibant. J. Immunol. 201, 343–349. doi: 10.4049/jimmunol.1701793
Azimi, E., Reddy, V. B., Pereira, P. J. S., Talbot, S., Woolf, C. J., and Lerner, E. A. (2017). Substance P activates Mas-related G protein-coupled receptors to induce itch. J. Allergy Clin. Immunol. 140, 447.e3–453.e3. doi: 10.1016/j.jaci.2016.12.980
Azimi, E., Reddy, V. B., Shade, K. C., Anthony, R. M., Talbot, S., Pereira, P. J., et al. (2016). Dual action of neurokinin-1 antagonists on Mas-related GPCRs. JCI Insight 1:e89362. doi: 10.1172/jci.insight.89362
Babina, M., Guhl, S., Artuc, M., and Zuberbier, T. (2018). Allergic FcepsilonRI- and pseudo-allergic MRGPRX2-triggered mast cell activation routes are independent and inversely regulated by SCF. Allergy 73, 256–260. doi: 10.1111/all.13301
Baldo, B. A., and Pham, N. H. (2012). Histamine-releasing and allergenic properties of opioid analgesic drugs: resolving the two. Anaesth. Intensive Care 40, 216–235. doi: 10.1177/0310057x1204000204
Benyon, R. C., Lowman, M. A., and Church, M. K. (1987). Human skin mast cells: their dispersion, purification, and secretory characterization. J. Immunol. 138, 861–867.
Bischoff, S. C., and Dahinden, C. A. (1992). c-kit ligand: a unique potentiator of mediator release by human lung mast cells. J. Exp. Med. 175, 237–244. doi: 10.1084/jem.175.1.237
Boland, J. W., and Pockley, A. G. (2018). Influence of opioids on immune function in patients with cancer pain: from bench to bedside. Br. J. Pharmacol. 175, 2726–2736. doi: 10.1111/bph.13903
Borriello, F., Granata, F., Varricchi, G., Genovese, A., Triggiani, M., and Marone, G. (2014). Immunopharmacological modulation of mast cells. Curr. Opin. Pharmacol. 17, 45–57. doi: 10.1016/j.coph.2014.07.002
Bot, I., de Jager, S. C., Bot, M., van Heiningen, S. H., de Groot, P., Veldhuizen, R. W., et al. (2010). The neuropeptide substance P mediates adventitial mast cell activation and induces intraplaque hemorrhage in advanced atherosclerosis. Circ. Res. 106, 89–92. doi: 10.1161/CIRCRESAHA.109.204875
Bradding, P., and Arthur, G. (2016). Mast cells in asthma–state of the art. Clin. Exp. Allergy 46, 194–263. doi: 10.1111/cea.12675
Brown, M. A., and Weinberg, R. B. (2018). Mast cells and innate lymphoid cells: underappreciated players in CNS autoimmune demyelinating disease. Front. Immunol. 9:514. doi: 10.3389/fimmu.2018.00514
Canonica, G. W., Senna, G., Mitchell, P. D., O’Byrne, P. M., Passalacqua, G., and Varricchi, G. (2016). Therapeutic interventions in severe asthma. World Allergy Organ J. 9:40. doi: 10.1186/s40413-016-0130-3
Casolaro, V., Galeone, D., Giacummo, A., Sanduzzi, A., Melillo, G., and Marone, G. (1989). Human basophil/mast cell releasability. V. Functional comparisons of cells obtained from peripheral blood, lung parenchyma, and bronchoalveolar lavage in asthmatics. Am. Rev. Respir. Dis. 139, 1375–1382. doi: 10.1164/ajrccm/139.6.1375
Church, M. K., Pao, G. J., and Holgate, S. T. (1982). Characterization of histamine secretion from mechanically dispersed human lung mast cells: effects of anti-IgE, calcium ionophore A23187, compound 48/80, and basic polypeptides. J. Immunol. 129, 2116–2121.
Conti, P., Caraffa, A., Ronconi, G., Conti, C. M., Kritas, S. K., Mastrangelo, F., et al. (2018). Impact of mast cells in depression disorder: inhibitory effect of IL-37 (new frontiers). Immunol. Res. 66, 323–331. doi: 10.1007/s12026-018-9004-9
de Paulis, A., Cirillo, R., Ciccarelli, A., de Crescenzo, G., Oriente, A., and Marone, G. (1991). Characterization of the anti-inflammatory effect of FK-506 on human mast cells. J. Immunol. 147, 4278–4285.
de Paulis, A., Marino, I., Ciccarelli, A., de Crescenzo, G., Concardi, M., Verga, L., et al. (1996). Human synovial mast cells. I. Ultrastructural in situ and in vitro immunologic characterization. Arthritis Rheum. 39, 1222–1233. doi: 10.1002/art.1780390723
de Paulis, A., Stellato, C., Cirillo, R., Ciccarelli, A., Oriente, A., and Marone, G. (1992). Anti-inflammatory effect of FK-506 on human skin mast cells. J. Invest. Dermatol. 99, 723–728.
Detoraki, A., Staiano, R. I., Granata, F., Giannattasio, G., Prevete, N., de Paulis, A., et al. (2009). Vascular endothelial growth factors synthesized by human lung mast cells exert angiogenic effects. J. Allergy Clin. Immunol. 123, 1142–1149. doi: 10.1016/j.jaci.2009.01.044
Devillier, P., Regoli, D., Asseraf, A., Descours, B., Marsac, J., and Renoux, M. (1986). Histamine release and local responses of rat and human skin to substance P and other mammalian tachykinins. Pharmacology 32, 340–347. doi: 10.1159/000138190
Dirmeier, M., Capellino, S., Schubert, T., Angele, P., Anders, S., and Straub, R. H. (2008). Lower density of synovial nerve fibres positive for calcitonin gene-related peptide relative to substance P in rheumatoid arthritis but not in osteoarthritis. Rheumatology 47, 36–40. doi: 10.1093/rheumatology/kem301
Douglas, S. D., and Leeman, S. E. (2011). Neurokinin-1 receptor: functional significance in the immune system in reference to selected infections and inflammation. Ann. N. Y. Acad. Sci. 1217, 83–95. doi: 10.1111/j.1749-6632.2010.05826.x
Dwyer, D. F., Barrett, N. A., and Austen, K. F. (2016). Expression profiling of constitutive mast cells reveals a unique identity within the immune system. Nat. Immunol. 17, 878–887. doi: 10.1038/ni.3445
Enerback, L. (1966a). Mast cells in rat gastrointestinal mucosa. 1. Effects of fixation. Acta Pathol. Microbiol. Scand. 66, 289–302. doi: 10.1111/apm.1966.66.3.289
Enerback, L. (1966b). Mast cells in rat gastrointestinal mucosa. 2. Dye-binding and metachromatic properties. Acta Pathol. Microbiol. Scand. 66, 303–312. doi: 10.1111/apm.1966.66.3.303
Enerback, L. (1966c). Mast cells in rat gastrointestinal mucosa. 3. Reactivity towards compound 48/80. Acta Pathol. Microbiol. Scand. 66, 313–322. doi: 10.1111/apm.1966.66.3.313
Fujisawa, D., Kashiwakura, J., Kita, H., Kikukawa, Y., Fujitani, Y., Sasaki-Sakamoto, T., et al. (2014). Expression of Mas-related gene X2 on mast cells is upregulated in the skin of patients with severe chronic urticaria. J. Allergy Clin. Immunol. 134, 622.e9–633.e9. doi: 10.1016/j.jaci.2014.05.004
Galdiero, M. R., Varricchi, G., and Marone, G. (2016). The immune network in thyroid cancer. Oncoimmunology 5:e1168556. doi: 10.1080/2162402X.2016.1168556
Galli, S. J. (2016). The mast cell-IgE paradox: from homeostasis to anaphylaxis. Am. J. Pathol. 186, 212–224. doi: 10.1016/j.ajpath.2015.07.025
Galli, S. J., Borregaard, N., and Wynn, T. A. (2011). Phenotypic and functional plasticity of cells of innate immunity: macrophages, mast cells and neutrophils. Nat. Immunol. 12, 1035–1044. doi: 10.1038/ni.2109
Gaudenzio, N., Sibilano, R., Marichal, T., Starkl, P., Reber, L. L., Cenac, N., et al. (2016). Different activation signals induce distinct mast cell degranulation strategies. J. Clin. Invest. 126, 3981–3998. doi: 10.1172/JCI85538
Genovese, A., Bouvet, J. P., Florio, G., Lamparter-Schummert, B., Björck, L., and Marone, G. (2000). Bacterial immunoglobulin superantigen proteins A and L activate human heart mast cells by interacting with immunoglobulin E. Infect. Immun. 68, 5517–5524. doi: 10.1128/iai.68.10.5517-5524.2000
Gentek, R., Ghigo, C., Hoeffel, G., Bulle, M. J., Msallam, R., Gautier, G., et al. (2018). Hemogenic endothelial fate mapping reveals dual developmental origin of mast cells. Immunity 48, 1160.e–1171.e. doi: 10.1016/j.immuni.2018.04.025
Grimsholm, O., Rantapaa-Dahlqvist, S., and Forsgren, S. (2005). Levels of gastrin-releasing peptide and substance P in synovial fluid and serum correlate with levels of cytokines in rheumatoid arthritis. Arthritis Res. Ther. 7, R416–R426. doi: 10.1186/ar1503
Gronblad, M., Korkala, O., Konttinen, Y. T., Nederstrom, A., Hukkanen, M., Tolvanen, E., et al. (1991). Silver impregnation and immunohistochemical study of nerves in lumbar facet joint plical tissue. Spine 16, 34–38. doi: 10.1097/00007632-199101000-00006
Guhl, S., Lee, H. H., Babina, M., Henz, B. M., and Zuberbier, T. (2005). Evidence for a restricted rather than generalized stimulatory response of skin-derived human mast cells to substance P. J. Neuroimmunol. 163, 92–101. doi: 10.1016/j.jneuroim.2005.02.015
Gupta, K., and Harvima, I. T. (2018). Mast cell-neural interactions contribute to pain and itch. Immunol. Rev. 282, 168–187. doi: 10.1111/imr.12622
Haidl, I. D., and Marshall, J. S. (2015). Human mast cell activation with viruses and pathogen products. Methods Mol. Biol. 1220, 179–201. doi: 10.1007/978-1-4939-1568-2_12
Hernanz, A., De Miguel, E., Romera, N., Perez-Ayala, C., Gijon, J., and Arnalich, F. (1993). Calcitonin gene-related peptide II, substance P and vasoactive intestinal peptide in plasma and synovial fluid from patients with inflammatory joint disease. Br. J. Rheumatol. 32, 31–35. doi: 10.1093/rheumatology/32.1.31
Kulka, M., Sheen, C. H., Tancowny, B. P., Grammer, L. C., and Schleimer, R. P. (2008). Neuropeptides activate human mast cell degranulation and chemokine production. Immunology. 123, 398–410. doi: 10.1111/j.1365-2567.2007.02705.x
Kumar, K., and Singh, S. I. (2013). Neuraxial opioid-induced pruritus: an update. J. Anaesthesiol. Clin. Pharmacol. 29, 303–307. doi: 10.4103/0970-9185.117045
Lansu, K., Karpiak, J., Liu, J., Huang, X. P., McCorvy, J. D., Kroeze, W. K., et al. (2017). In silico design of novel probes for the atypical opioid receptor MRGPRX2. Nat. Chem. Biol. 13, 529–536. doi: 10.1038/nchembio.2334
Lee, H., Kashiwakura, J., Matsuda, A., Watanabe, Y., Sakamoto-Sasaki, T., Matsumoto, K., et al. (2013). Activation of human synovial mast cells from rheumatoid arthritis or osteoarthritis patients in response to aggregated IgG through Fcgamma receptor I and Fcgamma receptor II. Arthritis Rheum. 65, 109–119. doi: 10.1002/art.37741
Levine, J. D., Clark, R., Devor, M., Helms, C., Moskowitz, M. A., and Basbaum, A. I. (1984). Intraneuronal substance P contributes to the severity of experimental arthritis. Science 226, 547–549. doi: 10.1126/science.6208609
Li, Z., Liu, S., Xu, J., Zhang, X., Han, D., Liu, J., et al. (2018). Adult connective tissue-resident mast cells originate from late erythro-myeloid progenitors. Immunity 49, 640–653. doi: 10.1016/j.immuni.2018.09.023
Liccardi, G., Salzillo, A., Spadaro, G., Senna, G., Canonica, W. G., D’Amato, G., et al. (2003). Anaphylaxis caused by skin prick testing with aeroallergens: case report and evaluation of the risk in Italian allergy services. J. Allergy Clin. Immunol. 111, 1410–1412. doi: 10.1067/mai.2003.1521
Marino, A., Sakamoto, T., Robador, P. A., Tomita, K., and Levi, R. (2017). S1P receptor 1-mediated anti-renin-angiotensin system cardioprotection: pivotal role of mast cell aldehyde dehydrogenase type 2. J. Pharmacol. Exp. Ther. 362, 230–242. doi: 10.1124/jpet.117.241976
Marone, G., Spadaro, G., Liccardo, B., Rossi, F. W., D’Orio, C., and Detoraki, A. (2006). Superallergens: a new mechanism of immunologic activation of human basophils and mast cells. Inflamm. Res. 55(Suppl. 1), S25–S27. doi: 10.1007/s00011-005-0025-1
Mashaghi, A., Marmalidou, A., Tehrani, M., Grace, P. M., Pothoulakis, C., and Dana, R. (2016). Neuropeptide substance P and the immune response. Cell Mol. Life Sci. 73, 4249–4264. doi: 10.1007/s00018-016-2293-z
McNeil, B. D., Pundir, P., Meeker, S., Han, L., Undem, B. J., Kulka, M., et al. (2015). Identification of a mast-cell-specific receptor crucial for pseudo-allergic drug reactions. Nature 519, 237–241. doi: 10.1038/nature14022
Melendez, G. C., Li, J., Law, B. A., Janicki, J. S., Supowit, S. C., and Levick, S. P. (2011). Substance P induces adverse myocardial remodelling via a mechanism involving cardiac mast cells. Cardiovasc. Res. 92, 420–429. doi: 10.1093/cvr/cvr244
Menkes, C. J., Renoux, M., Laoussadi, S., Mauborgne, A., Bruxelle, J., and Cesselin, F. (1993). Substance P levels in the synovium and synovial fluid from patients with rheumatoid arthritis and osteoarthritis. J. Rheumatol. 20, 714–717.
Miller, L. E., Justen, H. P., Scholmerich, J., and Straub, R. H. (2000). The loss of sympathetic nerve fibers in the synovial tissue of patients with rheumatoid arthritis is accompanied by increased norepinephrine release from synovial macrophages. FASEB J. 14, 2097–2107. doi: 10.1096/fj.99-1082com
Motakis, E., Guhl, S., Ishizu, Y., Itoh, M., Kawaji, H., de Hoon, M., et al. (2014). Redefinition of the human mast cell transcriptome by deep-CAGE sequencing. Blood 123, e58–e67. doi: 10.1182/blood-2013-02-483792
Mukai, K., Tsai, M., Saito, H., and Galli, S. J. (2018). Mast cells as sources of cytokines, chemokines, and growth factors. Immunol. Rev. 282, 121–150. doi: 10.1111/imr.12634
Ngkelo, A., Richart, A., Kirk, J. A., Bonnin, P., Vilar, J., Lemitre, M., et al. (2016). Mast cells regulate myofilament calcium sensitization and heart function after myocardial infarction. J. Exp. Med. 213, 1353–1374. doi: 10.1084/jem.20160081
O’Connor, T. M., O’Connell, J., O’Brien, D. I., Goode, T., Bredin, C. P., and Shanahan, F. (2004). The role of substance P in inflammatory disease. J. Cell Physiol. 201, 167–180. doi: 10.1002/jcp.20061
Okamura, Y., Mishima, S., Kashiwakura, J. I., Sasaki-Sakamoto, T., Toyoshima, S., Kuroda, K., et al. (2017). The dual regulation of substance P-mediated inflammation via human synovial mast cells in rheumatoid arthritis. Allergol. Int. 66S, S9–S20. doi: 10.1016/j.alit.2017.03.002
Olivera, A., Beaven, M. A., and Metcalfe, D. D. (2018). Mast cells signal their importance in health and disease. J. Allergy Clin. Immunol. 142, 381–393. doi: 10.1016/j.jaci.2018.01.034
Patella, V., Casolaro, V., Bjorck, L., and Marone, G. (1990). Protein L. A bacterial Ig-binding protein that activates human basophils and mast cells. J. Immunol. 145, 3054–3061.
Patella, V., de Crescenzo, G., Marino, I., Genovese, A., Adt, M., Gleich, G. J., et al. (1996). Eosinophil granule proteins activate human heart mast cells. J. Immunol. 157, 1219–1225.
Patella, V., Marino, I., Arbustini, E., Lamparter-Schummert, B., Verga, L., Adt, M., et al. (1998). Stem cell factor in mast cells and increased mast cell density in idiopathic and ischemic cardiomyopathy. Circulation 97, 971–978. doi: 10.1161/01.cir.97.10.971
Patella, V., Marino, I., Lamparter, B., Arbustini, E., Adt, M., and Marone, G. (1995). Human heart mast cells. Isolation, purification, ultrastructure, and immunologic characterization. J. Immunol. 154, 2855–2865.
Peachell, P. T., Columbo, M., Kagey-Sobotka, A., Lichtenstein, L. M., and Marone, G. (1988). Adenosine potentiates mediator release from human lung mast cells. Am. Rev. Respir. Dis. 138, 1143–1151. doi: 10.1164/ajrccm/138.5.1143
Pereira da Silva, J. A., and Carmo-Fonseca, M. (1990). Peptide containing nerves in human synovium: immunohistochemical evidence for decreased innervation in rheumatoid arthritis. J. Rheumatol. 17, 1592–1599.
Piliponsky, A. M., and Romani, L. (2018). The contribution of mast cells to bacterial and fungal infection immunity. Immunol. Rev. 282, 188–197. doi: 10.1111/imr.12623
Plein, L. M., and Rittner, H. L. (2018). Opioids and the immune system - friend or foe. Br. J. Pharmacol. 175, 2717–2725. doi: 10.1111/bph.13750
Rivellese, F., Mauro, D., Nerviani, A., Pagani, S., Fossati-Jimack, L., Messemaker, T., et al. (2018). Mast cells in early rheumatoid arthritis associate with disease severity and support B cell autoantibody production. Ann. Rheum. Dis. 77, 1773–1781. doi: 10.1136/annrheumdis-2018-213418
Rosa, A. C., and Fantozzi, R. (2013). The role of histamine in neurogenic inflammation. Br. J. Pharmacol. 170, 38–45. doi: 10.1111/bph.12266
Schulman, E. S., MacGlashan, D. W. Jr., Peters, S. P., Schleimer, R. P., Newball, H. H., Lichtenstein, L. M., et al. (1982). Human lung mast cells: purification and characterization. J. Immunol. 129,2662–2667.
Schwartz, L. B., Irani, A. M., Roller, K., Castells, M. C., and Schechter, N. M. (1987). Quantitation of histamine, tryptase, and chymase in dispersed human T and TC mast cells. J. Immunol. 138, 2611–2615.
Seegers, H. C., Hood, V. C., Kidd, B. L., Cruwys, S. C., and Walsh, D. A. (2003). Enhancement of angiogenesis by endogenous substance P release and neurokinin-1 receptors during neurogenic inflammation. J. Pharmacol. Exp. Ther. 306, 8–12. doi: 10.1124/jpet.103.050013
Shi, G. P., Bot, I., and Kovanen, P. T. (2015). Mast cells in human and experimental cardiometabolic diseases. Nat. Rev. Cardiol. 12, 643–658. doi: 10.1038/nrcardio.2015.117
Shi, G. P., Bot, I., and Kovanen, P. T. (2017). Reply: the complexity of substance P-mediated mast cell activation. Nat. Rev. Cardiol. 14:124. doi: 10.1038/nrcardio.2016.213
Siraganian, R. P. (1974). An automated continuous-flow system for the extraction and fluorometric analysis of histamine. Anal. Biochem. 57, 383–394. doi: 10.1016/0003-2697(74)90093-1
Skaper, S. D., Facci, L., and Giusti, P. (2014). Mast cells, glia and neuroinflammation: partners in crime? Immunology 141, 314–327. doi: 10.1111/imm.12170
Skaper, S. D., Facci, L., Zusso, M., and Giusti, P. (2017). Neuroinflammation, mast cells, and glia: dangerous liaisons. Neuroscientist 23, 478–498. doi: 10.1177/1073858416687249
Staiano, R. I., Loffredo, S., Borriello, F., Iannotti, F. A., Piscitelli, F., Orlando, P., et al. (2016). Human lung-resident macrophages express CB1 and CB2 receptors whose activation inhibits the release of angiogenic and lymphangiogenic factors. J. Leukoc. Biol. 99, 531–540. doi: 10.1189/jlb.3HI1214-584R
Steinhoff, M., Buddenkotte, J., and Lerner, E. A. (2018). Role of mast cells and basophils in pruritus. Immunol. Rev. 282, 248–264. doi: 10.1111/imr.12635
Steinhoff, M., Vergnolle, N., Young, S. H., Tognetto, M., Amadesi, S., Ennes, H. S., et al. (2000). Agonists of proteinase-activated receptor 2 induce inflammation by a neurogenic mechanism. Nat. Med. 6, 151–158. doi: 10.1038/72247
Stellato, C., Cirillo, R., de Paulis, A., Casolaro, V., Patella, V., Mastronardi, P., et al. (1992a). Human basophil/mast cell releasability. IX. Heterogeneity of the effects of opioids on mediator release. Anesthesiology 77, 932–940. doi: 10.1097/00000542-199211000-00016
Stellato, C., de Paulis, A., Ciccarelli, A., Cirillo, R., Patella, V., Casolaro, V., et al. (1992b). Anti-inflammatory effect of cyclosporin A on human skin mast cells. J. Invest. Dermatol. 98, 800–804.
Stellato, C., de Paulis, A., Cirillo, R., Mastronardi, P., Mazzarella, B., and Marone, G. (1991). Heterogeneity of human mast cells and basophils in response to muscle relaxants. Anesthesiology 74, 1078–1086. doi: 10.1097/00000542-199106000-00016
Subramanian, H., Gupta, K., and Ali, H. (2016). Roles of Mas-related G protein-coupled receptor X2 on mast cell-mediated host defense, pseudoallergic drug reactions, and chronic inflammatory diseases. J. Allergy Clin. Immunol. 138, 700–710. doi: 10.1016/j.jaci.2016.04.051
Tainsh, K. R., and Pearce, F. L. (1992). Mast cell heterogeneity: evidence that mast cells isolated from various connective tissue locations in the rat display markedly graded phenotypes. Int. Arch. Allergy Immunol. 98, 26–34. doi: 10.1159/000236161
Taracanova, A., Alevizos, M., Karagkouni, A., Weng, Z., Norwitz, E., Conti, P., et al. (2017). SP and IL-33 together markedly enhance TNF synthesis and secretion from human mast cells mediated by the interaction of their receptors. Proc. Natl. Acad. Sci. U.S.A. 114, E4002–E4009. doi: 10.1073/pnas.1524845114
Taracanova, A., Tsilioni, I., Conti, P., Norwitz, E. R., Leeman, S. E., and Theoharides, T. C. (2018). Substance P and IL-33 administered together stimulate a marked secretion of IL-1beta from human mast cells, inhibited by methoxyluteolin. Proc. Natl. Acad. Sci. U.S.A. 115, E9381–E9390. doi: 10.1073/pnas.1810133115
Tatemoto, K., Nozaki, Y., Tsuda, R., Konno, S., Tomura, K., Furuno, M., et al. (2006). Immunoglobulin E-independent activation of mast cell is mediated by Mrg receptors. Biochem. Biophys. Res. Commun. 349, 1322–1328. doi: 10.1016/j.bbrc.2006.08.177
Theoharides, T. C., Sismanopoulos, N., Delivanis, D. A., Zhang, B., Hatziagelaki, E. E., and Kalogeromitros, D. (2011). Mast cells squeeze the heart and stretch the gird: their role in atherosclerosis and obesity. Trends Pharmacol. Sci. 32, 534–542. doi: 10.1016/j.tips.2011.05.005
Theoharides, T. C., Stewart, J. M., Panagiotidou, S., and Melamed, I. (2016). Mast cells, brain inflammation and autism. Eur. J. Pharmacol. 778, 96–102. doi: 10.1016/j.ejphar.2015.03.086
Theoharides, T. C., Zhang, B., Kempuraj, D., Tagen, M., Vasiadi, M., Angelidou, A., et al. (2010). IL-33 augments substance P-induced VEGF secretion from human mast cells and is increased in psoriatic skin. Proc. Natl. Acad. Sci. U.S.A. 107, 4448–4453. doi: 10.1073/pnas.1000803107
Varricchi, G., Galdiero, M. R., and Tocchetti, C. G. (2017). Cardiac toxicity of immune checkpoint inhibitors: cardio-oncology meets immunology. Circulation 136, 1989–1992. doi: 10.1161/CIRCULATIONAHA.117.029626
Varricchi, G., Harker, J., Borriello, F., Marone, G., Durham, S. R., and Shamji, M. H. (2016). T follicular helper (Tfh) cells in normal immune responses and in allergic disorders. Allergy 71, 1086–1094. doi: 10.1111/all.12878
Varricchi, G., Loffredo, S., Borriello, F., Pecoraro, A., Rivellese, F., Genovese, A., et al. (2019). Superantigenic activation of human cardiac mast cells. Int. J. Mol. Sci. 20:1828. doi: 10.3390/ijms20081828
Varricchi, G., Raap, U., Rivellese, F., Marone, G., and Gibbs, B. F. (2018). Human mast cells and basophils-How are they similar how are they different? Immunol. Rev. 282, 8–34. doi: 10.1111/imr.12627
Vergnolle, N., Bunnett, N. W., Sharkey, K. A., Brussee, V., Compton, S. J., Grady, E. F., et al. (2001). Proteinase-activated receptor-2 and hyperalgesia: a novel pain pathway. Nat. Med. 7, 821–826. doi: 10.1038/89945
Vincent, L., Vang, D., Nguyen, J., Gupta, M., Luk, K., Ericson, M. E., et al. (2013). Mast cell activation contributes to sickle cell pathobiology and pain in mice. Blood 122, 1853–1862. doi: 10.1182/blood-2013-04-498105
Yosipovitch, G., Rosen, J. D., and Hashimoto, T. (2018). Itch: from mechanism to (novel) therapeutic approaches. J. Allergy Clin. Immunol. 142, 1375–1390. doi: 10.1016/j.jaci.2018.09.005
Yu, X., Kasprick, A., Hartmann, K., and Petersen, F. (2018). The role of mast cells in autoimmune bullous dermatoses. Front. Immunol. 9:386. doi: 10.3389/fimmu.2018.00386
Keywords: heart, histamine, leukotriene C4, mast cells, MRGPRX2, prostaglandin D2, substance P and tryptase
Citation: Varricchi G, Pecoraro A, Loffredo S, Poto R, Rivellese F, Genovese A, Marone G and Spadaro G (2019) Heterogeneity of Human Mast Cells With Respect to MRGPRX2 Receptor Expression and Function. Front. Cell. Neurosci. 13:299. doi: 10.3389/fncel.2019.00299
Received: 12 March 2019; Accepted: 18 June 2019;
Published: 03 July 2019.
Edited by:
Rashid Giniatullin, University of Eastern Finland, FinlandReviewed by:
Elsa Fabbretti, University of Trieste, ItalyLorenzo Di Cesare Mannelli, University of Florence, Italy
Copyright © 2019 Varricchi, Pecoraro, Loffredo, Poto, Rivellese, Genovese, Marone and Spadaro. This is an open-access article distributed under the terms of the Creative Commons Attribution License (CC BY). The use, distribution or reproduction in other forums is permitted, provided the original author(s) and the copyright owner(s) are credited and that the original publication in this journal is cited, in accordance with accepted academic practice. No use, distribution or reproduction is permitted which does not comply with these terms.
*Correspondence: Gilda Varricchi, gildanet@gmail.com; orcid.org/0000-0002-9285-4657; Gianni Marone, marone@unina.it orcid.org/0000-0002-9849-4701
†orcid.org/0000-0003-3617-2819
‡orcid.org/0000-0002-5871-1898
§ orcid.org/0000-0002-4723-0167
‖orcid.org/0000-0002-6759-7521