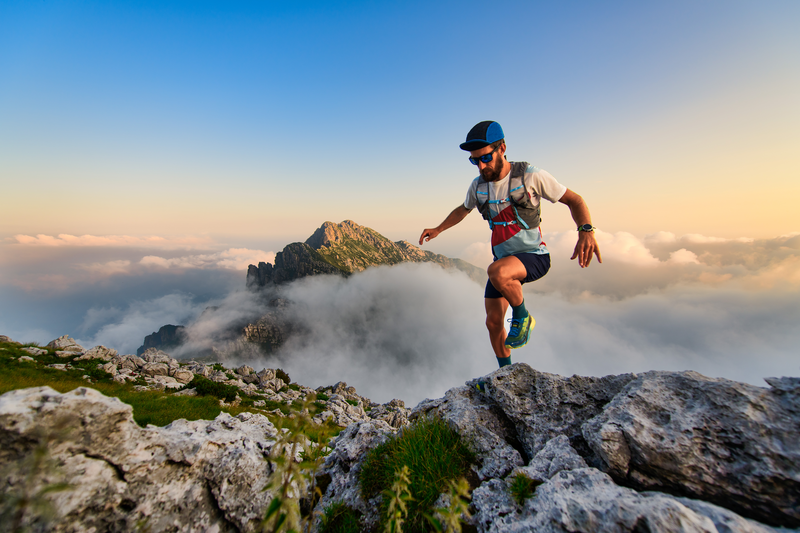
94% of researchers rate our articles as excellent or good
Learn more about the work of our research integrity team to safeguard the quality of each article we publish.
Find out more
MINI REVIEW article
Front. Cell. Neurosci. , 29 October 2018
Sec. Cellular Neuropathology
Volume 12 - 2018 | https://doi.org/10.3389/fncel.2018.00384
It has long been believed that kidney function is linked to brain activity. Clinical studies demonstrate that patients with chronic kidney disease (CKD) are more prone to cognitive impairment and Alzheimer’s disease (AD), and the degree of cognitive impairment is closely related to CKD progression and renal failure. Moreover, the fact that cognitive function in CKD patients is significantly improved after successful kidney transplantation reveals a linkage between CKD and AD. However, the mechanisms behind this linkage are unclear. The physiological function of the kidney is to maintain the stability of the internal environment, including the cerebrovascular circulation, whereas abnormal kidney function often leads to ischemia and hypoxia. Many CKD patients experience chronic hypoxia, and many urinary toxins accumulate after renal function is impaired. In this mini review, we will propose a novel perspective on the association between AD and CKD and the connection between the kidney and brain.
In traditional medicine, it is believed that the kidney is the congenital foundation of the human body, and it produces and stores an “essence.” The kidney was believed to play an important role in governing water, the vascular system, the heart, growth and the brain in ancient China, as shown in Figure 1. This means the kidney modulates the brain through the “essence” it produces. The “energy” in the brain would be insufficient if the “essence” in the kidney were lacking, resulting in cognitive function decline (Li et al., 2006). Images of the brain taken by MRI of patients with kidney essence deficiency syndrome have similar imaging patterns to the brains of AD patients (Guo et al., 2017).
FIGURE 1. The function of kidney in Traditional Chinese Medicine. The kidney store essence, and it governs brain through the essence. Besides, the kidney governs the water, vascular, heart, and growth in ancient China.
In 2012, a systematic meta-analysis of 54779 patients showed that the risk of cognitive impairment is significantly higher in patients with CKD than in patients without CKD (p < 0.001) (Etgen et al., 2012). CKD is characterized by a glomerular filtration rate (GFR) of less than 60 mL/min per 1⋅73 m2 or renal damage (structural or functional) for at least 3 months (Guo et al., 2017). Patients with CKD are prone to cognitive impairment at each phase (Bugnicourt et al., 2013). The lower the renal function in CKD patients is, the greater is the risk of cognitive impairment (Coppolino et al., 2018). Moreover, it has been found that a successful renal transplantation can significantly improve or even reverse cognitive impairment in patients with renal failure and the memory disorders that appeared during dialysis (Radiæ et al., 2011; Gupta et al., 2018). Furthermore, the improvement in cognitive function is closely related to renal function after transplantation and the stage of the patient’s CKD (Harciarek et al., 2009).
The kidney is the organ with the highest blood flow in the human body, as calculated by the blood flow per gram of organ. The high blood flow rate of the kidney far exceeds the needs of its own metabolism. In a sense, we understand that the kidney is an organ composed of large numbers of blood vessels that help maintain the stability of its internal environment. The blood flow through the kidneys is very fast, approximately 1200 mL per minute in a resting, healthy, normal adult, which is approximately 20–25% of the total cardiac output; moreover, the blood running through the kidneys is filtered and reabsorbed to excrete metabolic waste from the body and maintain the stability of the internal environment (the internal environment is the environment where the cells live directly in the body) (Sharma et al., 2016).
The oxygen consumption of the human brain is approximately 1/5 that of the whole body, and the amount of blood reaching the human brain is approximately 15% of the total cardiac output. The cerebral cortex is very sensitive to ischemia and hypoxia of the cerebral blood circulation (Attwell et al., 2010; Cipolla, 2016). Normal blood pressure levels allow the brain to obtain a sufficient cerebral blood supply. The balance of blood pressure relies on the complex regulatory mechanisms of neurohumoral systems, which involve all organs of the body, and the kidney is the hub of the blood pressure regulation system (Arora et al., 2015).
In the clinic, CKD is divided into 5 stages. Patients with CKD1 or CKD2 have mild renal injury, patients with CKD3 or CKD4 have moderate to severe renal injury, and stage CKD5 is end-stage renal disease (ESRD) or renal failure, requiring dialysis. CKD promotes the progression of AD (Etgen et al., 2012), which may be an opportunity but also a challenge for early AD diagnosis and treatment; additionally, the number of CKD patients is growing fast. For example, in 2012, the first multicenter study on CKD in China showed that the prevalence of AD was 10.8% in 50550 patients, and this rate exhibited an upward trend year by year (Zhang et al., 2012). In 2008, there were 65074 ESRD patients undergoing hemodialysis or peritoneal dialysis in China, the main factors threatening hemodialysis patients were cardiovascular and cerebrovascular diseases (i.e., stroke) (Zuo et al., 2010), and the raw annual mortality of patients undergoing maintenance hemodialysis in Beijing is gradually increasing (Cheng et al., 2012).
Alzheimer’s disease is a chronic progressive neurodegenerative disorder causing a significant cognitive deficit, and it is one of the most common types of dementia. A substantial amount of money and human resources is devoted to treating AD every year. The expenses associated with AD greatly increase the social, economic and medical burden of the disease. In 2016, there were 5.4 million AD patients who spent approximately $236 billion in the United States (Alzheimer’s Association, 2016). However, the pathogenesis of AD is still not clear, and there is no effective treatment for AD at present. However, the fact that CKD promotes the development of cognitive decline and AD results in a new field for exploring the pathogenesis of AD and sheds light on possibilities for the prevention and treatment of AD.
Vascular stiffness is defined as diminished vascular elasticity, and the duration of blood vessel expansion is extended. Vascular calcification is usually characterized by the formation of vascular stiffness, manifested as the excessive deposition of calcium on the vascular walls. Vascular stiffness and calcification are common types of vascular injuries. Vascular stiffness is observed in the progression of CKD (Mukai et al., 2018), and vascular stiffness is significantly associated with cognitive impairment (Rabkin, 2018). Interestingly, proteinuria and vascular stiffness are significantly correlated in AD patients, suggesting that vascular injury is involved in some pathological processes of AD in CKD patients (Oh et al., 2016).
As mentioned above, vascular disease is significantly more common in patients with CKD, and it is mainly caused by accumulated uremic toxin due to renal dysfunction. The European Uremic Toxin Work Group has listed more than 90 kinds of uremic toxins, such as phosphorus, aluminum, and ADMA (asymmetric dimethylarginine) (Yavuz et al., 2005). The accumulation of phosphorus promotes vascular calcification (Shanahan et al., 2011). In addition, an increase in ADMA in serum is related to vascular stiffness and cerebral blood flow in healthy people; this phenomenon is generally considered a potential early biomarker and can also be explained by impairment of the endothelium dependent on vasodilatation caused by ADMA (Aldámiz-Echevarría and Andrade, 2012). Moreover, it is also important in the regulation of cerebral blood vessels (Asif et al., 2013).
The elevation of plasma Hcy (serum homocysteine) is a strong risk factor of AD and vascular disease (Li et al., 2018; Smith et al., 2018). There is a mass accumulation of Hcy in patients with CKD, probably because the Hcy clearance ability is damaged in patients with renal dysfunction (Karmin and Siow, 2018; Ohishi et al., 2018). We know that vascular injury can be caused by elevated Hcy through endothelial dysfunction in patients with CKD (Lai and Kan, 2015), suggesting that cognitive decline and/or AD is caused by Hcy through vascular endothelial dysfunction in CKD patients.
The massive accumulation of urinary toxins can directly act on multiple organs in the body through blood flow. For example, there is a large accumulation of parathyroid hormone (PTH) in the blood when patients have renal dysfunction. PTH can pass through the blood-brain barrier, and one study found that the PTH2 receptor was widely distributed in the central nervous system (Dobolyi et al., 2010). It has also been shown that PTH is closely related to cognitive function (Lourida et al., 2015) and that cognitive function can be improved after parathyroidectomy in patients with secondary hyperparathyroidism, suggesting that renal dysfunction-induced accumulation of PTH affects cognitive function in CKD patients.
The urinary toxin aluminum can also pass through the blood-brain barrier and act on brain tissue. Aluminum is involved in metabolic processes and redox reactions in the central nervous system, and the level of aluminum in serum is a risk factor for AD (Adlard and Bush, 2018). The accumulation of aluminum after renal dysfunction can contribute to cognitive decline and AD in CKD patients.
Cognitive function abnormalities are characteristic of hemodialysis patients, and hemodialysis patients have more cerebral atrophy, partly caused by cerebral ischemia during dialysis (McIntyre and Goldsmith, 2015; Tsuruya and Yoshida, 2018). Patients with impaired renal function are more prone to cognitive impairment, which is mainly due to renal anemia (Kurella Tamura et al., 2011) and ischemic hypoxia of cells in the brain involved in the process of cognitive impairment in CKD patients.
The risks of cognitive impairment in AD patients with CKD are significantly higher than those in patients without CKD (Ito et al., 2018), not only in older CKD patients but also in young CKD patients (20–59 years old) (Hailpern et al., 2007). These risks can be explained by two factors: vascular injury and the direct neurotoxicity of uremic toxins caused by CKD. Figure 2 shows the proposed mechanism for cognitive decline in AD patients with CKD.
FIGURE 2. The mechanism about the cognitive decline or AD in CKD patients. The cognitive decline or AD can leaded by the accumulation of uremic toxins in serum through vascular injury and direct neurotoxicity in brain after renal failure, sunh as PTH, phosphorus, ADMA, etc.
There are many common characteristics between the pathogenesis of AD and that of CKD, including vascular dysfunction or degeneration (Li et al., 2011; Xue et al., 2014), aging (Baumgart et al., 2015; Kopp, 2018), hypertension (Forbes and Cooper, 2013), diabetes (Umegaki et al., 2013) and hyperlipidemia (Reitz et al., 2011; Wan et al., 2013; Ma et al., 2016). In addition, the expression level of APP in patients with kidney disease is higher, and a key protein, SorLA (sorting protein-related receptor), that regulates APP processing is simultaneously expressed in both kidney cells and neurons, and the gene’s polymorphism is related to late-onset AD (Yarbrough, 2010).
At present, diagnosis of AD is mainly determined by physicians’ subjective experience and neuropsychological tests (Robillard et al., 2018). Unfortunately, patients are already in late stages of the disease once AD clinical diagnoses are made. However, the best time to stop the progression of AD is before the onset of clinical symptoms. It is encouraging that we now understand not only that AD is related to CKD but also that both share similar pathological processes. This relationship may provide new insight into the pathogenesis of the disease since there are a few potential markers for the diagnosis of CKD and AD. For instance, we could validate whether there are any links between the biomarkers of CKD and AD and whether the biomarkers for CKD can be used for the diagnosis of AD.
The traditional pathological hallmarks in the AD brain are neuron/synapse loss and the presence of large numbers of senile plaques and neurofibrillary tangles in the cerebral cortex and hippocampus. Aβ protein is a major component of senile plaques and can be secreted directly into cerebrospinal fluid (CSF) by neurons (Blennow et al., 2010).
Interestingly, it was also found that serum Aβ levels were significantly higher in CKD patients (Kanemaru et al., 2016), possibly due to the decreased clearance of Aβ protein in the blood of CKD patients, suggesting that cognitive decline and AD related to CKD can be affected by Aβ protein in CKD patients. Many studies have reported that Aβ40/Aβ42 levels were significantly increased in the CSF of AD patients, and this parameter is very sensitive and specific (Hansson et al., 2010; Blennow and Zetterberg, 2018).
Neurofibrillary tangles are another characteristic pathological change in the AD brain, and abnormally phosphorylated tau proteins are the main constituent proteins of neurofibrillary tangles (Brici et al., 2018). An increase in tau protein/phosphorylated tau (p-tau) in the CSF is a sign of AD (Zhou et al., 2018). Moreover, studies show that changes in the tau protein can be associated with the pathology of AD after the elevation of aluminum in renal dialysis patients (CKD5) (Harrington et al., 1994).
Serum homocysteine (Hcy) is a sulfur-containing amino acid (Brustolin et al., 2010). As mentioned above, serum Hcy clearance is significantly decreased in patients with CKD and patients already prone to high Hcy in the blood (Karmin and Siow, 2018; Tabibzadeh et al., 2018). Interestingly, serum Hcy levels were found to be significantly higher in AD patients (Baumgart et al., 2015), and serum Hcy levels are a strong and independent risk factor of AD and dementia (Xue et al., 2014). Serum Hcy is involved in the pathogenesis of AD through endothelial cell dysfunction and small blood vessels by oxidative stress (Baumgart et al., 2015). Moreover, CKD is an independent risk factor for increased serum Hcy levels (Choi et al., 2014), and the elevation of Hcy caused by CKD was found to be associated with the reduction in Aβ42 in the CSF (Hansson et al., 2010). Thus, serum Hcy levels may be a novel maker of AD in patients with CKD.
Proteinuria and eGFR are common indicators for renal function (Jha et al., 2013; Conkar et al., 2018). Proteinuria is often found in patients with CKD. Under pathological conditions, the amount of protein in the urine increases, mainly due to damaged glomerular filtration barriers (endothelial cells, podocytes, glomerular basement membrane) and their increased permeability.
Glomerular filtration function is expressed by the GFR, [mL/(min 1.73 m2)], and the GFR is the amount of fluid produced by the two kidneys per unit of time. The GFR is currently calculated by the endogenous marker creatinine in the clinic. Creatinine is excreted by renal tubules and is not metabolized by the kidneys. In patients with CKD, excretion of creatinine by renal tubules is lower, the GFR is lower, and the serum concentration of creatinine is higher.
Proteinuria and eGFR are closely related to cognitive decline (Kurella Tamura et al., 2011; Etgen et al., 2012; Ito et al., 2018). One study found that the increase in ptau in the CSF and Hcy in the serum was significantly associated with a decrease in GFR in AD patients (Kanemaru et al., 2016), suggesting that there is a connection between CKD and the increase in ptau in the CSF. Additionally, the creatinine level was closely related to cognitive impairment (McAdams-DeMarco et al., 2016).
Erythropoietin (EPO) is mainly produced in the kidney (Bachmann et al., 1993); EPO promotes the formation of red blood cells and maintains normal hemoglobin concentrations. The concentrations of hemoglobin and EPO in the serum of CKD patients are significantly lower, and CKD patients are more prone to anemia and anemic hypoxia (Kutuby et al., 2015).
A large cohort study with 3591 subjects found that CKD patients were more likely to exhibit cognitive decline. However, after adjusting for hemoglobin concentration, this link became weak or disappeared (Kurella Tamura et al., 2011), suggesting that this strong correlation was mainly caused by the change in hemoglobin concentration in CKD patients. The level of hemoglobin in the serum was significantly correlated with cognitive impairment. Additionally, studies have demonstrated that EPO can have neuroprotective effects (Fan et al., 2012), the production of EPO can be stimulated by anemic hypoxia in the brain, and anemia is an independent risk factor of cognitive decline (Sousa et al., 2018).
The concentration of cystatin C is mainly determined by the GFR because the kidney is the only organ that cleanses cystatin C (Krawczeski et al., 2010). Research confirms that the gene encoding cystatin C (CST3) is a susceptible gene of late-onset AD. In addition, it has been found that cystatin C and Aβ colocalize in the amyloid deposition of brain parenchyma and cerebral vessels of AD patients (Sastre et al., 2004), and this study also found colocalization of cystatin C and APP in transfected cells and cell membranes through immunofluorescence analysis and a combination of cystatin C, full-length APP and secreted βAPP through western blots of immunoprecipitated cell lysates and culture proteins (Finckh et al., 2000).
The levels of cystatin C in the plasma of AD patients were lower than those in the age-matched control group (Chuo et al., 2007). It is unclear whether the deposition of cystatin C in the brain leads to a decrease in cystatin C in the peripheral plasma. Furthermore, if cystatin C concentrations increase in the plasma of CKD patients, it is unclear whether this would help promote binding and precipitation between cystatin C and APP in the brain. The level of cystatin C in the serum may be a potential new biomarker of AD and cognitive decline in CKD patients.
Uremic toxins have been among the most studied factors leading to uremia since 1840 when P.A. Piorry and D.I. Heritier proposed the concept of uremia (Richet, 1998). It has been found that there are more than 90 kinds of urinary toxins in uremic patients (Duranton et al., 2012), but their impact on the body is not clear. In recent years, increasing attention has been focused on small molecule toxins and intermediate-sized molecule toxins, such as PTH (Movilli et al., 2011; Neirynck et al., 2013), phosphorus, aluminum and ADMA. Some studies have found a significant increase in PTH in the blood of patients with CKD (Souberbielle et al., 2010; Lishmanov et al., 2012), and PTH is closely related to a decline in cognitive function (Lourida et al., 2015). Additionally, phosphorus and ADMA have confirmed involvement in the progress of vascular injury (van Kuijk et al., 2010; Shanahan et al., 2011). Some scholars have found that the level of aluminum is a risk factor for AD (Walton, 2013).
summary, numerous epidemiological studies demonstrate that there is a high incidence of cognitive impairment or AD-like dementia in CKD patients of all ages. This discovery highlights a new direction for the diagnosis and potential treatment of both CKD and AD. Vascular injury and the direct neurotoxicity of uremic toxins caused by renal dysfunction are the most reasonable mechanisms of the effects of CKD in AD patients. We might obtain unexpected results if we focus our attention on changes occurring in both the brain and the kidneys in further studies in the prevention and treatment of CKD and AD.
YaS and ZL contributed to drafting and revising this manuscript. YoS and HZ designed and revised this manuscript.
This study was supported in part by the grants from National Key R&D Program of China (No. 2016YFC1305500 to HZ), the National Natural Science Foundation of China (No. 61471399 to HZ, No. 61671479 to HZ, No. U1604284 to ZL, and No. 81670663 to ZL), the National Key Research and Development Program (2015BAI12B06 to HZ), and also National Institute on Aging (NIHR01AG032441-01 and RO1AG025888 to YoS).
The authors declare that the research was conducted in the absence of any commercial or financial relationships that could be construed as a potential conflict of interest.
Adlard, P. A., and Bush, A. I. (2018). Metals and Alzheimer’s disease: how far have we come in the clinic? J. Alzheimers Dis. 62, 1369–1379. doi: 10.3233/JAD-170662
Aldámiz-Echevarría, L., and Andrade, F. (2012). Asymmetric dimethylarginine, endothelial dysfunction and renal disease. Int. J. Mol. Sci. 13, 11288–11311. doi: 10.3390/ijms130911288
Alzheimer’s Association (2016). 2016 Alzheimer’s disease facts and figures. Alzheimers Dement. 12, 459–509. doi: 10.1016/j.jalz.2016.03.001
Arora, P., Golzy, M., Patel, N., Quigg, R., Carter, R. L., and Lohr, J. W. (2015). Renin-angiotensin-aldosterone system blockers in elderly adults with chronic kidney disease without diabetes mellitus or proteinuria. J. Am. Geriatr. Soc. 63, 2478–2484. doi: 10.1111/jgs.13842
Asif, M., Louis, S. R., McEvoy, M., and Mangoni, A. (2013). Asymmetric dimethylarginine: a possible link between vascular disease and dementia. Curr. Alzheimer Res. 10, 347–356. doi: 10.2174/1567205011310040001
Attwell, D., Buchan, A. M., Charpak, S., Lauritzen, M., Macvicar, B. A., and Newman, E. A. (2010). Glial and neuronal control of brain blood flow. Nature 468, 232–243. doi: 10.1038/nature09613
Bachmann, S., Le Hir, M., and Eckardt, K. U. (1993). Co-localization of erythropoietin mRNA and ecto-5’-nucleotidase immunoreactivity in peritubular cells of rat renal cortex indicates that fibroblasts produce erythropoietin. J. Histochem. Cytochem. 41, 335–341. doi: 10.1177/41.3.8429197
Baumgart, M., Snyder, H. M., Carrillo, M. C., Fazio, S., Kim, H., and Johns, H. (2015). Summary of the evidence on modifiable risk factors for cognitive decline and dementia: a population-based perspective. Alzheimers Dement. 11, 718–726. doi: 10.1016/j.jalz.2015.05.016
Blennow, K., Hampel, H., Weiner, M., and Zetterberg, H. (2010). Cerebrospinal fluid and plasma biomarkers in Alzheimer disease. Nat. Rev. Neurol. 6, 131–144. doi: 10.1038/nrneurol.2010.4
Blennow, K., and Zetterberg, H. (2018). Biomarkers for Alzheimer disease – current status and prospects for the future. J. Int. Med. doi: 10.1111/joim.12816 [Epub ahead of print].
Brici, D., Götz, J., and Nisbet, R. M. (2018). A novel antibody targeting tau phosphorylated at serine 235 detects neurofibrillary tangles. J. Alzheimers Dis. 61, 899–905. doi: 10.3233/JAD-170610
Brustolin, S., Giugliani, R., and Félix, T. M. (2010). Genetics of homocysteine metabolism and associated disorders. Braz. J. Med. Biol. Res. 43, 1–7. doi: 10.1590/S0100-879X2009007500021
Bugnicourt, J. M., Godefroy, O., Chillon, J. M., Choukroun, G., and Massy, Z. A. (2013). Cognitive disorders and dementia in CKD: the neglected kidney-brain axis. J. Am. Soc. Nephrol. 24, 353–363. doi: 10.1681/ASN.2012050536
Cheng, X., Nayyar, S., Wang, M., Li, X., Sun, Y., Huang, W., et al. (2012). Mortality rates among prevalent hemodialysis patients in Beijing: a comparison with USRDS data. Nephrol. Dial. Transplant. 28, 724–732. doi: 10.1093/ndt/gfs326
Choi, S. T., Kim, J. S., and Song, J. S. (2014). Elevated serum homocysteine levels were not correlated with serum uric acid levels, but with decreased renal function in gouty patients. J. Korean Med. Sci. 29, 788–792. doi: 10.3346/jkms.2014.29.6.788
Chuo, L. J., Sheu, W. H., Pai, M. C., and Kuo, Y. M. (2007). Genotype and plasma concentration of cystatin C in patients with late-onset Alzheimer disease. Dement. Geriatr. Cogn. Disord. 23, 251–257. doi: 10.1159/000100021
Cipolla, M. J. (2016). The cerebral circulation: colloquium series on integrated systems physiology: from molecule to function to disease. Morgan Claypool Life Sci. 8, 1–80.
Conkar, S., Mir, S., Karaslan, F. N., and Hakverdi, G. (2018). Comparing different estimated glomerular filtration rate equations in assessing glomerular function in children based on creatinine and cystatin C. J. Clin. Lab. Anal. doi: 10.1002/jcla.22413 [Epub ahead of print].
Coppolino, G., Bolignano, D., Gareri, P., Ruberto, C., Andreucci, M., Ruotolo, G., et al. (2018). Kidney function and cognitive decline in frail elderly: two faces of the same coin? Int. Urol. Nephrol. 50, 1505–1510. doi: 10.1007/s11255-018-1900-3
Dobolyi, A., Palkovits, M., and Usdin, T. B. (2010). The TIP39–PTH2 receptor system: unique peptidergic cell groups in the brainstem and their interactions with central regulatory mechanisms. Prog. Neurobiol. 90, 29–59. doi: 10.1016/j.pneurobio.2009.10.017
Duranton, F., Cohen, G., De Smet, R., Rodriguez, M., Jankowski, J., Vanholder, R., et al. (2012). Normal and pathologic concentrations of uremic toxins. J. Am. Soc. Nephrol. 23, 1258–1270. doi: 10.1681/ASN.2011121175
Etgen, T., Chonchol, M., Förstl, H., and Sander, D. (2012). Chronic kidney disease and cognitive impairment: a systematic review and meta-analysis. Am. J. Nephrol. 35, 474–482. doi: 10.1159/000338135
Fan, X., van Bel, F., van der, Kooij, M. A., et al. (2012). Hypothermia and erythropoietin for neuroprotection after neonatal brain damage. Pediatr. Res. 73, 18–23. doi: 10.1038/pr.2012.139
Finckh, U., von der Kammer, H., Velden, J., Michel, T., Andresen, B., Deng, A., et al. (2000). Genetic association of a cystatin C gene polymorphism with late-onset Alzheimer disease. Arch. Neurol. 57, 1579–1583. doi: 10.1001/archneur.57.11.1579
Forbes, J. M., and Cooper, M. E. (2013). Mechanisms of diabetic complications. Physiol. Rev. 93, 137–188. doi: 10.1152/physrev.00045.2011
Guo, Z., Liu, X., Cao, Y., Hou, H., Chen, X., Chen, Y., et al. (2017). Common 1 H-MRS characteristics in patients with Alzheimer’s disease and vascular dementia diagnosed with kidney essence deficiency syndrome: a preliminary study. Altern. Ther. Health Med. 23, 12–18.
Gupta, A., Thomas, T. S., Klein, J. A., Montgomery, R. N., Mahnken, J. D., Johnson, D. K., et al. (2018). Discrepancies between perceived and measured cognition in kidney transplant recipients: implications for clinical management. Nephron 138, 22–28. doi: 10.1159/000481182
Hailpern, S. M., Melamed, M. L., Cohen, H. W., and Hostetter, T. H. (2007). TH Moderate chronic kidney disease and cognitive function in adults 20 to 59 years of age: third national health and nutrition examination survey (NHANES III). J. Am. Soc. Nephrol. 18, 2205–2213. doi: 10.1681/ASN.2006101165
Hansson, O., Zetterberg, H., Vanmechelen, E., Vanderstichele, H., Andreasson, U., Londos, E., et al. (2010). Evaluation of plasma Aβ 40 and Aβ 42 as predictors of conversion to Alzheimer’s disease in patients with mild cognitive impairment. Neurobiol. Aging 31, 357–367. doi: 10.1016/j.neurobiolaging.2008.03.027
Harciarek, M., Biedunkiewicz, B., Lichodziejewska-Niemierkom, M., Debska-Slizień, A., and Rutkowski, B. (2009). Cognitive performance before and after kidney transplantation: a prospective controlled study of adequately dialyzed patients with end-stage renal disease. J. Int. Neuropsychol. Soc. 15, 684–694. doi: 10.1017/S1355617709990221
Harrington, C. R., Wischik, C. M., McArthur, F. K., Taylor, G. A., Edwardson, J. A., and Candy, J. M. (1994). Alzheimer’s-disease-like changes in tau protein processing: association with aluminium accumulation in brains of renal dialysis patients. Lancet 343, 993–997. doi: 10.1016/S0140-6736(94)90124-4
Ito, H., Antoku, S., Mori, T., Nakagawa, Y., Mizoguchi, K., Matsumoto, S., et al. (2018). Association between chronic kidney disease and the cognitive function in subjects without overt dementia. Clin. Nephrol. 89, 330–335. doi: 10.5414/CN109188
Jha, V., Garcia-Garcia, G., Iseki, K., Li, Z., Naicker, S., Plattner, B., et al. (2013). Chronic kidney disease: global dimension and perspectives. Lancet 382, 260–272. doi: 10.1016/S0140-6736(13)60687-X
Kanemaru, K., Kanemaru, A., and Murayama, S. (2016). Association between renal functions and csf biomarkers in alzheimer’s disease. Alzheimer’s Dement. J. Alzheimer’s Assoc. 12:665. doi: 10.1016/j.jalz.2016.06.1508
Karmin, O., and Siow, Y. L. (2018). Metabolic imbalance of homocysteine and hydrogen sulfide in kidney disease. Curr. Med. Chem. 25, 367–377. doi: 10.2174/0929867324666170509145240
Kopp, J. B. (2018). Global glomerulosclerosis in primary nephrotic syndrome: including age as a variable to predict renal outcomes. Kidney Int. 93, 1043–1044. doi: 10.1016/j.kint.2018.01.020
Krawczeski, C. D., Vandevoorde, R. G., Kathman, T., Bennett, M. R., Woo, J. G., Wang, Y., et al. (2010). Serum cystatin C is an early predictive biomarker of acute kidney injury after pediatric cardiopulmonary bypass. Clin. J. Am. Soc. Nephrol. 5, 1552–1557. doi: 10.2215/CJN.02040310
Kurella Tamura, M., Xie, D., Yaffe, K., Cohen, D. L., Teal, V., Kasner, S. E., et al. (2011). Vascular risk factors and cognitive impairment in chronic kidney disease: the Chronic Renal Insufficiency Cohort (CRIC) study. Clin. J. Am. Soc. Nephrol. 6, 248–256. doi: 10.2215/CJN.02660310
Kutuby, F., Wang, S., Desai, C., and Lerma, E. V. (2015). Anemia of chronic kidney disease. Dis. Mon. 61, 421–424. doi: 10.1016/j.disamonth.2015.08.002
Lai, W. K. C., and Kan, M. Y. (2015). Homocysteine-induced endothelial dysfunction. Ann. Nutr. Metab. 67, 1–12. doi: 10.1159/000443041
Li, J., Wang, Y. J., Zhang, M., Xu, Z. Q., Gao, C. Y., Fang, C. Q., et al. (2011). Vascular risk factors promote conversion from mild cognitive impairment to Alzheimer disease. Neurology 76, 1485–1491. doi: 10.1212/WNL.0b013e318217e7a4
Li, L., Wei, H. F., Zhang, L., Chu, J., and Zhao, L. (2006). Modern biological basis of chinese medical theory that “kidney nourishes marrow and brain is sea of marrow”. Zhongguo Zhong Yao Za Zhi 31, 1397–1400.
Li, T., Yu, B., Liu, Z., Li, J., Ma, M., Wang, Y., et al. (2018). Homocysteine directly interacts and activates the angiotensin II type I receptor to aggravate vascular injury. Nat. Commun. 9:11. doi: 10.1038/s41467-017-02401-7
Lishmanov, A., Dorairajan, S., Pak, Y., Chaudhary, K., and Chockalingam, A. (2012). Elevated serum parathyroid hormone is a cardiovascular risk factor in moderate chronic kidney disease. Int. Urol. Nephrol. 44, 541–547. doi: 10.1007/s11255-010-9897-2
Lourida, I., Thompson-Coon, J., Dickens, C. M., Soni, M., Kuźma, E., Kos, K., et al. (2015). Parathyroid hormone, cognitive function and dementia: a systematic review. PLoS One 10:e0127574. doi: 10.1371/journal.pone.0127574
Ma, C., Yin, Z., Zhu, P., Luo, J., Shi, X., and Gao, X. (2016). Blood cholesterol in late-life and cognitive decline: a longitudinal study of the chinese elderly. Mol. Neurodegener. 12:24. doi: 10.1186/s13024-017-0167-y
McAdams-DeMarco, M. A., Bae, S., Chu, N., Gross, A. L., Brown, C. H. I. V., Oh, E., et al. (2016). Dementia and Alzheimer’s disease among older kidney transplant recipients. J. Am. Soc. Nephrol. 28, 1575–1583. doi: 10.1681/ASN.2016080816
McIntyre, C. W., and Goldsmith, D. J. (2015). Ischemic brain injury in hemodialysis patients: which is more dangerous, hypertension or intradialytic hypotension? Kidney Int. 87, 1109–1115. doi: 10.1038/ki.2015.62
Movilli, E., Camerini, C., Gaggia, P., Poiatti, P., Pola, A., Viola, B. F., et al. (2011). Effect of post-dilutional on-line haemodiafiltration on serum calcium, phosphate and parathyroid hormone concentrations in uraemic patients. Nephrol. Dial. Transplant. 26, 4032–4037. doi: 10.1093/ndt/gfr179
Mukai, H., Svedberg, O., Lindholm, B., Dai, L., Heimbürger, O., and Barany, P. (2018). Skin autofluorescence, arterial stiffness and Framingham risk score as predictors of clinical outcome in chronic kidney disease patients: a cohort study. Nephrol. Dial Transplant. doi: 10.1093/ndt/gfx371 [Epub ahead of print].
Neirynck, N., Vanholder, R., Schepers, E., Eloot, S., and Pletinck, A. (2013). An update on uremic toxins. Int. Urol. Nephrol. 45, 139–150. doi: 10.1007/s11255-012-0258-1
Oh, Y. S., Kim, J. S., Park, J. W., An, J. Y., Park, S. K., Shim, Y. S., et al. (2016). Arterial stiffness and impaired renal function in patients with Alzheimer’s disease. Neurol. Sci. 37, 451–457. doi: 10.1007/s10072-015-2434-4
Ohishi, T., Fujita, T., Suzuki, D., Nishida, T., Asukai, M., and Matsuyama, Y. (2018). Serum homocysteine levels are affected by renal function during a 3-year period of minodronate therapy in female osteoporotic patients. J. Bone Miner Metab. doi: 10.1007/s00774-018-0920-5 [Epub ahead of print].
Rabkin, S. W. (2018). Is it time to utilize measurement of arterial stiffness to identify and reduce the risk of cognitive impairment? J. Clin. Hypertens. (Greenwich) 20, 31–32. doi: 10.1111/jch.13126
Radiæ, J., Ljutiæ, D., Radiæ, M., Kovaèiæ, V., Dodig-Æurkoviæ, K., and Šain, M. (2011). Kidney transplantation improves cognitive and psychomotor functions in adult hemodialysis patients. Am. J. Nephrol. 34, 399–406. doi: 10.1159/000330849
Reitz, C., Brayne, C., and Mayeux, R. (2011). Epidemiology of Alzheimer disease. Nat. Rev. Neurol. 7, 137–152. doi: 10.1038/nrneurol.2011.2
Robillard, J. M., Lai, J. A., Wu, J. M., Feng, T. L., and Hayden, S. (2018). Patient perspectives of the experience of a computerized cognitive assessment in a clinical setting. Alzheimer’s Dementia 4, 297–303. doi: 10.1016/j.trci.2018.06.003
Sastre, M., Calero, M., Pawlik, M., Mathews, P. M., Kumar, A., Danilov, V., et al. (2004). Binding of cystatin C to Alzheimer’s amyloid β inhibits in vitro amyloid fibril formation. Neurobiol. Aging 25, 1033–1043. doi: 10.1016/j.neurobiolaging.2003.11.006
Shanahan, C. M., Crouthamel, M. H., Kapustin, A., and Giachelli, C. M. (2011). Arterial calcification in chronic kidney disease: key roles for calcium and phosphate. Circ. Res. 109, 697–711. doi: 10.1161/CIRCRESAHA.110.234914
Sharma, S., Ruebner, R. L., Furth, S. L., Dodds, K. M., Rychik, J., and Goldberg, D. J. (2016). Assessment of kidney function in survivors following Fontan palliation. Congenit Heart Dis. 11, 630–636. doi: 10.1111/chd.12358
Smith, A. D., Refsum, H., Bottiglieri, T., Fenech, M., Hooshmand, B., McCaddon, A., et al. (2018). Homocysteine and dementia: an international consensus statement. J. Alzheimers Dis. 62, 561–570. doi: 10.3233/JAD-171042
Souberbielle, J. C., Roth, H., and Fouque, D. P. (2010). Parathyroid hormone measurement in CKD. Kidney Int. 77, 93–100. doi: 10.1038/ki.2009.374
Sousa, N. D. S., Menezes, T. N., Silva, N. A., Mdc, E. L., and Paiva, A. A. (2018). [prevalence of anemia and correlation between the concentration of hemoglobin and cognitive factors among the elderly]. Cien Saude Colet 23, 935–944. doi: 10.1590/1413-81232018233.09082016
Tabibzadeh, N., Mentaverri, R., Daroux, M., Mesbah, R., Delpierre, A., Paul, J. G., et al. (2018). Differential determinants of tubular phosphate reabsorption: insights on renal excretion of phosphates in kidney disease. Am. J. Nephrol. 47, 300–303. doi: 10.1159/000488864
Tsuruya, K., and Yoshida, H. (2018). Brain atrophy and cognitive impairment in chronic kidney disease. Contrib. Nephrol. 196, 27–36. doi: 10.1159/000485694
Umegaki, H., Hayashi, T., Nomura, H., Yanagawa, M., Nonogaki, Z., Nakshima, H., et al. (2013). Cognitive dysfunction: an emerging concept of a new diabetic complication in the elderly. Geriatr. Gerontol. Int. 13, 28–34. doi: 10.1111/j.1447-0594.2012.00922.x
van Kuijk, J. P., Flu, W. J., Chonchol, M., Valentijn, T. M., Verhagen, H. J., Bax, J. J., et al. (2010). Elevated preoperative phosphorus levels are an independent risk factor for cardiovascular mortality. Am. J. Nephrol. 32, 163–168. doi: 10.1159/000315856
Walton, J. R. (2013). Aluminum involvement in the progression of Alzheimer’s disease. J. Alzheimers. Dis. 35, 7–43. doi: 10.3233/JAD-121909
Wan, J., Wang, S., Haynes, K., Denburg, M. R., Shin, D. B., and Gelfand, J. M. (2013). Risk of moderate to advanced kidney disease in patients with psoriasis: population based cohort study. BMJ 347:f5961. doi: 10.1136/bmj.f5961
Xue, L., Lou, Y., Feng, X., Wang, C., Ran, Z., and Zhang, X. (2014). Prevalence of chronic kidney disease and associated factors among the Chinese population in Taian, China. BMC Nephrol. 15:205. doi: 10.1186/1471-2369-15-205
Yavuz, A., Tetta, C., Ersoy, F. F., D’intini, V., Ratanarat, R., De Cal, M., et al. (2005). Reviews: uremic toxins: a new focus on an old subject. Semin. Dial. 18, 203–211. doi: 10.1111/j.1525-139X.2005.18313.x
Zhang, L., Wang, F., Wang, L., Wang, W., Liu, B., Liu, J., et al. (2012). Prevalence of chronic kidney disease in China: a cross-sectional survey. Lancet 379, 815–822. doi: 10.1016/S0140-6736(12)60033-6
Zhou, Y., Shi, J., Chu, D., Hu, W., Guan, Z., Gong, C. X., et al. (2018). Relevance of phosphorylation and truncation of tau to the etiopathogenesis of alzheimer’s disease. Front. Aging Neurosci. 10:27. doi: 10.3389/fnagi.2018.00027
Keywords: Alzheimer’s disease, kidney function, connection, CKD, cognitive impairment
Citation: Shi Y, Liu Z, Shen Y and Zhu H (2018) A Novel Perspective Linkage Between Kidney Function and Alzheimer’s Disease. Front. Cell. Neurosci. 12:384. doi: 10.3389/fncel.2018.00384
Received: 04 July 2018; Accepted: 08 October 2018;
Published: 29 October 2018.
Edited by:
Rena Li, Roskamp Institute, United StatesReviewed by:
Zhongcong Xie, Harvard Medical School, United StatesCopyright © 2018 Shi, Liu, Shen and Zhu. This is an open-access article distributed under the terms of the Creative Commons Attribution License (CC BY). The use, distribution or reproduction in other forums is permitted, provided the original author(s) and the copyright owner(s) are credited and that the original publication in this journal is cited, in accordance with accepted academic practice. No use, distribution or reproduction is permitted which does not comply with these terms.
*Correspondence: Hanyu Zhu, a2lkbmV5MzAxQDEyNi5jb20=
†These authors have contributed equally to this work
Disclaimer: All claims expressed in this article are solely those of the authors and do not necessarily represent those of their affiliated organizations, or those of the publisher, the editors and the reviewers. Any product that may be evaluated in this article or claim that may be made by its manufacturer is not guaranteed or endorsed by the publisher.
Research integrity at Frontiers
Learn more about the work of our research integrity team to safeguard the quality of each article we publish.