- 1Key Laboratory of Arrhythmias, Ministry of Education, East Hospital, Tongji University School of Medicine, Shanghai, China
- 2Department of Anatomy and Neurobiology, Tongji University School of Medicine, Shanghai, China
- 3Mental Health Institute of the Second Xiangya Hospital, National Clinical Research Center on Mental Disorders, National Technology Institute on Mental Disorders, Key Laboratory of Psychiatry and Mental Health of Hunan Province, Central South University, Changsha, China
The central serotonin (5-HT) system is the main target of selective serotonin reuptake inhibitors (SSRIs), the first-line antidepressants widely used in current general practice. One of the prominent features of chronic SSRI treatment in rodents is the enhanced adult neurogenesis in the hippocampus, which has been proposed to contribute to antidepressant effects. Therefore, tremendous effort has been made to decipher how central 5-HT regulates adult hippocampal neurogenesis. In this paper, we review how changes in the central serotonergic system alter adult hippocampal neurogenesis. We focus on data obtained from three categories of genetically engineered mouse models: (1) mice with altered central 5-HT levels from embryonic stages, (2) mice with deletion of 5-HT receptors from embryonic stages, and (3) mice with altered central 5-HT system exclusively in adulthood. These recent findings provide unique insights to interpret the multifaceted roles of central 5-HT on adult hippocampal neurogenesis and its associated effects on depression.
Introduction
Neurons in the central nervous system are produced from neural stem/progenitor cells (NSPCs) at embryonic or early postnatal stages in a process called neurogenesis. In the adult brain, this new-neuron production machinery is limited to two regions: the subgranular zone (SGZ) of the hippocampus, which generates glutamatergic granule cells of dentate gyrus (DG), and the subventricular zone (SVZ) lining the lateral ventricles, which produces GABAergic/dopaminergic cells committed to the olfactory bulb (Vadodaria and Gage, 2014). To date, substantial progress has been made to elucidate the mechanisms underlying NSPC activity and subsequent neuronal differentiation and integration into the existing neural network. Key effectors in these different developmental steps include cell intrinsic and extrinsic factors, such as transcriptional factors, morphogens, growth factors, neurotransmitters, and network activity.
Among neurotransmitters, serotonin (5-HT) has attracted the most interest because of the enhancement of adult hippocampal neurogenesis induced by selective serotonin reuptake inhibitors (SSRIs), which might contribute to their antidepressant effects (Sahay and Hen, 2007; Vaidya et al., 2007). SSRIs primarily target the 5-HT system rather than other neurotransmitter systems. Both acute and chronic administration of SSRIs increases the synaptic (extracellular) serotonin concentration by several folds. In the majority of studies of SSRI function in adult hippocampal neurogenesis in rodents, chronic rather than acute administration of SSRIs enhances the proliferation of NSPCs, increases the survival of adult-born neurons, and accelerates the maturation of immature neurons (Malberg et al., 2000; Santarelli et al., 2003; Wang et al., 2008).
Although the higher extracellular level of 5-HT induced by chronic SSRI administration enhances adult neurogenesis, some different conclusions have been drawn from individual genetic mouse models with dysfunctional 5-HT systems. Here, we focus on conclusions from (1) mouse models with altered central 5-HT levels from embryonic stages, (2) mouse models with deletion of 5-HT receptors from embryonic stages, and (3) mouse models with an altered central 5-HT system exclusively in adulthood. We have summarized recent findings to better understand the complicated roles of the central 5-HT system in adult hippocampal neurogenesis.
Genetic Mouse Models with Altered Central 5-HT Levels from Embryonic Stages
5-HTergic neurons are differentiated from progenitor cells at embryonic day (E) 10.5–11.5, and serotonin is synthesized around E12.5 in mouse brain (Gaspar et al., 2003; Suri et al., 2015). Thus, conventional or “non-inducible” conditional genetic mouse models targeting the genes expressed in 5-HTergic neurons would lead to changes in 5-HT levels from embryonic stages.
Genetic Mouse Models with Deficiency of Central 5-HT from Embryonic Stages
In brain, tryptophan hydroxylase-2 (Tph2) is the rate-limiting enzyme in the process of central 5-HT synthesis (Zhang et al., 2004), and mouse models with loss or reduction of Tph2 function present defective 5-HT synthesis (Mosienko et al., 2015). Loss or reduction of 5-HT, however, does not affect the survival of 5-HTergic neurons in different Tph2-deficient mouse models (Gutknecht et al., 2008; Alenina et al., 2009; Jia et al., 2014), and therefore, these mice show central 5-HT deficiency rather than 5-HTergic neuronal loss. At young (42 days old) and adult (80 days old) stages, Tph2 conventional KO mice showed normal BrdU-labeled proliferating NSPCs and a baseline level of DCX-labeled immature neurons in the SGZ, but increase of adult neurogenesis induced by exercise is blocked in Tph2 KO mice (Klempin et al., 2013), indicating that exercise-induced adult neurogenesis requires an intact central 5-HT system. Our study confirmed this finding as shown by the normal basal proliferation rate of NSPCs in the DG of Tph2 conditional KO mice (Pet1-Cre; Tph2flox/flox; referred to as Tph2Pet1 CKO) before aging (Song et al., 2016b). In addition, Tph2-deficient mice do not have increased anxiety-like or depression-like behaviors (Savelieva et al., 2008; Mosienko et al., 2012; Angoa-Perez et al., 2014; Jia et al., 2014). The response to SSRIs is abolished in a subset of Tph2 KO mice (Angoa-Perez et al., 2014), thus showing that the antidepressant effects of SSRIs indeed partially depend on an intact 5-HT system, but central 5-HT deficiency is not a prerequisite in the onset of depression-like behaviors, at least in mice.
Vmat2 transports 5-HT from cytosol into synaptic vesicles (Reimer et al., 1998). By crossing Vmat2flox/flox mice with Sert-Cre mice, Vmat2sert-cre conditional KO (Sert-Cre; Vmat2flox/flox) mice can be generated, which leads to Vmat2 deletion in 5-HTergic neurons. In Sert-Cre mice, however, Cre recombinase expression is not limited in 5-HTergic neurons (Narboux-Neme et al., 2008), and this may lead to Vmat2 deletion in other neurons. Loss of Vmat2 in 5-HTergic neurons results in a decrease of 5-HT in brain, but it does not affect the survival of 5-HTergic neurons (Narboux-Neme et al., 2011). Adult Vmat2sert-cre CKO mice show normal proliferation of adult hippocampal progenitors but enhanced survival of newborn neurons (Diaz et al., 2013). Similar to Tph2-deficient mice, Vmat2sert-cre CKO mice do not show increased anxiety-like behaviors, despite the reduced level of 5-HT in the brain (Narboux-Neme et al., 2011).
Lmx1b and Pet1 are important transcriptional factors involved in the differentiation of 5-HTergic neurons. In Lmx1b conditional KO (Pet1-Cre; Lmx1bflox/flox, Lmx1bPet1 CKO) mice, almost all 5-HTergic neurons are lost, which irreversibly results in central 5-HT deficiency (Zhao et al., 2006; Dai et al., 2008). We found that Lmx1bPet1 CKO mice also show normal adult neurogenesis at young and adult age (Song et al., 2016b). Like Tph2Pet1 CKO mice, depression-like behaviors are normal at the baseline level but anxiety-like behaviors are reduced in Lmx1bPet1 CKO mice (Dai et al., 2008; Jia et al., 2014). Pet1 regulates Tph2 and Sert expression by directly binding to their promoter domains (Hendricks et al., 1999). Genes associated with 5-HTergic neurons are downregulated, and about 80% reduction of central 5-HT level is present in Pet1-deficient mice (Hendricks et al., 2003). Unlike Lmx1bPet1 CKO mice, most of 5-HTergic neurons survive in Pet1 KO mice (Krueger and Deneris, 2008). Similar to Vmat2sert-cre CKO mice, the survival rather than proliferation of adult-born hippocampal neurons is increased in the DG of Pet1 KO mice (Diaz et al., 2013). Overall, it is somewhat unexpected that these genetic mouse models with a deficiency in central 5-HT or loss of 5-HTergic neurons from embryonic stages display normal baseline level of proliferation in adult hippocampal neurogenesis at young and adult age.
Genetic Mouse Models with Elevated (Extracellular) 5-HT Levels from Embryonic Stages
Sert, the specific 5-HT transporter, is expressed in central 5-HTergic neurons and is responsible for transporting synaptic 5-HT back into 5-HTergic neuronal cytoplasm. In Sert KO mice, the extracellular 5-HT level is increased dramatically (Bengel et al., 1998; Shen et al., 2004). However, the 5-HT concentrations in some brain regions, including brain stem, forebrain, hippocampus, and striatum, are reduced significantly, which may be caused by the reduction of 5-HTergic neurons in Sert KO mice (Lira et al., 2003). A previous study showed that the proliferation of NSPCs in the DG of Sert KO mice is comparable to control mice (Schmitt et al., 2007), but a recent study reported an increased proliferation of NSPCs in Sert KO mice at adult age (Karabeg et al., 2013). In addition, more granule cells in the DG express immediate early genes (Karabeg et al., 2013), which might indicate higher activity of granule neurons in the DG of Sert KO mice.
Serotonin can be degraded by MaoA/B, whose deficiency leads to a higher 5-HT level in the brain (Chen et al., 2004). More proliferating cells in the DG are found in MaoA/B deficient mice (Singh et al., 2013). However, as MaoA/B also degrades other monoamines, dopamine and norepinephrine levels also are increased after deletion of MaoA/B (Chen et al., 2004). Therefore, the phenotype of adult hippocampal neurogenesis cannot be attributed entirely to an increase of the central 5-HT level. Table 1 provides a summary of findings about adult hippocampal neurogenesis from these genetic mouse models with altered 5-HT levels from embryonic stages.
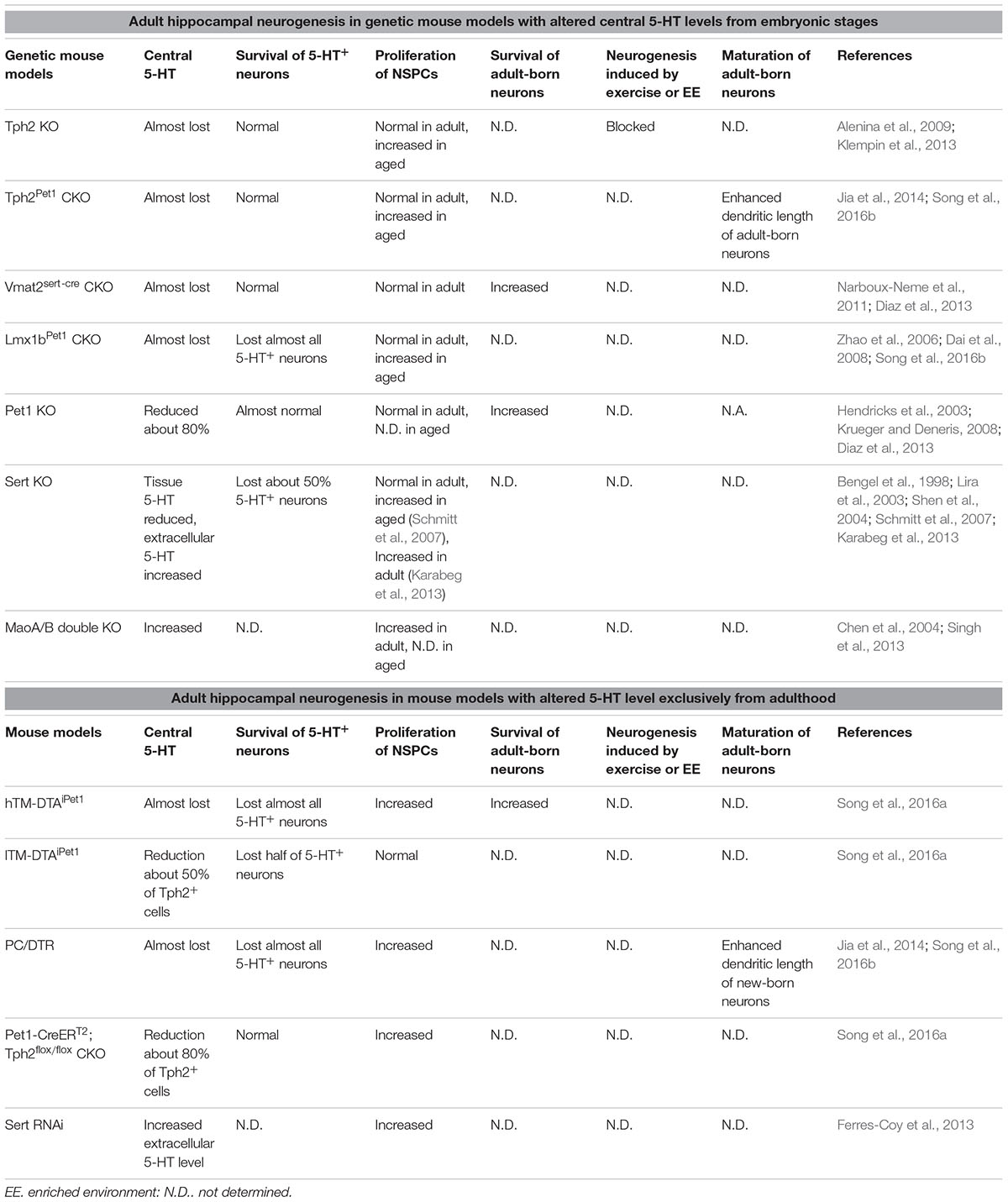
TABLE 1. Adult hippocampal neurogenesis in genetic mouse models with altered central 5-HT levels from embryonic stages or adulthood.
The decease or increase of central 5-HT levels from the embryonic stages does not simply show opposite effects on adult hippocampal neurogenesis, and most reports have demonstrated that the basal proliferation of adult hippocampal NSPCs is maintained at a normal rate in these genetic mouse models. As described in a review on this topic (Teissier et al., 2017), 5-HT is implicated in regulating brain development, including the establishment of its own axon projection map. Thus, developmental defects are present in the brain of these genetic mouse lines because of the loss of 5-HT, and they are not optimal tools to address the direct roles of central 5-HT in regulating adult neurogenesis.
Genetic Mouse Models with Deletion of 5-HT Receptors from Embryonic Stages
5-HT is released to synaptic cleft and functions after binding to its receptors. Thus far, 14 types of 5-HT receptors have been identified (Barnes and Sharp, 1999). The molecular and pharmacological features of 5-HT receptors have been reviewed elsewhere (Hoyer et al., 2002) and are not the major point of this review. How these receptors regulate adult neurogenesis is studied widely by acute or chronic administration of their agonists and antagonists. A recent review has summarized the effects of drugs on adult neurogenesis (Alenina and Klempin, 2015). Here, we focus on the data obtained from genetic mouse models of 5-HT receptors. Most of the genetic mouse models are conventional KO mice with total rather than specific deletion of 5-HT receptors.
5-HTR1A acts as both an autoreceptor and a heteroreceptor in mediating 5-HT function. 5-HTR1A inhibits firing of 5-HTergic neurons as an autoreceptor. It is highly expressed in many brain regions, including dorsal raphe nucleus and hippocampus. Most studies showed that the agonists of 5-HTR1A promote proliferation of NSPCs or survival of newborn neurons in adult hippocampal neurogenesis (Alenina and Klempin, 2015). Baseline level of adult neurogenesis is not affected in conventional 5-HTR1A KO mice, although increased proliferation of NSPCs in hippocampus induced by fluoxetine is blocked in these mice (Santarelli et al., 2003). A recent study found that higher proliferation of hippocampal NSPCs in mice housed in enriched environment is blocked in 5-HTR1A KO mice (Rogers et al., 2016). Different region-specific and time-controlled 5-HTR1A-deficient mice have been generated to investigate the function of 5-HTR1A in behaviors associated with mood and so on (Donaldson et al., 2013). More information and a precise conclusion about the function of 5-HTR1A in adult neurogenesis could be obtained from these genetic mouse models. Like 5-HTR1A, 5-HTR1B also acts as both an autoreceptor and a heteroreceptor. To date, no research has been performed to address adult neurogenesis in 5-HTR1B single KO mice. Double KOs of 5-HTR1A and 1B receptors, however, lead to decreased survival of adult-born neurons rather than proliferation of NSPCs in DG (Xia et al., 2012).
Similar to 5-HTR1A, baseline level of proliferation of NSPCs and survival of adult-born neurons is not affected in 5-HTR2B KO mice. However, the increased proliferation and survival induced by chronic fluoxetine administration is blocked in 5-HTR2B KO mice (Diaz et al., 2012). 5-HTR3 is the only ionotropic receptor in the 5-HT receptor family. The baseline level of proliferation of NSPCs and survival of adult-born neurons are not affected in 5-HTR3 KO mice; however, elevated adult neurogenesis and antidepressant-like behaviors induced by exercise is blocked in such mice (Kondo et al., 2015).
For 5-HTR4, its agonist has rapid anxiolytic–antidepressant effects (Mendez-David et al., 2014) and stimulates adult neurogenesis, but enhanced hippocampal neurogenesis is not required for these effects. In 5-HTR4 conventional KO mice, the progenitors and immature neurons are not affected at the baseline level, although the increase of these two types of cells induced by fluoxetine is blocked (Imoto et al., 2015). In this study (Imoto et al., 2015), the authors showed that 5-HTR4 is expressed in mature granule cells rather than in immature neurons in the hippocampus, which indicates an indirect involvement of 5-HTR4 in adult hippocampal neurogenesis. For 5-HTR7 receptor, the proliferation of NSPCs in the hippocampus is not changed in KO mice (Sarkisyan and Hedlund, 2009). Until now, adult neurogenesis has not been addressed in some 5-HT receptor KO mice, such as 5-HTR1D, 1E, 1F, and so on. Table 2 summarizes the adult neurogenesis in 5-HT receptor conventional KO mice.
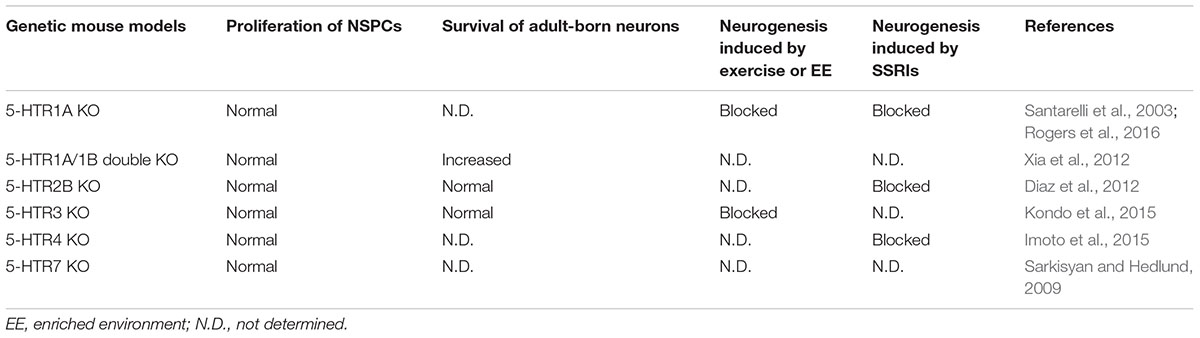
TABLE 2. Adult hippocampal neurogenesis in genetic mouse models with deletion of 5-HT receptors from embryonic stages.
5-HT receptors may have synergic effects to modulate adult neurogenesis. Additionally, in conventional KO mice with the defined deletion of a 5-HT receptor, the activity of other 5-HT receptors may compensate. Therefore, further study may need to investigate the adult hippocampal neurogenesis in multiple-gene KO mice with deletion of several receptors simultaneously.
Different receptors may play different roles in regulating adult hippocampal neurogenesis: some receptors contribute to the baseline level of adult hippocampal neurogenesis, whereas others are required for excise- or enriched environment-induced hippocampal neurogenesis.
Genetic Mouse Models with Altered 5-HT System Exclusively in Adulthood
In conventional or non-inducible KO mice, a change in the 5-HT level or 5-HT receptors begins in the embryonic stages, and as mentioned, developmental defects or possible compensations present in these mice interfere with conclusions obtained from these mice. Therefore, time-controlled inducible conditional KO mice with normally developed brains are required to address the roles of 5-HT system in adult hippocampal neurogenesis. In our recent work, time-controlled inducible genetic mouse models are generated with central 5-HT deficiency exclusively from adulthood. Three mouse models with time-controlled 5-HT deficiency have been used: (1) Pet1-Cre; Rosa26-DTR (diphtheria toxin receptor) mice obtained by crossing Pet1-Cre with Rosa26-DTR mice in which 5-HTergic neurons are depleted after diphtheria toxin (DT) is injected (Jia et al., 2014); (2) Pet1-CreERT2; Rosa26-DTA mice generated by crossing Pet1-CreERT2 with Rosa26-DTA mice in which 5-HTergic neurons would be deleted after tamoxifen is injected; and (3) Pet1-CreERT2; Tph2flox/flox CKO mice are generated by crossing Pet1-CreERT2 with Tph2flox/flox mice in which central 5-HT synthesis are blocked after tamoxifen is injected (Song et al., 2016a).
In these three mouse models, central 5-HT levels are reduced specifically after administration of DT or tamoxifen in adulthood, and these levels also can be controlled by the drug dosage (Song et al., 2016a). We found that adult hippocampal neurogenesis is enhanced as shown by increased proliferation of NSPCs and survival of newborn neurons in the DG of DT-injected Pet1-Cre; Rosa26-DTR mice (PC/DTR mice) and high-dose tamoxifen-administrated adult Pet1-CreERT2; Rosa26-DTA mice (hTM-DTAiPet1 mice) with a loss of about 95% of central 5-HTergic neurons (Jia et al., 2014; Song et al., 2016a). In contrast, the loss of half of the central 5-HTergic neurons in adult Pet1-CreERT2; Rosa26-DTA mice (lTM-DTAiPet1 mice) with a low dose of tamoxifen administration does not lead to significant alterations of adult hippocampal neurogenesis (Song et al., 2016a). In Pet1-CreERT2; Tph2flox/flox CKO mice with tamoxifen administration in adulthood, however, 5-HT level are estimated in 20% of controls, and the progenitors and immature neurons in the DG also are increased but to a lesser extent than in hTM-DTAiPet1 mice (Song et al., 2016a). Thus, lowering central 5-HT to an extremely low level also can enhance adult hippocampal neurogenesis in mice with normally developed brains. This result, in part, is consistent with a previous study demonstrating that survival rather than proliferation is enhanced in mice chronically treated with para-chlorophenylalanine (PCPA), an inhibitor of 5-HT synthesis (Diaz et al., 2013). In addition, we also found that the dendritic length of adult-born neurons is increased in DT-treated adult PC/DTR mice (Song et al., 2016b). Combined with data from administration of SSRIs, we conclude that in a normally developed brain, hippocampal neurogenesis is enhanced in two opposite conditions of central 5-HT levels, extremely low or high. It is possible that an extremely low or high central 5-HT level activates different 5-HT receptor combinations, which results in similar phenotypes of adult hippocampal neurogenesis.
Chronic administration of SSRIs, which inhibits the function of Sert and therefore augments extracellular 5-HT level, promotes adult hippocampal neurogenesis. Until now, however, there is no inducible Sert KO mouse available with Sert-deficiency exclusively in adulthood. In mice with downregulation of Sert by RNA interference in adulthood, the proliferation of NSPCs and the number of immature neurons are increased in the DG (Ferres-Coy et al., 2013). Because of the limitations of in vivo RNAi, time-controlled Sert conditional KO mice by crossing Sert-floxed mice (Chen et al., 2015) with Pet1-CreERT2 would be helpful to address the effect of higher extracellular 5-HT on adult hippocampal neurogenesis. Table 1 provides a summary of the mouse models discussed here.
Collectively, central 5-HT regulates adult neurogenesis in different ways, as shown by data from mice with dysfunctional 5-HT from embryonic stages or adulthood. The function of SSRIs on depression- and anxiety-like behaviors show age dependence (Ansorge et al., 2008; Homberg et al., 2010; Olivier et al., 2011), which may be caused by the broad and transient expression of Sert in the developing brain (Narboux-Neme et al., 2008; Homberg et al., 2010). Increased neurogenesis by SSRIs, however, is reduced or blocked in aged mouse models (Couillard-Despres et al., 2009), suggesting that aging is a key factor affecting adult hippocampal neurogenesis induced by experimental manipulations.
Late-Onset Elevated Neurogenesis in Genetic Mouse Models with Altered Central 5-HT Levels from Embryonic Stages
In Tph2 conventional KO mice, the proliferation of NSPCs in the DG is normal at young and adult age, but it is increased at aged stage (Klempin et al., 2013). Similar phenotypes are also present in Tph2 and Lmx1b conditional KO mice in which these genes have been deleted in the embryonic stage (Song et al., 2016b). Additionally, an increase in the dendritic length of newborn neurons is present in aged rather than young adult Tph2 and Lmx1b CKO mice (Song et al., 2016b). As mentioned, this late-onset phenotype of enhanced proliferation of NSPCs also is observed in Sert KO mice (Schmitt et al., 2007). It is still unclear why these mouse lines with altered 5-HT systems from the embryonic stage have normal hippocampal neurogenesis at young and adult age but show enhanced neurogenesis when aged.
Up- or downregulation of 5-HT system function does not simply cause opposite effects on adult hippocampal neurogenesis. 5-HT may function in different ways in regulating adult neurogenesis with altered 5-HT system from embryonic stages or from adulthood. Future studies are needed to explore the multifaceted roles of central 5-HT on adult hippocampal neurogenesis.
Author Contributions
N-NS, YH, XY, BL, Y-QD, and LZ wrote the paper.
Funding
This study was supported by National Natural Science Foundation of China (31100788 and 31671061 to LZ; 31030034, 81221002, 81571332 and 91232724 to Y-QD; 31528011 to BL; 81200933 to N-NS; 81101026 to YH).
Conflict of Interest Statement
The authors declare that the research was conducted in the absence of any commercial or financial relationships that could be construed as a potential conflict of interest.
References
Alenina, N., Kikic, D., Todiras, M., Mosienko, V., Qadri, F., Plehm, R., et al. (2009). Growth retardation and altered autonomic control in mice lacking brain serotonin. Proc. Natl. Acad. Sci. U.S.A. 106, 10332–10337. doi: 10.1073/pnas.0810793106
Alenina, N., and Klempin, F. (2015). The role of serotonin in adult hippocampal neurogenesis. Behav. Brain Res. 277, 49–57. doi: 10.1016/j.bbr.2014.07.038
Angoa-Perez, M., Kane, M. J., Briggs, D. I., Herrera-Mundo, N., Sykes, C. E., Francescutti, D. M., et al. (2014). Mice genetically depleted of brain serotonin do not display a depression-like behavioral phenotype. ACS Chem. Neurosci. 5, 908–919. doi: 10.1021/cn500096g
Ansorge, M. S., Morelli, E., and Gingrich, J. A. (2008). Inhibition of serotonin but not norepinephrine transport during development produces delayed, persistent perturbations of emotional behaviors in mice. J. Neurosci. 28, 199–207. doi: 10.1523/JNEUROSCI.3973-07.2008
Barnes, N. M., and Sharp, T. (1999). A review of central 5-HT receptors and their function. Neuropharmacology 38, 1083–1152. doi: 10.1016/S0028-3908(99)00010-6
Bengel, D., Murphy, D. L., Andrews, A. M., Wichems, C. H., Feltner, D., Heils, A., et al. (1998). Altered brain serotonin homeostasis and locomotor insensitivity to 3, 4-methylenedioxymethamphetamine (“Ecstasy”) in serotonin transporter-deficient mice. Mol. Pharmacol. 53, 649–655.
Chen, K., Holschneider, D. P., Wu, W., Rebrin, I., and Shih, J. C. (2004). A spontaneous point mutation produces monoamine oxidase A/B knock-out mice with greatly elevated monoamines and anxiety-like behavior. J. Biol. Chem. 279, 39645–39652. doi: 10.1074/jbc.M405550200
Chen, X., Ye, R., Gargus, J. J., Blakely, R. D., Dobrenis, K., and Sze, J. Y. (2015). Disruption of transient serotonin accumulation by non-serotonin-producing neurons impairs cortical map development. Cell Rep. doi: 10.1016/j.celrep.2014.12.033 [Epub ahead of print].
Couillard-Despres, S., Wuertinger, C., Kandasamy, M., Caioni, M., Stadler, K., Aigner, R., et al. (2009). Ageing abolishes the effects of fluoxetine on neurogenesis. Mol. Psychiatry 14, 856–864. doi: 10.1038/mp.2008.147
Dai, J. X., Han, H. L., Tian, M., Cao, J., Xiu, J. B., Song, N. N., et al. (2008). Enhanced contextual fear memory in central serotonin-deficient mice. Proc. Natl. Acad. Sci. U.S.A. 105, 11981–11986. doi: 10.1073/pnas.0801329105
Diaz, S. L., Doly, S., Narboux-Neme, N., Fernandez, S., Mazot, P., Banas, S. M., et al. (2012). 5-HT(2B) receptors are required for serotonin-selective antidepressant actions. Mol. Psychiatry 17, 154–163. doi: 10.1038/mp.2011.159
Diaz, S. L., Narboux-Neme, N., Trowbridge, S., Scotto-Lomassese, S., Kleine Borgmann, F. B., Jessberger, S., et al. (2013). Paradoxical increase in survival of newborn neurons in the dentate gyrus of mice with constitutive depletion of serotonin. Eur. J. Neurosci. 38, 2650–2658. doi: 10.1111/ejn.12297
Donaldson, Z. R., Nautiyal, K. M., Ahmari, S. E., and Hen, R. (2013). Genetic approaches for understanding the role of serotonin receptors in mood and behavior. Curr. Opin. Neurobiol. 23, 399–406. doi: 10.1016/j.conb.2013.01.011
Ferres-Coy, A., Pilar-Cuellar, F., Vidal, R., Paz, V., Masana, M., Cortes, R., et al. (2013). RNAi-mediated serotonin transporter suppression rapidly increases serotonergic neurotransmission and hippocampal neurogenesis. Transl. Psychiatry 3, e211. doi: 10.1038/tp.2012.135
Gaspar, P., Cases, O., and Maroteaux, L. (2003). The developmental role of serotonin: news from mouse molecular genetics. Nat. Rev. Neurosci. 4, 1002–1012. doi: 10.1038/nrn1256
Gutknecht, L., Waider, J., Kraft, S., Kriegebaum, C., Holtmann, B., Reif, A., et al. (2008). Deficiency of brain 5-HT synthesis but serotonergic neuron formation in Tph2 knockout mice. J. Neural Transm. 115, 1127–1132. doi: 10.1007/s00702-008-0096-6
Hendricks, T., Francis, N., Fyodorov, D., and Deneris, E. S. (1999). The ETS domain factor Pet-1 is an early and precise marker of central serotonin neurons and interacts with a conserved element in serotonergic genes. J. Neurosci. 19, 10348–10356.
Hendricks, T. J., Fyodorov, D. V., Wegman, L. J., Lelutiu, N. B., Pehek, E. A., Yamamoto, B., et al. (2003). Pet-1 ETS gene plays a critical role in 5-HT neuron development and is required for normal anxiety-like and aggressive behavior. Neuron 37, 233–247. doi: 10.1016/S0896-6273(02)01167-4
Homberg, J. R., Schubert, D., and Gaspar, P. (2010). New perspectives on the neurodevelopmental effects of SSRIs. Trends Pharmacol. Sci. 31, 60–65. doi: 10.1016/j.tips.2009.11.003
Hoyer, D., Hannon, J. P., and Martin, G. R. (2002). Molecular, pharmacological and functional diversity of 5-HT receptors. Pharmacol. Biochem. Behav. 71, 533–554. doi: 10.1016/S0091-3057(01)00746-8
Imoto, Y., Kira, T., Sukeno, M., Nishitani, N., Nagayasu, K., Nakagawa, T., et al. (2015). Role of the 5-HT4 receptor in chronic fluoxetine treatment-induced neurogenic activity and granule cell dematuration in the dentate gyrus. Mol. Brain 8, 29. doi: 10.1186/s13041-015-0120-3
Jia, Y. F., Song, N. N., Mao, R. R., Li, J. N., Zhang, Q., Huang, Y., et al. (2014). Abnormal anxiety- and depression-like behaviors in mice lacking both central serotonergic neurons and pancreatic islet cells. Front. Behav. Neurosci. 8:325. doi: 10.3389/fnbeh.2014.00325
Karabeg, M. M., Grauthoff, S., Kollert, S. Y., Weidner, M., Heiming, R. S., Jansen, F., et al. (2013). 5-HTT deficiency affects neuroplasticity and increases stress sensitivity resulting in altered spatial learning performance in the Morris water maze but not in the Barnes maze. PLoS ONE 8:e78238. doi: 10.1371/journal.pone.0078238
Klempin, F., Beis, D., Mosienko, V., Kempermann, G., Bader, M., and Alenina, N. (2013). Serotonin is required for exercise-induced adult hippocampal neurogenesis. J. Neurosci. 33, 8270–8275. doi: 10.1523/JNEUROSCI.5855-12.2013
Kondo, M., Nakamura, Y., Ishida, Y., and Shimada, S. (2015). The 5-HT3 receptor is essential for exercise-induced hippocampal neurogenesis and antidepressant effects. Mol. Psychiatry 20, 1428–1437. doi: 10.1038/mp.2014.153
Krueger, K. C., and Deneris, E. S. (2008). Serotonergic transcription of human FEV reveals direct GATA factor interactions and fate of Pet-1-deficient serotonin neuron precursors. J. Neurosci. 28, 12748–12758. doi: 10.1523/JNEUROSCI.4349-08.2008
Lira, A., Zhou, M., Castanon, N., Ansorge, M. S., Gordon, J. A., Francis, J. H., et al. (2003). Altered depression-related behaviors and functional changes in the dorsal raphe nucleus of serotonin transporter-deficient mice. Biol. Psychiatry 54, 960–971. doi: 10.1016/S0006-3223(03)00696-6
Malberg, J. E., Eisch, A. J., Nestler, E. J., and Duman, R. S. (2000). Chronic antidepressant treatment increases neurogenesis in adult rat hippocampus. J. Neurosci. 20, 9104–9110.
Mendez-David, I., David, D. J., Darcet, F., Wu, M. V., Kerdine-Romer, S., Gardier, A. M., et al. (2014). Rapid anxiolytic effects of a 5-HT(4) receptor agonist are mediated by a neurogenesis-independent mechanism. Neuropsychopharmacology 39, 1366–1378. doi: 10.1038/npp.2013.332
Mosienko, V., Beis, D., Pasqualetti, M., Waider, J., Matthes, S., Qadri, F., et al. (2015). Life without brain serotonin: reevaluation of serotonin function with mice deficient in brain serotonin synthesis. Behav. Brain Res. 277, 78–88. doi: 10.1016/j.bbr.2014.06.005
Mosienko, V., Bert, B., Beis, D., Matthes, S., Fink, H., Bader, M., et al. (2012). Exaggerated aggression and decreased anxiety in mice deficient in brain serotonin. Transl. Psychiatry 2, e122. doi: 10.1038/tp.2012.44
Narboux-Neme, N., Pavone, L. M., Avallone, L., Zhuang, X., and Gaspar, P. (2008). Serotonin transporter transgenic (SERTcre) mouse line reveals developmental targets of serotonin specific reuptake inhibitors (SSRIs). Neuropharmacology 55, 994–1005. doi: 10.1016/j.neuropharm.2008.08.020
Narboux-Neme, N., Sagne, C., Doly, S., Diaz, S. L., Martin, C. B., Angenard, G., et al. (2011). Severe serotonin depletion after conditional deletion of the vesicular monoamine transporter 2 gene in serotonin neurons: neural and behavioral consequences. Neuropsychopharmacology 36, 2538–2550. doi: 10.1038/npp.2011.142
Olivier, J. D., Blom, T., Arentsen, T., and Homberg, J. R. (2011). The age-dependent effects of selective serotonin reuptake inhibitors in humans and rodents: a review. Prog. Neuropsychopharmacol. Biol. Psychiatry 35, 1400–1408. doi: 10.1016/j.pnpbp.2010.09.013
Reimer, R. J., Fon, E. A., and Edwards, R. H. (1998). Vesicular neurotransmitter transport and the presynaptic regulation of quantal size. Curr. Opin. Neurobiol. 8, 405–412.
Rogers, J., Vo, U., Buret, L. S., Pang, T. Y., Meiklejohn, H., Zeleznikow-Johnston, A., et al. (2016). Dissociating the therapeutic effects of environmental enrichment and exercise in a mouse model of anxiety with cognitive impairment. Transl. Psychiatry 6, e794. doi: 10.1038/tp.2016.52
Sahay, A., and Hen, R. (2007). Adult hippocampal neurogenesis in depression. Nat. Neurosci. 10, 1110–1115. doi: 10.1038/nn1969
Santarelli, L., Saxe, M., Gross, C., Surget, A., Battaglia, F., Dulawa, S., et al. (2003). Requirement of hippocampal neurogenesis for the behavioral effects of antidepressants. Science 301, 805–809. doi: 10.1126/science.1083328
Sarkisyan, G., and Hedlund, P. B. (2009). The 5-HT7 receptor is involved in allocentric spatial memory information processing. Behav. Brain Res. 202, 26–31. doi: 10.1016/j.bbr.2009.03.011
Savelieva, K. V., Zhao, S., Pogorelov, V. M., Rajan, I., Yang, Q., Cullinan, E., et al. (2008). Genetic disruption of both tryptophan hydroxylase genes dramatically reduces serotonin and affects behavior in models sensitive to antidepressants. PLoS ONE 3:e3301. doi: 10.1371/journal.pone.0003301
Schmitt, A., Benninghoff, J., Moessner, R., Rizzi, M., Paizanis, E., Doenitz, C., et al. (2007). Adult neurogenesis in serotonin transporter deficient mice. J. Neural Transm. 114, 1107–1119. doi: 10.1007/s00702-007-0724-6
Shen, H. W., Hagino, Y., Kobayashi, H., Shinohara-Tanaka, K., Ikeda, K., Yamamoto, H., et al. (2004). Regional differences in extracellular dopamine and serotonin assessed by in vivo microdialysis in mice lacking dopamine and/or serotonin transporters. Neuropsychopharmacology 29, 1790–1799. doi: 10.1038/sj.npp.1300476
Singh, C., Bortolato, M., Bali, N., Godar, S. C., Scott, A. L., Chen, K., et al. (2013). Cognitive abnormalities and hippocampal alterations in monoamine oxidase A and B knockout mice. Proc. Natl. Acad. Sci. U.S.A. 110, 12816–12821. doi: 10.1073/pnas.1308037110
Song, N. N., Jia, Y. F., Zhang, L., Zhang, Q., Huang, Y., Liu, X. Z., et al. (2016a). Reducing central serotonin in adulthood promotes hippocampal neurogenesis. Sci. Rep. 6:20338. doi: 10.1038/srep20338
Song, N. N., Zhang, Q., Huang, Y., Chen, L., Ding, Y. Q., and Zhang, L. (2016b). Enhanced dendritic morphogenesis of adult hippocampal newborn neurons in central 5-HT-deficient mice. Stem Cell Res. 19, 6–11. doi: 10.1016/j.scr.2016.12.018
Suri, D., Teixeira, C. M., Cagliostro, M. K., Mahadevia, D., and Ansorge, M. S. (2015). Monoamine-sensitive developmental periods impacting adult emotional and cognitive behaviors. Neuropsychopharmacology 40, 88–112. doi: 10.1038/npp.2014.231
Teissier, A., Soiza-Reilly, M., and Gaspar, P. (2017). Refining the role of 5-HT in postnatal development of brain circuits. Front. Cell. Neurosci. 11:139. doi: 10.3389/fncel.2017.00139
Vadodaria, K. C., and Gage, F. H. (2014). SnapShot: adult hippocampal neurogenesis. Cell 156, 1114–1114.e1. doi: 10.1016/j.cell.2014.02.029
Vaidya, V. A., Vadodaria, K. C., and Jha, S. (2007). Neurotransmitter regulation of adult neurogenesis: putative therapeutic targets. CNS Neurol. Disord. Drug Targets 6, 358–374. doi: 10.2174/187152707783220910
Wang, J. W., David, D. J., Monckton, J. E., Battaglia, F., and Hen, R. (2008). Chronic fluoxetine stimulates maturation and synaptic plasticity of adult-born hippocampal granule cells. J. Neurosci. 28, 1374–1384. doi: 10.1523/JNEUROSCI.3632-07.2008
Xia, L., Delomenie, C., David, I., Rainer, Q., Marouard, M., Delacroix, H., et al. (2012). Ventral hippocampal molecular pathways and impaired neurogenesis associated with 5-HT(1)A and 5-HT(1)B receptors disruption in mice. Neurosci. Lett. 521, 20–25. doi: 10.1016/j.neulet.2012.05.046
Zhang, X., Beaulieu, J. M., Sotnikova, T. D., Gainetdinov, R. R., and Caron, M. G. (2004). Tryptophan hydroxylase-2 controls brain serotonin synthesis. Science 305, 217. doi: 10.1126/science.1097540
Zhao, Z. Q., Scott, M., Chiechio, S., Wang, J. S., Renner, K. J., Gereau, R. W., et al. (2006). Lmx1b is required for maintenance of central serotonergic neurons and mice lacking central serotonergic system exhibit normal locomotor activity. J. Neurosci. 26, 12781–12788. doi: 10.1523/JNEUROSCI.4143-06.2006
Keywords: adult hippocampal neurogenesis, 5-HT, 5-HT receptors, conventional knockout mouse, conditional knockout mouse
Citation: Song N -N, Huang Y, Yu X, Lang B, Ding Y -Q and Zhang L (2017) Divergent Roles of Central Serotonin in Adult Hippocampal Neurogenesis. Front. Cell. Neurosci. 11:185. doi: 10.3389/fncel.2017.00185
Received: 30 March 2017; Accepted: 16 June 2017;
Published: 30 June 2017.
Edited by:
Hansen Wang, University of Toronto, CanadaReviewed by:
Krishna Vadodaria, Salk Institute for Biological Studies, United StatesTing-Ting Huang, Stanford University, United States
Copyright © 2017 Song, Huang, Yu, Lang, Ding and Zhang. This is an open-access article distributed under the terms of the Creative Commons Attribution License (CC BY). The use, distribution or reproduction in other forums is permitted, provided the original author(s) or licensor are credited and that the original publication in this journal is cited, in accordance with accepted academic practice. No use, distribution or reproduction is permitted which does not comply with these terms.
*Correspondence: Lei Zhang, bGVpemhhbmcxMTIwQG91dGxvb2suY29t Yu-Qiang Ding, ZGluZ3l1cWlhbmdAdmlwLjE2My5jb20=