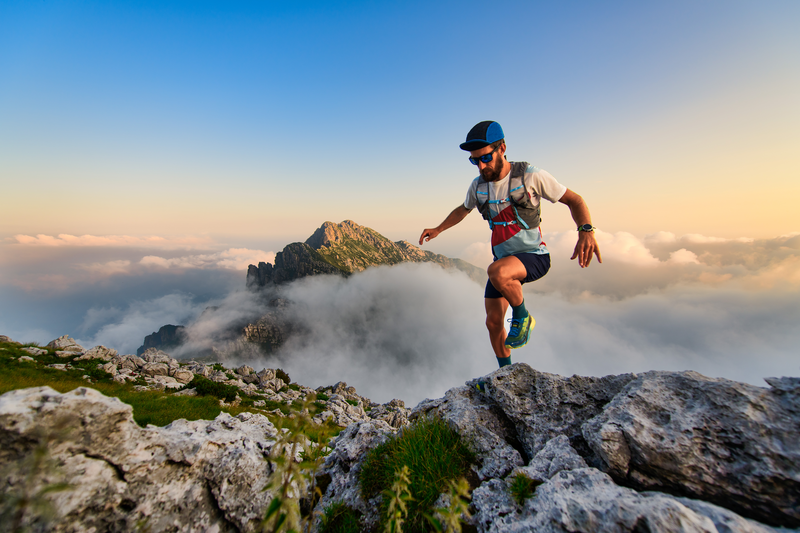
94% of researchers rate our articles as excellent or good
Learn more about the work of our research integrity team to safeguard the quality of each article we publish.
Find out more
ORIGINAL RESEARCH article
Front. Cell. Infect. Microbiol.
Sec. Clinical Microbiology
Volume 15 - 2025 | doi: 10.3389/fcimb.2025.1575824
This article is part of the Research Topic Perspectives in Clinical Microbiology for Combating Multi-drug Resistant Bacterial Infections: 2024/2025 View all 6 articles
The final, formatted version of the article will be published soon.
You have multiple emails registered with Frontiers:
Please enter your email address:
If you already have an account, please login
You don't have a Frontiers account ? You can register here
Background: Comparative studies of the bile microbiota in different biliary obstructive infections remain limited. This study aims to characterize bile microbiota and investigate differences in microbial profiles across various biliary obstructive diseases.Methods: This study included patients with biliary obstructive diseases admitted to Jinling Hospital and Suqian First Hospital. The cohort consisted of individuals with benign biliary disorders, malignant biliary obstruction, and biliary obstruction secondary to severe acute pancreatitis (SAP) or intestinal fistulas. A total of 133 bile samples were collected from 118 patients and analyzed using next-generation sequencing (NGS) targeting the bacterial 16S rRNA gene and the fungal internal transcribed spacer (ITS) gene. Clinical data, including routine culture results, were extracted from electronic medical records. Results: NGS targeting the 16S rRNA and ITS revealed a positive rate of 68.42% for bile samples, which was higher than the culture positivity rate of 60.15%, indicating a significant difference (Chi-square test, p < 0.05). The predominant bacteria identified in the bile samples through NGS were Klebsiella pneumoniae, Acinetobacter baumannii, and Escherichia coli. Bacterial species varied among benign biliary diseases, malignant obstructive diseases, and biliary obstruction caused by SAP or intestinal fistulas. Fungi were detected in 7.52% (10/133) of the samples, with 4 samples obtained from patients with biliary obstructive diseases due to SAP. Microbial diversity and clustering analysis showed no significant differences among various biliary disorders. Based on the culture results, the sensitivity and specificity of NGS were 81.82% and 69.64%, respectively.Conclusion: The composition of bile microbes may be related to the etiology of biliary obstruction. Klebsiella pneumoniae, Acinetobacter baumannii, and Escherichia coli are the predominant bacteria found in bile. NGS can be effectively applied for the identification and characterization of bile microbes associated with various biliary obstruction diseases.
Keywords: Biliary obstructive diseases, bile microbiome, 16S rRNA, ITS, Next-generation sequencing, Infection
Received: 13 Feb 2025; Accepted: 12 Mar 2025.
Copyright: © 2025 Wang, Wang, Cao, Chen, Yu and Sun. This is an open-access article distributed under the terms of the Creative Commons Attribution License (CC BY). The use, distribution or reproduction in other forums is permitted, provided the original author(s) or licensor are credited and that the original publication in this journal is cited, in accordance with accepted academic practice. No use, distribution or reproduction is permitted which does not comply with these terms.
* Correspondence:
Ning Sun, Jinling Hospital, Affiliated Hospital of Medical School, Nanjing University, Nanjing, China, Nanjing, China
Disclaimer: All claims expressed in this article are solely those of the authors and do not necessarily represent those of their affiliated organizations, or those of the publisher, the editors and the reviewers. Any product that may be evaluated in this article or claim that may be made by its manufacturer is not guaranteed or endorsed by the publisher.
Research integrity at Frontiers
Learn more about the work of our research integrity team to safeguard the quality of each article we publish.