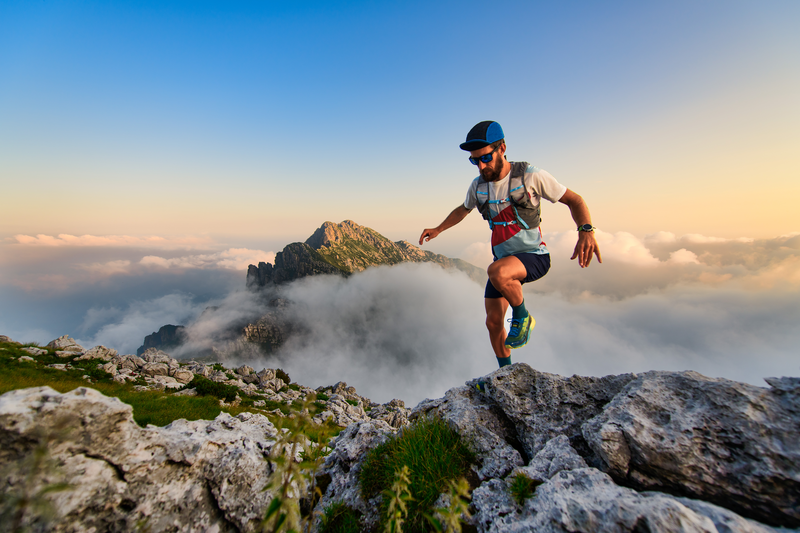
94% of researchers rate our articles as excellent or good
Learn more about the work of our research integrity team to safeguard the quality of each article we publish.
Find out more
ORIGINAL RESEARCH article
Front. Cell. Infect. Microbiol.
Sec. Antibiotic Resistance and New Antimicrobial drugs
Volume 15 - 2025 | doi: 10.3389/fcimb.2025.1565980
This article is part of the Research Topic Deciphering Antimicrobial Resistance: Genetic Insights and Perspectives View all articles
The final, formatted version of the article will be published soon.
You have multiple emails registered with Frontiers:
Please enter your email address:
If you already have an account, please login
You don't have a Frontiers account ? You can register here
Objectives: To characterize the genomes of two rare ST592 Klebsiella pneumoniae isolates and explore their evolution to carbapenem-resistant hypervirulent K. pneumoniae.Methods: Minimum inhibitory concentrations (MICs) were determined using a VITEK 2 compact system. Conjugation experiments were conducted using film matings. Whole genome sequencing (WGS) was performed using Illumina and Nanopore platforms. Antimicrobial resistance determinants were identified using the ABRicate program in the ResFinder database. Insertion sequences were identified using ISFinder. Bacterial virulence factors were identified using virulence factor database (VFDB). The K and O loci were tested using Kleborate. Multilocus sequence typing (MLST) and replicon types were identified by the Center for Genomic Epidemiology. Conjugation-related elements were predicted using oriTfinder. The plasmid structure was visualized using Circos, and a possible evolutionary model was constructed using Biorender.Results: Isolates KPZM6 and KPZM16 were identified as ST592 and KL57, respectively, and were collected from the same department. Antimicrobial susceptibility testing data revealed that KPZM16 possessed an extensively drug-resistant (XDR) profile, whereas KPZM6 was a susceptible K. pneumoniae. The hybrid assembly showed that both KPZM6 and KPZM16 had one pLVPK-like virulence plasmid carrying the rmpA, rmpA2, and iucABCD-iutA gene clusters. However, strain KPZM16 harbored one IncN plasmid carrying the carbapenem resistance genes blaNDM-1, dfrA14, and qnrS1. The results of conjugation experiments demonstrated that the plasmid could be transferred to the recipient strain. It is possible that the NDM-1-producing plasmid was transferred from KPZM6 to KPZM16 via conjugation, leading to the formation of CR-hvKP.Conclusions: This is the first study in which the complete genomic characteristics of the rare NDM-producing ST592 K. pneumoniae clinical isolate was performed. Our study provides a possible evolutionary hypothesis for the of hv-CRKP formation via conjugation. Early detection is recommended to avoid the extensive spread of this clone.
Keywords: WGS, ST592, NDM-1, evolution, CR-hvKp
Received: 24 Jan 2025; Accepted: 06 Mar 2025.
Copyright: © 2025 Zhang, Dong, Mao, Fang and Ying. This is an open-access article distributed under the terms of the Creative Commons Attribution License (CC BY). The use, distribution or reproduction in other forums is permitted, provided the original author(s) or licensor are credited and that the original publication in this journal is cited, in accordance with accepted academic practice. No use, distribution or reproduction is permitted which does not comply with these terms.
* Correspondence:
Junjie Ying, Quzhou City People's Hospital, Quzhou, China
Disclaimer: All claims expressed in this article are solely those of the authors and do not necessarily represent those of their affiliated organizations, or those of the publisher, the editors and the reviewers. Any product that may be evaluated in this article or claim that may be made by its manufacturer is not guaranteed or endorsed by the publisher.
Research integrity at Frontiers
Learn more about the work of our research integrity team to safeguard the quality of each article we publish.