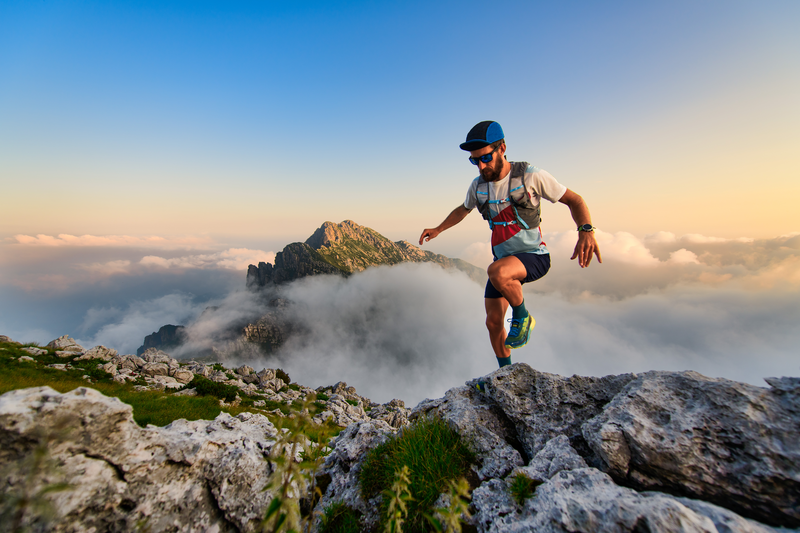
94% of researchers rate our articles as excellent or good
Learn more about the work of our research integrity team to safeguard the quality of each article we publish.
Find out more
REVIEW article
Front. Cell. Infect. Microbiol.
Sec. Intestinal Microbiome
Volume 15 - 2025 | doi: 10.3389/fcimb.2025.1565082
This article is part of the Research Topic Emerging Mechanisms of Host-Pathogen Interactions and immune responses View all 3 articles
The final, formatted version of the article will be published soon.
You have multiple emails registered with Frontiers:
Please enter your email address:
If you already have an account, please login
You don't have a Frontiers account ? You can register here
Ulcerative colitis (UC) is a chronic, non-specific inflammatory bowel disease characterized by inflammation and injury of the colonic mucosa, exhibiting an increasing global incidence. Although research into UC pathogenesis is ongoing, the precise mechanisms remain to be fully elucidated. Studies indicate that UC development results from a complex interplay of factors, including genetic predisposition, environmental exposures, gut microbial dysbiosis, and immune dysregulation. Specifically, UC pathogenesis involves aberrant immune responses triggered by interactions between the host and gut microbiota. A complex, dynamic relationship exists between the microbial community and the host immune system throughout UC pathogenesis. Accumulating evidence suggests that changes in microbiota composition significantly impact gut immunity. This review will examine the intricate balance between the gut microbiota and mucosal immunity in UC progression and discuss potential therapeutic applications, providing a reference for further clinical treatment of this patient population.
Keywords: Gut Microbiota, fecal microbiota transplantation, Inflammatory diseases, intestinal immunity, Herb medicine
Received: 22 Jan 2025; Accepted: 17 Mar 2025.
Copyright: © 2025 Bu, Chen, Jiang and Chen. This is an open-access article distributed under the terms of the Creative Commons Attribution License (CC BY). The use, distribution or reproduction in other forums is permitted, provided the original author(s) or licensor are credited and that the original publication in this journal is cited, in accordance with accepted academic practice. No use, distribution or reproduction is permitted which does not comply with these terms.
* Correspondence:
Fan Bu, Zhejiang Provincial People's Hospital, Hangzhou, 310014, Zhejiang Province, China
Disclaimer: All claims expressed in this article are solely those of the authors and do not necessarily represent those of their affiliated organizations, or those of the publisher, the editors and the reviewers. Any product that may be evaluated in this article or claim that may be made by its manufacturer is not guaranteed or endorsed by the publisher.
Research integrity at Frontiers
Learn more about the work of our research integrity team to safeguard the quality of each article we publish.