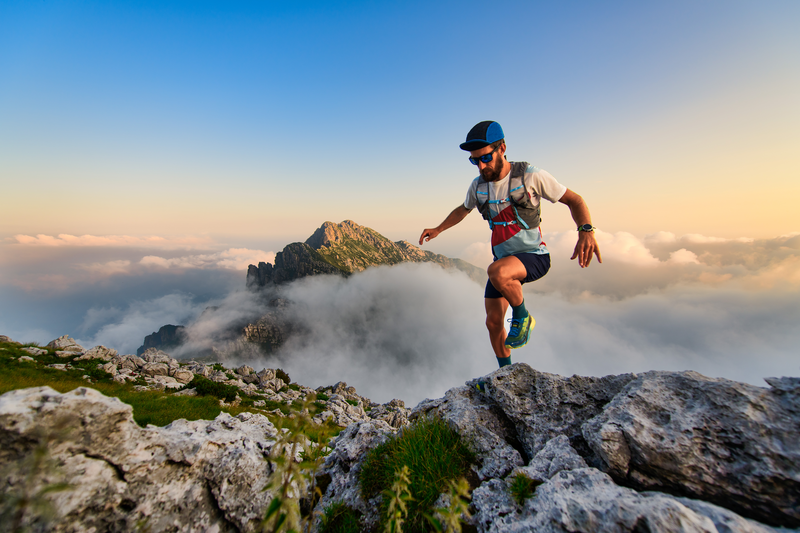
94% of researchers rate our articles as excellent or good
Learn more about the work of our research integrity team to safeguard the quality of each article we publish.
Find out more
ORIGINAL RESEARCH article
Front. Cell. Infect. Microbiol.
Sec. Biofilms
Volume 15 - 2025 | doi: 10.3389/fcimb.2025.1562402
The final, formatted version of the article will be published soon.
You have multiple emails registered with Frontiers:
Please enter your email address:
If you already have an account, please login
You don't have a Frontiers account ? You can register here
The World Health Organization has identified multi-drug resistant Klebsiella pneumoniae strains as the highest priority in 2024. Understanding the regulatory routes of virulence features is crucial for the development of novel anti-virulence strategies. SdiA, a LuxR-like quorum sensing (QS) receptor that responds to N-acyl-homoserine lactones (AHLs), is involved in the regulation of virulence traits in some Gram-negative bacteria. The function of this receptor in the virulence of K. pneumoniae remains uncertain. The objective of the present study was to elucidate the function of SdiA in K. pneumoniae biofilm formation and virulence. To this end, a genetic knockout of sdiA was conducted, and virulence-related phenotypic studies were performed following AHL provision. The results demonstrate that sdiA deficiency increases susceptibility to phage infection and human serum resistance, and promotes biofilm maturation and cell filamentation, although no effect on virulence was observed in vivo in the Galleria mellonella infection model. On the other hand, C6-HSL promoted sdiA-dependent biofilm maturation, capsule production and serum resistance while reducing virulence against G. mellonella in the absence of sdiA. The addition of C6-HSL did not affect phage susceptibility. The results of this study demonstrate that AHLs and SdiA exert a dual influence on virulence phenotypes, operating both independently and hierarchically. These findings provide new insights into the virulence of K. pneumoniae and its regulation by SdiA.
Keywords: Quorum Sensing, Biofilm, AHL (N-acyl-homoserine lactone), serum resistance, Bacteriophage, Galleria mellonella
Received: 17 Jan 2025; Accepted: 25 Feb 2025.
Copyright: © 2025 Silva-Bea, Maseda, Otero and Romero. This is an open-access article distributed under the terms of the Creative Commons Attribution License (CC BY). The use, distribution or reproduction in other forums is permitted, provided the original author(s) or licensor are credited and that the original publication in this journal is cited, in accordance with accepted academic practice. No use, distribution or reproduction is permitted which does not comply with these terms.
* Correspondence:
Ana Otero, Department of Microbiology and Parasitology, Faculty of Biology - Aquatic One Health Research Center (ARCUS), Universidade de Santiago de Compostela, Santiago de Compostela, Spain
Manuel Romero, Department of Microbiology and Parasitology, Faculty of Biology - Aquatic One Health Research Center (ARCUS), Universidade de Santiago de Compostela, Santiago de Compostela, Spain
Disclaimer: All claims expressed in this article are solely those of the authors and do not necessarily represent those of their affiliated organizations, or those of the publisher, the editors and the reviewers. Any product that may be evaluated in this article or claim that may be made by its manufacturer is not guaranteed or endorsed by the publisher.
Research integrity at Frontiers
Learn more about the work of our research integrity team to safeguard the quality of each article we publish.