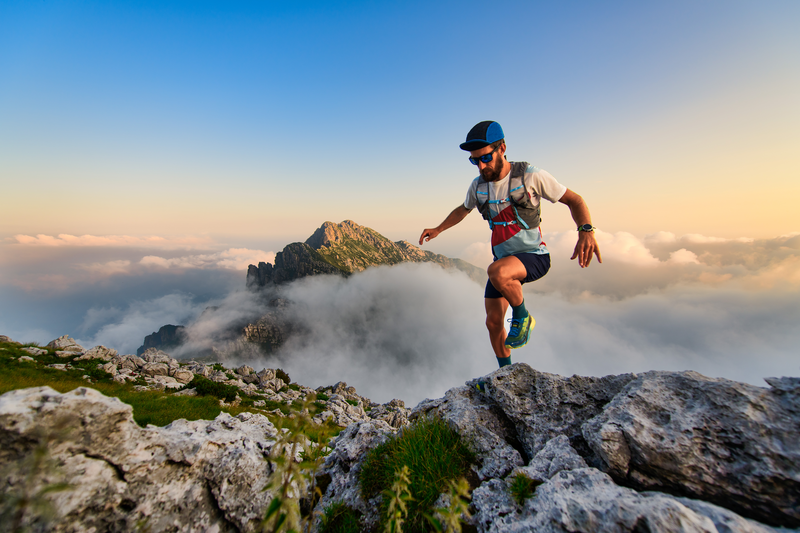
94% of researchers rate our articles as excellent or good
Learn more about the work of our research integrity team to safeguard the quality of each article we publish.
Find out more
MINI REVIEW article
Front. Cell. Infect. Microbiol.
Sec. Virus and Host
Volume 15 - 2025 | doi: 10.3389/fcimb.2025.1554090
This article is part of the Research Topic Recent Advancements in the Research Models of Infectious Diseases View all 4 articles
The final, formatted version of the article will be published soon.
You have multiple emails registered with Frontiers:
Please enter your email address:
If you already have an account, please login
You don't have a Frontiers account ? You can register here
Asymptomatic outcome accounts for most dengue virus infections and is likely to play an important role in maintaining virus circulation, contributing to its dissemination and shortening inter-epidemic periods. While dengue immunopathogenesis, investigation of potential therapeutics, and vaccine e icacy have been widely studied, only recently have inapparent infections begun to be comprehensively addressed as an integral and important part of the puzzle that is dengue infection. Animal models are one of the tools utilized to study dengue and, among these, mouse models have played an important role in understanding both dengue pathogenesis and the hosts' initial immune response. However, these models have mostly focused on untangling the drivers of disease severity ignoring asymptomatic dengue virus infections. In this mini-review, the authors propose to provide a concise overview of the current state-of-the-art of existing mouse models with potential use for studying asymptomatic dengue virus infections, elaborating on the pros and cons of the several models. Variations in experimental conditions, such as altering the viral load of the inoculum or employing di erent virus entry routes, especially in mice with partial or transient blockade of the type I interferon response, might be su icient to obtain both symptomatic and asymptomatic viremic mice. This would enable the study of factors involved in asymptomatic dengue virus infections.
Keywords: Dengue, mice models, Asymptomatic Infections, Inapparent infections, Dengue Virus, dengue fever, immune response
Received: 08 Jan 2025; Accepted: 21 Feb 2025.
Copyright: © 2025 Henriques, Rosa, Araújo and Vigário. This is an open-access article distributed under the terms of the Creative Commons Attribution License (CC BY). The use, distribution or reproduction in other forums is permitted, provided the original author(s) or licensor are credited and that the original publication in this journal is cited, in accordance with accepted academic practice. No use, distribution or reproduction is permitted which does not comply with these terms.
* Correspondence:
Ana Margarida Vigário, Projecto Medicina, Faculdade de Ciências da Vida, Universidade da Madeira, Funchal, Portugal
Disclaimer: All claims expressed in this article are solely those of the authors and do not necessarily represent those of their affiliated organizations, or those of the publisher, the editors and the reviewers. Any product that may be evaluated in this article or claim that may be made by its manufacturer is not guaranteed or endorsed by the publisher.
Research integrity at Frontiers
Learn more about the work of our research integrity team to safeguard the quality of each article we publish.