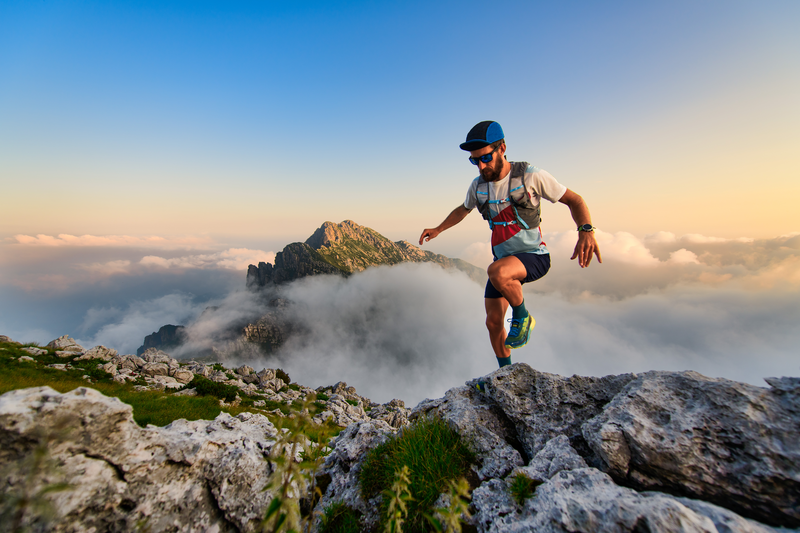
94% of researchers rate our articles as excellent or good
Learn more about the work of our research integrity team to safeguard the quality of each article we publish.
Find out more
ORIGINAL RESEARCH article
Front. Cell. Infect. Microbiol.
Sec. Clinical Microbiology
Volume 15 - 2025 | doi: 10.3389/fcimb.2025.1550908
This article is part of the Research Topic Advances in Vaginal Microbiome and Metabolite Research: Genetics, Evolution, and Clinical Perspectives View all articles
The final, formatted version of the article will be published soon.
You have multiple emails registered with Frontiers:
Please enter your email address:
If you already have an account, please login
You don't have a Frontiers account ? You can register here
Jiawei Ermiao Granule (JWEMG) is a traditional Chinese herbal formulation widely used in China for the treatment of human papilloma virus (HPV) infections. However, the mechanisms underlying their efficacy in clearing HPV infections remain unclear.This study aimed to elucidate the mechanisms by which JWEMG clears persistent HPV infections from a metabolomics perspective using modern analytical techniques.Untargeted and targeted metabolomics analyses were performed on vaginal lavage samples from 33 patients using liquid chromatograph mass spectrometer (LC-MS) and hydrophilic interaction liquid chromatography-mass spectrometry (HILIC-MS).Untargeted metabolomics identified 47 potential biomarkers through volcano plot analysis, among which 30 exhibited a reversal trend following JWEMG intervention. Kyoto Encyclopedia of Genes and Genomes (KEGG) pathway analysis suggested that JWEMG may exert therapeutic effects on patients with persistent HPV infections via pathways related to starch and sucrose metabolism, galactose metabolism, fructose and mannose metabolism, and amino sugar and nucleotide sugar metabolism.Targeted metabolomics revealed a significant increase in tyrosine levels in the vaginal/cervical microenvironment following JWEMG treatment. By integrating targeted and untargeted metabolomics, this study provides a comprehensive exploration of the holistic effects of JWEMG on HPV-infected patients, addressing the challenges of scientifically explaining the pharmacological mechanisms of multi-component, multi-target traditional Chinese medicines.
Keywords: Jiawei Ermiao Granule, HPV, untargeted metabolomics, Targeted metabolomics, amino acid metabolism, Tyrosine
Received: 24 Dec 2024; Accepted: 20 Mar 2025.
Copyright: © 2025 Li, Ning, Zhao, Xu, SI, Hua and Ren. This is an open-access article distributed under the terms of the Creative Commons Attribution License (CC BY). The use, distribution or reproduction in other forums is permitted, provided the original author(s) or licensor are credited and that the original publication in this journal is cited, in accordance with accepted academic practice. No use, distribution or reproduction is permitted which does not comply with these terms.
* Correspondence:
Qingling Ren, The First Clinical Medical School, Nanjing University of Chinese Medicine, Nanjing University of Chinese Medicine, Nanjing, China
Disclaimer: All claims expressed in this article are solely those of the authors and do not necessarily represent those of their affiliated organizations, or those of the publisher, the editors and the reviewers. Any product that may be evaluated in this article or claim that may be made by its manufacturer is not guaranteed or endorsed by the publisher.
Research integrity at Frontiers
Learn more about the work of our research integrity team to safeguard the quality of each article we publish.