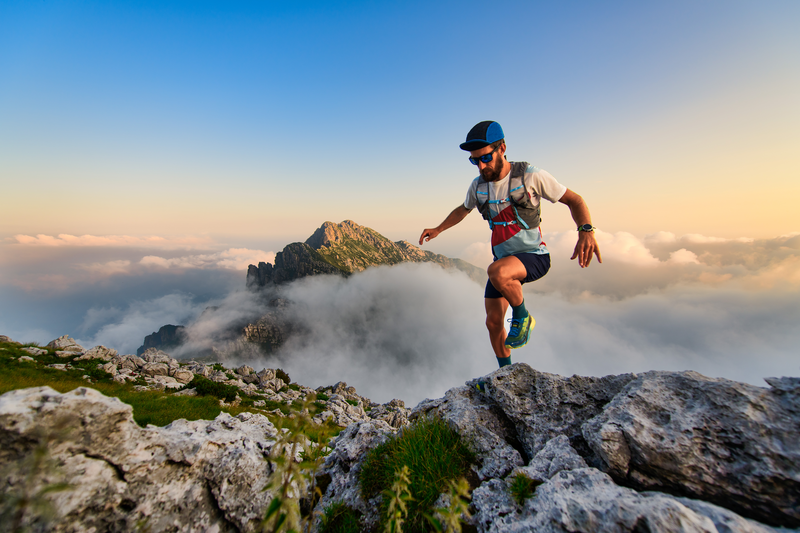
94% of researchers rate our articles as excellent or good
Learn more about the work of our research integrity team to safeguard the quality of each article we publish.
Find out more
ORIGINAL RESEARCH article
Front. Cell. Infect. Microbiol.
Sec. Clinical Infectious Diseases
Volume 15 - 2025 | doi: 10.3389/fcimb.2025.1549497
This article is part of the Research Topic Emerging concepts for respiratory viruses after the pandemic View all 4 articles
The final, formatted version of the article will be published soon.
You have multiple emails registered with Frontiers:
Please enter your email address:
If you already have an account, please login
You don't have a Frontiers account ? You can register here
Background:Non-pharmaceutical interventions (NPIs) implemented during the COVID-19 pandemic had a significant impact on the prevalence of various acute respiratory infections (ARIs) pathogens.Methods:We collected 337,310 real-time PCR results for 13 pathogens from clinical samples between January 2018 and January 2024 to assess the changes of ARIs among children before and after the COVID-19 pandemic.Results:A variety of ARIs pathogens, including Influenza A (Flu A), Influenza B (Flu B), Adenovirus (ADV), Rhinovirus (RhV), and Respiratory Syncytial Virus (RSV), as well as co-infecting bacterial such as Klebsiella pneumoniae (KPN), Pseudomonas aeruginosa (PAE), Streptococcus pneumoniae (SP), Haemophilus influenzae (HI), and Listeria monocytogenes (LP), reached a peak positive rate at the age of 3. The susceptible age of Mycoplasma pneumoniae (MP) was from 3 to 7 years old. Compared to the pre-COVID pandemic period, the positive rates of Flu A, MP, ADV, SP, HI, Staphylococcus aureus (SA) and KPN decreased during the COVID-19 pandemic. And the positive rates of Flu B and PAE increased. Compared to the period during the COVID-19 pandemic, the positive rates of Flu A, ADV, RSV, RhV, SP, HI, PKN, PAE and SA were increased after the pandemic. Conversely, the positive rates of MP, Flu B, and Parainfluenza virus (PIV) decreased.Conclusions:The implementation of NPIs interrupted the circulation of ARIs pathogens. However, release of NPIs and the reduced baseline of population immunity, may contribute to a resurgence of ARIs pathogens among children.
Keywords: COVID-19, respiratory infection, Non-pharmaceutical interventions, Children, qPCR
Received: 21 Dec 2024; Accepted: 10 Mar 2025.
Copyright: © 2025 Yue, Wu, Zeng, Li, Yang, Lv and Wang. This is an open-access article distributed under the terms of the Creative Commons Attribution License (CC BY). The use, distribution or reproduction in other forums is permitted, provided the original author(s) or licensor are credited and that the original publication in this journal is cited, in accordance with accepted academic practice. No use, distribution or reproduction is permitted which does not comply with these terms.
* Correspondence:
Ling Wang, Children's Hospital Affiliated to Shandong University, Ji nan, China
Disclaimer: All claims expressed in this article are solely those of the authors and do not necessarily represent those of their affiliated organizations, or those of the publisher, the editors and the reviewers. Any product that may be evaluated in this article or claim that may be made by its manufacturer is not guaranteed or endorsed by the publisher.
Research integrity at Frontiers
Learn more about the work of our research integrity team to safeguard the quality of each article we publish.