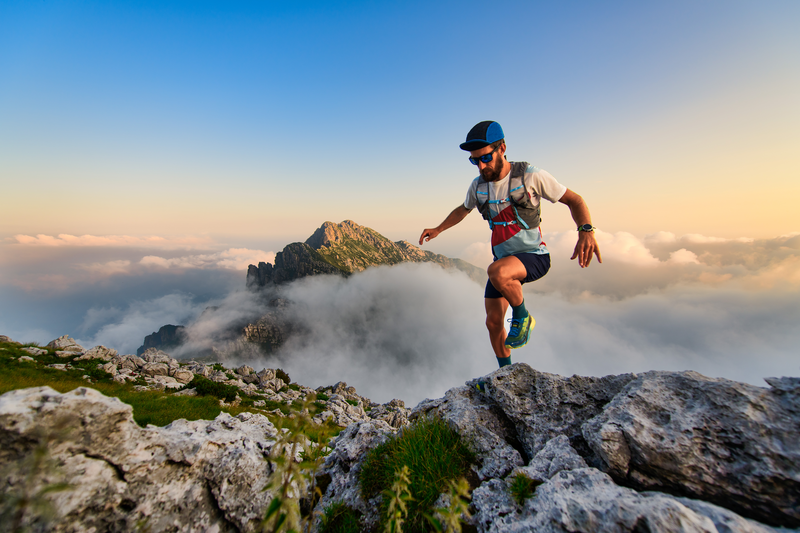
94% of researchers rate our articles as excellent or good
Learn more about the work of our research integrity team to safeguard the quality of each article we publish.
Find out more
ORIGINAL RESEARCH article
Front. Cell. Infect. Microbiol.
Sec. Fungal Pathogenesis
Volume 15 - 2025 | doi: 10.3389/fcimb.2025.1547800
This article is part of the Research Topic Evolutionary adaptation in human-infecting fungi: Ecological traits and pathogenicity View all articles
The final, formatted version of the article will be published soon.
You have multiple emails registered with Frontiers:
Please enter your email address:
If you already have an account, please login
You don't have a Frontiers account ? You can register here
Scedosporium species are filamentous fungi causing a wide spectrum of infections in healthy and debilitated individuals. Despite their clinical significance, the ecology of Scedosporium species remains understudied, particularly in the Middle East. In this study, we conducted an environmental survey of Scedosporium species in the North of Lebanon to elucidate their distribution and ecological preferences. Among the 155 soil samples collected from different environmental areas, 39 (25.16%) were positive for Scedosporium species, with a predominance of S. apiospermum (80.55%). Soil analysis revealed associations between the fungal presence and pH, nitrogen, phosphorus, and organic matter content. Moreover, genotyping analysis using MultiLocus Sequence Typing identified five major clusters. Interestingly, a number of Lebanese isolates formed an Asian-specific cluster (V) with one clinical Chinese isolate, whereas two clusters (II and III) showed a close association with German isolates, and clusters (I and IV) contained isolates with a global distribution. These findings provide new insights into the ecology of Scedosporium species, bridging a gap in our knowledge of their distribution on the Asian continent and laying the groundwork for future clinical investigations. Future international collaborations are essential to trace the origin of S. apiospermum.
Keywords: Scedosporium, Ecology, mlst, genotypes, Lebanon
Received: 18 Dec 2024; Accepted: 13 Feb 2025.
Copyright: © 2025 Mina, Yaakoub, Razafimandimby, Dwars, Wéry, Papon, Meyer and Bouchara. This is an open-access article distributed under the terms of the Creative Commons Attribution License (CC BY). The use, distribution or reproduction in other forums is permitted, provided the original author(s) or licensor are credited and that the original publication in this journal is cited, in accordance with accepted academic practice. No use, distribution or reproduction is permitted which does not comply with these terms.
* Correspondence:
Sara Mina, Beirut Arab University, Beirut, Lebanon
Disclaimer: All claims expressed in this article are solely those of the authors and do not necessarily represent those of their affiliated organizations, or those of the publisher, the editors and the reviewers. Any product that may be evaluated in this article or claim that may be made by its manufacturer is not guaranteed or endorsed by the publisher.
Research integrity at Frontiers
Learn more about the work of our research integrity team to safeguard the quality of each article we publish.