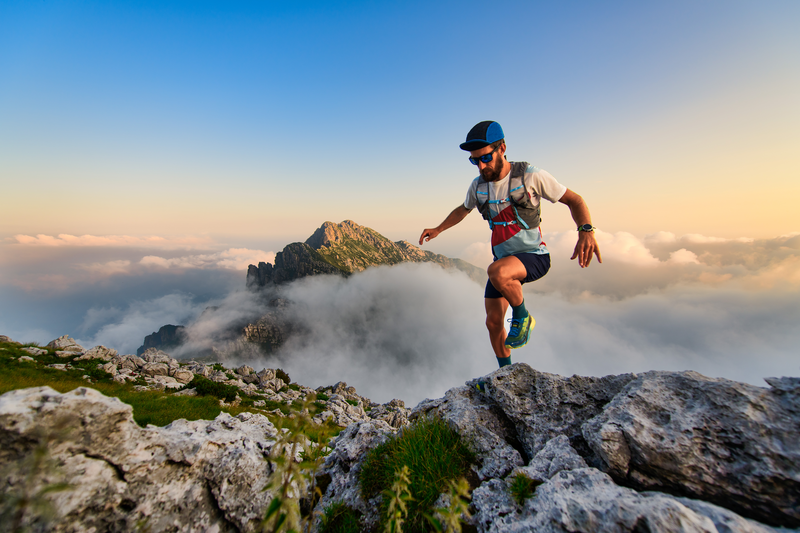
94% of researchers rate our articles as excellent or good
Learn more about the work of our research integrity team to safeguard the quality of each article we publish.
Find out more
ORIGINAL RESEARCH article
Front. Cell. Infect. Microbiol.
Sec. Extra-intestinal Microbiome
Volume 15 - 2025 | doi: 10.3389/fcimb.2025.1544794
This article is part of the Research Topic Advances in Vaginal Microbiome and Metabolite Research: Genetics, Evolution, and Clinical Perspectives View all 3 articles
The final, formatted version of the article will be published soon.
You have multiple emails registered with Frontiers:
Please enter your email address:
If you already have an account, please login
You don't have a Frontiers account ? You can register here
Objective. We investigated the changes in vaginal microbiota among females with persistent human papillomavirus (HPV) infection following cervical conization in Xinjiang, China. Methods. A total of 108 female participants were enrolled in the study, including 37 HPV-positive females without cervical conization (Group P1), 37 HPVpositive females after cervical conization (Group P2), and 34 HPV-negative females after cervical conization (Group N). DNA was extracted from vaginal secretions, and the V3-V4 regions of bacterial 16S rDNA were amplified and sequenced using NovaSeq technology. The diversity analysis of the bacterial microbiota was conducted using QIIME2 and R software, while the phenotypic analysis was performed with Bugbase software. Results. Lactobacillus was the predominant genus in the vaginal microbiota of women with persistent HPV infection after cervical conization in Xinjiang. Following partial cervical resection, the α-diversity of the vaginal microbiota decreased, particularly among patients who had cleared HPV. Bacterial vaginosis-associated anaerobes were common in the vaginal environment, with their relative abundance increasing in cases of persistent HPV infection. Postoperative persistent HPV infection was found to be correlated not only with pathogens linked to bacterial vaginosis but also with those associated with aerobic vaginitis. Gardnerella and Atopobium, as well as Bifidobacterium and Streptococcus, demonstrated a symbiotic synergy. Both Lactobacillus and Gardnerella exhibited negative correlations with many pathogenic bacteria. Anaerobic and biofilm formation were the most evident phenotypes in individuals with persistent HPV infection after conization. Conclusion. The vaginal microbiota of women with persistent HPV infection following cervical conization is characterized by the coexistence of Lactobacillus dominance and increased microbial diversity. Anaerobic bacteria and biofilm formation may play a significant role in the persistence of HPV infection postsurgery, and the role of Gardnerella in the vaginal flora under an HPV-infected state warrants further study.
Keywords: vaginal microbiota, HPV infection, Cervical Intraepithelial Neoplasia, Cervical conization, 16S rDNA
Received: 16 Dec 2024; Accepted: 21 Mar 2025.
Copyright: © 2025 Lingyun, Cailing, Yan, Yilidana, Lipeng and Yanjia. This is an open-access article distributed under the terms of the Creative Commons Attribution License (CC BY). The use, distribution or reproduction in other forums is permitted, provided the original author(s) or licensor are credited and that the original publication in this journal is cited, in accordance with accepted academic practice. No use, distribution or reproduction is permitted which does not comply with these terms.
* Correspondence:
Ma Cailing, State Key Laboratory of Pathogenesis, Prevention and Treatment of High Incidence Diseases in Central Asia; Department of Gynecology, the First Affiliated Hospital of Xinjiang Medical University, Urumqi, China
Disclaimer: All claims expressed in this article are solely those of the authors and do not necessarily represent those of their affiliated organizations, or those of the publisher, the editors and the reviewers. Any product that may be evaluated in this article or claim that may be made by its manufacturer is not guaranteed or endorsed by the publisher.
Research integrity at Frontiers
Learn more about the work of our research integrity team to safeguard the quality of each article we publish.