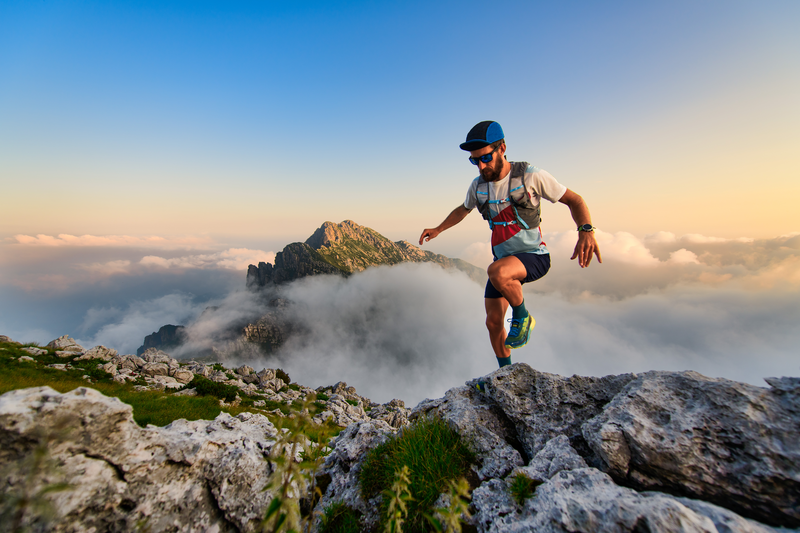
94% of researchers rate our articles as excellent or good
Learn more about the work of our research integrity team to safeguard the quality of each article we publish.
Find out more
ORIGINAL RESEARCH article
Front. Cell. Infect. Microbiol.
Sec. Biofilms
Volume 15 - 2025 | doi: 10.3389/fcimb.2025.1537564
This article is part of the Research Topic Mechanisms of microbial persistence and strategies to counter them View all 3 articles
The final, formatted version of the article will be published soon.
You have multiple emails registered with Frontiers:
Please enter your email address:
If you already have an account, please login
You don't have a Frontiers account ? You can register here
Staphylococcus aureus Sortase A can anchor virulence proteins, which are responsible for bacterial adhesion, biofilm formation and inflammation, to the cell membrane surface. The ability of β-lactam antibiotics to combat S. aureus infections is limited by the presence of β-lactamases in this pathogen. In this study, we determined that epicatechin gallate (ECG) and its analogues inhibited the transpeptidase activity of Sortase A by interacting with it directly, and the biofilm formation and adhesion abilities of the bacterium decreased after treatment with ECG and its analogues. Additionally, ECG bound to β-lactamase and reduced its ability to hydrolyse nitrocefin. Furthermore, ECG synergized with ampicillin (Amp), enhancing its bactericidal effects and inhibiting the formation of persisters. ECG did not affect the expression of Sortase A or β-lactamase but significantly alleviated the cytotoxicity of S. aureus USA300. In vivo, ECG alone or combined with Amp improved the survival of mice infected with S. aureus USA300, alleviated pathological tissue damage and pulmonary oedema, and reduced the extent of inflammation and level of colonization. The results of this study indicate that the active ingredients of green tea, especially ECG, have the potential to be developed as anti-S. aureus infection agents.
Keywords: ECG, Sortase A, β-lactamase, Biofilm, Persisters, Staphylococcus aureus
Received: 01 Dec 2024; Accepted: 28 Feb 2025.
Copyright: © 2025 Teng, Wang, Wen, Tian, Wang and Peng. This is an open-access article distributed under the terms of the Creative Commons Attribution License (CC BY). The use, distribution or reproduction in other forums is permitted, provided the original author(s) or licensor are credited and that the original publication in this journal is cited, in accordance with accepted academic practice. No use, distribution or reproduction is permitted which does not comply with these terms.
* Correspondence:
Guizhen Wang, Jilin Engineering Normal University, Changchun, China
Disclaimer: All claims expressed in this article are solely those of the authors and do not necessarily represent those of their affiliated organizations, or those of the publisher, the editors and the reviewers. Any product that may be evaluated in this article or claim that may be made by its manufacturer is not guaranteed or endorsed by the publisher.
Research integrity at Frontiers
Learn more about the work of our research integrity team to safeguard the quality of each article we publish.