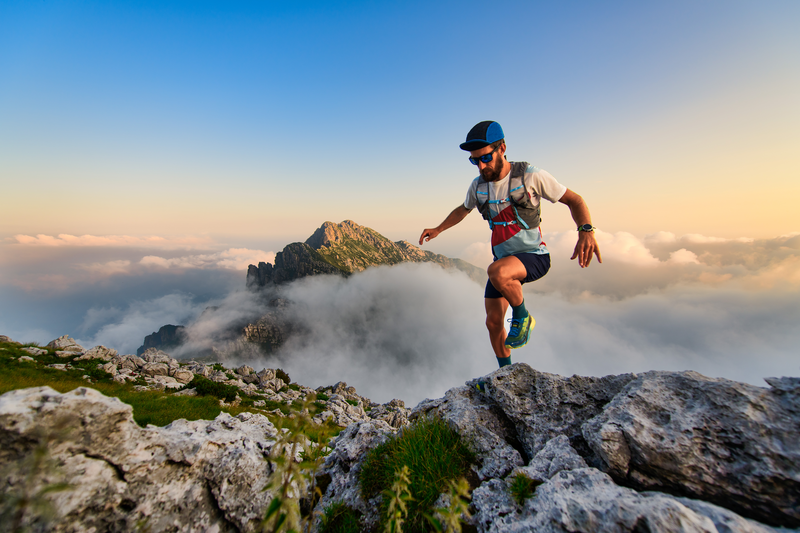
94% of researchers rate our articles as excellent or good
Learn more about the work of our research integrity team to safeguard the quality of each article we publish.
Find out more
ORIGINAL RESEARCH article
Front. Cell. Infect. Microbiol.
Sec. Intestinal Microbiome
Volume 15 - 2025 | doi: 10.3389/fcimb.2025.1535204
This article is part of the Research Topic Harnessing the Medicinal Potential of Gut Microbiota for Human Health View all 3 articles
The final, formatted version of the article will be published soon.
You have multiple emails registered with Frontiers:
Please enter your email address:
If you already have an account, please login
You don't have a Frontiers account ? You can register here
The intestinal microbiota is important for the health of the host and recent studies have shown that some genes of the host regulated the composition of the intestinal microbiota. Group 10 phospholipase A2 (PLA2G10) is a member of the lipolytic enzyme family PLA2, which hydrolyze the ester bond at the sn-2 position of phospholipids to produce free fatty acids and lysophospholipids. PLA2G10 is secreted into the intestinal lumen, but its impact on the gut microbiota remains unclear. In this study, we generated intestine-specific Pla2g10 knock-in mice, and used 16S RNA sequencing to compare their gut microbiota with that of their wild-type (WT) littermates. Results showed that gut-specific Pla2g10 knock-in induced both PLA2G10 mRNA and protein levels in the colon. Moreover, intestinal Pla2g10 overexpression reduced the α-diversity of the gut microbiota relative to that of WT mice. The abundance of Bacteroidetes was lower in the Pla2g10 knock-in mice than that in the control mice, while the ratio of Firmicutes/Bacteroidetes was higher. Furthermore, the abundance of the genus Allobaculum was reduced, whereas the abundance of beneficial bacteria genera, including Enterorhabdus, Dubosiella, and Lactobacillus, was increased by host intestinal Pla2g10 overexpression. In summary, intestinal Pla2g10 overexpression increased the proportions of beneficial bacterial in the colonic chyme of mice, providing a potential therapeutic target for future improvement of the gut microbiota.
Keywords: PLA2G10, Gut Microbiota, intestine, Allobaculum, Dubosiella
Received: 27 Nov 2024; Accepted: 26 Feb 2025.
Copyright: © 2025 Liao, Jiang, Che, Fang, Xu, Lin, Zhuo, Li, Liu, Sun, Wu, Wang, Feng, Cao and Hua. This is an open-access article distributed under the terms of the Creative Commons Attribution License (CC BY). The use, distribution or reproduction in other forums is permitted, provided the original author(s) or licensor are credited and that the original publication in this journal is cited, in accordance with accepted academic practice. No use, distribution or reproduction is permitted which does not comply with these terms.
* Correspondence:
Hairui Wang, Chengdu Research Base of Giant Panda Breeding, Chengdu, Sichuan Province, China
Bin Feng, Sichuan Agricultural University, Ya'an, China
Disclaimer: All claims expressed in this article are solely those of the authors and do not necessarily represent those of their affiliated organizations, or those of the publisher, the editors and the reviewers. Any product that may be evaluated in this article or claim that may be made by its manufacturer is not guaranteed or endorsed by the publisher.
Research integrity at Frontiers
Learn more about the work of our research integrity team to safeguard the quality of each article we publish.