- 1Office of Research Administration, Chiang Mai University, Chiang Mai, Thailand
- 2Department of Biology, Faculty of Science, Chiang Mai University, Chiang Mai, Thailand
- 3Centre for Mountain Futures (CMF), Kunming Institute of Botany, Kunming, Yunnan, China
- 4Honghe Center for Mountain Futures, Kunming Institute of Botany, Chinese Academy of Sciences, Kunming, Yunnan, China
- 5Department of Economic Plants and Biotechnology, Yunnan Key Laboratory for Wild Plant Resources, Kunming Institute of Botany, Chinese Academy of Sciences, Kunming, Yunnan, China
- 6The Center for International Forestry Research and World Agroforestry (CIFOR-ICRAF) China Program, World Agroforestry (ICRAF), Kunming, Yunnan, China
- 7Department of Botany and Microbiology, College of Science, King Saud University, Riyadh, Saudi Arabia
- 8Biology Division, Vishnugupta Vishwavidyapeetam, Gokarna, India
- 9Center of Excellence in Microbial Diversity and Sustainable Utilization, Chiang Mai University, Chiang Mai, Thailand
- 10Academy of Science, The Royal Society of Thailand, Bangkok, Thailand
Endophytic fungi are a well-known fascinating host-associated fungal group that can enhance plant growth and fitness by producing various bioactive secondary metabolites. They are an excellent source of industrial enzymes for potential secondary metabolite synthesis, which is useful in green agriculture, biotechnology, and pharmaceuticals. Itea is a valuable plant genus since it naturally contains rare sugar; however, endophytic fungi associated in this host have not yet been documented. In the present study, 11 strains of endophytic fungi were isolated and primarily identified as pestalotioid taxa based on morphological characteristics exhibited in vitro. Eleven strains of Pestalotiopsis-like taxa were isolated from the healthy leaves, stems, and roots of Itea japonica and I. riparia from Chiang Mai Province, Thailand. Species delimitation was based on morphology, multiloci phylogeny of a concatenated ITS, tub2, and tef1-α sequence data, and nucleotide polymorphism analyses. Neopestalotiopsis iteae and Pseudopestalotiopsis iteae are proposed as new species on I. japonica and I. riparia, respectively. Neopestalotiopsis chrysea, N. haikouensis, and Pestalotiopsis jinchanghensis are described as new records on I. riparia. Owing to the conspecific relationship based on multiloci phylogeny and identically nucleotide pairwise comparison of sufficient gene regions, several species are synonymized including Neopestalotiopsis cercidicola and N. terricola as N. haikouensis, N. umbrinospora as N. chrysea, and Pestalotiopsis zhaoqingensis as P. jinchanghensis. The updated phylogenetic trees, nucleotide comparisons, and morphological descriptions are herein provided and discussed for the taxonomic placements of these new species and records. This study is the first to investigate Itea endophytes in Thailand, and it reveals the intra- and interspecific relationships of pestalotioid species, which need to be further reevaluated because of ambiguous taxa.
1 Introduction
Pestalotioid fungi are generally referred to as appendage-bearing coelomycetes that have multi-septate and fusiform conidia in the family Sporocadaceae (Amphisphaeriales, Sordariomycetes), including genera Neopestalotiopsis, Pestalotiopsis, and Pseudopestalotiopsis (Maharachchikumbura et al., 2011; 2014; Peng et al., 2022; Razaghi et al., 2024). Pestalotiopsis and allied genera are taxonomically, chemically, and ecologically diverse, which have been associated with a broad range of host plants through their life cycle as endophytes, pathogens, and saprobes (Peng et al., 2022; Giri and Pradhan, 2023; Hsu et al., 2024). The natural classification of this group based on the conidial morphology suggests concolorous median cells in Pestalotiopsis and Pseudopestalotiopsis, and versicolorous median cells in Neopestalotiopsis (Maharachchikumbura et al., 2014; Peng et al., 2022; Sun et al., 2023). Compared with Pestalotiopsis, Pseudopestalotiopsis has darker colored median cells (Maharachchikumbura et al., 2014; Nozawa et al., 2018; Sun et al., 2023). However, the characteristics of conidia (e.g., color, size, and shape) and conidial appendages (e.g., number, length, shape, branched or unbranched, and presence or absence of knobbed tips) are overlapping (Maharachchikumbura et al., 2014; Liu et al., 2017; Tsai et al., 2021). The multiloci phylogeny of the concatenated ITS, tub2, and tef1-α coupled with morphology has proved to be reliable for the taxonomic circumscription of pestalotioid species (Maharachchikumbura et al., 2012; 2014; Nozawa et al., 2017; 2018; Norphanphoun et al., 2019; Sun et al., 2023; Hsu et al., 2024).
Ecologically, Pestalotiopsis-like species are important as phytopathogens, endophytes, and saprobes and have a wide range of distribution in the temperate regions and tropics (Maharachchikumbura et al., 2012; 2014; Gu et al., 2022; Peng et al., 2022; Giri and Pradhan, 2023; Hsu et al., 2024). As plant pathogens, they cause various diseases, resulting in significant loss in various economic plants such as avocado (Fiorenza et al., 2022), eucalyptus (Diogo et al., 2021), blueberry (Zheng et al., 2023), macadamia (Prasannath et al., 2021), mangrove-associated hosts (Norphanphoun et al., 2019), pine (Monteiro et al., 2022), rose (Peng et al., 2022), and tea (Liu et al., 2017; Chen et al., 2018; Tsai et al., 2021). Endophytic pestalotioid fungi are considered as a valuable source for producing secondary metabolites with diverse chemical structures and promising antibacterial, antifungal, and anticancer properties (Xu et al., 2010; Palwe et al., 2021; Wu et al., 2022; Giri and Pradhan, 2023). Since the discovery of the antitumor drug taxol from Pestalotiopsis microspora, which was earlier isolated from Taxus wallachiana (Strobel et al., 1996), more studies have focused on Pestalotiopsis and allied genera.
Itea is a plant genus in Iteaceae, including approximately 21 species, widely distributed in Africa, Asia, and North America (POWO, 2024; Song and Zhang, 2024). Itea japonica is an edible species, native to Japan, and located in the temperate regions (Ayers et al., 2014; POWO, 2024), whereas I. riparia is endemic to China, Laos, Myanmar, Thailand, and Vietnam and is located in the tropical region (POWO, 2024; Song and Zhang, 2024). Itea species are recognized for their nutritional and medicinal value owing to the presence of rare sugars (e.g., D-allulose and allitol) and other bioactive compounds (e.g., coumarins, flavonoids, and terpenoids), which exhibited antioxidant, anti-hepatocellular carcinoma, anti-tuberculosis, and glycosidase inhibitory activities (Luo et al., 2014; Zeng et al., 2015; Lang et al., 2022; Feng et al., 2024). Previous studies have isolated some pathogenic and endophytic fungi from Itea species (Farr et al., 2021). However, fungi associated with I. japonica and I. riparia have not yet been reported. Therefore, it is worthy to investigate the fungi associated to this host plant as it could offer a promising resource for new discoveries of useful bioactive compounds and enzymes. As part of our study on endophytic fungi associated with Itea species, we recovered 11 Pestalotiopsis-like taxa from fresh tissues of I. japonica and I. riparia in Chiang Mai Province, Thailand. Therefore, the aims of this study are to identify these novel strains using morphological and molecular approaches and to examine their intra- and intergeneric affinities within the pestalotioid species.
2 Materials and methods
2.1 Collection and isolation of endophytic fungi
Fresh and healthy samples (viz., leaves, stems, and roots) of Itea japonica and I. riparia were collected from Chiang Mai Province, northern Thailand. The plant samples were initially rinsed with running tap water and cut into small sections (5 mm × 5 mm). The surface sterilization of plant tissues was carried out by immersing in distilled water for 1 min, 70% alcohol for 30 s, and 2% NaOCl for 30 s, in this order. Finally, the plant sections were thoroughly rinsed in sterile distilled water, air dried, and placed on potato dextrose agar (PDA) plates. After incubation at 25°C for 24–48 h, the hyphal tips grown on the margin of the plant tissues were picked up and inoculated onto new PDA plates to obtain the pure cultures. The cultures were incubated at 25°C for 1–2 months for the sporulation and morphological examination (Mattoo and Nonzom, 2022). The living cultures were deposited in the Culture Collection of Sustainable Development of Biological Resources Laboratory, Faculty of Science, Chiang Mai University, Chiang Mai Province, Thailand (SDBR-CMU) and the Kunming Institute of Botany Culture Collection, Kunming, China (KUNCC). To preserve the dried fungal samples (herbarium), cultures were dried in the hot air oven at 45–50°C for 1–2 days and deposited at the Herbarium of the Department of Biology, Faculty of Science, Chiang Mai University, Chiang Mai Province, Thailand (CMUB). The newly described taxa were registered for the Index Fungorum numbers (https://indexfungorum.org/Names/IndexFungorumRegisterName.asp; accessed on 13 November 2024).
2.2 Morphological observation and examination
The morphological characteristics of endophytic fungi (i.e., conidiomata, conidiophores, conidiogenous cells, and conidia) grown on PDA after 30 days were observed using a Nikon SMZ800N stereo microscope (Nikon Instruments Inc., Melville, NY, USA) and a Nikon Eclipse Ni compound microscope attached to a Nikon DS-Ri2 camera system (Nikon Instruments Inc., Melville, NY, USA). Permanent slides were prepared by adding lactoglycerol and sealed with clear nail polish. The measurements of fungal structures were carried out using a Tarosoft (R) Image Frame Work version 0.9.7 program. The photographic plates were edited and combined using Adobe Photoshop version 21.2.4 (Adobe Systems, San Jose, CA, USA).
2.3 DNA extraction, PCR amplification, and sequencing
The Biospin Fungus Genomic DNA Extraction Kit (BioFlux, Hangzhou, China) was used to extract DNA from 7-day-old colonies grown on PDA at 25°C. The internal transcribed spacer region of ribosomal DNA (ITS) was amplified using primers ITS5 and ITS4 (White et al., 1990); the beta-tubulin (tub2) was amplified using primers T1 and Bt2b (Glass and Donaldson, 1995; O’Donnell and Cigelnik, 1997); and the translation elongation factor-1 alpha (tef1-α) was amplified using primers EF1-728F and EF2 (O’Donnell et al., 1998; Carbone and Kohn, 1999). The PCR conditions for the three loci were set up following Sun et al. (2023) and Gao et al. (2024). For Pseudopestalotiopsis species, the beta-tubulin (tub2) was additionally amplified using primers Bt2a and Bt2b (Glass and Donaldson, 1995; O’Donnell and Cigelnik, 1997) with the PCR condition as described by Maharachchikumbura et al. (2012). The PCR amplification was performed in a 25-μL reaction volume, containing 12.5 μL of Master Mix (mixture of EasyTaqTM DNA Polymerase, dNTPs, and optimized buffer; Beijing TransGen Biotech Co., Ltd., Chaoyang District, Beijing, China), 8.5 μL of double-distilled water (ddH2O), 2 μL of DNA template, and 1 μL of each forward and reverse primer (10 μM). The purification of PCR products and DNA sequencing were conducted by TsingKe Company (Kunming City, Yunnan Province, China).
2.4 Phylogenetic analyses
The sequences were checked and assembled using BioEdit version 7.0.5.3 (Hall, 1999). The homogeneous sequences were obtained from relevant publications (Li et al., 2022; Zhang et al., 2022; 2024; Sun et al., 2023; Dong et al., 2023; Hsu et al., 2024) and the results of BLAST searches that were downloaded from GenBank (www.ncbi.nlm.nih.gov/blast/; accessed on 1 September 2024) (Tables 1–3). Alignments for each locus were carried out using the MAFFT v7.307 online version (Katoh et al., 2019; https://mafft.cbrc.jp/alignment/server/, accessed on 1 September 2024) and manually verified in BioEdit version 7.0.5.3 (Hall, 1999). To construct the phylogenetic analyses, maximum likelihood (ML) was previously applied for single locus, and further combined datasets of ITS, tub2, and tef1-α gene loci were analyzed by Bayesian inference (BI) and ML when the tree topology of each locus is congruent. ML analysis was generated with 1,000 bootstrap (BS) iterations and the GTRGAMMA model of nucleotide evolution using RAxML-HPC2 on XSEDE (v.8.2.12) (Stamatakis, 2014) via the online web platform CIPRES Science Gateway V3.3 (Miller et al., 2010). The best-fit substitution models were evaluated using MrModeltest v.2.3 (Nylander, 2004). BI analysis was implemented with MrBayes on XSEDE v.3.2.7a (Huelsenbeck and Ronquist, 2001; Zhaxybayeva and Gogarten, 2002; Ronquist et al., 2012) via the same platform (Miller et al., 2010). Six simultaneous Markov chains were run for 10 million generations but were automatically terminated when the standard deviation of split frequency approached 0.01. The trees were sampled every 100th generation, and the first 25% of sampled trees were removed during the burn-in phase of analyses, which were evaluated by Tracer v. 1.7 (Rambaut et al., 2018). The phylograms were displayed and modified with the FigTree v1.4.0 program (Rambaut and Drummond, 2012) and Adobe Illustrator version 24.3 (Adobe Systems, San Jose, CA, the USA). The newly generated sequences were submitted to the NCBI database to obtain GenBank accession numbers.
3 Results
3.1 Multiloci phylogenetic analyses
Analysis 1: the combined sequence dataset of ITS, tub2, and tef1-α comprised 158 Neopestalotiopsis strains and two outgroup taxa, Pestalotiopsis diversiseta (MFLUCC 12-0287) and P. spathulata (CBS 356.86) (Figure 1). The aligned dataset consisted of a total 1,896 characters with gaps (ITS: 1–541 bp, tub2: 542–1340 bp, tef1-α: 1341–1896 bp). The best-scoring RAxML tree was selected to represent the relationships among taxa, with a final likelihood value of −11,777.047879. The matrix contained 944 distinct alignment patterns, with 17.98% undetermined characters or gaps. Estimated base frequencies were as follows: A = 0.235628, C = 0.271348, G = 0.215132, and T = 0.277892; substitution rates were AC = 0.918956, AG = 2.618624, AT = 1.144576, CG = 0.760024, CT = 3.458802, and GT = 1.000000; gamma distribution shape parameter α = 0.361998; Tree-Length = 1.334915. For BI analysis, the best-fitting model of each locus and the final average standard deviation of split frequencies are shown in Table 4. Similar tree topologies were acquired by ML and Bayesian posterior probability (BYPP) analyses. The phylogenetic tree revealed that a new species, Neopestalotiopsis iteae (SDBR-CMU515), formed an independent branch sister to N. cocoës (0.91 BYPP; Figure 1). The new strain, SDBR-CMU517, is closely related to N. cercidicola, N. haikouensis, and N. terricola (Figure 1), whereas the new strain, SDBR-CMU516, grouped with N. chrysea and N. umbrinospora (Figure 1).
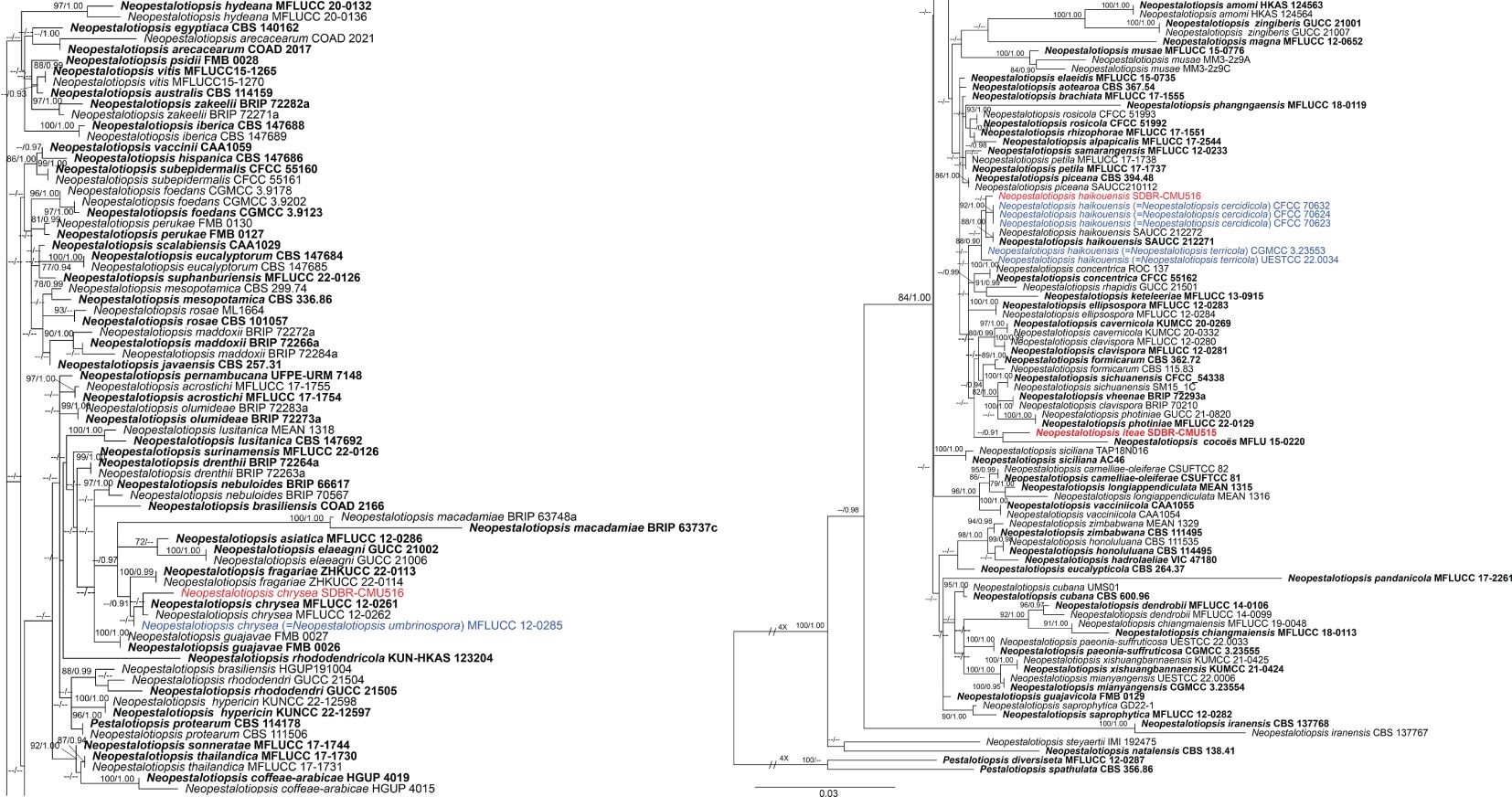
Figure 1. Phylogram constructed by maximum likelihood (ML) analyses of combined ITS, tub2, and tef1-α sequence dataset of Neopestalotiopsis. Bootstrap support valued for ML and Bayesian posterior probabilities (BYPP) equal to or greater than 70% and 0.90 are shown above the nodes as ML/BYPP. The new isolates are indicated in red and the ex-type strains are in bold. The synonymized taxon is indicated in blue. The tree is rooted with Pestalotiopsis diversiseta (MFLUCC 12-0287) and P. spathulata (CBS 356.86).

Table 4. The best-fit substitution model and the average standard deviation of split frequencies resulting from the Bayesian inference (BI) analysis. .
Analysis 2: the combined sequence dataset of ITS, tub2, and tef1-α comprised 186 Pestalotiopsis strains and two outgroup taxa, Neopestalotiopsis cubana (CBS 114178) and N. protearum (ZHKUCC 23-0825) (Figure 2). The aligned dataset consisted of a total 1,959 characters with gaps (ITS: 1–604 bp, tub2: 605–1399 bp, tef1-α: 1400–1959 bp). The best-scoring RAxML tree was selected to represent the relationships among taxa, with a final likelihood value of −19,588.100995. The matrix contained 1,090 distinct alignment patterns, with 23.02% undetermined characters or gaps. Estimated base frequencies were as follows: A = 0.238520, C = 0.293691, G = 0.214954, and T = 0.252835; substitution rates were AC = 1.093468, AG = 2.897598, AT = 1.166594, CG = 1.024049, CT = 4.038506, and GT = 1.000000; gamma distribution shape parameter α = 0.338893, Tree-Length = 2.486656. For BI, the best-fitting model of each locus and the final average standard deviation of split frequencies are shown in Table 4. Similar tree topologies were acquired from ML and BYPP analyses. In the phylogenetic tree (Figure 2), the new strain, SDBR-CMU518, clustered together with Pestalotiopsis jinchanghensis and P. zhaoqingensis (86% ML and 1.00 BYPP; Figure 2).
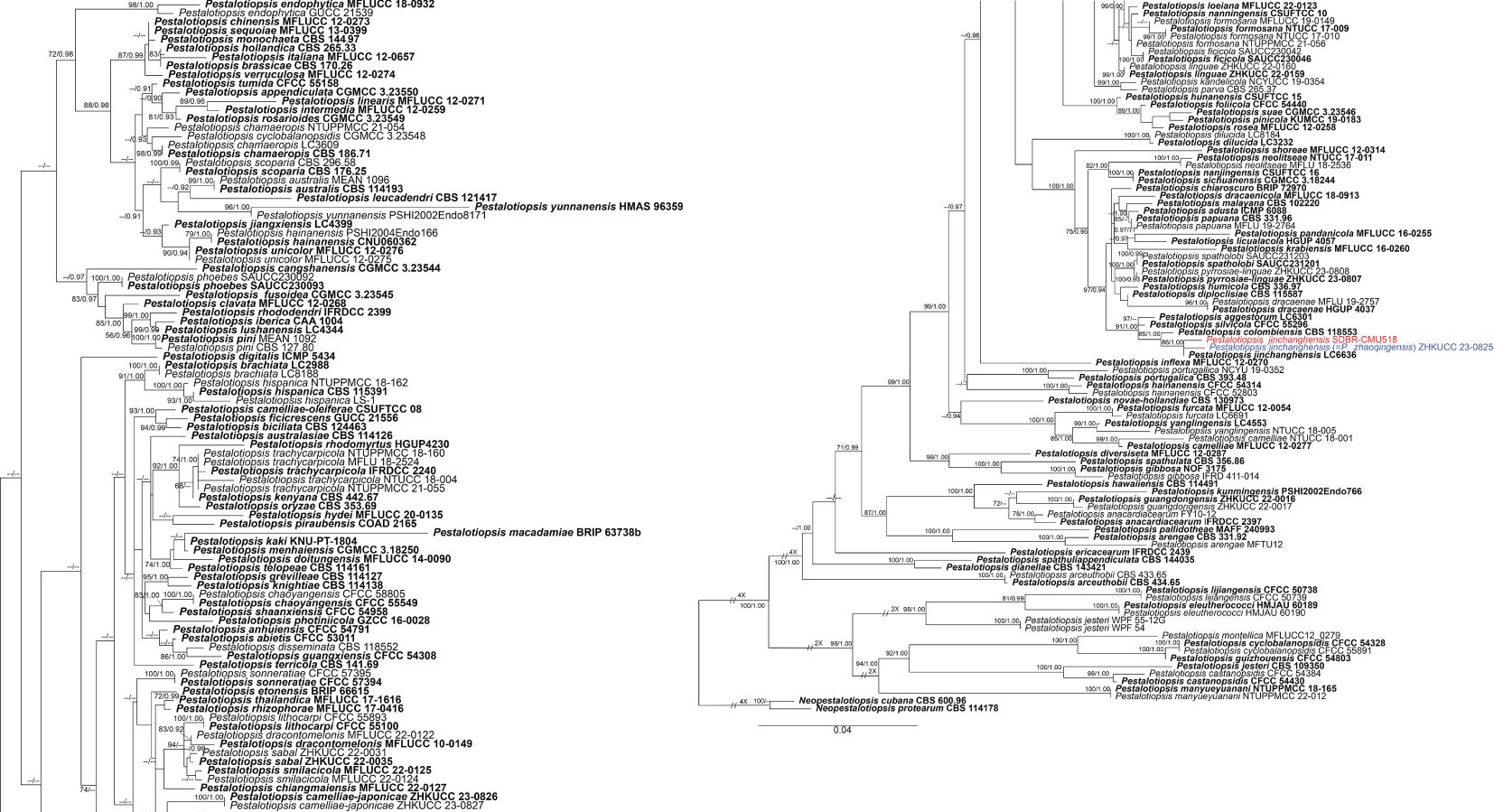
Figure 2. Phylogram constructed by maximum likelihood (ML) analyses of combined ITS, tub2, and tef1-α sequence dataset of Pestalotiopsis. Bootstrap support valued for ML and Bayesian posterior probabilities (BYPP) equal to or greater than 70% and 0.90 are shown above the nodes as ML/BYPP. The new isolates are indicated in red and the ex-type strains are in bold. The synonymized taxon is indicated in blue. The tree is rooted with Neopestalotiopsis cubana (CBS 114178) and N. protearum (ZHKUCC 23-0825).
Analysis 3: the combined sequence dataset of ITS, tub2, and tef1-α comprised 44 Pseudopestalotiopsis strains and two outgroup taxa, Pestalotiopsis linearis (MFLUCC 12-0271) and P. trachycarpicola (IFRDCC 2240) (Figure 3). The aligned dataset consisted of a total 1,848 characters with gaps (ITS: 1–550 bp, tef1-α: 551–1067 bp, tub2: 1068–1848 bp). The best-scoring RAxML tree was selected to represent the relationships among taxa, with a final likelihood value of −6,642.700192. The matrix contained 554 distinct alignment patterns, with 16.44% undetermined characters or gaps. Estimated base frequencies were as follows: A = 0.243417, C = 0.274291, G = 0.213172, and T = 0.269120; substitution rates were AC = 1.025029, AG = 2.296252, AT = 1.005669, CG = 0.871957, CT = 3.231208, and GT = 1.000000; gamma distribution shape parameter α = 0.306881, Tree-Length = 0.625052. For BI analysis, the best-fitting model of each locus and the final average standard deviation of split frequencies are shown in Table 4. Similar tree topologies were acquired by ML and BYPP analyses. The phylogenetic tree showed that Pseudopestalotiopsis iteae (SDBR-CMU514, SDBR-CMU519, SDBR-CMU520, SDBR-CMU521, SDBR-CMU522, SDBR-CMU523, and SDBR-CMU524) grouped with Ps. theae strain NTUCC 18-067 (70% ML and 0.92 BYPP) and formed a distinct subclade basal to the ex-epitype of Ps. theae (MFLUCC 12-0055) (Figure 3).
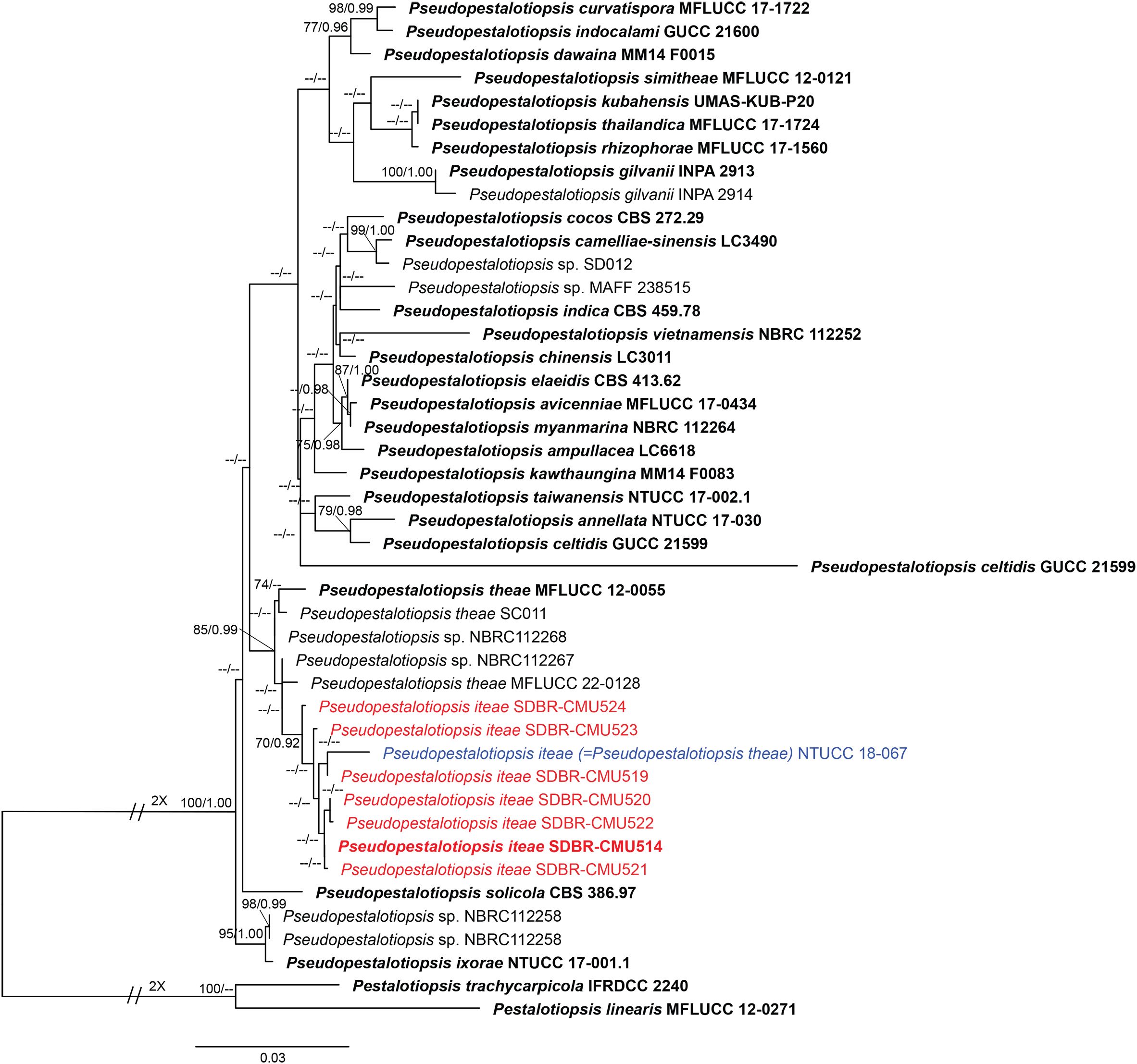
Figure 3. Phylogram constructed by maximum likelihood (ML) analyses of combined ITS, tub2, and tef1-α sequence dataset of Pseudopestalotiopsis. Bootstrap support valued for ML and Bayesian posterior probabilities (BYPP) equal to or greater than 70% and 0.90 are shown above the nodes as ML/BYPP. The new isolates are indicated in red and the ex-type strains are in bold. The synonymized taxon is indicated in blue. The tree is rooted with Pestalotiopsis linearis (MFLUCC 12-0271) and P. trachycarpicola (IFRDCC 2240).
3.2 Taxonomy
Neopestalotiopsis chrysea (Maharachch. & K.D. Hyde) Maharachch., K.D. Hyde & Crous, Stud. Mycol. 79: 138 (2014) (Figure 4).
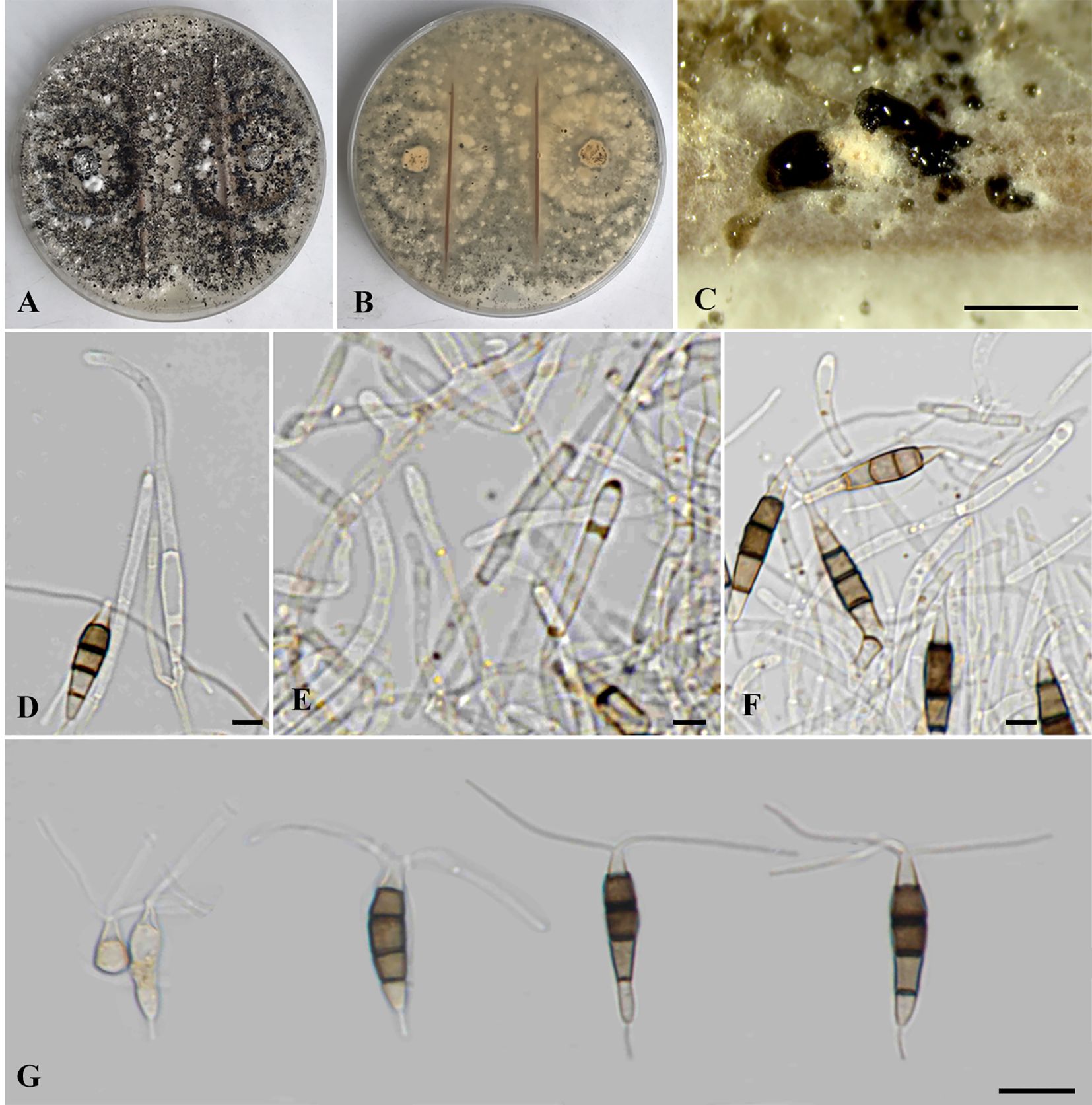
Figure 4. Neopestalotiopsis chrysea (SDBR-CMU516). (A) Surface of colonies on PDA. (B) Reverse of colonies on PDA. (C) Conidiomata and conidia masses. (D–F) Conidiophores, conidiogenous cells and conidia. (G) Conidia. Scale bars: (B, C) 500 μm, (D–F) 5 μm, and (G) 10 μm.
≡ Pestalotiopsis chrysea Maharachch. and K.D. Hyde, Fungal Divers. 56(1): 107 (2012).
= Neopestalotiopsis umbrinospora (Maharachch. and K.D. Hyde) Maharachch., K.D. Hyde & Crous, Stud. Mycol. 79: 149 (2014).
Typification: CHINA, Guangxi Province, Shangsi, Shiwandashan, Wangle, dead leaves of unidentified plant, 2 January 1997, W.P. Wu (HMAS042855, holotype; MFLU 12-0411, isotype, ex-type culture NN042855 = MFLUCC 12-0261).
Description of the new collection: Endophytic on healthy roots of Itea riparia. Sexual morph: Undetermined. Asexual morph: Conidiomata up to 600 μm diameter, globose to subglobose, solitary to aggregated, black, semi-immersed on PDA, releasing globose, brown to black, slimy conidial masses. Conidiophores up to 65 μm long × 2–3 μm wide, hyaline, brown septa, branched. Conidiogenous cells 6–22 × 1.5–3 μm ( = 15.5 × 2 μm, n = 10), discrete, holoblastic, subcylindrical to lageniform, hyaline, smooth-walled. Conidia 18.5–27.5 × 4–6 μm ( = 22 × 5 μm, n = 30), fusiform to elongated fusiform, or ellipsoid, narrower towards the basal cells, straight to slightly curved, 4-septate; basal cell 3–7 μm long, subcylindrical to obconic, hyaline to pale brown, thin and smooth-walled; three median cells 12–16 μm long, sometimes hyaline to pale brown, one median cell when immature, pale brown to dark brown, with thick and darker brown septa, versicolored, second cell from base 4–4.5 μm, pale to light brown; third cell 4–4.5 μm, darker brown to olivaceous; fourth cell 4–4.5 μm, pale brown to olivaceous; apical cell 3–5 μm long, hyaline, obconic to conic; with two to three apical appendages, arising from the apical crest, filiform, unbranched, 24–30 μm long; with a single basal appendage, filiform, unbranched, centric, 3–6 μm long.
Culture characteristics: Colonies on PDA reached at 7 cm diameter after 5 days at 25°C, irregular form, raised elevation, crenate edge, whitish, with sparse aerial mycelium on surface, yellowish to pale brown in reverse. Sporulation on PDA at 25°C after 30 days, with numerous black fruiting bodies.
Material examined: THAILAND, Chiang Mai Province, Mae Rim District, on healthy roots of Itea riparia (Iteaceae), 10 July 2023, J. Monkai, IT107 (CMUB 40073), living culture, SDBR-CMU516 = KUNCC 24-18917, dried culture permanently preserved in a metabolically inactive state, CMUB 40073.
GenBank number: PQ521226 (ITS), PQ560699 (tub2), and PQ529177 (tef1-α).
Habitats and host: Dead and living tissue of Carya illinoinensis, Itea riparia, Vaccinium ashei, and unidentified plants (Maharachchikumbura et al., 2012; 2014; Shi et al., 2017; Wu et al., 2021; this study).
Distribution: China (Anhui, Fujian, Guangxi and Hunan Provinces) and Thailand (Maharachchikumbura et al., 2012; 2014; Shi et al., 2017; Wu et al., 2021; this study).
Notes: Based on the nucleotide BLAST search of ITS sequence, the new isolate of Neopestalotiopsis chrysea (SDBR-CMU516) showed the closest similarity with Neopestalotiopsis sp. 15 SSNM-2014 strain CBS 177.25 (100%), Pestalotiopsis sp. LH162 (100%), and N. clavispora strain YZM-1 (100%). Based on the nucleotide BLAST search of tub2 sequence, N. chrysea (SDBR-CMU516) showed the closest similarity with Neopestalotiopsis sp. strain LC3480 (99.35%), N. asiatica isolate JGGH5 (99.35%), and N. chrysea isolate LSCKS81 (99.35%). Based on the nucleotide BLAST search of tef1-α sequence, N. chrysea (SDBR-CMU516) showed the closest similarity with Pestalotiopsis chrysea strain MFLUCC12-0261 (99.59%), Neopestalotiopsis sp. strain LC3480 (99.59%), and N. chrysea strain FZXM038 (99.38%).
Our new strain (SDBR-CMU516) was phylogenetically close to Neopestalotiopsis chrysea and N. umbrinospora (Figure 1). The nucleotide comparison between our new strain (SDBR-CMU516) and N. chrysea (MFLUCC 12-0261, ex-type) showed 0.9% (4/430) and 0.4% (2/487) bp difference in tub2 and tef1-α (whereas those in ITS are identical). The nucleotide comparison between our new strain (SDBR-CMU516) and N. umbrinospora (MFLUCC 12-0285, ex-type) showed 0.9% (4/430) and 0.8% (4/487) bp difference in tub2 and tef1-α (whereas those in ITS are identical). The nucleotide comparison between N. chrysea (MFLUCC 12-0261, ex-type) and N. umbrinospora (MFLUCC 12-0285, ex-type) showed 0.4% (2/487) in tef1-α (whereas those in ITS and tub2 are identical).
Pestalotiopsis chrysea and P. umberspora were initially introduced by Maharachchikumbura et al. (2012), which transferred to Neopestalotiopsis chrysea and N. umbrinospora based on morphology and molecular phylogeny (Maharachchikumbura et al., 2014). Neopestalotiopsis chrysea was described by their distinct yellowish conidiogenous cells, conidia, and colony (Maharachchikumbura et al., 2012; 2014), whereas N. umbrinospora was characterized by its umber color of the median cells of the conidia (Maharachchikumbura et al., 2012; 2014). Both species were isolated from dead plant materials from Guangxi and Hunan China (Maharachchikumbura et al., 2012; 2014). Although there are only few base-pair differences between N. umbrinospora and N. chrysea, Maharachchikumbura et al. (2012; 2014) treated N. umbrinospora differently from the latter species due to its umber color and relatively wider conidia (9–25 × 6–8 μm vs. 20–24 × 5.5–7 μm), whereas our new strain (SDBR-CMU516) shares similar conidial shapes, color, and size to N. chrysea in producing darker brown to olivaceous conidia (18.5–27.5 × 4–6 μm vs. 20–24 × 5.5–7 μm) with three-tubular apical appendages (24–30 μm vs. 22–30 μm) (Maharachchikumbura et al., 2012; 2014). Therefore, we identified this strain as a new host record of N. chrysea on Itea based on morphological and phylogenetic lines of evidence. Furthermore, N. umbrinospora is synonymized under N. chrysea herein due to their conspecific relationship based on phylogenetic evidence coupled with the nucleotide pairwise comparison of the sufficient genes.
Neopestalotiopsis haikouensis Z.X. Zhang, J.W. Xia, and X.G. Zhang, MycoKeys 88: 181 (2022) (Figure 5).
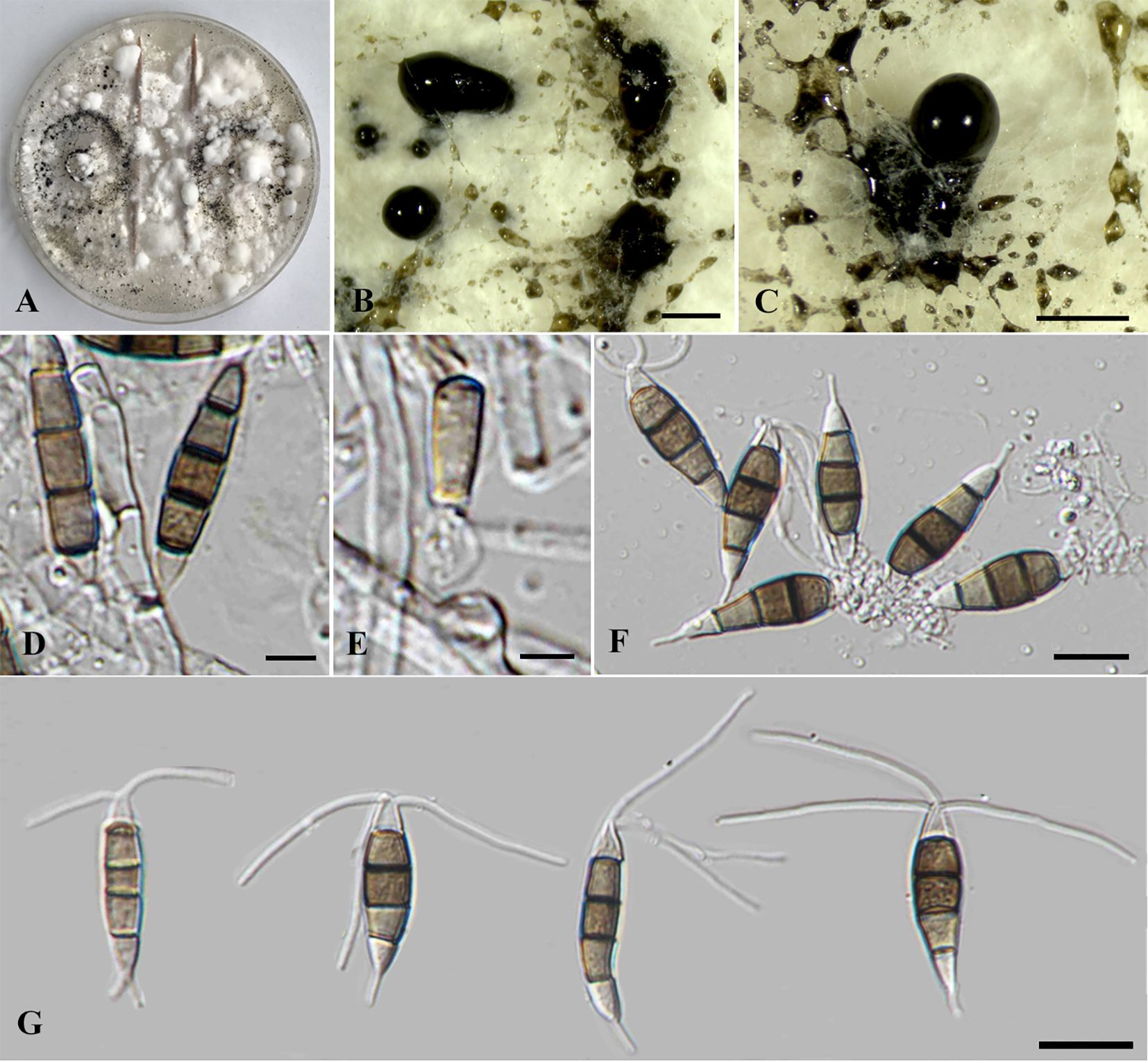
Figure 5. Neopestalotiopsis haikouensis (SDBR-CMU517). (A) Surface of colonies on PDA. (B, C) Conidiomata and conidia masses. (D–F) Conidiophores, conidiogenous cells, and conidia. (G) Conidia. Scale bars: (B, C) 500 μm, (D–F) 5 μm, and (G) 10 μm.
= Neopestalotiopsis cercidicola W.S. Zhang and X.L. Fan, J. Fungi 10: 475 (2024).
= Neopestalotiopsis terricola W.L. Li and Jian K. Liu, J. Fungi 8: 1175 (2022).
Typification: CHINA, Hainan Province, Haikou City: East Harbour National Nature Reserve, on diseased leaves of Ilex chinensis, 23 May 2021, Z.X. Zhang (HSAUP212271, holotype), ex-type living culture: SAUCC212271.
Description of the new collection: Endophytic on healthy stems of Itea riparia. Sexual morph: Undetermined. Asexual morph: Conidiomata up to 500 μm diameter, pycnidial, globose to subglobose, solitary to aggregated, black, semi-immersed on PDA, releasing globose, brown to black, slimy conidial masses. Conidiophores up to 140 μm long × 2–3.5 μm wide, hyaline, dark brown septa, branched, sometimes giving rise to conidia. Conidiogenous cells 4–8.5 × 2.5–6 μm ( = 7 × 4 μm, n = 10), discrete, holoblastic, subcylindrical to ampulliform, hyaline, smooth-walled. Conidia 20–30 × 5–7 μm ( = 25 × 6 μm, n = 30), fusiform to elongated fusiform, or ellipsoid, sometimes slightly wider in the upper median cells, straight to slightly curved, 4-septate; basal cell 1–6 μm long, obconic to conic, hyaline, thin and smooth-walled; three median cells 13–18.5 μm long, pale brown when immature, pale brown to dark brown, darker at septa, versicolored, verruculose, second cell from base 4–6 μm, pale brown; third cell 4.5–5.5 μm, dark brown to olivaceous; fourth cell 3.5–6 μm, pale brown to olivaceous; apical cell 3.5–5 μm long, hyaline, subcylindrical to obconic; with two to three apical appendages (mostly three), arising from the apical crest, filiform, unbranched, 25–32.5 μm long; with a single basal appendages, filiform, unbranched, centric, 3–12 μm long.
Culture characteristics: Colonies on PDA reached at 7 cm diameter after 5 days at 25°C irregular form, raised elevation, crenate edge, whitish, cottony, with dense aerial mycelium on surface. Sporulation on PDA at 25°C after 30 days, with numerous black fruiting bodies.
Material examined: THAILAND, Chiang Mai Province, Mae Rim District, on living stems of Itea riparia (Iteaceae), 10 July 2023, J. Monkai, IT92 (CMUB40074), living culture, SDBR-CMU517 = KUNCC 24-18918, dried culture permanently preserved in a metabolically inactive state, CMUB40074.
GenBank number: PQ521227 (ITS), PQ560700 (tub2), and PQ529178 (tef1-α).
Habitats and host: Dead and living tissue of Castanea mollissima, Cercis chinensis, Ilex chinensis, Itea riparia, Paeonia suffruticosa, and Olea europaea (Jiang et al., 2021; Li et al., 2022; Zhang et al., 2022; 2024; this study).
Distribution: China (Hainan, Sichuan and Yunnan Provinces) and Thailand (Jiang et al., 2021; Li et al., 2022; Zhang et al., 2022; 2024; this study).
Notes: Based on the nucleotide BLAST search of ITS sequence, the new isolate of Neopestalotiopsis haikouensis (SDBR-CMU517) showed the closest similarity with N. saprophytica (100%), Hymenopleella hippophaeicola isolate SF134_3_1 (100%), and Pestalotiopsis microspora isolate WH55 (100%). Based on the nucleotide BLAST search of tub2 sequence, N. haikouensis (SDBR-CMU517) showed the closest similarity with Neopestalotiopsis sp. strain PP026 (99.73%), N. clavispora isolate MCH21 (99.73%), and N. clavispora isolate SGP37 (99.73%). Based on the nucleotide BLAST search of tef1-α sequence, N. haikouensis (SDBR-CMU517) showed the closest similarity with N. protearum strain GBLZ16PE-007 (99.8%), N. protearum strain GUCC 23-0329 (99.8%), and Neopestalotiopsis sp. strain LC2945 (99.8%).
In the phylogenetic analyses (Figure 1), our new strain (SDBR-CMU517) clustered with Neopestalotiopsis cercidicola, N. haikouensis, and N. terricola. The nucleotide comparison among our new strain (SDBR-CMU517) and the ex-type strains of N. cercidicola, N. haikouensis, and N. terricola indicated low nucleotide difference (less than 1%) (Table 5). Zhang et al. (2022) introduced N. haikouensis as a leaf spot disease of Ilex chinensis. Lately, N. terricola and N. cercidicola were isolated as pathogens of Paeonia suffruticosa (Li et al., 2022) and Cercis chinensis (Zhang et al., 2024), respectively. Based on morphology, our new strain (SDBR-CMU517) shares similar conidial color (pale brown) and size (20–30 × 5–7 μm) to N. haikouensis (16–22 × 4.5–7 μm) (Zhang et al., 2022) and N. cercidicola (17.5–23.5 × 5.5–8.5 μm) (Zhang et al., 2024) (Table 6), whereas the conidia of N. terricola is wider and darker in the middle cell (20–23 × 8–9.5 μm), compared to others (Li et al., 2022) (Table 6). Li et al. (2022) noted the variation of conidial color and size among strains of N. terricola depending on host substrates. The ex-type strain of N. terricola (CGMCC3.23553), which was isolated from diseased leaves of Paeonia suffruticosa, had darker and relatively wider conidia than other strains including the strain UESTCC 22.0034, isolated from infected olive leaves (see Figures 6 and 9 in Li et al., 2022), and two strains (CFCC 54337 and CFCC 54340) recovered from diseased leaves of Castanea mollissima (see Figure 17 in Jiang et al., 2021) (Table 6). Moreover, we could detect the varieties of conidial shape and size in our samples, which is shown in Figure 5G. Therefore, because of the morphological and phylogenetic consistencies, we synonymized N. terricola and N. cercidicola under N. haikouensis following the prior publication. Likewise, our new strain is reported as a new host record of N. haikouensis on Itea and it is also a new geographical record in Thailand.
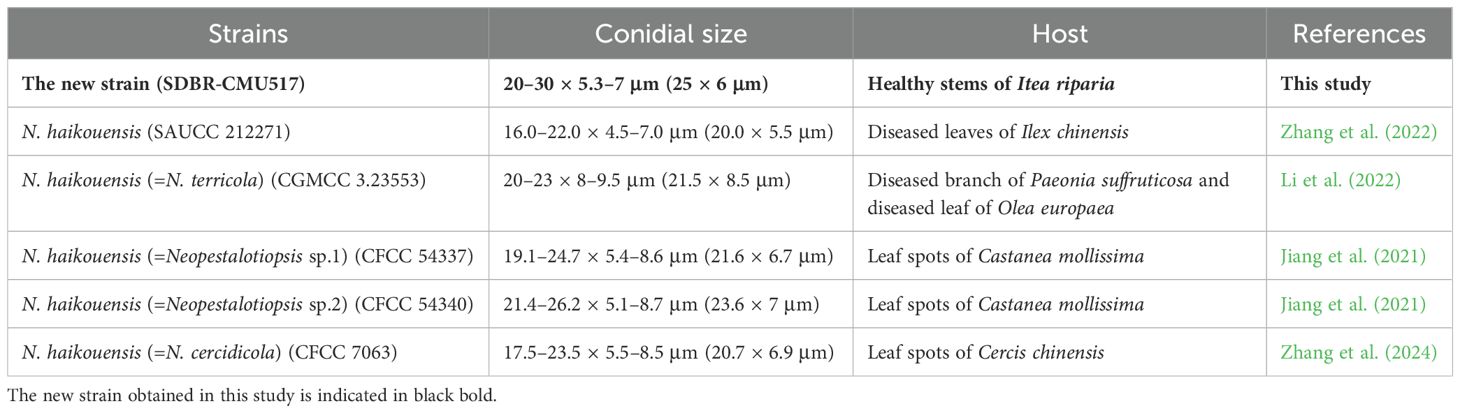
Table 6. The comparison of conidial morphology and host between the closely related strains of Neopestalotiopsis haikouensis.
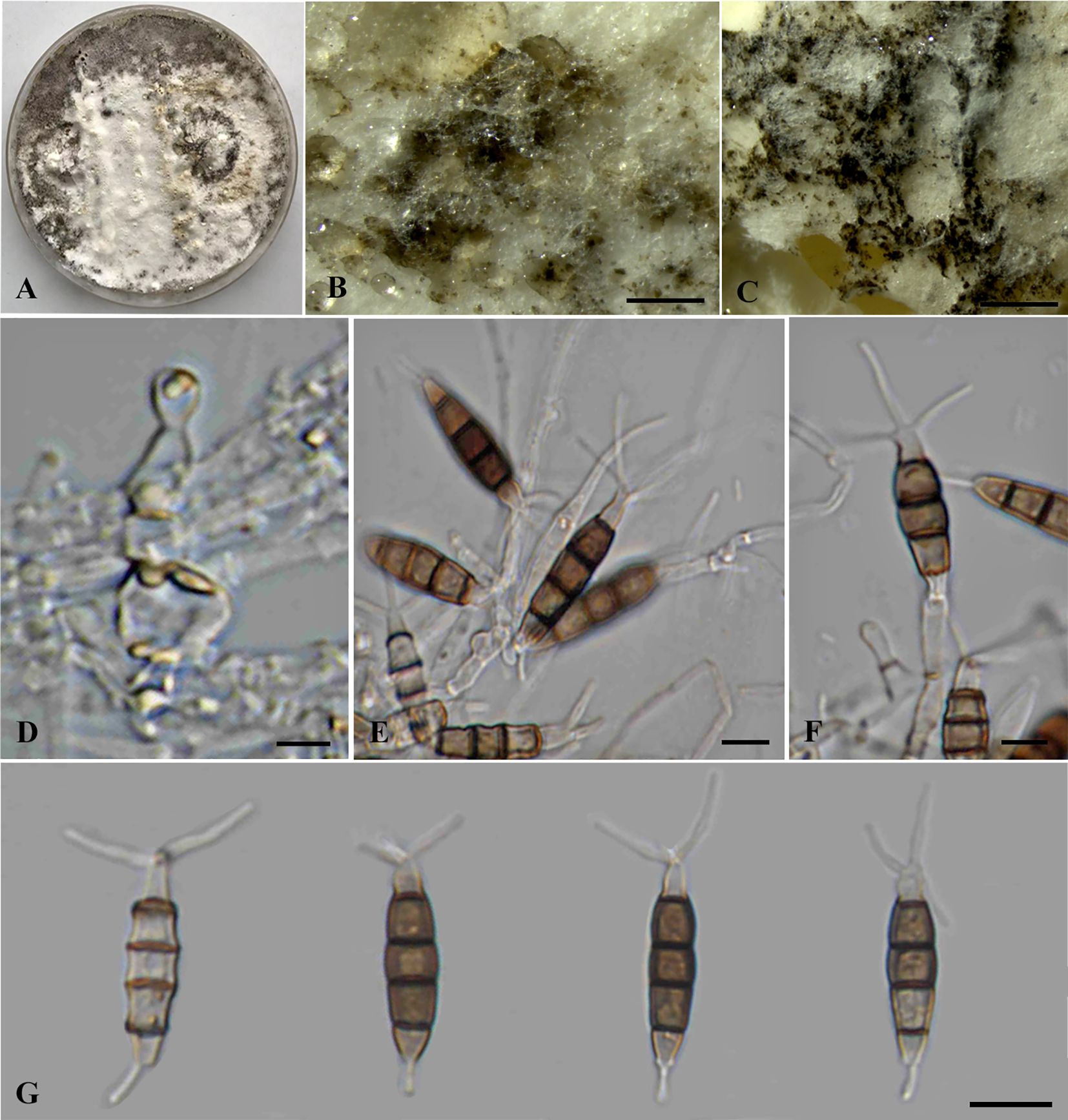
Figure 6. Neopestalotiopsis iteae (SDBR-CMU515, ex-type). (A) Surface of colonies on PDA. (B, C) Conidiomata. (D–F) Conidiophores, conidiogenous cells, and conidia. (G) Conidia. Scale bars: (B, C) 500 μm, (D–F) 5 μm, and (G) 10 μm.
Neopestalotiopsis iteae Monkai, Phookamsak, Bhat & S. Lumyong, sp. nov. (Figure 6).
Index Fungorum number: IF902438.
Etymology: Refers to the host genus, Itea from which the holotype was collected.
Endophytic on healthy stems of Itea japonica. Sexual morph: Undetermined. Asexual morph: Conidiomata up to 400 μm diameter, globose to subglobose, solitary to aggregated, black, raising above surface of PDA. Conidiophores up to 50 μm long × 2–3 μm wide, hyaline, dark brown septa, branched. Conidiogenous cells 4–9.5 × 2.5–4 μm ( = 6 × 3 μm, n = 10), discrete, holoblastic, subcylindrical to ampulliform, hyaline, smooth-walled. Conidia 17.5–26 × 4.5–6.5 μm ( = 22.5 × 5.5 μm, n = 30), fusiform, ellipsoid, straight, 4-septate; basal cell 4–5.5 μm long, obconic to conic, hyaline, thin and smooth-walled; three median cells 12–17.5 μm long, initially hyaline with brown septa, becoming brown to pale brown, with darker brown septa, slightly constricted at septa, with second cell from base 4–6.5 μm, pale brown to olivaceous; third cell 3.5–6 μm, brown to olivaceous; fourth cell 4–6 μm, brown to olivaceous; apical cell 4.5–6 μm long, hyaline, subcylindrical to obconic; with two to three apical appendages, not arising from the apical crest, but each inserted at a different locus in the upper half of the apical cell, filiform, unbranched, 8.5–14 μm long; with a single basal appendages, filiform, unbranched, 4.5–6 μm long.
Culture characteristics: Colonies on PDA reached at 7 cm diameter after 5 days at 25°C, circular form, flat elevation, undulage edge, whitish to gray, with dense aerial mycelium on surface. Sporulation on PDA at 25°C after 30 days, with numerous black fruiting bodies.
Material examined: THAILAND, Chiang Mai Province, Hang Dong District, on living stems of Itea japonica (Iteaceae), 7 February 2023, J. Monkai, IT41 (CMUB40072, holotype), ex-type living culture, SDBR-CMU515 = KUNCC 24-18919, dried culture permanently preserved in a metabolically inactive state, CMUB40072.
GenBank number: PQ521228 (ITS), PQ560701 (tub2), and PQ529179 (tef1-α).
Notes: Based on the nucleotide BLAST search of ITS sequence, Neopestalotiopsis iteae sp. nov. (SDBR-CMU515) showed the closest similarity with N. clavispora isolate MI003 (100%), N. saprophytica (100%), and Pestalotiopsis maculans isolate RM1.18A.01 (100%). Based on the nucleotide BLAST search of tub2 sequence, N. iteae (SDBR-CMU515) showed the closest similarity with N. piceana (99.12%), N. piceana strain CBS 225.30 (99.12%), and Neopestalotiopsis sp. strain PP026 (99.12%). Based on the nucleotide BLAST search of tef1-α sequence, N. iteae (SDBR-CMU515) showed the closest similarity with Neopestalotiopsis sp. isolate YJ11-0708 (98.57%), N. clavispora isolate SGP35 (98.57%), and N. clavispora isolate MCH27 (98.57%).
Phylogenetically, Neopestalotiopsis iteae formed a stable clade sister to N. cocoës (0.91 BYPP; Figure 1). The nucleotide comparison between N. iteae (SDBR-CMU515) and N. cocoës (MFLUCC 15-0152, ex-type) showed 0.4% (2/520) and 2.4% (6/253) bp differences in ITS and tef1-α (where tub2 sequence data of N. cocoës were not available for comparison). It should be noted that the tef1-α sequence data of N. cocoës are very short (approximately 200–300 bp). The low support between N. iteae and N. cocoës might be coming from the uncompleted sequence data of N. cocoës. Morphologically, N. iteae can be distinguished from N. cocoës in having longer and narrower conidia (17.5–26 × 4.5–6.5 μm vs. 19–22.5 × 7.5–9.5 μm) and slightly constricted septa (Hyde et al., 2016). Based on the lines of evidence in morphology and phylogeny coupled with a significant nucleotide pairwise difference of tef1-α sequence, N. iteae was introduced as a novel species.
Pestalotiopsis jinchanghensis Liu, Hou, Raza and Cai, Scientific Reports 7 (no. 866): 8 (2017) (Figure 7).
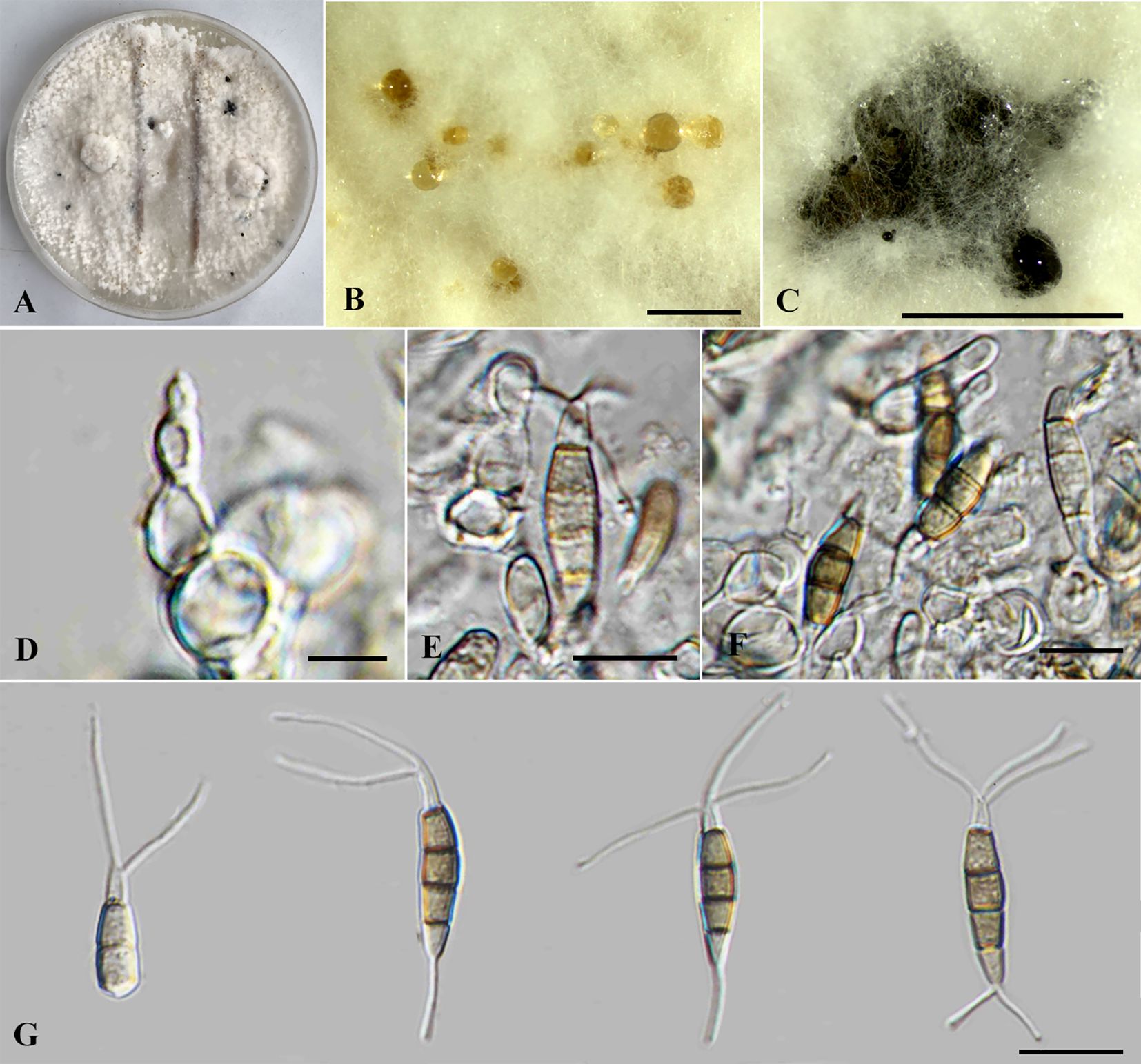
Figure 7. Pestalotiopsis jinchanghensis (SDBR-CMU518). (A) Surface of colonies on PDA. (B, C) Conidiomata and conidia masses. (D–F) Conidiogenous cells with attached conidia. (G) Conidia. Scale bars: (B, C) 500 μm and (D–G) 10 μm.
= Pestalotiopsis zhaoqingensis H.J. Zhao and W. Dong, Mycosphere 14(1): 2238 (2023).
Typification: CHINA, Yunnan Province, Xishuangbanna, Jinchanghe, on leaves of Camellia sinensis, 20 April 2015, F. Liu, HMAS 247061 (holotype), ex-type living culture CGMCC 3.18158 (= LC6636).
Description of the new collection: Endophytic on healthy stems of Itea riparia. Sexual morph: Undetermined. Asexual morph: Conidiomata up to 600 μm diameter, pycnidial, globose to subglobose, solitary, black, semi-immersed on PDA, releasing globose, brown to black, slimy conidial masses. Conidiophores often reduced to conidiogenous cells. Conidiogenous cells 4–10 × 2.5–8.5 μm ( = 7.5 × 6.5 μm, n = 10), discrete, holoblastic, ampulliform to lageniform, hyaline, smooth-walled. Conidia 19–27 × 4–6 μm ( = 23.5 × 5 μm, n = 30), fusiform to elongated fusiform, straight to slightly curved, 4-septate; basal cell 3.5–4.5 μm long, obconic to conic, hyaline, thin and smooth-walled; three median cells 12–16.5 μm long, sometimes hyaline, two median cells when immature, brown to olivaceous, septa and periclinal walls darker than the rest of the cell, concolorous, wall rugose, second cell from base 4–5 μm; third cell 3.5–5.5 μm; fourth cell 4–5.5 μm; apical cell 3.5–4.5 μm long, hyaline, subcylindrical to obconic; with two to three tubular apical appendages, arising from the apical crest, filiform, unbranched, 14–27 μm long; with 1(–2) tubular basal appendages, filiform, unbranched, centric, 5–11.5 μm long.
Culture characteristics: Colonies on PDA reached at 7 cm diameter after 5 days at 25°C, irregular form, raised elevation, crenate edge, whitish, cottony, with dense aerial mycelium on surface. Sporulation on PDA at 25°C after 30 days, with few black fruiting bodies.
Material examined: THAILAND, Chiang Mai Province, Mae Rim District, on living stems of Itea riparia (Iteaceae), 10 July 2023, J. Monkai, IT86 (CMUB40075), living culture, SDBR-CMU518 = KUNCC 24-18920), dried culture permanently preserved in a metabolically inactive state, CMUB40075.
GenBank number: PQ521229 (ITS), PQ560702 (tub2), and PQ529180 (tef1-α).
Habitats and host: Dead and living tissue of Camellia sinensis, Itea riparia, and unidentified plants (Liu et al., 2017; Dong et al., 2023; this study).
Distribution: China (Yunnan and Guangdong Provinces) and Thailand (Liu et al., 2017; Dong et al., 2023; this study).
Notes: Based on the nucleotide BLAST search of ITS sequence, the new isolate of Pestalotiopsis jinchanghensis (SDBR-CMU518) showed the closest similarity with Pestalotiopsis sp. NJ-2022e strain SAUCC230044 (99.83%), Pestalotiopsis sp. strain JMB08_3B2 (99.67%), and P. malayana strain SAUCC230483 (99.66%). Based on the nucleotide BLAST search of tub2 sequence, P. jinchanghensis (SDBR-CMU518) showed the closest similarity with P. zhaoqingensis strain ZHKUCC 23-0825 (99.87%), P. jinchanghensis strain LC8190 (99.87%), and P. jinchanghensis strain LC8191 (99.87%). Based on the nucleotide BLAST search of tef1-α sequence, P. jinchanghensis (SDBR-CMU518) showed the closest similarity with P. jinchanghensis strain LC8191 (99.79%), P. jinchanghensis strain LC6636 (99.79%), and P. jinchanghensis strain LC8190 (99.79%).
The combined phylogenetic tree indicated that our new strain (SDBR-CMU518) clustered in the same clade with Pestalotiopsis jinchanghensis and P. zhaoqingensis with 86% ML, 1.00 BYPP statistical support (Figure 2). The nucleotide comparison between our new strain (SDBR-CMU518) and P. jinchanghensis (CGMCC 3.18158, ex-type) showed 0.2% (1/475) base-pair difference in tef1-α (whereas those in ITS and tub2 are identical). The nucleotide comparison between our new strain (SDBR-CMU518) and P. zhaoqingensis (ZHKUCC 23-0825, ex-type) showed 1% (6/563), 0.1% (1/127), and 0.2% (1/447) bp differences in ITS, tef1-α, and tub2. In addition, the nucleotide comparison between P. jinchanghensis (CGMCC 3.18158, ex-type) and P. zhaoqingensis (ZHKUCC 23-0825, ex-type) showed 1.2% (6/500) and 0.2% (1/449) bp differences in ITS and tef1-α (whereas those in tub2 are identical). Pestalotiopsis jinchanghensis was identified as a disease on Camellia sinensis (Liu et al., 2017), and P. zhaoqingensis was isolated from dead unknown plants in China (Dong et al., 2023). Although there were relatively low nucleotide variations between P. jinchanghensis and P. zhaoqingensis, Dong et al. (2023) justified P. zhaoqingensis as distinct species based on morphology, wherein it produced comparatively shorter conidia (17–24 × 4–8 μm vs. 22–32 × 5.5–8.5 μm) and branched apical appendages. Our new strain (SDBR-CMU518) mostly resembles P. jinchanghensis in having unbranched apical appendages (Liu et al., 2017). However, the conidial size of our new isolate showed to be overlapping with both P. jinchanghensis and P. zhaoqingensis (19–27 × 4–6 μm vs. 22–32 × 5.5–8.5 μm vs. 17–24 × 4–8 μm). Based on morphological and phylogenetic lines of evidence as well as an identical nucleotide pairwise, we treated the new strain as a new host record of P. jinchanghensis on Itea and the species is reported from Thailand for the first time, while P. zhaoqingensis is synonymized under P. jinchanghensis herein.
Pseudopestalotiopsis iteae Monkai, Phookamsak, Bhat & S. Lumyong, sp. nov. (Figure 8).
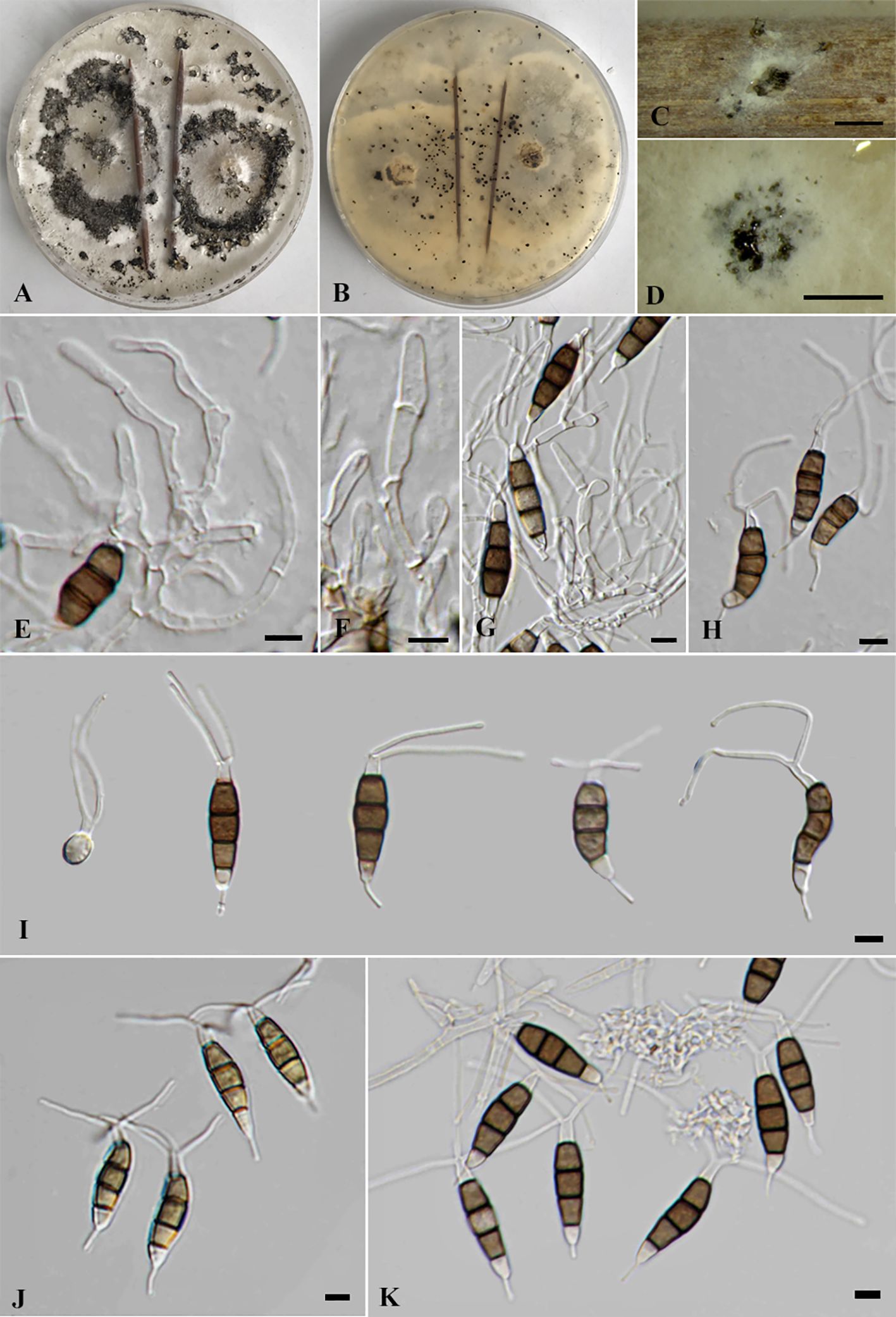
Figure 8. Pseudopestalotiopsis iteae [SDBR-CMU514, ex-type (A–I)], SDBR-CMU523 (J), and SDBR-CMU524 (K). (A) Surface of colonies on PDA. (B) Reverse of colonies on PDA. (C, D) Conidiomata and conidia masses. (E–G) Conidiophores, conidiogenous cells, and conidia. (H–K) Conidia. Scale bars: (C, D) 500 μm and (E–K) 5 μm.
Index Fungorum number: IF902439.
Etymology: Refers to the host genus from which the holotype was collected, Itea.
Endophytic on healthy leaves and roots of Itea riparia. Sexual morph: Undetermined. Asexual morph: Conidiomata up to 500 μm diameter, globose to subglobose, solitary to aggregated, black, semi-immersed on PDA, releasing globose, brown to black, slimy conidial masses. Conidiophores 34–52 × 1.5–3 μm ( = 43 × 2 μm, n = 10), hyaline, septate, branched. Conidiogenous cells 9.5–16.5 × 2–4 μm ( = 12.5 × 3 μm, n = 10), discrete, holoblastic, subcylindrical to ampulliform, hyaline, smooth-walled. Conidia 20–27 × 4.5–7 μm ( = 24 × 6 μm, n = 30), fusiform to ellipsoid, straight to slightly curved in C-form or S-form, 4-septate; basal cell 3–4.5 μm long, obconic to conic, hyaline, thin and smooth-walled; three median cells 12–17 μm long, sometimes hyaline, one to three median cells when immature, brown to dark brown, with thick verruculose walls, constricted at septa, septa and periclinal walls darker than the rest of the cell, concolorous, second cell from base 4–5.5 μm; third cell 4–5.5 μm; fourth cell 4–6.5 μm; apical cell 4–5.5 μm long, hyaline, subcylindrical to obconic; with two to three tubular apical appendages (mostly three), arising from the upper portion of apical cell, filiform, unbranched, knobbed, 18–21 μm long; with a single basal appendages (mostly one), filiform, unbranched, slightly knobbed, 4–7 μm long.
Culture characteristics: Colonies on PDA reached 7 cm diameter after 5 days at 25°C, irregular form, umbonate elevation, crenate edge, whitish, cottony, with dense aerial mycelium on surface, pale brown to white in reverse, Sporulation on PDA at 25°C after 30 days, with numerous black fruiting bodies.
Material examined: THAILAND, Chiang Mai Province, Mae Rim District, on living leaves of Itea riparia (Iteaceae), 10 July 2023, J. Monkai, IT48 (CMUB40071, holotype), ex-type living culture, SDBR-CMU514 = KUNCC24-18921, dried culture permanently preserved in a metabolically inactive state, CMUB40071; ibid., on living leaves of Itea riparia (Iteaceae), 10 July 2023, J. Monkai, IT49, living culture, SDBR-CMU519 = KUNCC24-18922; ibid., on living leaves of Itea riparia (Iteaceae), 10 July 2023, J. Monkai, IT50, living culture, SDBR-CMU520 = KUNCC24-18923; ibid., on living leaves of Itea riparia (Iteaceae), 10 July 2023, J. Monkai, IT61, living culture, SDBR-CMU521 = KUNCC24-18924; ibid., on living leaves of Itea riparia (Iteaceae), 10 July 2023, J. Monkai, IT62, living culture, SDBR-CMU522 = KUNCC24-18925; ibid., on living leaves of Itea riparia (Iteaceae), 10 July 2023, J. Monkai, IT73, living culture, SDBR-CMU523 = KUNCC24-18926; ibid., on living roots of Itea riparia (Iteaceae), 10 July 2023, J. Monkai, IT99, living culture, SDBR-CMU524 = KUNCC24-18927.
GenBank number: SDBR-CMU514; PQ521230 (ITS), PQ560703 (tub2), PQ529181 (tef1-α), SDBR-CMU519; PQ521231 (ITS), PQ560704 (tub2), PQ529182 (tef1-α), SDBR-CMU520; PQ521232 (ITS), PQ560705 (tub2), PQ529183 (tef1-α), SDBR-CMU521; PQ521233 (ITS), PQ560706 (tub2), PQ529184 (tef1-α), SDBR-CMU522; PQ521234 (ITS), PQ560707 (tub2), PQ529185 (tef1-α), SDBR-CMU523; PQ521235 (ITS), PQ560708 (tub2), PQ529186 (tef1-α), SDBR-CMU524; PQ521236 (ITS), PQ560709 (tub2), PQ529187 (tef1-α).
Notes: Based on the nucleotide BLAST search of ITS sequence, Pseudopestalotiopsis iteae sp. nov. (SDBR-CMU514) showed the closest similarity with Pseudopestalotiopsis sp. isolate ERS19.48.Le.D (99.63%), Ps. theae strain KU20018.104 (99.63%), and Pestalotiopsis theae isolate CPO/Pe (99.63%). Based on the nucleotide BLAST search of tub2 sequence, Ps. iteae (SDBR-CMU514) showed the closest similarity with Ps. theae isolate TN07 (98.97%), Ps. theae strain GUCC 23-0406 (99.46%), and Ps. theae strain GUCC 23-0472 (99.86%). Based on the nucleotide BLAST search of tef1-α sequence, Ps. iteae (SDBR-CMU514) showed the closest similarity with Ps. theae strain GUCC 23-0406 (99.60%), Pseudopestalotiopsis sp. 2-KW-2016 strain: 10 (99.60%), and Pseudopestalotiopsis sp. 2-KW-2016 strain: 11 (99.39%).
Phylogenetic tree of combined ITS, tub2, and tef1-α sequence data revealed that Pseudopestalotiopsis iteae (SDBR-CMU514, SDBR-CMU519, SDBR-CMU520, SDBR-CMU521, SDBR-CMU522, SDBR-CMU523, and SDBR-CMU524) are clustered together with Ps. theae strain NTUCC 18-067, with significant support (70% ML, 0.92 BYPP), which are distant from the ex-epitype of Ps. theae (MFLUCC 12-0055) (Figure 3). The nucleotide comparison between Ps. iteae (SDBR-CMU514) and Ps. theae (MFLUCC 12-0055) showed 0.2% (1/491), 0.7% (3/426), and 1.8% (9/493) bp difference in ITS, tub2, and tef1-α (Table 7), respectively. Moreover, the nucleotide differences between the epitype of Ps. theae and other Ps. iteae strains are provided in Table 7. The morphological characteristics of Ps. iteae resemble those of Ps. theae in having brown concolorous median cells, constricted at septa with knobbed apical appendages (Maharachchchikumbura et al., 2013). However, Ps. iteae has narrower conidia (24 × 6 vs. 25.5 × 7.6 μm) and shorter apical appendages (18–21 μm vs. 22.5–31 μm) than Ps. theae (Maharachchikumbura et al., 2013). Thus, we introduced Ps. iteae as a new species based on the differences of nucleotide pairwise comparison of the sufficient gene (tef1-α) and morphological characteristics.
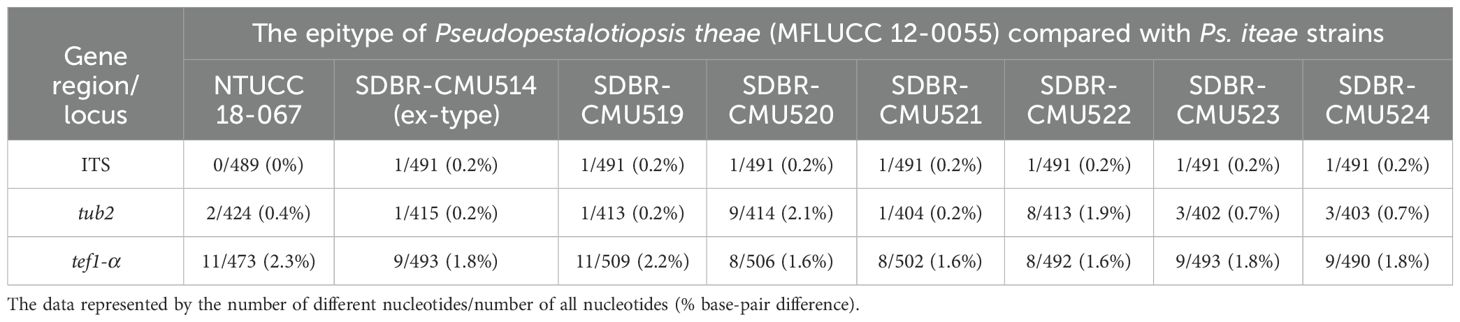
Table 7. Nucleotide differences between the epitype of Pseudopestalotiopsis theae and Ps. iteae strains.
We noticed that the conidial characteristics varied between the strains of Pseudopestalotiopsis iteae. Compared with the type strain, strain SDBR-CMU523 produced shorter conidia with pale brown color (21 × 5 vs. 24 × 6 μm) and shorter appendages without knobbed tips (11–19 vs. 18–21 μm) (Figure 8J), while the strain SDBR-CMU524 has relatively longer conidia that are not constricted at septa (26 × 5 vs. 24 × 6 μm) and longer appendages (15–32 vs. 11–19 μm) than the type strain (Figure 8K). In phylogeny, strain SDBR-CMU524 formed a separated branch to other strains of Ps. iteae (Figure 3). However, there are too few base-pair differences in ITS, tub2, and tef1-α (less than 1%) between the ex-type strain of Ps. iteae (SDBR-CMU514) and strain SDBR-CMU524. The strain SDBR-CMU524 isolated from healthy roots and other strains isolated from healthy leaves of Itea spp. should also be noted. It is indicated that Ps. iteae has also high intraspecific variation, similar to Ps. theae.
Pseudopestalotiopsis theae (≡ Pestalotia theae) was originally isolated from Camellia sinensis in Taiwan, then Maharachchikumbura et al. (2013) designated the epitype of Ps. theae (MFLUCC 12-0055) from a sample collected from the same host in Thailand. Tsai et al. (2021) isolated a strain of Ps. theae (NTUCC 18-067) as a pathogen on C. sinensis in Taiwan, representing it as the reference strain. Our phylogeny demonstrated that this strain is not consistent with Ps. theae and their nucleotide variation of tef1-α is greater (2.3% bp difference) (Table 7), regarding it as Ps. iteae. Also, the ex-epitype strain of Ps. theae (MFLUCC 12-0055) is closely related with Ps. theae strain SC011 and Ps. theae strain MFLUCC 22-0128, which are from Thailand (Maharachchikumbura et al., 2013; 2014; Sun et al., 2023), and Pseudopestalotiopsis sp. strains NBRC112267 and NBRC112268, which are from Myanmar (Nozawa et al., 2017). Thus, these strains could be treated as Ps. theae.
Additionally, we examined the few nucleotide differences of tub2 (less than 1%) between the ex-type of Pseudopestalotiopsis iteae, epitype of Ps. theae, and strain NTUCC 18-067. The tub2 sequence data of those strains lacked the first 300–400 base-pair positions in the alignment of all Pseudopestalotiopsis taxa. This may be caused by the different primers used for PCR amplification of tub2, which are BT2A/BT2B in those studies (Maharachchikumbura et al., 2013; Tsai et al., 2021), while a primer pair, T1/BT2B, was used in our study and other studies (i.e. Norphanphoun et al., 2019; Sun et al., 2023). To clarify this issue, we then amplified our strains with the BT2A/BT2B, and the results did not show any significant nucleotide variation. Thus, the epitype of Ps. theae, strain NTUCC 18-067, and other related strains should be further analyzed for tub2 using T1/BT2B primers as it allows longer base-pair length (up to 800 bp).
4 Discussion
The nomenclature and classification of pestalotioid fungi are remarkably challenging. At the generic level, phylogenetic analyses of combined ITS, tub2, and tef1-α provide sufficient distinction between Neopestalotiopsis, Pestalotiopsis, and Pseudopestalotiopsis (Maharachchikumbura et al., 2014; 2016; Norphanphoun et al., 2019; Sun et al., 2023), whereas the morphological circumscriptions of these genera remain inconclusive (Liu et al., 2017). At the species level, the combination of multi-locus phylogeny, morphology, and ecology has been employed for determining the interspecific variation (Maharachchikumbura et al., 2014; 2016; Tsai et al., 2018; 2021; Chen et al., 2018; Gu et al., 2022). According to their phenotypic plasticity that largely relies on environmental conditions and poor resolution derived from phylogenetic reconstruction, standard criteria for species delineation are still uncertain (Xiong et al., 2022; Guterres et al., 2023; Hsu et al., 2024). Recent studies recovered several undetermined pestalotioid taxa, most of which were not assigned a species name (Jiang et al., 2021; Tsai et al., 2021; Guterres et al., 2023; Hsu et al., 2024; Wang et al., 2024). During our taxonomic investigation of endophytic fungi associated with Itea spp., five Pestalotiopsis-like species were characterized using morphology, nucleotide pairwise comparison, and phylogenetic evidence. Two new species (Neopestalotiopsis iteae and Pseudopestalotiopis iteae) and three hitherto known species (Pestalotiopsis jinchanghensis, N. chrysea, and N. haikouensis) were reported for the first time on this host plant and were discussed accordingly.
The novel species, Neopestalotiopsis iteae, is phylogenetically adjacent to N. cocoës (Figure 1), but it produces constricted septate and longer conidia than the latter species. We also detected a significant nucleotide difference in tef1-α sequence and thus reported it as a new species. Our study pointed out that the phylogenetic placement of N. iteae received poor support value (Figure 1). This phenomenon was also observed in previous studies in which the phylograms of Neopestalotiopsis had unstable topology and mostly contained weak support (Liu et al., 2017; Tsai et al., 2021; Hsu et al., 2024), whereas a new record of N. chrysea was assigned based on the evidence of phylogenetic analyses and nucleotide differences of the sufficient genes. The novel strain (SDBR-CMU516) shared a close phylogenetic relationship with N. chrysea and N. umbrinospora (Figure 1), and it has overlapped conidia and apical appendage characteristics with N. chrysea, whereas N. umbrinospora produces umber-colored and comparatively wider conidia. Owing to the low nucleotide difference of ITS, tub2, and tef1-α sequences (below 1%) found among these strains, we synonymized N. umbrinospora under N. chrysea. Another new record of Neopestalotiopsis was described; the strain SDBR-CMU517 was phylogenetically located in the same clade with N. cercidicola, N. terricola, and N. haikouensis (Figure 1). The nucleotide comparison of ITS, tub2, and tef1-α sequence among those strains was insignificant (lower than 1%) (Table 5). Moreover, the strains of N. terricola showed high variation of conidial dimensions and color intensities on different host substrates (Table 6). We also observed this phenotypic variation of conidia in the new strain (Figure 5G). Therefore, we designated our strain as N. haikouensis and synonymized N. terricola and N. cercidicola under N. haikouensis based on phylogenetic and morphological congruency.
Moreover, a new isolate of Pestalotiopsis was reported; the strain SDBR-CMU518 has a close phylogenetic relationship with P. jinchanghensis and P. zhaoqingensis (Figure 2). The conidial morphology of this isolate is more similar to the ex-type of P. jinchanghensis, whose apical appendages are not branched, whereas P. zhaoqingensis has branched apical appendages and relatively shorter conidia. Based on their nucleotide differences being not significant (below 1.5%) coupled with phylogenetic data, we considered our new strain to belong to P. jinchanghensis and synonymized P. zhaoqingensis under P. jinchanghensis.
Another new species described in this study is Pseudopestalotiopsis iteae, which is characterized by the production of conidia with brown concolorous median cells, constricted at septa with spatulate apical appendage. The novel strains (SDBR-CMU514, SDBR-CMU519, SDBR-CMU520, SDBR-CMU521, SDBR-CMU522, SDBR-CMU523, and SDBR-CMU524) constitute a distinct clade and sister to Ps. theae. Furthermore, the nucleotide variation of tef1-α among the ex-type strains of Ps. theae and Ps. iteae was greater than 1.5% (Table 7), validating it as a new species based on the recommendation of Jeewon and Hyde (2016) and Chethana et al. (2021). Notably, some strains of Ps. iteae exhibited highly variable conidial shapes and sizes (Figures 8I–K), which were also observed in Ps. theae strains.
In summary, the three-locus phylogeny (ITS, tub2, and tef1-α) does not provide a strong support for species boundaries of pestalotioid fungi. Hence, the morphological characteristics and nucleotide polymorphisms were herein applied to elucidate their interspecific differences. The morphological characteristics of pestalotioid fungi are always similar and difficult to distinguish from each other. Likewise, their morphological characteristics showed a high intraspecific variation under different conditions of fungal growth media, hosts, temperatures, and other environmental factors (personal observation). Thus, it is unreliable to define new pestaloioid species using host, culture, and morphological comparisons. The significant phenotypic and genetic variations among pestalotioid species and strains have been addressed in recent studies (Norphanphoun et al., 2019; Li et al., 2022; Sun et al., 2023; Koodalugodaarachchi et al., 2024), concurring with the present study. Our phylogeny also contained unclear phylogenetic lineages including Neopestalotiopsis chrysea, N. haikouensis, Pestalotiopsis jinchanghensis, Pseudopestalotiopsis theae, and Ps. iteae, representing them as cryptic species. Therefore, further collections and more cultures are required for the reevaluation of intra- and interspecific relationships among these species. Moreover, additional informative loci, such as rpb2, and whole genome analysis are absolutely needed to evaluate the better taxonomic resolution of this fungal group.
The majority of pestalotioid taxa have been reported as plant pathogens (Maharachchikumbura et al., 2011; 2014; Peng et al., 2022; Giri and Pradhan, 2023; Hsu et al., 2024). Likewise, the species identified in this study—Neopestalotiopsis chrysea, N. haikouensis, and Pestalotiopsis jinchanghensis—were previously documented as causative agents of disease in significant plants (Liu et al., 2017; Shi et al., 2017; Jiang et al., 2021; Wu et al., 2021; Li et al., 2022; Zhang et al., 2022; 2024). Notably, some species exhibited distinct life modes including endophytes, saprobes, and phytopathogens. For example, N. chrysea was initially found as saprobes on unknown plants (Maharachchikumbura et al., 2012; 2014) and later reported as pathogens on blueberry and pecan in China (Shi et al., 2017; Wu et al., 2021). Pestalotiopsis jinchanghensis was introduced by Liu et al. (2017) as tea pathogen and was recently found as saprobes on unknown plants in China (Dong et al., 2023). Moreover, some species have a broad range of host association; for example, N. haikouensis could infect various plant taxa, such as Castanea mollissima, Cercis chinensis, Ilex chinensis, Paeonia suffruticosa, and Olea europaea (Jiang et al., 2021; Li et al., 2022; Zhang et al., 2022; 2024). These studies emphasize their abilities of lifestyle shift and host adaptation. Since we discovered endophytic pestalotioid fungi from Itea, further studies should be conducted to determine their pathogenicity as they may be a potential source of diseases.
To date, few fungi on the Itea species have been recorded (Farr et al., 2021), including only two pestalotioid species (Pestalotiopsis acacia on Itea chinensis var. oblonga from China and P. gracilis on I. oldhamii from Japan). Our study indicates that Itea contains a significant diversity of pestalotioid species. Moreover, we have observed that distinct pestalotioid species seem to prefer responsive tissues of Itea. Most strains of Pseudopestalotiopsis were found on leaves of I. riparia. Most Pestalotiopsis and Neopestalotiopsis species were found on stems of I. japonica and I. riparia. Only one species of Pseudopestalotiopsis and one Neopestalotiopsis species were found on roots of I. riparia. Our findings enhance the knowledge of taxonomic diversity of pestalotioid fungi in Thailand and highlight that Itea spp. could offer a high degree of undetermined fungal diversity. Since Itea is a significant source of rare sugar such as D-allulose, future studies are needed to examine the new endophytic fungal strains for the biosynthesis of rare sugar and other secondary metabolites. This would provide useful applications for these novel microbial resources in the pharmaceutical and food industries as well as in sustainable agriculture.
Data availability statement
The datasets presented in this study can be found in online repositories. The names of the repository/repositories and accession number(s) can be found below: https://www.ncbi.nlm.nih.gov/genbank/ ITS: PQ521226, PQ521227, PQ521228, PQ521229, PQ521230, PQ521231, PQ521232, PQ521233, PQ521234, PQ521235, PQ521236; tub2: PQ560699, PQ560700, PQ560701, PQ560702, PQ560703, PQ560704, PQ560705, PQ560706, PQ560707, PQ560708, PQ560709; tef1-α: PQ529177, PQ529178, PQ529179, PQ529180, PQ529181, PQ529182, PQ529183, PQ529184, PQ529185, PQ529186, PQ529187.
Author contributions
JM: Conceptualization, Data curation, Formal Analysis, Investigation, Methodology, Project administration, Writing – original draft, Writing – review & editing. RP: Conceptualization, Data curation, Funding acquisition, Investigation, Methodology, Project administration, Writing – original draft, Writing – review & editing. DB: Investigation, Writing – original draft, Writing – review & editing. TE: Investigation, Methodology, Writing – review & editing. JX: Funding acquisition, Supervision, Writing – review & editing. SL: Conceptualization, Funding acquisition, Supervision, Writing – review & editing.
Funding
The author(s) declare that financial support was received for the research and/or publication of this article. This study was supported by the CMU Proactive Researcher program, Chiang Mai University, Chiang Mai, Thailand (grant number 814/2567).
Acknowledgments
Jutamart Monkai gratefully acknowledges Chiang Mai University for providing financial support and laboratory facilities. The authors extend their gratitude to the Biology Experimental Center, Germplasm Bank of Wild Species, Kunming Institute of Botany, Chinese Academy of Sciences for providing the facilities of molecular laboratory. Assist. Prof. Dr. Verasak Sahachaisaree is thanked for his valuable help with sample collection. Dr. Shaun Pennycook is thanked for the nomenclatural review. Rungtiwa Phookamsak sincerely acknowledges the Introducing Talents Start-up Fund of Kunming Institute of Botany, Chinese Academy of Sciences, Yunnan Revitalization Talent Support Program “Young Talent” Project (grant no. YNWR-QNBJ-2020-120), Yunnan Revitalization Talent Support Program: High-end Foreign Expert Project, Independent Research of Department of Economic Plants and Biotechnology, Yunnan Key Laboratory for Wild Plant Resources, Kunming Institute of Botany, Chinese Academy of Sciences (grant no. Y537731261), and Yunnan Provincial Department of Human Resources and Social Security, Yunnan Province Foreign Expert Project (project no. 202505AO120002). Jianchu Xu thanks Yunnan Department of Sciences and Technology of China (grant nos. 202302AE090023 and 202303AP140001). D. Jayarama Bhat gratefully acknowledges the financial support provided under the Distinguished Scientist Fellowship Programme (DSFP) at King Saud University, Riyadh, Saudi Arabia.
Conflict of interest
The authors declare that the research was conducted in the absence of any commercial or financial relationships that could be construed as a potential conflict of interest.
Generative AI statement
The author(s) declare that no Generative AI was used in the creation of this manuscript.
Publisher’s note
All claims expressed in this article are solely those of the authors and do not necessarily represent those of their affiliated organizations, or those of the publisher, the editors and the reviewers. Any product that may be evaluated in this article, or claim that may be made by its manufacturer, is not guaranteed or endorsed by the publisher.
References
Ayers, B. J., Hollinshead, J., Saville, A. W., Nakagawa, S., Adachi, I., Kato, A., et al. (2014). Iteamine, the first alkaloid isolated from Itea virginica L. inflorescence. Phytochemistry 100, 126–131. doi: 10.1016/j.phytochem.2014.01.012
Carbone, I., Kohn, L. M. (1999). A method for designing primer sets for speciation studies in filamentous ascomycetes. Mycologia 91, 553–556. doi: 10.1080/00275514.1999.12061051
Chen, Y., Zeng, L., Shu, N., Jiang, M., Wang, H., Huang, Y., et al. (2018). Pestalotiopsis-like species causing gray blight disease on Camellia sinensis in China. Plant Dis. 102, 98–106. doi: 10.1094/PDIS-02-19-0264-RE
Chethana, K. T., Manawasinghe, I. S., Hurdeal, V. G., Bhunjun, C. S., Appadoo, M. A., Gentekaki, E., et al. (2021). What are fungal species and how to delineate them? Fungal Divers. 109, 1–25. doi: 10.1007/s13225-021-00483-9
Diogo, E., Gonçalves, C. I., Silva, A. C., Valente, C., Bragança, H., Phillips, A. J. (2021). Five new species of Neopestalotiopsis associated with diseased Eucalyptus spp. in Portugal. Mycol. Progr. 20, 1441–1456. doi: 10.1007/s11557-021-01741-5
Dong, W., Hyde, K. D., Jeewon, R., Liao, C. F., Zhao, H. J., Kularathnage, N. D., et al. (2023). Mycosphere notes 449–468: Saprobic and endophytic fungi in China, Thailand, and Uzbekistan. Mycosphere 14, 2208–2262. doi: 10.5943/mycosphere/14/1/26
Farr, D. F., Rossman, A. Y., Castlebury, L. A. (2021). United states national fungus collections fungus-host dataset. Ag. Data Commons. doi: 10.15482/USDA.ADC/1524414
Feng, Y., Jian, J., Cheng, L., Luo, G., Yang, W. (2024). Two new compounds isolated from the Itea omeiensis and their anti-oxidant activities. Chem. Biodivers. 21, e202301881. doi: 10.1002/cbdv.202301881
Fiorenza, A., Gusella, G., Aiello, D., Polizzi, G., Voglmayr, H. (2022). Neopestalotiopsis siciliana sp. nov. and N. rosae causing stem lesion and dieback on avocado plants in Italy. J. Fungi. 8, 562. doi: 10.3390/jof8060562
Gao, Y., Zhong, T., Wanasinghe, D. N., Eungwanichayapant, P. D., Jayawardena, R. S., Hyde, K. D., et al. (2024). Phlyctema yunnanensis (Dermateaceae, Helotiales), a novel species from herbaceous plants in grassland ecosystems of Yunnan, China. Stud. Fungi. 9, e019. doi: 10.48130/sif-0024-0020
Giri, S., Pradhan, P. (2023). Notes on phytopathogenic fungi reported from Sikkim, India and their broad inter-taxa affinities to plant hosts as inferred from data mining. Stud. Fungi. 8, 8. doi: 10.48130/SIF-2023-0008
Glass, N. L., Donaldson, G. C. (1995). Development of primer sets designed for use with the PCR to amplify conserved genes from filamentous ascomycetes. Appl. Environ. Microbiol. 61, 1323–1330. doi: 10.1128/aem.61.4.1323-1330.1995
Gu, R., Bao, D. F., Shen, H. W., Su, X. J., Li, Y. X., Luo, Z. L. (2022). Endophytic Pestalotiopsis species associated with Rhododendron in Cangshan Mountain, Yunnan Province, China. Front. Microbiol. 13. doi: 10.3389/fmicb.2022.1016782
Guterres, D. C., Silva, M. A., Martins, M. D., Azevedo, D. M. Q., Lisboa, D. O., Pinho, D. B., et al. (2023). Leaf spot caused by Neopestalotiopsis species on Arecaceae in Brazil. Australas. Plant Pathol. 52, 47–62. doi: 10.1007/s13313-022-00893-6
Hall, T. A. (1999). BioEdit: a user-friendly biological sequence alignment editor and analysis program for Windows 95/98/NT. Nucleic Acids Symp. Ser. 41, 95–98. doi: 10.14601/Phytopathol_Mediterr-14998u1.29
Hsu, S. Y., Xu, Y. C., Lin, Y. C., Chuang, W. Y., Lin, S. R., Stadler, M., et al. (2024). [amp]]#xFEFF; Hidden diversity of Pestalotiopsis and Neopestalotiopsis (Amphisphaeriales, Sporocadaceae) species allied with the stromata of entomopathogenic fungi in Taiwan. MycoKeys 101, 275. doi: 10.3897/mycokeys.101.113090
Huelsenbeck, J. P., Ronquist, F. (2001). MRBAYES: Bayesian inference of phylogenetic trees. Bioinformatics 17, 754–755. doi: 10.1093/bioinformatics/17.8.754
Hyde, K. D., Hongsanan, S., Jeewon, R., Bhat, D. J., Mckenzie, E. H. C., Jones, E. B. G., et al. (2016). Fungal diversity notes 367–490: taxonomic and phylogenetic contributions to fungal taxa. Fungal Divers. 80, 1–270. doi: 10.1007/s13225-016-0373-x
Jeewon, R., Hyde, K. D. (2016). Establishing species boundaries and new taxa among fungi: recommendations to resolve taxonomic ambiguities. Mycosphere 7, 1669–1677. doi: 10.5943/mycosphere/7/11/4
Jiang, N., Fan, X., Tian, C. (2021). Identification and characterization of leaf-inhabiting fungi from Castanea plantations in China. J. Fungi. 7, 64. doi: 10.3390/jof7010064
Katoh, K., Rozewicki, J., Yamada, K. D. (2019). MAFFT online service: multiple sequence alignment, interactive sequence choice and visualization. Brief. Bioinform. 20, 1160–1166. doi: 10.1093/bib/bbx108
Koodalugodaarachchi, V., Chethana, K. T., Jayawardena, R. S., Bundhun, D., Aluthmuhandiram, J. V., Suwannarach, N., et al. (2024). New records of Pestalotioid species associated with leaf spot disease on Camellia sinensis from northern Thailand. Phytotaxa 647, 91–114. doi: 10.11646/phytotaxa.647.1.5
Lang, T. Q., Luo, G. Y., Pu, W. C., Wang, Z. W., Wang, J., Tian, X. L., et al. (2022). Three new 3-formyl-2-arylbenzofurans from Itea yunnanensis and their anti-hepatocellular carcinoma effects. Nat. Prod. Res. 36, 1205–1214. doi: 10.1080/14786419.2020.1867130
Li, W. L., Dissanayake, A. J., Zhang, T., Maharachchikumbura, S. S., Liu, J. K. (2022). Identification and pathogenicity of pestalotiod fungi associated with woody oil plants in Sichuan province, China. J. Fungi. 8, 1175. doi: 10.3390/jof8111175
Liu, F., Hou, L., Raza, M., Cai, L. (2017). Pestalotiopsis and allied genera from Camellia, with description of 11 new species from China. Sci. Rep. 7, 866. doi: 10.1038/s41598-017-00972-5
Luo, G., Zhou, M., Liu, Y., Ye, Q., Gu, J., Huang, T., et al. (2014). 3-Formyl-2-arylbenzofurans from the aerial parts of Itea ilicifolia. Phytochem. Lett. 10, 19–22. doi: 10.1016/j.phytol.2014.06.019
Maharachchikumbura, S. S., Chukeatirote, E., Guo, L. D., Crous, P. W., Mckenzie, E. H., Hyde, K. D. (2013). Pestalotiopsis species associated with Camellia sinensis (tea). Mycotaxon 123, 47–61. doi: 10.5248/123.47
Maharachchikumbura, S. S., Guo, L. D., Cai, L., Chukeatirote, E., Wu, W. P., Sun, X., et al. (2012). A multi-locus backbone tree for Pestalotiopsis, with a polyphasic characterization of 14 new species. Fungal Divers. 56, 95–129. doi: 10.1007/s13225-012-0198-1
Maharachchikumbura, S. S., Guo, L. D., Chukeatirote, E., Bahkali, A. H., Hyde, K. D. (2011). Pestalotiopsis—morphology, phylogeny, biochemistry and diversity. Fungal Divers. 50, 167–187. doi: 10.1007/s13225-011-0125-x
Maharachchikumbura, S. S., Guo, L. D., Liu, Z. Y., Hyde, K. D. (2016). Pseudopestalotiopsis ignota and Ps. camelliae spp. nov. associated with grey blight disease of tea in China. Mycol. Prog. 15, 1–7. doi: 10.1007/s11557-016-1162-3
Maharachchikumbura, S. S., Hyde, K. D., Groenewald, J. Z., Xu, J., Crous, P. W. (2014). Pestalotiopsis revisited. Stud. Mycol. 79, 121–186. doi: 10.1016/j.simyco.2014.09.005
Mattoo, A. J., Nonzom, S. (2022). Investigating diverse methods for inducing sporulation in endophytic fungi. Stud. Fungi. 7, 1–10. doi: 10.48130/SIF-2022-0016
Miller, M. A., Pfeiffer, W., Schwartz, T. (2010). “Creating the CIPRES Science Gateway for inference of large phylogenetic trees,” in 2010 gateway computing environments workshop (GCE) (New Orleans, LA, USA: IEEE (Institute of Electrical and Electronics Engineers)), 1–8. doi: 10.1109/GCE.2010.5676129
Monteiro, P., Gonçalves, M. F., Pinto, G., Silva, B., Martín-García, J., Diez, J. J., et al. (2022). Three novel species of fungi associated with pine species showing needle blight-like disease symptoms. Eur. J. Plant Pathol. 162, 183–202. doi: 10.1007/s10658-021-02395-5
Norphanphoun, C., Jayawardena, R. S., Chen, Y., Wen, T. C., Meepol, W., Hyde, K. D. (2019). Morphological and phylogenetic characterization of novel pestalotioid species associated with mangroves in Thailand. Mycosphere 10, 531–578. doi: 10.5943/mycosphere/10/1/9
Nozawa, S., Ando, K., Phay, N., Watanabe, K. (2018). Pseudopestalotiopsis dawaina sp. nov. and Ps. kawthaungina sp. nov.: two new species from Myanmar. Mycol. Prog. 17, 865–870. doi: 10.1007/s11557-018-1398-1
Nozawa, S., Yamaguchi, K., Le, T. H. Y., Van Hop, D., Phay, N., Ando, K., et al. (2017). Identification of two new species and a sexual morph from the genus Pseudopestalotiopsis. Mycoscience 58, 328–337. doi: 10.1007/BF02464139
Nylander, J. A. (2004). MrModeltest 2. Program Distributed by the Author (Uppsala, Sweden: Department of Systematic Zoology; Evolutionary Biology Centre, Uppsala University).
O’Donnell, K., Cigelnik, E. (1997). Two divergent intragenomic rDNA ITS2 types within a monophyletic lineage of the fungus Fusarium are nonorthologous. Mol. Phylogenet. Evol. 7, 103–116. doi: 10.1006/mpev.1996.0376
O’Donnell, K., Kistler, H. C., Cigelnik, E., Ploetz, R. C. (1998). Multiple evolutionary origins of the fungus causing Panama disease of banana: concordant evidence from nuclear and mitochondrial gene genealogies. Proc. Natl. Acad. Sci. 95, 2044–2049. doi: 10.1073/pnas.95.5.2044
Palwe, S. D., Borde, M. Y., Sonawane, H. B. (2021). Endophytic fungi: a source of potential anticancer compounds. Stud. Fungi. 6, 188–203. doi: 10.1016/j.crphar.2021.100050
Peng, C., Crous, P. W., Jiang, N., Fan, X. L., Liang, Y. M., Tian, C. M. (2022). Diversity of sporocadaceae (pestalotioid fungi) from rosa in China. Pers.: Mol. Phylogeny. Evol. Fungi. 49, 201–260. doi: 10.3767/persoonia.2022.49.07
POWO (2024). Plants of the World Online (Kew: Royal Botanic Gardens). Available at: http://www.plantsoftheworldonline.org/ (Accessed November 01, 2024).
Prasannath, K., Shivas, R. G., Galea, V. J., Akinsanmi, O. A. (2021). Neopestalotiopsis species associated with flower diseases of Macadamia integrifolia in Australia. J. Fungi. 7, 771. doi: 10.3390/jof7090771
Rambaut, A., Drummond, A. J. (2012). FigTree: Tree Figure Drawing Tool (Edinburgh, UK: Institute of Evolutionary Biology, University of Edinburgh).
Rambaut, A., Drummond, A. J., Xie, D., Baele, G., Suchard, M. A. (2018). Posterior summarization in Bayesian phylogenetics using Tracer 1.7. Syst. Biol. 67, 901–904. doi: 10.1093/sysbio/syy032
Razaghi, P., Raza, M., Han, S. L., Ma, Z. Y., Cai, L., Zhao, P., et al. (2024). Sporocadaceae revisited. Stud. Mycol. 109, 155–272. doi: 10.3114/sim.2024.109.03
Ronquist, F., Teslenko, M., van der Mark, P., Ayres, D. L., Darling, A., Höhna, S., et al. (2012). MrBayes 3.2: efficient Bayesian phylogenetic inference and model choice across a large model space. Syst. Biol. 61, 539–542. doi: 10.1093/sysbio/sys029
Shi, L. B., Li, Y., Fei, N. Y., Fu, J. F., Yan, X. R. (2017). First report of Neopestalotiopsis chrysea causing twig dieback of rabbiteye blueberry (Vaccinium ashei) in China. Plant Dis. 101, 506–506. doi: 10.1094/PDIS-09-16-1282-PDN
Song, Z. Q., Zhang, B. Y. (2024). [amp]]#xFEFF; Taxonomic notes on the genus Itea (Iteaceae). PhytoKeys 239, 59. doi: 10.3897/phytokeys.239.117851
Stamatakis, A. (2014). RAxML version 8: A tool for phylogenetic analysis and post-analysis of large phylogenies. Bioinformatics 30, 1312–1313. doi: 10.1093/bioinformatics/btu033
Strobel, G., Yang, X., Sears, J., Kramer, R., Sidhu, R. S., Hess, W. M. (1996). Taxol from Pestalotiopsis microspora, an endophytic fungus of Taxus wallachiana. Microbiology 142, 435–440. doi: 10.1099/13500872-142-2-435
Sun, Y. R., Jayawardena, R. S., Sun, J. E., Wang, Y. (2023). Pestalotioid species associated with medicinal plants in southwest China and Thailand. Microbiol. Spectr. 11, e03987–e03922. doi: 10.1128/spectrum.03987-22
Tsai, I., Chung, C. L., Lin, S. R., Hung, T. H., Shen, T. L., Hu, C. Y., et al. (2021). Cryptic diversity, molecular systematics, and pathogenicity of genus Pestalotiopsis and allied genera causing gray blight disease of tea in Taiwan, with a description of a new Pseudopestalotiopsis species. Plant Dis. 105, 425–443. doi: 10.1094/PDIS-05-20-1134-RE
Tsai, I., Maharachchikumbura, S. S., Hyde, K. D., Ariyawansa, H. A. (2018). Molecular phylogeny, morphology and pathogenicity of Pseudopestalotiopsis species on Ixora in Taiwan. Mycol. Prog. 17, 941–952. doi: 10.1007/s11557-018-1404-7
Wang, Y., Tsui, K. M., Chen, S., You, C. (2024). [amp]]#xFEFF; Diversity, pathogenicity and two new species of pestalotioid fungi (Amphisphaeriales) associated with Chinese Yew in Guangxi, China. MycoKeys 102, 201. doi: 10.3897/mycokeys.102.113696
White, T. J., Bruns, T., Lee, S. J. W. T., Taylor, J. W. (1990). “Amplification and direct sequencing of fungal ribosomal RNA genes for phylogenetics,” in PCR protocols: a guide to methods and applications, vol. 18 . Eds. Innes, M. A., Gelfand, D. H., Sninsky, J. J., White, T. J. (Academic Press, San Diego, CA, USA), 315–322.
Wu, F., Kong, W. L., Zhang, Y., Wu, T. Y., Sun, X. R., Wu, X. Q. (2021). First report of leaf spot disease caused by Neopestalotiopsis chrysea on Carya illinoinensis in China. Plant Dis. 105, 221. doi: 10.1094/PDIS-05-20-1125-PDN
Wu, C., Wang, Y., Yang, Y. (2022). Pestalotiopsis diversity: Species, dispositions, secondary metabolites, and bioactivities. Molecules 27, 8088. doi: 10.3390/molecules27228088
Xiong, Y. R., Manawasinghe, I. S., Maharachchikumbura, S. S. N., Lu, L., Dong, Z. Y., Xiang, M. M., et al. (2022). Pestalotioid species associated with palm species from Southern China. Curr. Res. Environ. Appl. Mycol. 12, 285–321. doi: 10.5943/cream/12/1/18
Xu, J., Ebada, S. S., Proksch, P. (2010). Pestalotiopsis a highly creative genus: chemistry and bioactivity of secondary metabolites. Fungal Divers. 44, 15–31. doi: 10.1007/s13225-010-0055-z
Zeng, Y., Dou, D., Zhang, Y., Zhang, L., Sun, Y. (2015). Rare sugars and antioxidants in Itea virginica, Itea oblonga Hand.-Mazz., and Itea yunnanensis Franch leaves. Int. J. Food Prop. 18, 2549–2560. doi: 10.1080/10942912.2014.917099
Zhang, W., Li, Y., Lin, L., Jia, A., Fan, X. (2024). Updating the species diversity of pestalotioid fungi: four new species of Neopestalotiopsis and Pestalotiopsis. J. Fungi. 10, 475. doi: 10.3390/jof10070475
Zhang, Z., Liu, R., Liu, S., Mu, T., Zhang, X., Xia, J. (2022). [amp]]#xFEFF; Morphological and phylogenetic analyses reveal two new species of Sporocadaceae from Hainan, China. MycoKeys 88, 171. doi: 10.3897/mycokeys.88.82229
Zhaxybayeva, O., Gogarten, J. P. (2002). Bootstrap, Bayesian probability and maximum likelihood mapping: exploring new tools for comparative genome analyses. BMC Genomics 3, 1–15. doi: 10.1186/1471-2164-3-4
Keywords: Neopestalotiopsis, Pestalotiopsis, polyphasic taxonomic approach, Pseudopestalotiopsis, Sporocadaceae
Citation: Monkai J, Phookamsak R, Bhat DJ, Ei TSZ, Xu J and Lumyong S (2025) Novel endophytic pestalotioid species associated with Itea in Thailand. Front. Cell. Infect. Microbiol. 15:1532712. doi: 10.3389/fcimb.2025.1532712
Received: 22 November 2024; Accepted: 26 February 2025;
Published: 03 April 2025.
Edited by:
Ahmed M. Abdel-Azeem, Suez Canal University, EgyptReviewed by:
Danushka Sandaruwan Tennakoon, Shenzhen University, ChinaHiran Kanti Santra, Vidyasagar University, India
Copyright © 2025 Monkai, Phookamsak, Bhat, Ei, Xu and Lumyong. This is an open-access article distributed under the terms of the Creative Commons Attribution License (CC BY). The use, distribution or reproduction in other forums is permitted, provided the original author(s) and the copyright owner(s) are credited and that the original publication in this journal is cited, in accordance with accepted academic practice. No use, distribution or reproduction is permitted which does not comply with these terms.
*Correspondence: Saisamorn Lumyong, c2Nib2kwMDlAZ21haWwuY29t; Rungtiwa Phookamsak, cGhvb2thbXNha0BtYWlsLmtpYi5hYy5jbg==