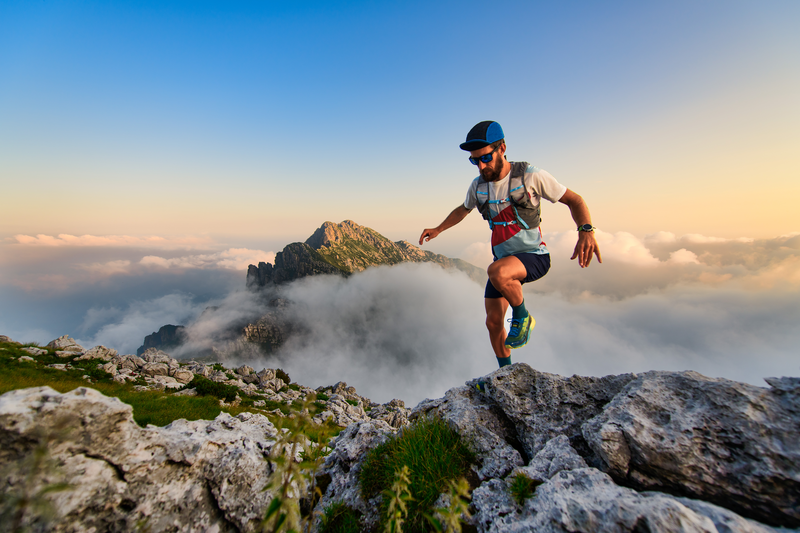
94% of researchers rate our articles as excellent or good
Learn more about the work of our research integrity team to safeguard the quality of each article we publish.
Find out more
REVIEW article
Front. Cell. Infect. Microbiol.
Sec. Intestinal Microbiome
Volume 15 - 2025 | doi: 10.3389/fcimb.2025.1521754
This article is part of the Research Topic Influence of Maternal Dietary Patterns on Offspring Gut Microbiome and Immunity View all 3 articles
The final, formatted version of the article will be published soon.
You have multiple emails registered with Frontiers:
Please enter your email address:
If you already have an account, please login
You don't have a Frontiers account ? You can register here
The gut microbiota is the collective term for the microorganisms that reside in the human gut. In recent years, advances in sequencing technology and bioinformatics gradually revealed the role of gut microbiota in human health. Dramatic changes in the gut microbiota occur during pregnancy due to hormonal and dietary changes, and these changes have been associated with certain gestational diseases such as preeclampsia (PE) and gestational diabetes mellitus (GDM). Modulation of gut microbiota has also been proposed as a potential treatment for these gestational diseases. The present article aims to review current reports on the association between gut microbiota and gestational diseases, explore possible mechanisms, and discuss the potential of probiotics in gestational diseases. Uncovering the link between gut microbiota and gestational diseases could lead to a new therapeutic approach.
Keywords: Gut Microbiota, Preeclampsia, Insulin Resistance, Probiotics, postpartum depression, gestational diabetes mellitus
Received: 02 Nov 2024; Accepted: 20 Feb 2025.
Copyright: © 2025 Xie, Chen, Shan, Pan and Hu. This is an open-access article distributed under the terms of the Creative Commons Attribution License (CC BY). The use, distribution or reproduction in other forums is permitted, provided the original author(s) or licensor are credited and that the original publication in this journal is cited, in accordance with accepted academic practice. No use, distribution or reproduction is permitted which does not comply with these terms.
* Correspondence:
Yayi Hu, West China Second University Hospital, Sichuan University, Chengdu, China
Disclaimer: All claims expressed in this article are solely those of the authors and do not necessarily represent those of their affiliated organizations, or those of the publisher, the editors and the reviewers. Any product that may be evaluated in this article or claim that may be made by its manufacturer is not guaranteed or endorsed by the publisher.
Research integrity at Frontiers
Learn more about the work of our research integrity team to safeguard the quality of each article we publish.