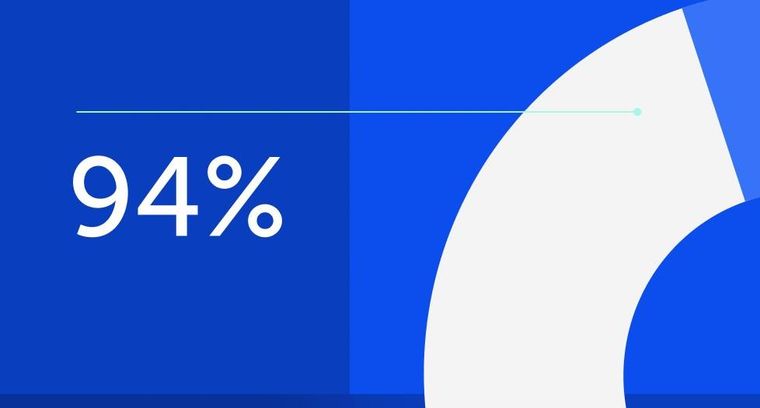
94% of researchers rate our articles as excellent or good
Learn more about the work of our research integrity team to safeguard the quality of each article we publish.
Find out more
REVIEW article
Front. Cell. Infect. Microbiol., 01 April 2025
Sec. Clinical Microbiology
Volume 15 - 2025 | https://doi.org/10.3389/fcimb.2025.1512891
The traditional view of sterile urine has been challenged by advancements in next-generation sequencing, revealing that the urinary microbiome significantly influences individual health and various urinary system diseases. Urinary tract infections in patients with neurogenic bladder are highly prevalent, recurrent, and lifelong. If frequent urinary tract infections are not adequately managed, they may ultimately lead to chronic renal failure. The excessive use of antibiotics to prevent and treat urinary tract infections may lead to increased bacterial resistance, limiting future therapeutic options. This review summarizes commonly used microbiome research techniques and urine collection methods, compiles current studies on the urinary microbiome in neurogenic bladder patients, and discusses the potential implications of urinary microbiome composition for preventing, diagnosing, and treating urinary tract infections. By summarizing current research findings, we aim to enhance understanding of the urinary microbiome in neurogenic bladder patients and promote the standardization and clinical translation of microbiome research.
Neurogenic bladder (NB) refers to a range of lower urinary tract dysfunctions caused by neurological disorders, manifesting as urinary incontinence, urinary retention, or a combination of both (Amarenco et al., 2017). It severely impacts the patient’s quality of life and causes embarrassment, discomfort, and social isolation (Alghamdi et al., 2023). In addition to the inability to control urination, NB can result in severe complications such as urinary tract infections (UTIs), urinary tract stones, vesicoureteral reflux, and autonomic dysreflexia (Ginsberg et al., 2021). The clinical presentation of NB depends on the location of the neurological lesion and its relationship with the central nervous system (Figure 1) (Kumar and Biswas, 2022). The most common causes of NB include spinal cord injury (SCI), multiple sclerosis, Parkinson’s disease, and cerebrovascular disease (Panicker, 2020).
Lower urinary tract dysfunction is the core manifestation of NB and an important trigger for recurrent urinary tract infections (rUTIs). Chronic ischemia of the bladder wall caused by lower urinary tract pressure, bladder mucosal damage caused by overdistension, and frequent catheterization collectively constitute the most challenging clinical complication of rUTIs (Linsenmeyer, 2018). NB Patients have their urinary systems constantly exposed to a high-risk environment for bacterial colonization, significantly increasing the occurrence rate of rUTIs (Milicevic et al., 2024). Recurrent infections exacerbate underlying symptoms such as frequency, urgency, and incontinence, further damaging the patient’s quality of life. The continuous invasion by pathogens and the synergistic effect of high bladder pressure increases the risk of upper urinary tract injury. Studies indicate that the prevalence of chronic kidney disease in NB patients is more than three times higher than in the general population, and chronic renal failure is often the outcome of this pathological process (Kari, 2006; Sung et al., 2018).
Treatment strategies for NB include conservative approaches (fluid management, pelvic floor muscle exercises, clean intermittent catheterization, and indwelling catheters), pharmacological treatments (anticholinergic drugs and alpha blockers), non-pharmacological interventions (sacral nerve stimulation and acupuncture), and surgical options (botulinum toxin injections, augmentation cystoplasty, and continent cutaneous urinary diversion) (DeWitt-Foy and Elliott, 2022). In recent years, significant advancements have been made in the management of NB and the prevention of its complications (Koukourikis et al., 2023), such as the use of mesenchymal stem cells to improve impaired bladder function and the application of urinary interleukin-6 to distinguish asymptomatic bacteriuria (ASB) from non-febrile UTIs (Sundén et al., 2017; Chen et al., 2022).
Despite substantial progress in the diagnostic methods and therapeutic strategies for NB and its urological complications, there remains an unmet clinical need for early diagnostic tools and novel effective treatments for UTIs in NB patients. Research into the human microbiome has provided valuable perspectives on the microbial composition of the urinary system and its changes under pathological conditions, potentially aiding in the development of novel prevention, diagnostic, and treatment strategies. Studies on gut microbiota have demonstrated that microbial communities and their metabolites interact with the human immune system and various diseases, such as inflammatory bowel disease, irritable bowel syndrome, colorectal cancer, and neurodegenerative disorders (Sebastián Domingo and Sánchez Sánchez, 2018; Goyal et al., 2021; Si et al., 2021). Similarly, the urinary microbiome is closely related to urinary system diseases, including UTIs, overactive bladder, bladder cancer, interstitial cystitis, etc (Lee et al., 2020).
This review summarizes commonly used techniques for urinary microbiome research and key considerations for sample collection. We also review current studies on the urinary microbiome in healthy individuals and NB patients and analyze the microbiome’s dynamic changes in different situations. Furthermore, we explore the microbiome’s potential as a distinct biomarker for early prediction and diagnosis, along with its role in rUTIs prevention and treatment.
We systematically searched for literature published before 2024 to assess the current research progress on the urinary microbiome in neurogenic bladder and its application in urinary tract infections. We searched PubMed, Web of Science, and Google Scholar databases, focusing on high-quality relevant studies. The included studies focused on the urinary microbiome composition in healthy individuals and NB patients, its mechanisms in UTIs, and therapeutic interventions like probiotic therapy. Articles that were not peer-reviewed, case reports, unpublished abstracts, and non-English studies were excluded. A total of 28 studies met the predefined inclusion criteria after screening. Keywords used in the search included “healthy,” “neurogenic bladder,” “neurogenic lower urinary tract dysfunction,” “urine,” “urinary,” “microbiome,” “microbiota,” “fungi,” “virus,” “virome,” “phage,” “metagenome,” “urinary tract infection,” “probiotics,” “fecal microbiota transplantation,” and “therapy.”
Traditionally, urinary microbiome detection has relied on standard urine cultures. However, this method has low detection rates for slow-growing and anaerobic microorganisms (Legaria et al., 2022). Next-generation sequencing (NGS) has facilitated the comprehensive characterization of the urinary microbiome, with primary methods including 16S rRNA gene sequencing (16S rRNA GS) and whole genome shotgun sequencing (WGS) (Ishihara et al., 2020).
Researchers extract DNA from samples using DNA isolation kits such as PowerSoil and BiOstic (Karstens et al., 2021), and then subsequently choose whether to perform sequencing via 16S rRNA GS or WGS. The 16S rRNA gene is universally present in all bacteria and archaea, with a full length of approximately 1500 bp, containing 9 variable regions (V1-V9) and 10 conserved regions (Abellan-Schneyder et al., 2021). Most 16S rRNA GS protocols use the V3-V4 or V4 hypervariable regions for PCR amplification and sequencing, which can classify most microorganisms to the genus level. Third-generation sequencing platforms, such as PacBio SMRT, can provide full-length sequencing to identify species-level diversity in certain microorganisms (Buetas et al., 2024). WGS involves randomly fragmenting microbial genomic DNA, adding universal primers to the ends of the fragments for PCR amplification and sequencing, and then assembling the short fragments into longer sequences. This method enables the detection of all microorganisms (including fungi and viruses) and provides their complete genomic sequences (Zhou et al., 2022).
Both 16S rRNA GS and WGS have their advantages and disadvantages. 16S rRNA GS primarily investigates microorganisms’ types, relative abundance, and evolutionary relationships. With second-generation sequencing technology, 16S rRNA GS typically only identifies microorganisms at the genus level without accurately determining species or subspecies levels and does not provide genomic sequence information beyond the primer-specified amplicon. WGS, on the other hand, allows for in-depth analysis of species classification, gene function, and metabolic networks based on 16S rRNA GS. However, it is more costly, and researchers need to choose the appropriate method based on their research objectives.
After selecting the method, techniques such as Illumina MiSeq can be used for adapter ligation, PCR amplification, library preparation, and sequencing. The resulting sequences can be analyzed using computational tools like QIIME2, Kraken2, and MetaPhlAn3 to obtain sequencing results (Min et al., 2022).
A limitation of microbial sequencing is its inability to distinguish live from dead bacteria (Aragón et al., 2018). Enhanced quantitative urine culture (EQUC), an improvement of the standard urine culture (SUC) protocol, has been developed to address this. SUC involves inoculating 1 μL of urine onto blood agar or MacConkey agar plates and incubating under standard ambient atmospheric, aerobic conditions at 35°C for 18-24 hours. In contrast to SUC, EQUC has a lower detection threshold (10 CFU/mL, compared to the 1000 CFU/mL of SUC), more diverse culture conditions (blood agar plate, MacConkey, chocolate, and colistin-nalidixic acid agars), growth temperatures (20°C, 30°C, 35°C), atmospheric conditions (air, CO2, anaerobic), incubation times (24-48 hours), and urine volumes (1, 10, and 100 μL) (Gasiorek et al., 2019). EQUC expands the range of potentially detectable live bacteria, including aerobic, microaerophilic, slow-growing anaerobic, facultative anaerobes, and fastidious bacteria (Deen et al., 2023). In urinary microbiome research, EQUC has shown higher sensitivity than SUC in detecting urogenital pathogens (Price et al., 2016; Modena et al., 2017).
Since microbial community classification analysis has been the primary objective in most urinary microbiome studies, the convenient and cost-effective 16S rRNA GS method has been the predominant approach (Neugent et al., 2020). Increasingly, researchers are combining 16S rRNA GS with EQUC or WGS to enhance detection and analysis (Antunes-Lopes et al., 2020).
Determining the adequate volume of urine to obtain sufficient bacterial DNA is crucial, as the microbial biomass in urine is relatively low, averaging less than 104 CFU/ml (O’Hara and Shanahan, 2006). Based on current studies involving the urinary microbiome, collecting at least 10 ml of urine is generally considered feasible (Xu et al., 2021; Retchless et al., 2022; Yoo et al., 2022).
Contamination is another challenge in urine collection. The primary methods include midstream voiding, catheterization, and suprapubic aspiration. Due to the proximity of the urethral opening to other high-microbe-density anatomical structures, such as the urothelium, surrounding glands, vagina, or intestines, bacterial contamination can introduce sampling bias (Kim et al., 2022). Significant differences in Lactobacillus, Neisseria, and Veillonella are observed between midstream urine samples and catheterized samples (Pohl et al., 2020; Hrbacek et al., 2021). Although suprapubic aspiration avoids contact with other areas and provides a more accurate microbiome composition of urine (Eliacik et al., 2016), it is complex and less well-accepted by patients. Existing studies suggest that the microbiome composition in urine obtained via suprapubic aspiration and catheterization is essentially similar. Hence catheter-based urine sample collection is widely employed in urinary microbiome research (Wolfe et al., 2012).
Finally, since urine cannot always be analyzed immediately after collection, appropriate storage methods are crucial for the accuracy and reproducibility of research. Studies vary in terms of urine storage time, temperature, and the use of DNA preservatives (Karstens et al., 2016; Komesu et al., 2017). Typically, urine should be transferred to 4°C within 4 hours of collection and then frozen at -80°C for long-term storage. Preservatives like AssayAssure® do not introduce significant microbial bias, and it is recommended to add them directly at a 1:10 ratio (Brubaker et al., 2021a). Research indicates that using preservatives, minimizing storage time, and maintaining lower temperatures are conducive factors for preserving the integrity of the urinary microbiome (Jung et al., 2019). In cases where neither preservatives nor temporary refrigeration is available, the urine should be promptly transferred to -80°C within 3 hours after collection.
To understand the characteristics of the urinary microbiome in NB patients, the first step is to identify which microbiome members are consistently found in the urine of healthy individuals. The urinary microbiome in healthy populations exhibits individual variations, with its composition influenced by ethnicity, age, sex, and diet. In general, the urinary microbiome demonstrates a gender—and age-dependent composition (Table 1).
At the phylum level, both male and female urinary microbiomes are dominated by Firmicutes, which make up more than two-thirds of the entire microbiome composition, followed by Actinobacteria, Bacteroidetes, and Proteobacteria (Pohl et al., 2020; Perez-Carrasco et al., 2021). At the genus level, male children (<18 years) generally lack significant characteristic microbiota, with the only notable feature being Streptococcus in prepuberty (3-12 years). The urinary microbiome predominantly comprises Corynebacterium, Pseudomonas, and Prevotella in adult males. Female urinary microbiomes exhibit changes according to developmental stages, with Bifidobacterium and Veillonella being the most common in the pre-toilet-trained stage (<3 years). There is higher genus diversity in prepuberty, with Veillonella, Prevotella, and Dialister dominating. Microbial diversity decreases post-puberty (>12 years), and Lactobacillus dominates, similar to the adult female microbiome. In adult females, the urinary microbiome is predominantly composed of Lactobacillus, Streptococcus, and Gardnerella (Fouts et al., 2012; Curtiss et al., 2017; Pohl et al., 2020; Storm et al., 2022). Gardnerella and Lactobacillus are more frequently found in younger women, whereas Escherichia and Mobiluncus are more common in postmenopausal women (Curtiss et al., 2018; Price et al., 2020a).
It is difficult to establish a unified and constant definition of the urinary microbiome in healthy populations, and these age- and sex-related variations are important considerations when evaluating the urinary microbiome and its role in health and disease. Understanding these patterns is crucial for developing targeted therapies and diagnostic tools based on urinary microbiome composition.
The urinary microbiome in NB patients is disrupted compared to healthy individuals, with key features including an increase in pathogenic pathogens and a decrease in the abundance of protective microbiota (Table 2). Existing studies indicate that the disease, bladder management methods, and disease progression influence this ecological imbalance. Fouts conducted a comparative analysis of the urinary microbiome in 27 NB patients with spinal cord injury and 26 healthy controls, and the study revealed NB patients had significantly decreased abundances of Lactobacillus, Corynebacterium, and Prevotella compared to healthy controls. At the same time, Klebsiella, Escherichia, and Enterococcus increased significantly. In female NB patients, regardless of the urination method, the relative abundance of Lactobacillus was lower than that in healthy female controls (Fouts et al., 2012). Further analysis showed that the abundance of Lactobacillus in NB patients decreased by twofold compared to healthy controls (P=0.02), with the key species Lactobacillus crispatus nearly disappearing, while Escherichia coli (P=0.005) and Enterococcus faecalis (P=0.028) significantly increased (Groah et al., 2016). Lane’s study highlighted that the urinary microbiome of NB patients is generally dominated by a single species, with Unclassified Enterobacteriaceae being the most prevalent, followed by Escherichia (Lane et al., 2022). This “pathogen-protection” imbalance is similarly prominent in the pediatric NB group. Forster investigated the urinary microbiome of NB children using clean intermittent catheterization (CIC) and found that the most abundant bacteria were Unspecified Enterobacteriaceae (mean abundance 56%), followed by Klebsiella (19%), Staphylococcus (7%), and Streptococcus (3%), consistent with studies in adults (Forster et al., 2020). De Maio found that the abundance of Proteobacteria (commonly considered the major urinary tract pathogens in NB patients) was significantly higher in NB children compared to healthy controls (35.5% vs. 2.8%, p<0.001). At the genus level, Bifidobacterium, Enterococcus, and Escherichia abundances were higher in NB children (9.2% vs. 1.6%, 10.8% vs. 1.2%, 28.6% vs. 3.8%, p<0.001).
NB patients often use indwelling catheters and CIC for bladder emptying. Invasive catheterization procedures may remodel the urinary microbiome structure through several mechanisms, including physical damage to the urinary epithelium, loss of key colonizing microbiota, promotion of pathogen biofilm formation, and disruption of the anatomical barrier leading to cross-ecological niche microbial displacement. Research has shown that NB patients using suprapubic drainage and CIC have significantly higher levels of Enterobacteriaceae and lower levels of Lactobacillaceae compared to non-NB patients. This difference was not observed in NB patients who had spontaneous voiding. In NB patients, those using catheters had higher urinary microbiome diversity than spontaneous voiding patients. Among them, patients using CIC had lower α diversity compared to those using indwelling catheters (1.16 vs 1.85, p=0.01), with no significant difference in β diversity (Groah et al., 2016; Lane et al., 2022). A similar conclusion was found in another study, where CIC patients exhibited higher α and β diversity in their urinary microbiomes than non-CIC patients. The abundance of Bacteroidetes in CIC samples was significantly lower than in non-CIC samples (10.7% vs 18.1%, p = 0.02), whereas Proteobacteria was more abundant in CIC samples (41.1% vs 21.2%, p = 0.02). At the genus level, Cutibacterium was relatively more abundant in CIC patient samples than in non-CIC samples (4.9% vs 1.2%, p = 0.01), while Faecalibacterium, Lactobacillus, Staphylococcus, and Streptococcus showed significantly lower abundances (De Maio et al., 2023).
Studies on the time dimension have revealed a trend of urinary microbiome imbalance in NB patients. The urinary microbiome in healthy individuals exhibits dynamic variability and resilience against disturbances. For example, in women, the α-diversity of the urinary microbiome increases under the influence of menstruation and sexual activity, with an increased abundance of Streptococcus and Staphylococcus. However, the microbiome quickly returns to baseline after these disturbances (Price et al., 2020b). Longitudinal studies on NB patients show a loss of the dynamic balance of the urinary microbiome compared to healthy populations. Within 2 months after injury, the microbiome composition is statistically similar to that of healthy controls, but after 1 year, Lactobacillus almost disappears, and Enterococcus and Escherichia become predominant (Fouts et al., 2012). This dynamic evolution suggests that after developing NB, the urinary microbiome loses its resistance to disturbances, and changes in the microbiome may contribute to the high risk of UTIs after NB.
Fungi and viruses are frequently neglected parts of urinary microbiome studies, comprising only a tiny fraction of the urine microbiome. Research using WGS shows that eukaryotes and viruses account for 0.05% and 0.0027% (Moustafa et al., 2018), most of which cannot be isolated through urine culture (Ackerman and Underhill, 2017). Research in healthy individuals indicates that the urinary microbiome commonly contains fungi such as Saccharomyces, Malassezia, Candida, Clavispora, and Meyerozyma (Moustafa et al., 2018; Wehedy et al., 2022), as well as viruses like HPV, BK polyomavirus, JC polyomavirus, and bacteriophages (Santiago-Rodriguez et al., 2015; Garretto et al., 2018; Moustafa et al., 2018; Garretto et al., 2019). These microbes’ abundance changes may correlate with multiple diseases. Nickel observed significantly higher detection rates of Candida and Saccharomyces during flares of urological chronic pelvic pain syndrome (15.7% vs. 3.9%) (Nickel et al., 2016), and Candida and Malassezia showed higher abundance in patients with more severe symptoms compared to those with milder symptoms (Nickel et al., 2020). Moreover, Adenovirus, HPV, and cytomegalovirus infections have been associated with lower urinary tract symptoms (Brubaker et al., 2021b). Several studies have identified the lytic activity of phages against common pathogens of UTIs and used them as an alternative therapy to antibiotics to inhibit multidrug-resistant uropathogens (Chegini et al., 2021; Subramanian, 2024).
Our understanding of fungal and viral diversity in the urinary tract of NB patients is still in its nascent stages, with a plethora of unexplored microbial communities remaining to be investigated. Documenting the differences in the microbiome between healthy individuals and symptomatic patients is a crucial initial step towards comprehending the potential role played by specific components of this class of urinary microbes in lower urinary tract health. Recent studies have observed potential associations between viral communities and overactive bladder, noting differences in the abundance of Actinobacteriophages and JC virus between asymptomatic and symptomatic individuals (Miller-Ensminger et al., 2018; Sun et al., 2022). However, there is currently a lack of targeted research and comprehensive descriptions of urinary fungi and viruses among NB patients. Their interactions with other constituents within the urinary microbiome and their associations with clinical symptoms and complications following NB remain pressing issues requiring resolution.
Accurately distinguishing between ASB and UTIs in NB patients remains a significant challenge. ASB is defined as the presence of ≥105 CFU/ml of a single uropathogen in a clean-catch midstream urine culture without clinical signs and symptoms (Luu and Albarillo, 2022), which is particularly prevalent in NB patients, with 50% to 75% of their urine cultures showing positive results. UTIs are one of the most frequent complications in patients with NB, with a prevalence of about 70% (Figure 2) (Penders et al., 2003; Liu et al., 2023; Fitzpatrick et al., 2024).
However, conventional UTIs diagnostic criteria—urinary symptoms (such as dysuria, odor, and cloudiness), inflammatory markers (pyuria, WBC ≥10/ml), and positive urine cultures—are often unreliable in NB patients due to the altered bladder physiology compared to health individuals (Goebel et al., 2021). The etiology of NB, NB classification, bladder management strategies, the patient’s level of voluntary sensation, and the nervous system status all influence clinical presentation and symptom reporting (Tractenberg et al., 2018). For instance, SCI patients with NB often have reduced or absent sensory perception below the injury level (Jahromi et al., 2014). Research indicates that only 61% of SCI patients with NB can accurately predict when they are amid UTIs (Linsenmeyer and Oakley, 2003).
Catheter use further complicates diagnosis. Catheterization is a common bladder management technique for NB patients; a single microbial pathogen often causes short-term catheter-associated UTIs, but most UTIs in patients with long-term catheterization (>30 days) are essentially multiple microbial infections (Klein and Hultgren, 2020). SUC are designed to detect a limited number of common aerobic urinary pathogens. This approach inevitably leads to high false negatives and diagnostic limitations of SUC in UTIs (Price et al., 2016).
There is no widely acceptable and precise definition for UTIs in NB patients. The empirical treatment approaches often lead to overdiagnosis of UTIs and unnecessary antibiotic use, which contributes to an increasing rate of antibiotic-resistant infections in these patients (Forster et al., 2017; Fitzpatrick et al., 2018). In order to improve diagnostic accuracy, reduce the recurrence of UTIs, and address the growing issue of antibiotic resistance, there is a pressing need for more precise methods explicitly tailored for NB patients. Techniques such as 16S rRNA GS, EQUC, and WGS are expected to provide more comprehensive detection and monitoring of the dynamic changes in the urinary microbiome (McDonald et al., 2017; Almas et al., 2023; Zhao et al., 2024). These methods hold promise for accurately identifying the urinary tract status at a given time and using the presence of characteristic microorganisms or specific changes in the abundance of resident microbiota as diagnostic, predictive, and prognostic markers for UTIs.
UTIs is the most common complication in NB patients. RUTIs, defined as three or more episodes annually or two or more episodes within six months, account for about 30% of NB patients (Theisen et al., 2020). Frequent pathogens in rUTIs comprise Escherichia coli, Klebsiella, Proteus, Pseudomonas, and Enterococcus, with Escherichia coli responsible for over half of these infections (Dinh et al., 2020; Weyler et al., 2021; Alghoraibi et al., 2023). Although antibiotics are effective in the short term, their prolonged use to achieve the traditional “sterile urine” aim disrupts protective and beneficial urinary microbiota. Recent studies associate this with “urinary tract microecosystem dysbiosis,” which increases the risk of pathogenic colonization, escalates multidrug-resistant strains in the urinary tract, and exacerbates the challenges in managing UTIs, eventually results in upper urinary tract damage, kidney function deterioration, sepsis, and other severe consequences, significantly impacting lower urinary tract health and long-term prognosis (Finucane, 2017; Wu et al., 2022). Treatments based on the urinary microbiome offer an alternative paradigm for NB patients to combat UTIs beyond antibiotic therapies by maintaining the ecological balance of the lower urinary tract, replenishing protective characteristic taxa, or directly targeting urinary pathogens (Table 3).
Lactobacillus is considered a key microbial component of a healthy microbiome, and urinary studies in NB patients have revealed a marked decline in its abundance. As one of the most promising measures for UTIs prevention and treatment, Lactobacillus can modulate immune responses, inhibit the growth of pathogenic organisms, and reduce their adhesion to bladder epithelial cells (de Llano et al., 2017; Putonti et al., 2020; Tkhruni et al., 2020). For instance, Lactobacillus rhamnosus GR-1 has been shown to secrete various immunomodulatory proteins (such as GroEL, Elongation factor Tu, and NLP/P60), enhance NF-κB activity in T24 bladder cells (Petrova et al., 2021). It can downregulate the virulence of uropathogenic Escherichia coli and inhibit biofilm formation by pathogens via lectin-like proteins 1 and 2 (Petrova et al., 2016). Research has increasingly focused on using probiotics—particularly those based on Lactobacillus strains—to prevent UTIs and reduce their severity. However, Lactobacillus exhibits strain specificity, meaning that different strains vary in inhibitory mechanisms and effectiveness. This may require selecting specific probiotics or using combinations to target different pathogenic strains. The European Association of Urology guidelines recommend Lactobacillus rhamnosus GR-1, Lactobacillus reuteri B-54, Lactobacillus reuteri RC-14, Lactobacillus casei strain Shirota, and Lactobacillus crispatus CTV-05 for the prevention of rUTIs caused by various factors (Chen et al., 2023).
In addition to regulating the inflammatory response, Lactobacillus also possesses the ability to migrate from the gastrointestinal tract through the rectum and anus to the urethra (Reid and Bruce, 2006). Early studies evaluated the oral probiotics approach positively, suggesting it could effectively prevent rUTIs (Falagas et al., 2006). However, recent research has indicated that the effectiveness of oral probiotics in preventing UTIs does not show satisfactory differences compared to antibiotics or placebo.
Beerepoot conducted a randomized, double-blind trial (ISRCTN50717094) comparing Lactobacillus rhamnosus GR-1 and Lactobacillus RC-14 with trimethoprim-sulfamethoxazole in 252 postmenopausal women suffering from rUTIs (Beerepoot et al., 2012). The administration of oral probiotic capsules significantly reduced the mean number of symptomatic UTIs among these women after 12 months, decreasing from 6.8 to 3.3. However, this did not satisfy the noninferiority criteria when compared to the antibiotic group, which also showed a decrease from 7.0 to 3.3. Another finding was that the probiotic group did not show an increase in bacterial resistance. However, the antibiotic group experienced an increase in resistance rates from 20%-40% to 80%-95%, highlighting the potential benefit of probiotics in avoiding antibiotic resistance. Wolff used the same oral probiotics method to investigate 7 healthy premenopausal women (NCT03250208) and found no significant change in the ratio of uropathogens to Lactobacillus in urine compared to a placebo (Wolff et al., 2019). Probiotics were not detected in the urine samples either. However, the study’s small sample size and reliance on EQUC for bacterial identification rather than high-throughput sequencing limit the conclusiveness of these findings.
NB patient studies have yielded comparable conclusions. A randomized, placebo-controlled trial (ACTRN12610000512022) conducted by Toh, utilizing identical oral probiotics in 207 SCI patients with NB, also demonstrated no significant effect of probiotics on preventing UTIs in this population (Toh et al., 2019). Nevertheless, Lactobacillus rhamnosus GR-1 and Lactobacillus RC-14 positively inhibited the colonization of multidrug-resistant Gram-negative bacteria (Toh et al., 2020).
In studies involving other probiotic combinations, Bossa observed that oral Lactobacillus rhamnosus GG with Bifidobacterium BB-12 could alter the catheter microbiome of SCI patients with NB during treatment (ACTRN12610000512022). However, this alteration is transient, the microbiome quickly returns to its original state after discontinuing the treatment (Bossa et al., 2017). Daniel conducted a study (NCT03462160) involving the oral administration of Lactobacillus rhamnosus PL1 and Lactobacillus plantarum PM1 in 54 children diagnosed with rUTIs, comprising 42 individuals with bladder dysfunction and 4 with NB (Daniel et al., 2020). The probiotic group showed a reduction in UTIs frequency and antibiotic treatment days, but these differences were not statistically significant compared to the placebo group.
Although oral probiotic therapy does not show a significant advantage over antibiotics in preventing UTIs, it mitigates the heightened risk of antibiotic resistance, indicating that probiotics may be a viable alternative to antibiotics. One plausible explanation for the comparable reduction in UTIs incidence observed in the placebo group is that participants may have unconsciously enhanced their aseptic techniques during clean catheterization and perineal care following enrollment, which is closely related to UTIs occurrences, though these relevant factors were not addressed in the studies. A recent study indicates that combining oral probiotic capsules ProBalans (containing Lactobacillus acidophilus LA3, Bifidobacterium animalis subsp. lactis BLC1, and Lactobacillus casei BGP93) with antibiotics provides better outcomes for UTIs treatment compared to antibiotics alone (Mula et al., 2024).
The female urogenital microbiome exhibits interrelatedness, with samples from the same female urinary tract and vaginal revealing identical strains between these anatomical sites. This association extends beyond common pathogens, such as Escherichia coli and Streptococcus, to include beneficial bacteria closely linked to health, including Lactobacillus iners, Lactobacillus crispatus, Lactobacillus jensenii, and Lactobacillus gasseri (Thomas-White et al., 2018; Komesu et al., 2020; Atkins et al., 2024). A plausible hypothesis is that bacteria may transfer between the vagina and the urethra by “hitchhiking” with the movement of other microorganisms or through mechanical motion of adjacent areas (Muok and Briegel, 2021). This finding provides further theoretical support for the potential of influencing urinary microbiota through interventions targeting the vaginal microbiome.
The healthy vaginal microbiome is dominated primarily by Lactobacillus, which helps resist pathogen colonization by lowering vaginal pH and modulating immune responses (Holm et al., 2023). Some vaginal microbiome acting as a “hidden pathogen” that facilitates the pathogenesis of harmful bacteria, like Gardnerella vaginalis, can induce the release of Escherichia coli from latent bladder reservoirs leading to UTIs, trigger apoptosis and desquamation of bladder epithelial cells and mediate acute kidney injury through IL-1 receptor pathways (Lewis and Gilbert, 2020). Low abundance of Lactobacillus has been associated with higher disease risk, and supplementing with vaginal probiotics may effectively modulate the microbiome to prevent disease occurrence (Holdcroft et al., 2023).
Stapleton conducted a Phase II clinical trial (NCT00305227) involving vaginal suppositories containing Lactobacillus crispatus in 100 young women with a history of rUTIs (Stapleton et al., 2011). High levels of Lactobacillus crispatus colonization (≥106 16S RNA gene per swab from the vagina) were observed in 93% of the participants within the experimental group, which was associated with a significant reduction in UTIs incidence. In contrast, the placebo group demonstrated a Lactobacillus crispatus high-level colonization rate of only 68%, and there was no observed decrease in UTIs rates regardless of colonization levels, indicating that exogenous probiotic intervention provides a notable advantage over endogenous vaginal microbiome recolonization. Similarly, Sadahira utilized vaginal suppositories containing Lactobacillus crispatus to reduce the frequency of recurrent cystitis in postmenopausal women and confirm the successful colonization of lactobacilli in the vagina (Sadahira et al., 2021; Sekito et al., 2023).
A recent study shows that the combined use of vaginal probiotic suppositories with oral probiotics (jRCTs061180053) can significantly reduce the incidence of UTIs and alleviates patients’ discomfort (Gupta et al., 2024). However, current studies on transvaginal probiotic administration predominantly involve premenopausal or postmenopausal women, with limited research available on patients with NB and no relevant studies addressing changes in the urinary microbiome after treatment.
It is difficult for bacteria to colonize the bladder, and successful bladder colonization partially depends on impaired bladder emptying (Wullt et al., 1998). The urodynamic defects observed in NB patients facilitate the establishment of non-virulent strains in the urinary tract.
Forster conducted a study about bladder instillation of Lactobacillus rhamnosus GG in 10 NB patients (Forster et al., 2021). They used standard urine cultures and 16S rRNA GS for multiple analyses of urine samples 7-10 days after the instillation. They discovered a significant disparity in β diversity of the microbiome before and after the instillation but no variance in the α diversity. After the instillation, significant shifts were observed in the relative abundances of Escherichia, Prevotella, and Lactobacillus in the urinary microbiome of most patients. However, the high individual heterogeneity made identifying consistent trends in specific taxa. Another study involving 103 adults and children with neurogenic lower urinary tract dysfunction due to SCI or other conditions described trends in urinary microbiome changes (Tractenberg et al., 2021; Groah et al., 2023). Participants used CIC to empty their bladders and performed bladder instillation one to two times when symptoms of foul-smelling or cloudy urine appeared. The study found that bladder instillation of Lactobacillus rhamnosus GG significantly reduced the α diversity of the urinary microbiome, along with a decrease in the abundance of Escherichia, Shigella, and Aerococcus. In contrast, there were no significant changes in the abundance of other urinary pathogens (such as Klebsiella and Proteus) or commensal bacteria (Lactobacillus, Veillonella, Staphylococcus, and Streptococcus).
Contrary to expectations, existing studies have not observed a consistent increase in Lactobacillus abundance following bladder instillation. The changes in the urinary microbiome may be attributed to the fluid’s mechanical flushing effect. Further efficacy studies are needed to confirm the role of probiotics. Currently, a randomized controlled comparative effectiveness clinical trial is underway, aiming to compare bladder instillation of probiotics with saline bladder irrigation (Groah and Tractenberg, 2022).
Fecal microbiota transplantation (FMT) aims to eliminate the colonization of pathogenic multidrug-resistant organisms in the gut, improve dysbiotic microenvironment conditions, and enhance resistance to pathogen colonization by transferring the fecal microbiota from healthy individuals to the gastrointestinal tract of specific patients through oral capsules or nasogastric tubes (Yoon et al., 2019; Wuethrich et al., 2021). FMT has successfully treated recurrent Clostridium difficile infections and reduced antibiotic-resistance genes associated with multidrug-resistant organisms (Nooij et al., 2024; Rashidi et al., 2024).
Due to the anatomical proximity of the urethra to the anus, the urinary system is often directly influenced by the microbiota of the digestive tract. Concurrently, the recent discovery of the brain-gut-bladder axis offers new insights into the mechanisms of UTIs (Worby et al., 2022). Dysbiosis of the gut microbiota has been linked to increased rUTIs, suggesting novel strategies for mitigating UTIs risk by enhancing the gut microbial environment (Choi et al., 2024; Ruţa et al., 2024).
The application of FMT in patients with UTIs caused by multidrug-resistant organisms has demonstrated promising clinical efficacy and economic benefits (Grosen et al., 2019; Baek et al., 2023). Hocquart conducted a study on patients suffering from both irritable bowel syndrome and rUTIs, 30g of feces from a healthy individual dissolved in 400 milliliters of saline and removed impurities, the solution was delivered via a nasogastric tube following an enema. During an 8-month follow-up, patients showed significant improvement in irritable bowel symptoms and no further occurrences of UTIs (Hocquart et al., 2019). Additionally, a case report by Bier highlighted the successful treatment of UTIs caused by extended-spectrumβ-lactamase -producing Klebsiella pneumoniae infection using an oral freeze-dried fecal capsule preparation known as PRIM-DJ2727 (60 grams of total stool lyophilized into 1 gram per dose), sequencing revealed an increase in urinary microbiome α diversity after FMT, accompanied by a reduction in the abundance of Klebsiella pneumoniae (Bier et al., 2023).
The role of FMT in the successful treatment of rUTIs may be complex. All studies have small sample sizes and lack systematic descriptions of dynamic changes in the urinary microbiome following FMT. Current research on FMT primarily focuses on gastrointestinal diseases (Porcari et al., 2023). In addition, addressing the potential psychological resistance patients may have toward fecal matter poses a challenge for future research (Yu et al., 2023).
Bacteriophages have shown high lytic activity against common uropathogens in vitro (Sybesma et al., 2016). Their movement and bacterial lysis efficiency are severely limited in low-humidity environments, making the liquid environment of the urinary system an ideal setting for medical applications (Żaczek et al., 2020). However, single phage therapy is limited in its ability to treat a variety of microbial infections due to its high specificity, and there is a risk of bacterial resistance to phages evolving. Hence, the combination of phages, phage lytic enzymes, and antibiotics is the preferred treatment approach (Malik et al., 2020).
Leitner conducted a randomized, double-blind, controlled trial (NCT03140085) involving 113 adult males with UTIs undergoing transurethral resection of the prostate. Participants received a 7 day bladder instillation of Pyophage (a commercial bacteriophage produced by the Eliava Institute) (Leitner et al., 2021b). The results showed no statistically significant differences in treatment efficacy among the groups (success rates: bacteriophage 18% vs. antibiotic 35% vs. placebo 28%). However, the bacteriophage treatment appeared to be more reliable regarding safety, with adverse event rates of 21% for bacteriophage, 30% for antibiotics, and 41% for placebo. Leitner also pointed out the potential use of phage therapy in NB individuals resulting from SCI and initiated a further clinical trial in 2020 to investigate phage therapy for catheter-associated UTIs (Leitner et al., 2021a).
The combination of bacteriophages and probiotics offers a promising new approach. Competitive alternative therapies using probiotics alone may be limited by ecological microenvironmental disorders in different sites such as the gut and vagina. Even if probiotics work well in vitro, a healthy gut or pathological bladder consists of already populated bacterial ecological niches, which may be at a disadvantage in the frequency-dependent competition for microenvironments when probiotics are administered orally or by bladder drip, which is in line with the widespread conclusion that probiotics have inconsistent clinical efficacy (Lerner et al., 2019). The efficient infection characteristics of bacteriophages may create favorable conditions for probiotics to colonize vacant niches, subsequently inhibiting or replacing target microorganisms through frequency-dependent competition (Forsyth et al., 2023). In vitro and animal studies have demonstrated superior synergistic effects of ST131-specific phages combined with Escherichia coli Nissle 1917, highlighting their potential clinical application for more effectively reducing or displacing uropathogens or combating the colonization of multidrug-resistant bacteria (Porter et al., 2022; Forsyth et al., 2023).
The study of the urinary microbiome in neurogenic bladder is gradually breaking through the conventional paradigm of sterile urine, revealing the complex ecology of the urinary system disrupted by neurological control mechanisms. It uncovers the cascade imbalance pattern in NB patients: “protective microbiota decline—pathogenic bacteria expansion—frequent UTIs—renal function damage.” Additionally, it identifies the synergistic effect of the disease’s natural progression and catheter insertion as an important intervention, providing evidence for defining clinical intervention windows.
Studies have confirmed that specific bacterial genera, such as Lactobacillus and Enterococcus, may be microbiological biomarkers, breaking through the diagnostic bottleneck. Constructing a UTIs risk stratification model based on microbial community characteristics provides auxiliary diagnostic tools. It promotes transforming the diagnostic and treatment model from “symptom-driven” to “microbial alert.”
More notably, microbiome-based therapies show potential in overcoming the dilemma of multiple drug resistance. By tailoring individualized treatment plans based on patients’ urinary microbiome baseline characteristics, a shift from passive infection control to active ecological regulation can be achieved.
However, there is limited research on NB, and some results exhibit heterogeneity. This heterogeneity may be attributed to small patient sample sizes, low urinary microbiome biomass, individual differences, variations in sample collection methods, and contamination during the collection process. Moreover, current research does not consider the different types of NB, and variations in urodynamics could result in individual differences in the urinary microbiome. Future research should refine methods to prevent the distortion of valuable results already obtained due to the aforementioned factors. Establishing standardized sampling protocols, reporting guidelines, and efficacy evaluation systems, along with developing a dynamic temporal database for the urinary microbiome in NB patients, will be essential. Furthermore, constructing a spatiotemporal microbiota-host interaction network that integrates the gut, vaginal, and urinary microbiomes following interventions such as probiotic therapy could provide deeper insights beyond those offered by cross-sectional studies.
In summary, current research on the urinary microbiome is facilitating the translation of basic research into clinical applications. The goal is to transform the urinary microbiome from an observational marker into a modifiable therapeutic target, reshaping the management paradigm of NB—from fragmented symptom control to precise ecological regulation, ultimately improving long-term patient outcomes and quality of life.
JZ: Writing – original draft, Writing – review & editing, Conceptualization. YL: Writing – original draft, Writing – review & editing. HD: Writing – original draft, Writing – review & editing. ZL: Writing – original draft, Writing – review & editing. XW: Writing – original draft, Writing – review & editing. DY: Methodology, Resources, Writing – review & editing. FG: Methodology, Supervision, Writing – review & editing. JL: Supervision, Writing – review & editing.
The author(s) declare that no financial support was received for the research and/or publication of this article.
The authors declare that the research was conducted in the absence of any commercial or financial relationships that could be construed as a potential conflict of interest.
The author(s) declare that no Generative AI was used in the creation of this manuscript.
All claims expressed in this article are solely those of the authors and do not necessarily represent those of their affiliated organizations, or those of the publisher, the editors and the reviewers. Any product that may be evaluated in this article, or claim that may be made by its manufacturer, is not guaranteed or endorsed by the publisher.
Żaczek, M., Weber-Dąbrowska, B., Górski, A. (2020). Phages as a cohesive prophylactic and therapeutic approach in aquaculture systems. Antibiotics (Basel Switzerland) 9, 564. doi: 10.3390/antibiotics9090564
Abellan-Schneyder, I., MatChado, M. S., Reitmeier, S., Sommer, A., Sewald, Z., Baumbach, J., et al. (2021). Primer, pipelines, parameters: issues in 16S rRNA gene sequencing. mSphere 6, e01202–e01220. doi: 10.1128/mSphere.01202-20
Ackerman, A. L., Underhill, D. M. (2017). The mycobiome of the human urinary tract: potential roles for fungi in urology. Ann. Trans. Med. 5, 31. doi: 10.21037/atm.2016.12.69
Alghamdi, M. A., Amer, K. A., Aldosari, A. A. S., Al Qahtani, R. F., Shar, H. S., Al-Tarish, L. M., et al. (2023). Assessment of the impact of lower urinary tract dysfunction on quality of life in multiple sclerosis patients in Saudi Arabia-A cross-sectional study. Healthcare (Basel Switzerland) 11, 2694. doi: 10.3390/healthcare11192694
Alghoraibi, H., Asidan, A., Aljawaied, R., Almukhayzim, R., Alsaydan, A., Alamer, E., et al. (2023). Recurrent urinary tract infection in adult patients, risk factors, and efficacy of low dose prophylactic antibiotics therapy. J. Epidemiol. Global Health 13, 200–211. doi: 10.1007/s44197-023-00105-4
Almas, S., Carpenter, R. E., Rowan, C., Tamrakar, V. K., Bishop, J., Sharma, R. (2023). Advantage of precision metagenomics for urinary tract infection diagnostics. Front. Cell. Infection Microbiol. 13, 1221289. doi: 10.3389/fcimb.2023.1221289
Amarenco, G., Sheikh Ismaël, S., Chesnel, C., Charlanes, A., Le Breton, F. (2017). Diagnosis and clinical evaluation of neurogenic bladder. Eur. J. Phys. Rehabil. Med. 53, 975–980. doi: 10.23736/S1973-9087.17.04992-9
Antunes-Lopes, T., Vale, L., Coelho, A. M., Silva, C., Rieken, M., Geavlete, B., et al. (2020). The role of urinary microbiota in lower urinary tract dysfunction: A systematic review. Eur. Urol. Focus 6, 361–369. doi: 10.1016/j.euf.2018.09.011
Aragón, I. M., Herrera-Imbroda, B., Queipo-Ortuño, M. I., Castillo, E., Del Moral, J. S.-G., Gómez-Millán, J., et al. (2018). The urinary tract microbiome in health and disease. Eur. Urol. Focus 4, 128–138. doi: 10.1016/j.euf.2016.11.001
Atkins, H., Sabharwal, B., Boger, L., Stegman, N., Kula, A., Wolfe, A. J., et al. (2024). Evidence of Lactobacillus strains shared between the female urinary and vaginal microbiota. Microbial Genomics 10, 1267. doi: 10.1099/mgen.0.001267
Baek, O. D., Hjermitslev, C. K., Dyreborg, L., Baunwall, S. M.D., Høyer, K. L., Rågård, N., et al. (2023). Early economic assessment of faecal microbiota transplantation for patients with urinary tract infections caused by multidrug-resistant organisms. Infect. Dis. Ther. 12, 1429–1436. doi: 10.1007/s40121-023-00797-y
Beerepoot, M. A. J., ter Riet, G., Nys, S., van der Wal, W. M., de Borgie, C. A. J. M., de Reijke, T. M., et al. (2012). Lactobacilli vs antibiotics to prevent urinary tract infections: a randomized, double-blind, noninferiority trial in postmenopausal women. Arch. Internal Med. 172, 704–712. doi: 10.1001/archinternmed.2012.777
Bier, N., Hanson, B., Jiang, Z.-D., DuPont, H. L., Arias, C. A., Miller, W. R. (2023). A case of successful treatment of recurrent urinary tract infection by extended-spectrum β-lactamase producing klebsiella pneumoniae using oral lyophilized fecal microbiota transplant. Microbial Drug Resistance (Larchmont N.Y.) 29, 34–38. doi: 10.1089/mdr.2022.0031
Bossa, L., Kline, K., McDougald, D., Lee, B. B., Rice, S. A. (2017). Urinary catheter-associated microbiota change in accordance with treatment and infection status. PloS One 12, e0177633. doi: 10.1371/journal.pone.0177633
Brubaker, L., Gourdine, J.-P. F., Siddiqui, N. Y., Holland, A., Halverson, T., Limeria, R., et al. (2021a). Forming consensus to advance urobiome research. mSystems 6, e0137120. doi: 10.1128/mSystems.01371-20
Brubaker, L., Putonti, C., Dong, Q., Wolfe, A. J. (2021b). The human urobiome. Mamm. Genome 32, 232–238. doi: 10.1007/s00335-021-09862-8
Buetas, E., Jordán-López, M., López-Roldán, A., D’Auria, G., Martínez-Priego, L., De Marco, G., et al. (2024). Full-length 16S rRNA gene sequencing by PacBio improves taxonomic resolution in human microbiome samples. BMC Genomics 25, 310. doi: 10.1186/s12864-024-10213-5
Chegini, Z., Khoshbayan, A., Vesal, S., Moradabadi, A., Hashemi, A., Shariati, A. (2021). Bacteriophage therapy for inhibition of multi drug-resistant uropathogenic bacteria: a narrative review. Ann. Clin. Microbiol. Antimicrobials 20, 30. doi: 10.1186/s12941-021-00433-y
Chen, Y.-C., Lee, W.-C., Chuang, Y.-C. (2023). Emerging non-antibiotic options targeting uropathogenic mechanisms for recurrent uncomplicated urinary tract infection. Int. J. Mol. Sci. 24, 7055. doi: 10.3390/ijms24087055
Chen, J., Wang, L., Liu, M., Gao, G., Zhao, W., Fu, Q., et al. (2022). Implantation of adipose-derived mesenchymal stem cell sheets promotes axonal regeneration and restores bladder function after spinal cord injury. Stem Cell Res. Ther. 13, 503. doi: 10.1186/s13287-022-03188-1
Choi, J., Thänert, R., Reske, K. A., Nickel, K. B., Olsen, M. A., Hink, T., et al. (2024). Gut microbiome correlates of recurrent urinary tract infection: a longitudinal, multi-center study. EClinicalMedicine 71, 102490. doi: 10.1016/j.eclinm.2024.102490
Curtiss, N., Balachandran, A., Krska, L., Peppiatt-Wildman, C., Wildman, S., Duckett, J. (2017). A case controlled study examining the bladder microbiome in women with Overactive Bladder (OAB) and healthy controls. Eur. J. Obstetrics Gynecology Reprod. Biol. 214, 31–35. doi: 10.1016/j.ejogrb.2017.04.040
Curtiss, N., Balachandran, A., Krska, L., Peppiatt-Wildman, C., Wildman, S., Duckett, J. (2018). Age, menopausal status and the bladder microbiome. Eur. J. Obstetrics Gynecology Reprod. Biol. 228, 126–129. doi: 10.1016/j.ejogrb.2018.06.011
Daniel, M., Szymanik-Grzelak, H., Turczyn, A., Pańczyk-Tomaszewska, M. (2020). Lactobacillus rhamnosus PL1 and Lactobacillus plantarum PM1 versus placebo as a prophylaxis for recurrence urinary tract infections in children: a study protocol for a randomised controlled trial. BMC Urol. 20, 168. doi: 10.1186/s12894-020-00723-1
Deen, N. S., Ahmed, A., Tasnim, N. T., Khan, N. (2023). Clinical relevance of expanded quantitative urine culture in health and disease. Front. Cell. Infection Microbiol. 13, 1210161. doi: 10.3389/fcimb.2023.1210161
de Llano, D. G., Arroyo, A., Cárdenas, N., Rodríguez, J. M., Moreno-Arribas, M. V., Bartolomé, B. (2017). Strain-specific inhibition of the adherence of uropathogenic bacteria to bladder cells by probiotic Lactobacillus spp. Pathog. Dis. 75. doi: 10.1093/femspd/ftx043
De Maio, F., Grotti, G., Mariani, F., Buonsenso, D., Santarelli, G., Bianco, D. M., et al. (2023). Profiling the urobiota in a pediatric population with neurogenic bladder secondary to spinal dysraphism. Int. J. Mol. Sci. 24, 8261. doi: 10.3390/ijms24098261
DeWitt-Foy, M. E., Elliott, S. P. (2022). Neurogenic bladder: assessment and operative management. Urologic Clinics North America 49, 519–532. doi: 10.1016/j.ucl.2022.04.010
Dinh, A., Hallouin-Bernard, M.-C., Davido, B., Lemaignen, A., Bouchand, F., Duran, C., et al. (2020). Weekly sequential antibioprophylaxis for recurrent urinary tract infections among patients with neurogenic bladder: A randomized controlled trial. Clin. Infect. Dis. 71, 3128–3135. doi: 10.1093/cid/ciz1207
Eliacik, K., Kanik, A., Yavascan, O., Alparslan, C., Kocyigit, C., Aksu, N., et al. (2016). A comparison of bladder catheterization and suprapubic aspiration methods for urine sample collection from infants with a suspected urinary tract infection. Clin. Pediatr. 55, 819–824. doi: 10.1177/0009922815608278
Falagas, M. E., Betsi, G. I., Tokas, T., Athanasiou, S. (2006). Probiotics for prevention of recurrent urinary tract infections in women: a review of the evidence from microbiological and clinical studies. Drugs 66, 1253–1261. doi: 10.2165/00003495-200666090-00007
Finucane, T. E. (2017). Urinary tract infection”-requiem for a heavyweight. J. Am. Geriatrics Soc. 65, 1650–1655. doi: 10.1111/jgs.14907
Fitzpatrick, M. A., Suda, K. J., Safdar, N., Burns, S. P., Jones, M. M., Poggensee, L., et al. (2018). Changes in bacterial epidemiology and antibiotic resistance among veterans with spinal cord injury/disorder over the past 9 years. J. Spinal Cord Med. 41, 199–207. doi: 10.1080/10790268.2017.1281373
Fitzpatrick, M. A., Wirth, M., Burns, S. P., Suda, K. J., Weaver, F. M., Collins, E., et al. (2024). Management of asymptomatic bacteriuria and urinary tract infections in patients with neurogenic bladder and factors associated with inappropriate diagnosis and treatment. Arch. Phys. Med. Rehabil. 105, 112–119. doi: 10.1016/j.apmr.2023.09.023
Forster, C. S., Courter, J., Jackson, E. C., Mortensen, J. E., Haslam, D. B. (2017). Frequency of multidrug-resistant organisms cultured from urine of children undergoing clean intermittent catheterization. J. Pediatr. Infect. Dis. Soc. 6, 332–338. doi: 10.1093/jpids/piw056
Forster, C. S., Hsieh, M. H., Pérez-Losada, M., Caldovic, L., Pohl, H., Ljungberg, I., et al. (2021). A single intravesical instillation of Lactobacillus rhamnosus GG is safe in children and adults with neuropathic bladder: A phase Ia clinical trial. J. Spinal Cord Med. 44, 62–69. doi: 10.1080/10790268.2019.1616456
Forster, C. S., Panchapakesan, K., Stroud, C., Banerjee, P., Gordish-Dressman, H., Hsieh, M. H. (2020). A cross-sectional analysis of the urine microbiome of children with neuropathic bladders. J. Pediatr. Urol. 16, 593.e1–593.e8. doi: 10.1016/j.jpurol.2020.02.005
Forsyth, J. H., Barron, N. L., Scott, L., Watson, B. N. J., Chisnall, M. A. W., Meaden, S., et al. (2023). Decolonizing drug-resistant E. coli with phage and probiotics: breaking the frequency-dependent dominance of residents. Microbiol. (Reading England) 169, 1352. doi: 10.1099/mic.0.001352
Fouts, D. E., Pieper, R., Szpakowski, S., Pohl, H., Knoblach, S., Suh, M.-J., et al. (2012). Integrated next-generation sequencing of 16S rDNA and metaproteomics differentiate the healthy urine microbiome from asymptomatic bacteriuria in neuropathic bladder associated with spinal cord injury. J. Trans. Med. 10, 174. doi: 10.1186/1479-5876-10-174
Garretto, A., Miller-Ensminger, T., Wolfe, A. J., Putonti, C. (2019). Bacteriophages of the lower urinary tract. Nat. Rev. Urol. 16, 422–432. doi: 10.1038/s41585-019-0192-4
Garretto, A., Thomas-White, K., Wolfe, A. J., Putonti, C. (2018). Detecting viral genomes in the female urinary microbiome. J. Gen. Virol. 99, 1141–1146. doi: 10.1099/jgv.0.001097
Gasiorek, M., Hsieh, M. H., Forster, C. S. (2019). Utility of DNA next-generation sequencing and expanded quantitative urine culture in diagnosis and management of chronic or persistent lower urinary tract symptoms. J. Clin. Microbiol. 58, e00204–e00219. doi: 10.1128/JCM.00204-19
Ginsberg, D. A., Boone, T. B., Cameron, A. P., Gousse, A., Kaufman, M. R., Keays, E., et al. (2021). The AUA/SUFU guideline on adult neurogenic lower urinary tract dysfunction: treatment and follow-up. J. Urol. 206, 1106–1113. doi: 10.1097/JU.0000000000002239
Goebel, M. C., Trautner, B. W., Grigoryan, L. (2021). The five ds of outpatient antibiotic stewardship for urinary tract infections. Clin. Microbiol. Rev. 34, e0000320. doi: 10.1128/CMR.00003-20
Goyal, D., Ali, S. A., Singh, R. K. (2021). Emerging role of gut microbiota in modulation of neuroinflammation and neurodegeneration with emphasis on Alzheimer’s disease. Prog. Neuropsychopharmacol. Biol. Psychiatry 106, 110112. doi: 10.1016/j.pnpbp.2020.110112
Groah, S. L., Pérez-Losada, M., Caldovic, L., Ljungberg, I. H., Sprague, B. M., Castro-Nallar, E., et al. (2016). Redefining healthy urine: A cross-sectional exploratory metagenomic study of people with and without bladder dysfunction. J. Urol. 196 (2), 579–587. doi: 10.1016/j.juro.2016.01.088
Groah, S. L., Rounds, A. K., Pérez-Losada, M. (2023). Intravesical lactobacillus rhamnosus GG alters urobiome composition and diversity among people with neurogenic lower urinary tract dysfunction. Topics Spinal Cord Injury Rehabil. 29, 44–57. doi: 10.46292/sci23-00004
Groah, S. L., Tractenberg, R. E. (2022). Intravesical Lactobacillus rhamnosus GG versus Saline Bladder Wash: Protocol for a Randomized, Controlled, Comparative Effectiveness Clinical Trial. Topics Spinal Cord Injury Rehabil. 28, 12–21. doi: 10.46292/sci22-00005
Grosen, A. K., Povlsen, J. V., Lemming, L. E., Jørgensen, S. M. D., Dahlerup, J. F., Hvas, C. L. (2019). Faecal microbiota transplantation eradicated extended-spectrum beta-lactamase-producing klebsiella pneumoniae from a renal transplant recipient with recurrent urinary tract infections. Case Rep. Nephrol. Dialysis 9, 102–107. doi: 10.1159/000502336
Gupta, V., Mastromarino, P., Garg, R. (2024). Effectiveness of prophylactic oral and/or vaginal probiotic supplementation in the prevention of recurrent urinary tract infections: A randomized, double-blind, placebo-controlled trial. Clin. Infect. Diseases: Off. Publ. Infect. Dis. Soc. America 78, 1154–1161. doi: 10.1093/cid/ciad766
Hocquart, M., Pham, T., Kuete, E., Tomei, E., Lagier, J. C., Raoult, D. (2019). Successful fecal microbiota transplantation in a patient suffering from irritable bowel syndrome and recurrent urinary tract infections. Open Forum Infect. Dis. 6, ofz398. doi: 10.1093/ofid/ofz398
Holdcroft, A. M., Ireland, D. J., Payne, M. S. (2023). The vaginal microbiome in health and disease-what role do common intimate hygiene practices play? Microorganisms 11, 298. doi: 10.3390/microorganisms11020298
Holm, J. B., France, M. T., Gajer, P., Ma, B., Brotman, R. M., Shardell, M., et al. (2023). Integrating compositional and functional content to describe vaginal microbiomes in health and disease. Microbiome 11, 259. doi: 10.1186/s40168-023-01692-x
Hrbacek, J., Morais, D., Cermak, P., Hanacek, V., Zachoval, R. (2021). Alpha-diversity and microbial community structure of the male urinary microbiota depend on urine sampling method. Sci. Rep. 11, 23758. doi: 10.1038/s41598-021-03292-x
Ishihara, T., Watanabe, N., Inoue, S., Aoki, H., Tsuji, T., Yamamoto, B., et al. (2020). Usefulness of next-generation DNA sequencing for the diagnosis of urinary tract infection. Drug Discoveries Ther. 14, 42–49. doi: 10.5582/ddt.2020.01000
Jahromi, M. S., Mure, A., Gomez, C. S. (2014). UTIs in patients with neurogenic bladder. Curr. Urol. Rep. 15, 433. doi: 10.1007/s11934-014-0433-2
Jung, C. E., Chopyk, J., Shin, J. H., Lukacz, E. S., Brubaker, L., Schwanemann, L. K., et al. (2019). Benchmarking urine storage and collection conditions for evaluating the female urinary microbiome. Sci. Rep. 9, 13409. doi: 10.1038/s41598-019-49823-5
Kari, J. A. (2006). Neuropathic bladder as a cause of chronic renal failure in children in developing countries. Pediatr. Nephrol. 21, 517–520. doi: 10.1007/s00467-006-0034-5
Karstens, L., Asquith, M., Davin, S., Stauffer, P., Fair, D., Gregory, W. T., et al. (2016). Does the urinary microbiome play a role in urgency urinary incontinence and its severity? Front. Cell. Infection Microbiol. 6. doi: 10.3389/fcimb.2016.00078
Karstens, L., Siddiqui, N. Y., Zaza, T., Barstad, A., Amundsen, C. L., Sysoeva, T. A. (2021). Benchmarking DNA isolation kits used in analyses of the urinary microbiome. Sci. Rep. 11, 6186. doi: 10.1038/s41598-021-85482-1
Kim, J. K., Song, S. H., Jung, G., Song, B., Hong, S. K. (2022). Possibilities and limitations of using low biomass samples for urologic disease and microbiome research. Prostate Int. 10, 169–180. doi: 10.1016/j.prnil.2022.10.001
Klein, R. D., Hultgren, S. J. (2020). Urinary tract infections: microbial pathogenesis, host-pathogen interactions and new treatment strategies. Nat. Rev. Microbiol. 18, 211–226. doi: 10.1038/s41579-020-0324-0
Komesu, Y. M., Dinwiddie, D. L., Richter, H. E., Lukacz, E. S., Sung, V. W., Siddiqui, N. Y., et al. (2020). Defining the relationship between vaginal and urinary microbiomes. Am. J. Obstetrics Gynecology 222, 154.e1–154.e10. doi: 10.1016/j.ajog.2019.08.011
Komesu, Y. M., Richter, H. E., Dinwiddie, D. L., Siddiqui, N. Y., Sung, V. W., Lukacz, E. S., et al. (2017). Methodology for a vaginal and urinary microbiome study in women with mixed urinary incontinence. Int. Urogynecology J. 28, 711–720. doi: 10.1007/s00192-016-3165-7
Koukourikis, P., Papaioannou, M., Papanikolaou, D., Apostolidis, A. (2023). Urine biomarkers in the management of adult neurogenic lower urinary tract dysfunction: A systematic review. Diagnostics (Basel Switzerland) 13, 468. doi: 10.3390/diagnostics13030468
Kumar, S. J., Biswas, D. A. (2022). Anatomical aspects of neurogenic bladder and the approach in its management: A narrative review. Cureus 14, e31165. doi: 10.7759/cureus.31165
Lane, G., Gracely, A., Bassis, C., Greiman, S. E., Romo, P. B., Clemens, J. Q., et al. (2022). Distinguishing features of the urinary bacterial microbiome in patients with neurogenic lower urinary tract dysfunction. J. Urol. 207, 627–634. doi: 10.1097/JU.0000000000002274
Lee, K. W., Song, H. Y., Kim, Y. H. (2020). The microbiome in urological diseases. Invest. Clin. Urol. 61, 338–348. doi: 10.4111/icu.2020.61.4.338
Legaria, M. C., Barberis, C., Famiglietti, A., De Gregorio, S., Stecher, D., Rodriguez, C. H., et al. (2022). Urinary tract infections caused by anaerobic bacteria. Utility anaerobic urine culture. Anaerobe 78, 102636. doi: 10.1016/j.anaerobe.2022.102636
Leitner, L., McCallin, S., Kessler, T. M. (2021a). Bacteriophages: what role may they play in life after spinal cord injury? Spinal Cord 59, 967–970. doi: 10.1038/s41393-021-00636-2
Leitner, L., Ujmajuridze, A., Chanishvili, N., Goderdzishvili, M., Chkonia, I., Rigvava, S., et al. (2021b). Intravesical bacteriophages for treating urinary tract infections in patients undergoing transurethral resection of the prostate: a randomised, placebo-controlled, double-blind clinical trial. Lancet Infect. Dis. 21, 427–436. doi: 10.1016/S1473-3099(20)30330-3
Lerner, A., Shoenfeld, Y., Matthias, T. (2019). Probiotics: if it does not help it does not do any harm. Really? Microorganisms 7, 104. doi: 10.3390/microorganisms7040104
Lewis, A. L., Gilbert, N. M. (2020). Roles of the vagina and the vaginal microbiota in urinary tract infection: evidence from clinical correlations and experimental models. GMS Infect. Dis. 8, Doc02. doi: 10.3205/id000046
Linsenmeyer, T. A. (2018). Catheter-associated urinary tract infections in persons with neurogenic bladders. J. Spinal Cord Med. 41, 132–141. doi: 10.1080/10790268.2017.1415419
Linsenmeyer, T. A., Oakley, A. (2003). Accuracy of individuals with spinal cord injury at predicting urinary tract infections based on their symptoms. J. Spinal Cord Med. 26, 352–357. doi: 10.1080/10790268.2003.11753705
Liu, J., Luo, C., Xiao, W., Xu, T. (2023). Urinary tract infections and intermittent catheterization among patients with spinal cord injury in Chinese community. Sci. Rep. 13, 17683. doi: 10.1038/s41598-023-44697-0
Luu, T., Albarillo, F. S. (2022). Asymptomatic bacteriuria: prevalence, diagnosis, management, and current antimicrobial stewardship implementations. Am. J. Med. 135, e236–e244. doi: 10.1016/j.amjmed.2022.03.015
Malik, S., Sidhu, P. K., Rana, J. S., Nehra, K. (2020). Managing urinary tract infections through phage therapy: a novel approach. Folia Microbiologica 65, 217–231. doi: 10.1007/s12223-019-00750-y
McDonald, M., Kameh, D., Johnson, M. E., Johansen, T. E. B., Albala, D., Mouraviev, V. (2017). A head-to-head comparative phase II study of standard urine culture and sensitivity versus DNA next-generation sequencing testing for urinary tract infections. Rev. Urol. 19, 213–220. doi: 10.3909/riu0780
Milicevic, S., Sekulic, A., Nikolic, D., Tomasevic-Todorovic, S., Lazarevic, K., Pelemis, S., et al. (2024). Urinary tract infections in relation to bladder emptying in patients with spinal cord injury. J. Clin. Med. 13, 3898. doi: 10.3390/jcm13133898
Miller-Ensminger, T., Garretto, A., Brenner, J., Thomas-White, K., Zambom, A., Wolfe, A. J., et al. (2018). Bacteriophages of the urinary microbiome. J. Bacteriol. 200, e00738–e00717. doi: 10.1128/JB.00738-17
Min, K., Kim, H. T., Lee, E. H., Park, H., Ha, Y.-S. (2022). Bacteria for treatment: microbiome in bladder cancer. Biomedicines 10, 1783. doi: 10.3390/biomedicines10081783
Modena, B. D., Milam, R., Harrison, F., Cheeseman, J. A., Abecassis, M. M., Friedewald, J. J., et al. (2017). Changes in urinary microbiome populations correlate in kidney transplants with interstitial fibrosis and tubular atrophy documented in early surveillance biopsies. Am. J. Transplant. 17, 712–723. doi: 10.1111/ajt.14038
Moustafa, A., Li, W., Singh, H., Moncera, K. J., Torralba, M. G., Yu, Y., et al. (2018). Microbial metagenome of urinary tract infection. Sci. Rep. 8, 4333. doi: 10.1038/s41598-018-22660-8
Mula, D., Dervishi, R., Hoxha, R., Dervishi, L., Gashi, M. M., Muçaj, S., et al. (2024). A comparison of outcomes from antibiotic treatment with and without probiotics in 897 patients with lower urogenital tract infections, including cystitis, urethritis, prostatitis, and vulvovaginitis. Med. Sci. Monitor Basic Res. 30, e943939. doi: 10.12659/MSMBR.943939
Muok, A. R., Briegel, A. (2021). Intermicrobial hitchhiking: how nonmotile microbes leverage communal motility. Trends Microbiol. 29, 542–550. doi: 10.1016/j.tim.2020.10.005
Neugent, M. L., Hulyalkar, N. V., Nguyen, V. H., Zimmern, P. E., De Nisco, N. J. (2020). Advances in understanding the human urinary microbiome and its potential role in urinary tract infection. mBio 11, e00218–e00220. doi: 10.1128/mBio.00218-20
Nickel, J. C., Stephens, A., Landis, J. R., Mullins, C., van Bokhoven, A., Lucia, M. S., et al. (2016). Assessment of the lower urinary tract microbiota during symptom flare in women with urologic chronic pelvic pain syndrome: A MAPP network study. J. Urol. 195, 356–362. doi: 10.1016/j.juro.2015.09.075
Nickel, J. C., Stephens, A., Landis, J. R., Mullins, C., van Bokhoven, A., Anger, J. T., et al. (2020). Urinary fungi associated with urinary symptom severity among women with interstitial cystitis/bladder pain syndrome (IC/BPS). World J. Urol. 38, 433–446. doi: 10.1007/s00345-019-02764-0
Nooij, S., Vendrik, K. E. W., Zwittink, R. D., Ducarmon, Q. R., Keller, J. J., Kuijper, E. J., et al. (2024). Long-term beneficial effect of faecal microbiota transplantation on colonisation of multidrug-resistant bacteria and resistome abundance in patients with recurrent Clostridioides difficile infection. Genome Med. 16, 37. doi: 10.1186/s13073-024-01306-7
O’Hara, A. M., Shanahan, F. (2006). The gut flora as a forgotten organ. EMBO Rep. 7, 688–693. doi: 10.1038/sj.embor.7400731
Panicker, J. N. (2020). Neurogenic bladder: epidemiology, diagnosis, and management. Semin. Neurol. 40, 569–579. doi: 10.1055/s-0040-1713876
Penders, J., Huylenbroeck, A. a. Y., Everaert, K., Van Laere, M., Verschraegen, G. L. C. (2003). Urinary infections in patients with spinal cord injury. Spinal Cord 41, 549–552. doi: 10.1038/sj.sc.3101499
Perez-Carrasco, V., Soriano-Lerma, A., Soriano, M., Gutiérrez-Fernández, J., Garcia-Salcedo, J. A. (2021). Urinary microbiome: yin and yang of the urinary tract. Front. Cell. Infect. Microbiol. 11, 617002. doi: 10.3389/fcimb.2021.617002
Petrova, M. I., Imholz, N. C. E., Verhoeven, T. L. A., Balzarini, J., Van Damme, E. J. M., Schols, D., et al. (2016). Lectin-Like Molecules of Lactobacillus rhamnosus GG Inhibit Pathogenic Escherichia coli and Salmonella Biofilm Formation. PloS One 11, e0161337. doi: 10.1371/journal.pone.0161337
Petrova, M. I., Reid, G., Ter Haar, J. A. (2021). Lacticaseibacillus rhamnosus GR-1, a.k.a. Lactobacillus rhamnosus GR-1: Past and Future Perspectives. Trends Microbiol. 29, 747–761. doi: 10.1016/j.tim.2021.03.010
Pohl, H. G., Groah, S. L., Pérez-Losada, M., Ljungberg, I., Sprague, B. M., Chandal, N., et al. (2020). The urine microbiome of healthy men and women differs by urine collection method. Int. Neurourology J. 24, 41–51. doi: 10.5213/inj.1938244.122
Porcari, S., Benech, N., Valles-Colomer, M., Segata, N., Gasbarrini, A., Cammarota, G., et al. (2023). Key determinants of success in fecal microbiota transplantation: From microbiome to clinic. Cell Host Microbe 31, 712–733. doi: 10.1016/j.chom.2023.03.020
Porter, S. B., Johnston, B. D., Kisiela, D., Clabots, C., Sokurenko, E. V., Johnson, J. R. (2022). Bacteriophage Cocktail and Microcin-Producing Probiotic Escherichia coli Protect Mice Against Gut Colonization With Multidrug-Resistant Escherichia coli Sequence Type 131. Front. Microbiol. 13, 887799. doi: 10.3389/fmicb.2022.887799
Price, T. K., Dune, T., Hilt, E. E., Thomas-White, K. J., Kliethermes, S., Brincat, C., et al. (2016). The clinical urine culture: enhanced techniques improve detection of clinically relevant microorganisms. J. Clin. Microbiol. 54, 1216–1222. doi: 10.1128/JCM.00044-16
Price, T. K., Hilt, E. E., Thomas-White, K., Mueller, E. R., Wolfe, A. J., Brubaker, L. (2020a). The urobiome of continent adult women: a cross-sectional study. BJOG: an Int. J. obstetrics gynaecology 127, 193–201. doi: 10.1111/1471-0528.15920
Price, T. K., Wolff, B., Halverson, T., Limeira, R., Brubaker, L., Dong, Q., et al. (2020b). Temporal dynamics of the adult female lower urinary tract microbiota. mBio 11, e00475–e00420. doi: 10.1128/mBio.00475-20
Putonti, C., Shapiro, J. W., Ene, A., Tsibere, O., Wolfe, A. J. (2020). Comparative Genomic Study of Lactobacillus jensenii and the Newly Defined Lactobacillus mulieris Species Identifies Species-Specific Functionality. mSphere 5, e00560–e00520. doi: 10.1128/mSphere.00560-20
Rashidi, A., Ebadi, M., Rehman, T. U., Elhusseini, H., Kazadi, D., Halaweish, H., et al. (2024). Long- and short-term effects of fecal microbiota transplantation on antibiotic resistance genes: results from a randomized placebo-controlled trial. Gut Microbes 16, 2327442. doi: 10.1080/19490976.2024.2327442
Reid, G., Bruce, A. W. (2006). Probiotics to prevent urinary tract infections: the rationale and evidence. World J. Urol. 24, 28–32. doi: 10.1007/s00345-005-0043-1
Retchless, A. C., Itsko, M., Bazan, J. A., Turner, A. N., Hu, F., Joseph, S. J., et al. (2022). Evaluation of urethrotropic-clade meningococcal infection by urine metagenomic shotgun sequencing. J. Clin. Microbiol. 60, e0173221. doi: 10.1128/JCM.01732-21
Ruţa, F., Pribac, M., Mardale, E., Suciu, S., Maior, R., Bogdan, S., et al. (2024). Associations between gut microbiota dysbiosis and other risk factors in women with a history of urinary tract infections. Nutrients 16, 1753. doi: 10.3390/nu16111753
Sadahira, T., Wada, K., Araki, M., Mitsuhata, R., Yamamoto, M., Maruyama, Y., et al. (2021). Efficacy of Lactobacillus vaginal suppositories for the prevention of recurrent cystitis: A phase II clinical trial. Int. J. Urology: Off. J. Japanese Urological Assoc. 28, 1026–1031. doi: 10.1111/iju.14636
Santiago-Rodriguez, T. M., Ly, M., Bonilla, N., Pride, D. T. (2015). The human urine virome in association with urinary tract infections. Front. Microbiol. 6, 14. doi: 10.3389/fmicb.2015.00014
Sebastián Domingo, J. J., Sánchez Sánchez, C. (2018). From the intestinal flora to the microbiome. Rev. Esp. Enferm. Dig. 110, 51–56. doi: 10.17235/reed.2017.4947/2017
Sekito, T., Wada, K., Ishii, A., Iwata, T., Matsubara, T., Tomida, S., et al. (2023). Etiology of recurrent cystitis in postmenopausal women based on vaginal microbiota and the role of Lactobacillus vaginal suppository. Front. Microbiol. 14, 1187479. doi: 10.3389/fmicb.2023.1187479
Si, H., Yang, Q., Hu, H., Ding, C., Wang, H., Lin, X. (2021). Colorectal cancer occurrence and treatment based on changes in intestinal flora. Semin. Cancer Biol. 70, 3–10. doi: 10.1016/j.semcancer.2020.05.004
Stapleton, A. E., Au-Yeung, M., Hooton, T. M., Fredricks, D. N., Roberts, P. L., Czaja, C. A., et al. (2011). Randomized, placebo-controlled phase 2 trial of a Lactobacillus crispatus probiotic given intravaginally for prevention of recurrent urinary tract infection. Clin. Infect. Dis. 52, 1212–1217. doi: 10.1093/cid/cir183
Storm, D. W., Copp, H. L., Halverson, T. M., Du, J., Juhr, D., Wolfe, A. J. (2022). A Child’s urine is not sterile: A pilot study evaluating the Pediatric Urinary Microbiome. J. Pediatr. Urol. 18, 383–392. doi: 10.1016/j.jpurol.2022.02.025
Subramanian, A. (2024). Emerging roles of bacteriophage-based therapeutics in combating antibiotic resistance. Front. Microbiol. 15. doi: 10.3389/fmicb.2024.1384164
Sun, Q., Li, L., Zhou, H., Wu, Y., Gao, Y., Wu, B., et al. (2022). The detection of urinary viruses is associated with aggravated symptoms and altered bacteriome in female with overactive bladder. Front. Microbiol. 13, 984234. doi: 10.3389/fmicb.2022.984234
Sundén, F., Butler, D., Wullt, B. (2017). Triggered urine interleukin-6 correlates to severity of symptoms in nonfebrile lower urinary tract infections. J. Urol. 198, 107–115. doi: 10.1016/j.juro.2017.01.070
Sung, B. M., Oh, D.-J., Choi, M. H., Choi, H. M. (2018). Chronic kidney disease in neurogenic bladder. Nephrol. (Carlton Vic.) 23, 231–236. doi: 10.1111/nep.12990
Sybesma, W., Zbinden, R., Chanishvili, N., Kutateladze, M., Chkhotua, A., Ujmajuridze, A., et al. (2016). Bacteriophages as potential treatment for urinary tract infections. Front. Microbiol. 7, 465. doi: 10.3389/fmicb.2016.00465
Theisen, K. M., Mann, R., Roth, J. D., Pariser, J. J., Stoffel, J. T., Lenherr, S. M., et al. (2020). Frequency of patient-reported UTIs is associated with poor quality of life after spinal cord injury: a prospective observational study. Spinal Cord 58, 1274–1281. doi: 10.1038/s41393-020-0481-z
Thomas-White, K., Forster, S. C., Kumar, N., Van Kuiken, M., Putonti, C., Stares, M. D., et al. (2018). Culturing of female bladder bacteria reveals an interconnected urogenital microbiota. Nat. Commun. 9, 1557. doi: 10.1038/s41467-018-03968-5
Tkhruni, F. N., Aghajanyan, A. E., Balabekyan, T. R., Khachatryan, T. V., Karapetyan, K. J. (2020). Characteristic of bacteriocins of lactobacillus rhamnosus BTK 20-12 potential probiotic strain. Probiotics Antimicrob. Proteins 12, 716–724. doi: 10.1007/s12602-019-09569-y
Toh, S.-L., Lee, B. B., Ryan, S., Simpson, J. M., Clezy, K., Bossa, L., et al. (2019). Probiotics [LGG-BB12 or RC14-GR1] versus placebo as prophylaxis for urinary tract infection in persons with spinal cord injury [ProSCIUTTU]: a randomised controlled trial. Spinal Cord 57, 550–561. doi: 10.1038/s41393-019-0251-y
Toh, S.-L., Lee, B. B., Simpson, J. M., Rice, S. A., Kotsiou, G., Marial, O., et al. (2020). Effect of probiotics on multi-resistant organism colonisation in persons with spinal cord injury: secondary outcome of ProSCIUTTU, a randomised placebo-controlled trial. Spinal Cord 58, 755–767. doi: 10.1038/s41393-020-0420-z
Tractenberg, R. E., Groah, S. L., Frost, J. K., Rounds, A. K., Davis, E., Ljungberg, I. H., et al. (2021). Effects of intravesical lactobacillus rhamnosus GG on urinary symptom burden in people with neurogenic lower urinary tract dysfunction. PM R: J. injury function Rehabil. 13, 695–706. doi: 10.1002/pmrj.12470
Tractenberg, R. E., Groah, S. L., Rounds, A. K., Ljungberg, I. H., Schladen, M. M. (2018). Preliminary validation of a Urinary Symptom Questionnaire for individuals with Neuropathic Bladder using Intermittent Catheterization (USQNB-IC): A patient-centered patient reported outcome. PloS One 13, e0197568. doi: 10.1371/journal.pone.0197568
Wehedy, E., Murugesan, S., George, C. R., Shatat, I. F., Al Khodor, S. (2022). Characterization of the urinary metagenome and virome in healthy children. Biomedicines 10, 2412. doi: 10.3390/biomedicines10102412
Weyler, M., Jaekel, A., Kirschner-Hermanns, R., Kowollik, M., Zeller, F., Knuepfer, S. (2021). Electrochemically activated solution as bladder irrigation—An individual curative trial in patients with neurogenic lower urinary tract dysfunction and recurrent urinary infections. Neurourology Urodynamics 40, 1796–1803. doi: 10.1002/nau.24745
Wolfe, A. J., Toh, E., Shibata, N., Rong, R., Kenton, K., Fitzgerald, M., et al. (2012). Evidence of uncultivated bacteria in the adult female bladder. J. Clin. Microbiol. 50, 1376–1383. doi: 10.1128/JCM.05852-11
Wolff, B. J., Price, T. K., Joyce, C. J., Wolfe, A. J., Mueller, E. R. (2019). Oral probiotics and the female urinary microbiome: a double-blinded randomized placebo-controlled trial. Int. Urol. Nephrol. 51, 2149–2159. doi: 10.1007/s11255-019-02282-3
Worby, C. J., Schreiber, H. L., Straub, T. J., van Dijk, L. R., Bronson, R. A., Olson, B. S., et al. (2022). Longitudinal multi-omics analyses link gut microbiome dysbiosis with recurrent urinary tract infections in women. Nat. Microbiol. 7, 630–639. doi: 10.1038/s41564-022-01107-x
Wu, S.-Y., Jhang, J.-F., Liu, H.-H., Chen, J.-T., Li, J.-R., Chiu, B., et al. (2022). Long-term surveillance and management of urological complications in chronic spinal cord-injured patients. J. Clin. Med. 11, 7307. doi: 10.3390/jcm11247307
Wuethrich, I., Pelzer, W., Khodamoradi, Y., Vehreschild, M. J. G. T. (2021). The role of the human gut microbiota in colonization and infection with multidrug-resistant bacteria. Gut Microbes 13, 1–13. doi: 10.1080/19490976.2021.1911279
Wullt, B., Connell, H., Röllano, P., Månsson, W., Colleen, S., Svanborg, C. (1998). Urodynamic factors influence the duration of Escherichia coli bacteriuria in deliberately colonized cases. J. Urol. 159, 2057–2062. doi: 10.1016/S0022-5347(01)63246-4
Xu, H., Tamrat, N. E., Gao, J., Xu, J., Zhou, Y., Zhang, S., et al. (2021). Combined signature of the urinary microbiome and metabolome in patients with interstitial cystitis. Front. Cell. Infection Microbiol. 11, 711746. doi: 10.3389/fcimb.2021.711746
Yoo, J.-J., Song, J. S., Kim, W. B., Yun, J., Shin, H. B., Jang, M.-A., et al. (2022). Gardnerella vaginalis in recurrent urinary tract infection is associated with dysbiosis of the bladder microbiome. J. Clin. Med. 11, 2295. doi: 10.3390/jcm11092295
Yoon, Y. K., Suh, J. W., Kang, E.-J., Kim, J. Y. (2019). Efficacy and safety of fecal microbiota transplantation for decolonization of intestinal multidrug-resistant microorganism carriage: beyond Clostridioides difficile infection. Ann. Med. 51, 379–389. doi: 10.1080/07853890.2019.1662477
Yu, Y., Wang, W., Zhang, F. (2023). The next generation fecal microbiota transplantation: to transplant bacteria or virome. Advanced Sci. (Weinheim Baden-Wurttemberg Germany) 10, e2301097. doi: 10.1002/advs.202301097
Zhao, M., Qi, S., Sun, Y., Zheng, X. (2024). Comparison of polymerase chain reaction and next-generation sequencing with conventional urine culture for the diagnosis of urinary tract infections: A meta-analysis. Open Med. (Warsaw Poland) 19, 20240921. doi: 10.1515/med-2024-0921
Keywords: microbiome, urine, neurogenic bladder, urinary tract infections, diagnostics, treatment
Citation: Zhang J, Lei Y, Du H, Li Z, Wang X, Yang D, Gao F and Li J (2025) Exploring urinary microbiome: insights into neurogenic bladder and improving management of urinary tract infections. Front. Cell. Infect. Microbiol. 15:1512891. doi: 10.3389/fcimb.2025.1512891
Received: 17 October 2024; Accepted: 13 March 2025;
Published: 01 April 2025.
Edited by:
Christoph Gabler, Free University of Berlin, GermanyReviewed by:
Juan Xicohtencatl-Cortes, Hospital Infantil de México Federico Gómez, MexicoCopyright © 2025 Zhang, Lei, Du, Li, Wang, Yang, Gao and Li. This is an open-access article distributed under the terms of the Creative Commons Attribution License (CC BY). The use, distribution or reproduction in other forums is permitted, provided the original author(s) and the copyright owner(s) are credited and that the original publication in this journal is cited, in accordance with accepted academic practice. No use, distribution or reproduction is permitted which does not comply with these terms.
*Correspondence: Jianjun Li, Y3JyYzEwMEAxNjMuY29t
Disclaimer: All claims expressed in this article are solely those of the authors and do not necessarily represent those of their affiliated organizations, or those of the publisher, the editors and the reviewers. Any product that may be evaluated in this article or claim that may be made by its manufacturer is not guaranteed or endorsed by the publisher.
Research integrity at Frontiers
Learn more about the work of our research integrity team to safeguard the quality of each article we publish.