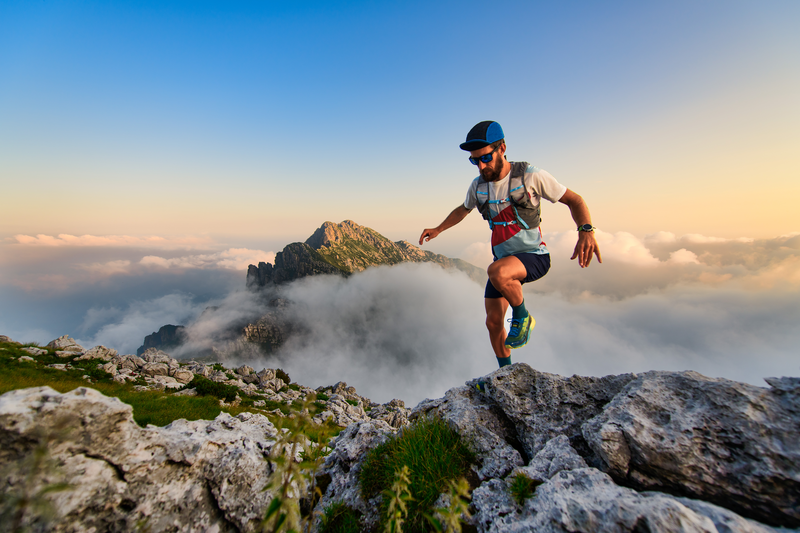
95% of researchers rate our articles as excellent or good
Learn more about the work of our research integrity team to safeguard the quality of each article we publish.
Find out more
ORIGINAL RESEARCH article
Front. Cell. Infect. Microbiol.
Sec. Clinical Infectious Diseases
Volume 15 - 2025 | doi: 10.3389/fcimb.2025.1509496
This article is part of the Research Topic Molecular mechanisms and clinical studies of multi-organ dysfunction in sepsis associated with pathogenic microbial infection View all 9 articles
The final, formatted version of the article will be published soon.
You have multiple emails registered with Frontiers:
Please enter your email address:
If you already have an account, please login
You don't have a Frontiers account ? You can register here
Objective: To investigate the diagnostic and prognostic value of miR-9-5p in peripheral blood mononuclear cells in sepsis patients.Methods: Differentially expressed miR-9-5p in sepsis were screened from a database and available literature. Subsequently, iBMDM cell validation was conducted and the expression level of miR-9-5p in peripheral blood mononuclear cells was determined using RT-qPCR in 69 sepsis patients and 30 non-sepsis patients with infections, 24 hours after ICU admission. A control group that comprised 35 healthy individuals, matched for age and sex, was set up from those who agreed to provide blood samples laboratory analysis.On admission to the ICU, the levels of miR-9-5p were significantly higher in sepsis patients (10.13 [3.21, 24.94]) than in non-sepsis patients with infections (2.08 [1.68, 3.18]) and healthy controls (0.86 [0.36, 1.88]) (p < 0.001). The miR-9-5p levels were positively correlated with the severity of the disease as indicated by the SOFA score (r=0.656, P<0.001). The AUC of miR-9-5p in predicting sepsis, septic shock, and in-hospital death was 0.825, 0.821, and 0.845, respectively.Elevated expression levels of miR-9-5p in peripheral blood mononuclear cells are significantly associated with an increased risk of sepsis and septic shock, and also indicate a higher risk of organ dysfunction.
Keywords: Sepsis, peripheral blood mononuclear cell, MicroRNA-9-5p, diagnosis, Prognosis prediction
Received: 11 Oct 2024; Accepted: 31 Mar 2025.
Copyright: © 2025 Ye, Wang, Ji, Li, Liang, Liu, Cao and Shao. This is an open-access article distributed under the terms of the Creative Commons Attribution License (CC BY). The use, distribution or reproduction in other forums is permitted, provided the original author(s) or licensor are credited and that the original publication in this journal is cited, in accordance with accepted academic practice. No use, distribution or reproduction is permitted which does not comply with these terms.
* Correspondence:
Miao Liu, Anhui Medical University, Hefei, China
Limian Cao, Anhui Medical University, Hefei, China
Min Shao, Anhui Medical University, Hefei, China
Disclaimer: All claims expressed in this article are solely those of the authors and do not necessarily represent those of their affiliated organizations, or those of the publisher, the editors and the reviewers. Any product that may be evaluated in this article or claim that may be made by its manufacturer is not guaranteed or endorsed by the publisher.
Research integrity at Frontiers
Learn more about the work of our research integrity team to safeguard the quality of each article we publish.