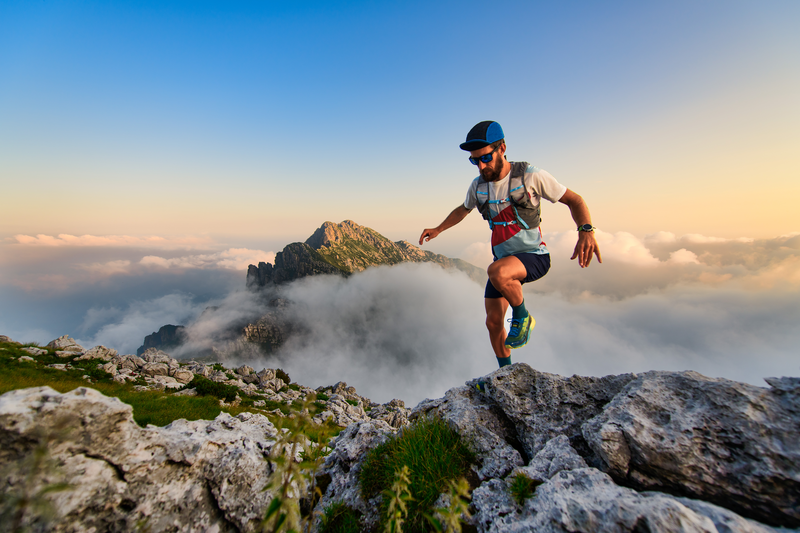
94% of researchers rate our articles as excellent or good
Learn more about the work of our research integrity team to safeguard the quality of each article we publish.
Find out more
ORIGINAL RESEARCH article
Front. Cell. Infect. Microbiol.
Sec. Clinical Microbiology
Volume 15 - 2025 | doi: 10.3389/fcimb.2025.1496829
This article is part of the Research Topic Development of point-of-care sensors for diagnosis of bacterial-associated infections View all 4 articles
The final, formatted version of the article will be published soon.
You have multiple emails registered with Frontiers:
Please enter your email address:
If you already have an account, please login
You don't have a Frontiers account ? You can register here
In light of the absence of rapid and precise diagnostic laboratory tests for the detection of Mycoplasma pneumoniae (MP), a prominent etiological agent implicated in a range of respiratory infections, we developed and evaluated a rapid and straightforward loop-mediated isothermal amplification (LAMP) assay targeting the MP community-acquired respiratory distress syndrome toxin (CARDS TX) gene. The LAMP assay was performed at 65 °C for a duration of 60 minutes, yielding a minimum detection concentration of MP CARDS TX at 0.4986 pg/μL. The assay exhibited no cross-reactivity with 13 other prevalent pathogens associated with respiratory infections or with other common bacterial toxin genes. To further substantiate the validity of the LAMP assay, 200 pharyngeal swabs or bronchoalveolar lavage (BAL) samples were collected from inpatients diagnosed with communityacquired pneumonia (CAP) between June 2021 and July 2022. The results were compared with those obtained by the quantitative real-time PCR (qPCR) method for verification purposes. Of the 200 clinical specimens, 11 exhibited positive results for MP by LAMP, and 10 displayed positive results for MP by qPCR (P=1.000). In summary, a sensitive, specific, straightforward, and expeditious LAMP method for CARDS TX identification was developed to facilitate rapid detection of MP in point-ofcare settings. This assay enables early and accurate diagnosis, even in resource-limited environments, which is important for proper antibiotic treatment and prognosis of MP infection.
Keywords: community-acquired respiratory distress syndrome toxin, Detection methods, Loop-mediated isothermal amplification assay, Mycoplasma pneumoniae, MP pneumonia
Received: 15 Sep 2024; Accepted: 14 Feb 2025.
Copyright: © 2025 Fang, Xie, Zhang, Zhang, Yang, Shi, Xin, Bai, Niu and Yuan. This is an open-access article distributed under the terms of the Creative Commons Attribution License (CC BY). The use, distribution or reproduction in other forums is permitted, provided the original author(s) or licensor are credited and that the original publication in this journal is cited, in accordance with accepted academic practice. No use, distribution or reproduction is permitted which does not comply with these terms.
* Correspondence:
Xin Yuan, Department of Respiratory and Critical Care Medicine, Fifth Medical Center of the PLA General Hospital, Beijing, China
Disclaimer: All claims expressed in this article are solely those of the authors and do not necessarily represent those of their affiliated organizations, or those of the publisher, the editors and the reviewers. Any product that may be evaluated in this article or claim that may be made by its manufacturer is not guaranteed or endorsed by the publisher.
Research integrity at Frontiers
Learn more about the work of our research integrity team to safeguard the quality of each article we publish.