- 1Department of Biomedical Science and Technology, School of Biological Sciences, Ramakrishna Mission Vivekananda Educational and Research Institute (RKMVERI), Kolkata, India
- 2Department of Agricultural Biotechnology, Ramakrishna Mission Vivekananda Educational and Research Institute (RKMVERI), Kolkata, India
- 3Department of Microbiology, University of Calcutta, Kolkata, India
- 4Chaudhary Charan Singh Haryana Agricultural University (CCS HAU), Hisar, Haryana, India
Amoebae, fascinatingly diverse protists, showcase a dual nature that positions them as both friends and foes in our world. These organisms, defined by their distinctive pseudopodia, span a spectrum from harmful to helpful. On the darker side, species like Entamoeba histolytica pose serious health risks, causing intestinal and liver diseases, while the infamous “brain-eating” Naegleria fowleri leads to fatal primary amoebic meningoencephalitis (PAM), with a daunting 97% mortality rate. Other free-living amoebae, including Acanthamoeba castellanii and Balamuthia mandrillaris, also threaten the human central nervous system. Yet, beyond these dangers, amoebae play critical ecological roles. They function as nature’s recyclers, decomposing organic material and nourishing aquatic ecosystems, while also serving as food for various organisms. Moreover, certain amoebae help control plant pathogens and offer insight into human disease, proving valuable as model organisms in biomedical research. This review sheds light on the complex, multifaceted world of amoebae, highlighting their dual role as pathogens and as key contributors to vital ecological processes, as well as their significant impact on research and their promising potential for enhancing human well-being.
1 Introduction
Amoebae, within the Protista kingdom, encompasses over 17,000 species, exhibiting diverse lifestyles from free-living to parasitic, thriving in both aquatic and terrestrial ecosystems, and predominantly preying on bacteria and algae, or as parasitic to humans and other animals (Rodríguez-Zaragoza, 1994; Dunn et al., 2017; Gao et al., 2019). These enigmatic creatures play multifaceted roles in nature and do not constitute a unified group; rather, they are dispersed across various eukaryotic supergroups. Many amoeboid organisms also have multiple morphologies in their lifecycles, such as flagellated forms of N. fowleri, reticulated forms of Corallomyxa, and multicellular slugs such as Dictyostelium discoidium, all of which play diverse roles ranging from their positive ecological contributions to their potential as threats to ecosystems and human health (Grell, 1988; Schaap, 2007; Dunn et al., 2017; Brunet et al., 2021; Kin and Schaap, 2021; Borkens, 2024). The beneficial aspects of amoebae reveal their crucial ecological importance as decomposers, thriving in aquatic environments where they break down organic matter and recycle essential nutrients (Sagova-Mareckova et al., 2021; Shi et al., 2021). Amoebae can directly and indirectly impact plants and having ability to kill plant pathogens (Long et al., 2018). Moreover, they serve as a fundamental food source for various organisms, including small fish and insects, and sustain intricate food chains (Gryseels et al., 2012; Long et al., 2018). In addition, some amoebae have developed symbiotic relationships with certain organisms, resulting in mutual benefits through nutrient exchange and protection (Shu et al., 2018; Sørensen et al., 2019). In recent years, there has been a growing acceptance of amoeba as a valuable model for investigating a wide range of human diseases (Nguyen et al., 2014; Ahmed Khan et al., 2015; Wu et al., 2020; Ghosh et al., 2022; Viennet et al., 2023). This recognition indicates the increasing relevance and utility of amoebae in biomedical research.
However, the detrimental aspects of amoebae highlight their potential to become pathogens, leading to serious health risks for both humans and animals. Notably, E. histolytica infections are highly prevalent in the tropical regions of the developing world, affecting millions of people. The disease can manifest as severe intestinal and liver ailments, with approximately 10% of patients experiencing life-threatening complications (Rao et al., 2009; Kantor et al., 2018).
The unsightly aspect of amoebae becomes starkly apparent in the form of a staggering mortality rate exceeding 97%, all attributable to N. fowleri, also known as the ‘brain-eating amoeba,’ causes primary amoebic meningoencephalitis (PAM), a fatal human disease (Salazar-Ardiles et al., 2022). In some instances, certain amoebae can transform into opportunistic invaders, disrupting native species and biodiversity within various ecosystems (Guimaraes et al., 2016; Betanzos et al., 2019). Among the deadliest pathogens are free-living amoebae (FLA) such as N. fowleri, A. castellanii, and B. mandrillaris (Güémez and García, 2021). Acanthamoeba and Balamuthia, both opportunistic free-living amoebae, cause granulomatous amoebic encephalitis (GAE) and can also cause disseminated disease in immunosuppressed patients (Schafer et al., 2015; Gray et al., 2016; Kalra et al., 2020; Kofman and Guarner, 2022). Acanthamoeba also causes corneal infection known as Acanthamoeba keratitis. It has been reported that more than 80% of patients with Acanthamoeba keratitis use contact lenses, and the infections start from contamination of contact lenses with Acanthamoeba (Kalra et al., 2020; Raghavan and Rammohan, 2024). Despite being serious human pathogens, these free-living amoebae are only poorly studied from both the identification and infection perspective (epidemiological) as well as diagnostic and therapeutic (clinical) perspectives. In our ecosystem, everything including humans, animals, plants, and the environment is intrinsically interconnected, and when some intervention occurs, mainly through the action of man himself, everyone suffers the consequences. In this regard, “One Health” approaches are becoming increasingly essential in the world we live in (Leal dos Santos et al., 2022; Viani et al., 2024). Infection by N. fowleri is highly lethal to humans and can also infect cattle; therefore, its implications for health approaches through the One Health concept are gaining attention (Figure 1A). A recent report highlighted that N. fowleri can also infect cattle, potentially increasing infections in humans, which is a significant concern. Daft et al. reported a case of fatal meningoencephalitis caused by N. fowleri in Holstein cattle (Daft et al., 2005). Since N. fowleri can infect cattle, these animals can serve as hosts where the amoeba may multiply, potentially increasing environmental contamination and thereby the risk of human infections. This underscores the need to consider the ecological importance of interactions between amoebae and other microorganisms within the ‘One Health’ Approach, emphasizing a comprehensive health strategy (Leal dos Santos et al., 2022). Infection by N. fowleri is extremely lethal to humans and can also infect cattle. Therefore, implications for health approaches through the One Health concept are emerging (Figure 1A). Recognizing their zoonotic nature, we must consider their implications for both animal and human health when addressing these pathogens. This review explores the multifaceted nature of amoebae, focusing on their roles as both beneficial organisms and potential threats to various ecosystems and human health.
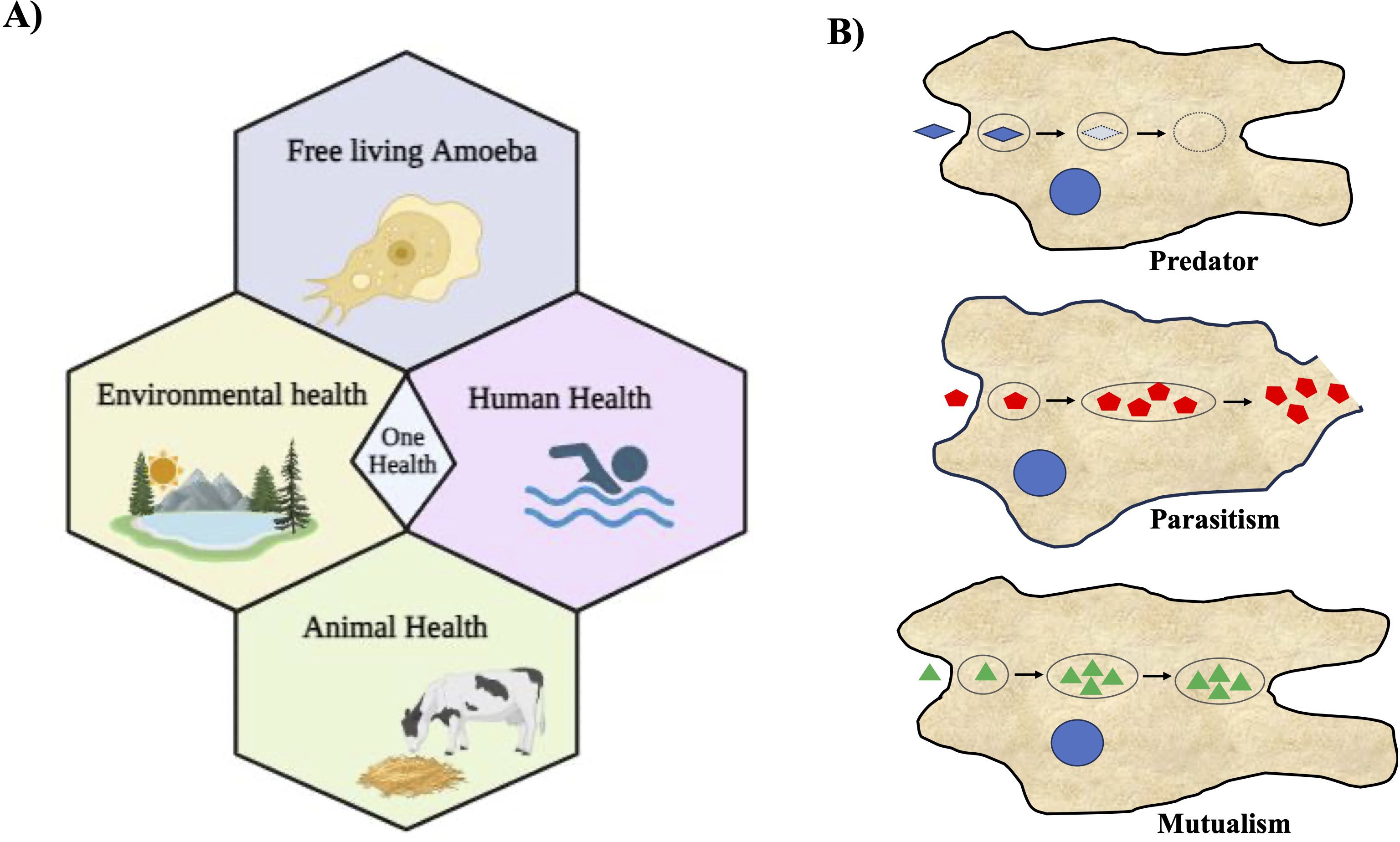
Figure 1. Free-living amoebae are vital in the One Health Approach and their diverse associations with microorganisms. (A) This illustration depicts the significance of free-living amoebae and their multifaceted implications within the context of the One Health Approach. It underscores the importance of recognizing the interplay between human, animal, and environmental health when considering the impact of these amoebae. It emphasizes the role of free-living amoebae in ecological processes and microbial communities, thereby contributing to environmental health. (B) Schematic illustration depicting various types of association of amoebae with other microorganisms. Three distinct types of amoeba-bacterium interactions are delineated: Predation, Parasitism, and Mutualism. In this representation, blue microorganisms symbolize prey, while red bacteria denote pathogenic species capable of replication and escape into the cytosol and the environment; and green represent the endosymbionts living within amoebae.
2 The harmful aspect of amoebae
Human Entamoeba infection is a parasitic disease caused by various species of the genus Entamoeba, with Entamoeba histolytica being the most common pathogenic species (Kantor et al., 2018). This infection primarily affects the gastrointestinal system and can lead to a range of clinical manifestations, including diarrhea and liver abscesses in severe cases (Johnson et al., 1968; Kagan, 1975; Matsubayashi et al., 2014; Cooper et al., 2015). Entamoeba is a global health concern, particularly in areas with poor sanitation and limited access to clean water (Nasrallah et al., 2022; Fu et al., 2023). It is estimated that over 50 million people worldwide are infected with E. histolytica (Bercu et al., 2007; Walters et al., 2019). The infection is most prevalent in tropical and subtropical regions, including parts of Africa, Asia, and Latin America. Risk factors for Entamoeba infection include poor sanitation and contamination of drinking water sources with human feces can facilitate the transmission of the parasite (Holtan, 1988; Mackey-Lawrence and Petri, 2011). Living conditions where living environments can increase the likelihood of person-to-person transmission of the parasite. Malnourished individuals may be more susceptible to severe forms of the disease and travelers to regions with a high prevalence of Entamoeba infection are at increased risk, especially if they do not follow hygiene recommendations (Petri et al., 2009; Morgado et al., 2016; Chidebelu and Nweze, 2020). E. histolytica possesses an array of virulence factors that enable it to colonize and invade the human host (Tillack et al., 2007; Padilla-Vaca and Anaya-Velázquez, 2010; Marie and Petri, 2014). These factors include specialized surface lectins and adhesins that facilitate adherence to and invasion of the host’s intestinal mucosa as well as the formation of durable cysts for transmission. The ability of the parasite to actively invade host tissues is aided by cysteine proteases and other enzymes that degrade host proteins, whereas its capacity to evade the host immune response is achieved through various mechanisms, such as altering cytokine profiles and phagocytosing immune cells. Furthermore, Gal/GalNAc lectin plays a pivotal role in adhesion, tissue-destructive lesions, and pathogenesis (Padilla-Vaca et al., 1999; Pacheco et al., 2004). The interplay of these virulence factors with host factors ultimately determines the outcome of E. histolytica infection, ranging from asymptomatic colonization to severe, potentially life-threatening diseases. Other symptoms include abdominal pain, cramping, and weight loss. Some infected individuals may remain asymptomatic carriers, shedding the parasite in their stool without experiencing any noticeable symptoms (Gathiram and Jackson, 1987; Tachibana et al., 2000; Blessmann et al., 2003).
E. histolytica alternates between cyst (environmentally resilient, transmittable) and trophozoite (disease-causing) stages and several transcription factors are crucial for this stage conversion (Ehrenkaufer et al., 2009; Manna et al., 2018; Manna and Singh, 2019). One of the most severe complications of E. histolytica infection is the development of liver abscesses (Usuda et al., 2022; Akhondi and Sabih, 2023; Jackson-Akers et al., 2023). This occurs when the parasite invades the intestinal wall and travels through the bloodstream to other organs including the liver and lungs. Abscesses can form in the liver, leading to symptoms such as fever, right upper abdominal pain, and jaundice. Entamoeba require high concentrations of iron for survival and reproduction. Ferritin is an iron storage protein that is mainly found in the liver and spleen of mammals. Thereby, the liver provides a plentiful source of iron, helping amoebae to multiply in that organ, making it a primary target for infection. Mortality associated with Entamoeba infection is primarily linked to severe cases and complications, particularly liver abscesses. If left untreated, liver abscesses can be life-threatening. Timely diagnosis and appropriate medical intervention are crucial for reducing the mortality rates associated with E. histolytica infection. As the world continues to face emerging infectious diseases, understanding the pathogenesis of amoeba-related diseases could help mitigate their impact. There is particular promise in the use of advanced molecular techniques, such as CRISPR-Cas9, to understand the genetic basis of pathogenicity and resistance in amoebae (Kangussu-Marcolino et al., 2021).
3 The unsightly aspect of amoebae
The world of amoebae is as intriguing as it is complex, showing a plethora of adaptations that have allowed them to thrive in a diverse array of environments. While their resilience and versatility often earn them admiration, there exists a less explored and rather unsettling facet – the “ugly” side of amoebae, where their adaptability can take on a sinister twist. Amoebae, seemingly innocuous single-celled organisms, have demonstrated the ability to endure and even flourish in adverse environmental conditions (Salazar-Ardiles et al., 2022). Their ability to tolerate extreme temperatures, varying levels of acidity, and even polluted or toxic environments is a testament to their tenacity (Lam et al., 2019). These adaptability to conditions that might be deemed inhospitable by many organisms underscores the remarkable nature of the amoebae. It is almost as if they hold the blueprint for survival, adapting their morphology and physiological processes to navigate through challenges that would prove fatal to others (Salazar-Ardiles et al., 2022). Certain amoebae, under specific circumstances, can exploit their adaptive processes to become opportunistic invaders. In doing so, they threaten the delicate equilibrium of ecosystems by outcompeting native species and disrupting biodiversity (Rodríguez-Zaragoza, 1994; Samba-Louaka et al., 2019). This metamorphosis from harmless inhabitants to invasive aggressors highlights the dual nature of their adaptability, one that can be both awe-inspiring and alarming.
Venturing further into the realm of concern, the category of free-living amoebae (FLA) unveils some of the most notorious amoebic pathogens (Visvesvara and Stehr-Green, 1990; el-Fakahany et al., 1997; da Rocha-Azevedo et al., 2009; Thomas et al., 2010). These FLA, including species such as N. fowleri, A castellanii, and B. mandrillaris, carry the potential for devastation and death. N. fowleri colloquially dubbed the ‘brain-eating amoeba,’ strikes terror with its grim reputation– a mortality rate that reaches a staggering 97% (Güémez and García, 2021).
The emergence of N. fowleri as a human pathogen is nothing short of nightmares. Its entry into the human body typically occurs through the nasal passages while swimming in warm freshwater, and from there, it invades the brain, causing severe inflammation and destruction (Grace et al., 2015; Güémez and García, 2021). PAM, the condition caused by this relentless amoeba, progresses rapidly, often with a span of only a few days between initial symptoms and fatal outcomes (Ghanchi et al., 2017). The severity of the disease leaves little room for medical intervention to have a meaningful impact. A. castellanii, another member of the FLA, is proficient in causing chaos. Although its modus operandi differs from that of N. fowleri, the outcomes are similar. It can lead to a variety of infections in humans, affecting the eyes, skin, and central nervous system (Martinez, 1991). Acanthamoeba keratitis, an eye infection, is particularly troubling because it can result in vision loss or even blindness if left untreated (Imam and Mahgoub, 2008). B. mandrillaris reveals yet another alarming and deadly aspect of amoebic infections. This rare but devastating pathogen causes granulomatous amoebic encephalitis (GAE), a brain infection that often proves fatal. Its ability to evade the immune system and persist undetected for long periods makes it particularly dangerous, adding to the growing concern about amoebic diseases in human health (Matin et al., 2008; Siddiqui and Khan, 2015).
4 The beneficial aspect of amoebae
Amoebae play a crucial role in nutrient cycling, ecosystem balance, and have potential applications in medical and biotechnological research. The relationship between amoebae and bacteria can range from mutual to parasitic interactions (Shi et al., 2021). Phagocytosis is the process by which a living cell engulfs other cells or particles. Amoeba feed on bacteria, fungi, or other protists by engulfing them by phagocytosis into their digestive vacuoles and thus, amoebae act as predators of microorganisms (Van der Henst et al., 2016). Amoebae can form various relationships with other microorganisms (Figure 1B). There were three primary categories of interactions between amoebae and different bacteria, as summarized in Table 1. These interactions are: i) Predation: in this interaction, amoebae act as predators, preying on other microorganisms as their primary food source (Anscombe and Singh, 1948; Eisenberg et al., 1989; Van der Henst et al., 2016; Brock et al., 2018; Bornier et al., 2021; Shi et al., 2021); ii) Parasitism: amoebae engage in parasitism when they encounter pathogenic bacteria, which can replicate within the amoeba, potentially causing harm, and can also escape into the amoeba’s cytosol and the surrounding environment (Bozue and Johnson, 1996; Greub and Raoult, 2004; Thomas et al., 2006; Cervero-Aragó et al., 2014; Delafont et al., 2014; Shi et al., 2021; Price et al., 2024); iii) Mutualism: some amoebae have mutualistic relationships with other organisms (DiSalvo et al., 2015; Shu et al., 2018; Garcia et al., 2019). These endosymbionts provide benefits to amoebae while also benefiting from the association. These three types of interactions illustrate the dynamic relationships that amoebae can establish with various microorganisms in their environments.
There is evidence that amoeba act as a training ground for human pathogens that support the growth of human pathogens, such as Legionella pneumophila (Molmeret et al., 2005). On the other hand, amoebae show parasitic interactions with human pathogenic bacteria via phagocytosis (Rodríguez-Zaragoza, 1994). This interaction between amoebae and microorganisms is useful in biomedical research. These amoebae can resist bacteria that are pathogenic to humans (Greub and Raoult, 2004). Phagocytosis can be influenced by prey size, the presence of surface molecular patterns, hydrophobicity, seasonal variation, and prey density. To protect against phagocytosis, the pathogens produce extracellular capsules. This phenomenon was confirmed by Klebsiella pneumoniae forming a capsule against D. discoideum amoeba (Rodríguez-Zaragoza, 1994). Therefore, pathogens form the same capsule to prevent phagocytosis by mammalian cells. Clinical isolates of K. pneumoniae and Acinetobactor baumannii are known to show thick capsules and polysaccharide capsule formation. During the experiment, a mutated mucoid strain was introduced to assess amoeba (Bornier et al., 2021). Mutation in the auto-kinase domain of Wzc caused regulatory defects in capsule production, but this did not make the process of isolation of amoeba from the bacteria difficult. It was thought earlier that capsule formation protects pathogens from phagocytosis (Bornier et al., 2021). Subsequently, it was shown that the capsule provided partial protection. Capsules altered the predation kinetics of amoeba. Bactericidal activity differed among the different amoeba isolates. Bactericidal activity means how fast amoeba can kill a bacterial population. Isolates of Vermamoeba vermiformis required 24 h to kill the bacterial population, whereas isolates of Acanthamoeba required almost 48 h to kill the bacterial population (Fouque et al., 2015). Isolates of Tetramitus and Vahlkampfia could reduce the bacterial population by first–10-100 folds but after some time, their bactericidal activity stopped, causing equilibrium. Free-living amoebae thrive in the natural environment, mainly in the soil; thus, they can be exposed to bacterial complexes and can discriminate and feed on specific bacteria. Our natural environment acts as a rich source of diverse amoeba with broad-spectrum predatory activities against human pathogens (Bornier et al., 2021). Amoebae have been increasingly recognized as a valuable model for studying various human diseases (Thewes et al., 2019). Despite the vast evolutionary distance between amoebae and humans, they share many cellular processes and genetic pathways, making them relevant models for investigating disease mechanisms and potential therapeutic targets (Eichinger et al., 2005). This article discusses the use of amoebae as a model for different human diseases and highlights the insights gained from these studies (Figure 2).
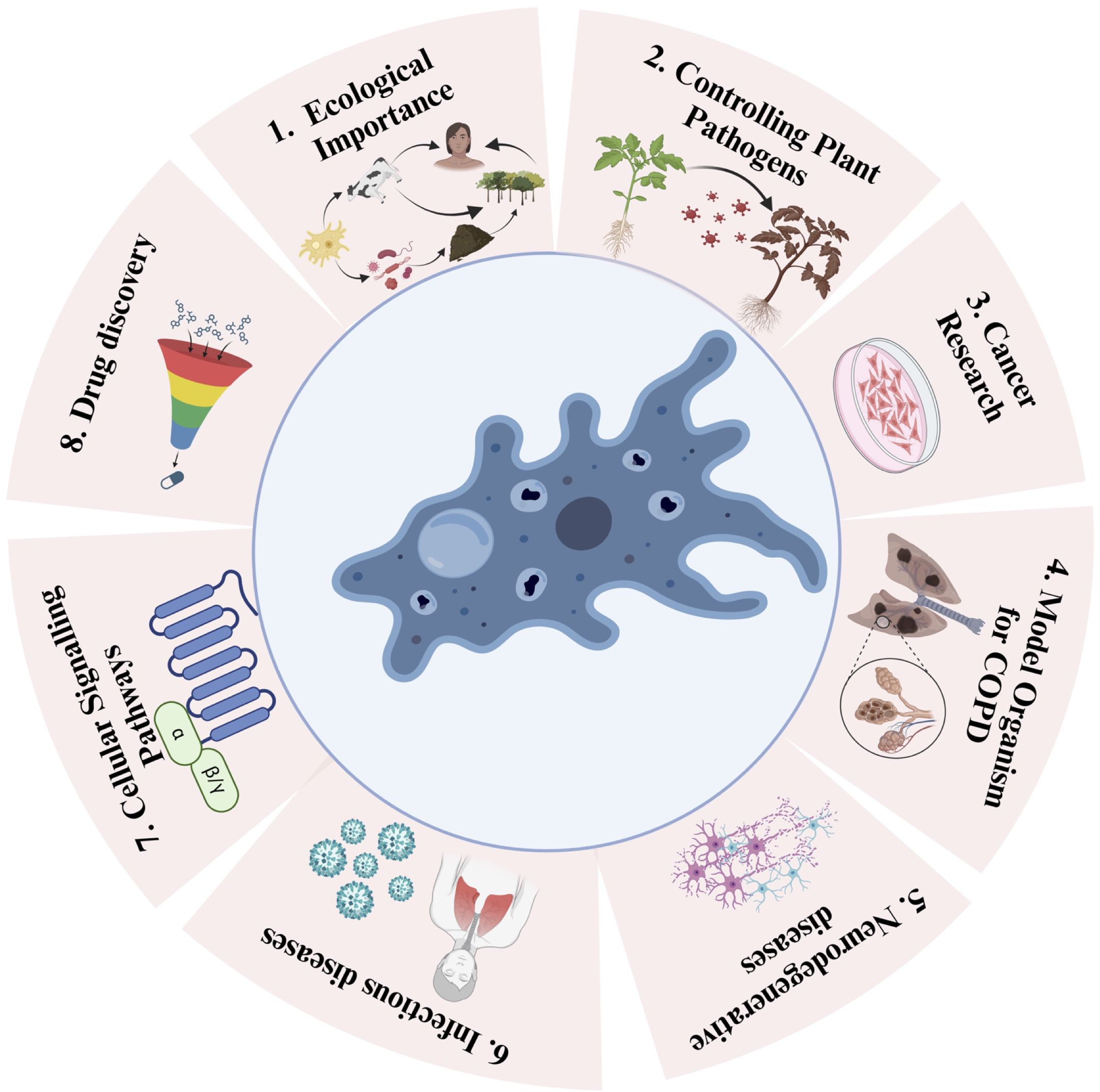
Figure 2. Schematic depiction of the versatile applications of amoebae and their positive impacts. Utilizing Amoebae for 1. Ecological Importance (e.g., Acanthamoeba sp, Dictiostelium sp, Vermamoeba sp), 2. Controlling Plant Pathogens (e.g., Acanthamoeba sp, Dictiostelium sp), 3. Cancer Research (e.g., Dictiostelium discoideum, Acanthamoeba sp), 4. Model in and Chronic Obstructive Pulmonary Disease (COPD) Studies (e.g., Dictiostelium discoideum), 5. Neurodegenerative diseases (e.g., Dictiostelium discoideum), 6. Infectious Diseases (e.g., Dictiostelium discoideum), 7. Cellular Signaling Pathways (e.g., Dictiostelium sp), and 8. Drug Discovery (e.g., Amoeba proteus).
4.1 Ecological importance
Amoebae play an essential role in nutrient cycling and ecological balance by participating in decomposition, regulating microbial populations, supporting soil health, and maintaining food webs in both terrestrial and aquatic environments (Clarholm, 1981). Amoebae are vital decomposers that consume bacteria, fungi and organic material, breaking down complex organic matter into simpler nutrients, which are then released into the soil and aquatic systems. This nutrient release is critical for supporting primary producers like plants and algae (Clarholm, 1981). These processes contribute to nutrient cycling by facilitating the recycling of nitrogen, phosphorus, and other essential nutrients, which are necessary for ecosystem productivity (Wilkinson, 2008). Amoebae also act as predators of bacteria and other microorganisms, helping to regulate microbial populations. By preventing any single microbial species from becoming overly dominant, amoebae help maintain microbial diversity, which is essential for ecological resilience and nutrient dynamics (Rodríguez-Zaragoza, 1994). This predation helps control pathogenic bacteria populations as well, indirectly protecting plant and animal health (Bonkowski and Brandt, 2002). In soils, amoebae contribute to soil structure and fertility by consuming microbes and releasing nutrients into the rhizosphere (root zone), which benefits plant growth. Amoebae are also involved in symbiotic relationships with bacteria and fungi that enhance nutrient availability in the soil, playing a fundamental role in humus formation, which is essential for soil health (Rønn et al., 2002). This activity improves soil moisture retention and nutrient availability, which supports a balanced microbial (Adl and Gupta, 2006). In aquatic systems, amoebae are integral to the microbial loop, where they recycle nutrients by consuming bacteria and returning nutrients to the water. This nutrient recycling supports primary producers like phytoplankton, which form the base of aquatic food webs, linking microbial communities to larger organisms and ensuring the flow of energy throughout the ecosystem (Sherr and Sherr, 1988; Hwang and Heath, 1997). Amoebae populations can be indicators of environmental health due to their sensitivity to changes in environmental conditions, such as nutrient levels, pollution, and habitat disturbances. Changes in amoebae populations may signal shifts in ecosystem health, making them valuable bioindicators in both terrestrial and aquatic environments (da Silva et al., 2022). Analyzing the ecological balance of amoebae and their persistence can be studied by using Geographic Information Systems (GIS) and remote sensing technologies to assess the ecological balance of amoebae populations and their persistence in various environments (Carella et al., 2022; Viani et al., 2023). By leveraging spatial data and environmental indicators, this approach aims to identify suitable habitats, monitor distribution patterns, and pinpoint key environmental proxies that influence amoebae ecology. The integration of satellite imagery, digital elevation models, and spatial analysis can help delineate areas of high suitability, shedding light on how factors such as temperature, humidity, soil composition, and vegetation cover impact the survival and distribution of amoebae species (Orusa et al., 2020; Carella et al., 2022). This information is critical for understanding the environmental dynamics that support the presence of these organisms, with potential applications in ecosystem management, disease control, and conservation strategies.
4.2 Use of amoeba controlling plant pathogen
Plant pathogens are usually bacteria, viruses, fungi, oomycetes, or nematodes that can cause diseases in plants, leading to significant economic losses in agriculture and horticulture (Wang et al., 2022). Controlling plant pathogens requires various strategies depending on the specific pathogen and the affected crop (Pandit et al., 2022). Implementing good agricultural practices, such as crop rotation, proper irrigation, and balanced fertilization, helps maintain plant health and reduce susceptibility to pathogens (McDonald and Stukenbrock, 2016; Samaddar et al., 2021). Natural host-plant resistant plant varieties are an effective control strategy for managing these pathogens. Biological control, by introducing natural enemies or beneficial microorganisms, suppresses pathogen growth (Pandit et al., 2022). Although chemical control with fungicides and bactericides can be used, careful application is essential to avoid harming the environment and non-target organisms. The enforcement of quarantine measures prevents pathogens from spreading into new areas. The use of amoebae as a means of controlling plant pathogens has gained attention as a promising biological control strategy (Pandit et al., 2022). Certain amoebae, particularly those belonging to the group known as free-living amoebae (FLA), exhibit predatory behavior towards plant pathogens (Bornier et al., 2021). When introduced into soil or plant environments, these amoebae actively feed on pathogenic microorganisms, thereby suppressing their growth. By preying on harmful fungi, bacteria, and other plant pathogens, amoebae help to maintain plant health and reduce the risk of diseases (Long et al., 2018, 2019). This eco-friendly approach offers a sustainable alternative to chemical control methods, minimizing environmental impacts, and safeguarding non-target organisms. Although research in this area is ongoing, harnessing the potential of amoebae as biocontrol agents holds promise for enhancing agricultural practices and crop protection (Rodríguez-Zaragoza, 1994; Long et al., 2019). The soil-borne fungus Rzioctonia solani can affect many important crop plants, including rice, where it causes sheath blight disease. It is a necrotic disease of rice that can be identified by lesions initiating at the apical ends of rice sheaths. A significant interaction between FLA and Rhizoctonia solani was observed by scanning electron microscopy, which helped to suppress the growth of fungal mycelia (Long et al., 2019). A bacteria called Xanthomonas oryzae causes bacterial blights in rice (Jain et al., 2023). According to previous studies, amoeba-bacterial interactions can lead to the biocontrol of plant pathogenic bacteria in plants. Five different amoebae were used for this study: Acanthamoeba polyphaga, A. lenticulata, A. castellanii, Vermamoeba vermiformis and D. discoideum with two pathovars of X. oryzae. The study reported that A. lenticulata, A. polyphaga, V. vermiformis and D. discoideum significantly reduced the number of bacteria after 24 h, and A. lenticulata and V. vermiformis had the strongest effect on pathogenicity. These amoebae lyse bacterial cells not through phagocytosis but by secreting some toxic compounds. However, sometimes X. oryzae forms a bacterial biofilm as a defense mechanism that cannot be degraded by the amoebae (Long et al., 2018).
4.3 Amoeba as a model in cancer research
Amoebae, particularly D. discoideum, have been employed in cancer research because of their ability to undergo a unique process called chemotaxis, which resembles the movement of cancer cells toward chemotactic gradients (Roussos et al., 2011). This similarity allows researchers to study cancer cell migration and invasion. Additionally, amoeba models have been employed to investigate the effects of potential anticancer drugs on cell migration and invasion, aiding in the identification of novel therapeutic agents (Roussos et al., 2011). To understand the process of differentiation or de-differentiation in the context of genes, proteins, and the environment for therapeutic and diagnostic uses, evolutionary lower organisms, such as Acanthamoeba can be used because they show cellular differentiation and de-differentiation properties that can explain the transition of normal cells to dormant cells in the primary stage of research (Figure 1B). The FLA contains two stages in its life cycle, a trophozoite stage where they are metabolically active and reproduce by mitotic division, and a cyst stage where they have minimal metabolic activity. Cyst formation occurs when the environment is unfavorable, and the return of favorable environmental conditions encourages cyst de-differentiation into the trophozoite form (Ahmed Khan et al., 2015). D. discoideum is an amoeba that has been used to study cancer-associated genes. Phosphatase and tensin homolog (PTEN) in cancer cells is one of the most frequently mutated tumor suppressor genes (Viennet et al., 2023). PTEN plays a crucial role in both cancer and amoeba cells (Chow and Baker, 2006). In cancer cells, mutations or alterations in PTEN can lead to uncontrolled cell growth and division, contributing to the development and progression of cancer. In amoeba cells, PTEN is involved in regulating cell growth and survival, similar to its role in normal mammalian cells. It helps maintain proper cell function and prevents excessive cell proliferation (Nguyen et al., 2014). The study of PTEN in both cancer and amoeba cells is important for understanding cellular processes, disease mechanisms, and potential therapeutic targets in cancer treatment (Nguyen et al., 2014; Mathavarajah et al., 2021). There are similarities between Entamoeba and cancer cells in the formation of Multinucleated Giant Cells (MGCs) and polyploid cells, making amoebae a promising model system for studying polyploidy in the context of cancer (Manna et al., 2020a, 2020b; Hazra and Manna, 2023; Hazra et al., 2023).
4.4 Model organism for chronic obstructive pulmonary disease
COPD is a complex respiratory disease that primarily affects humans and is often caused by smoking and exposure to pollutants. Scientists typically use animal models or in vitro cell cultures to study COPD and to develop potential therapies (Figure 1B). Recently, they discovered that the social amoeba D. discoideum reacts with cigarette smoke or cigarette smoke extract (CSE). Therefore, they can be used as models to identify new potential ways to adopt preventive strategies that will be helpful in the protection of airways against cigarette smoke (Wu et al., 2020; Ghosh et al., 2022). In COPD, as airway remodeling is linked to mitochondrial dysfunction, overexpression of canonical inner mitochondrial membrane transport proteins measured in the cDNA library can increase mitochondrial metabolism and protect against CSE (Kliment et al., 2021).
4.5 Amoeba in neurodegenerative disease research
While amoebae are considerably simpler than humans and lack a nervous system, they offer a unique platform for understanding fundamental cellular processes, particularly those related to protein aggregation, cellular stress responses, and mitochondrial dysfunction, which are central to the pathogenesis of neurodegenerative diseases. Several amoebae species share molecular and functional similarities with the neurons in the human brain. Researchers have utilized these amoebae models to study neurodegenerative diseases such as Alzheimer’s and Parkinson’s diseases (Haver and Scaglione, 2021; Storey et al., 2022). One of the key hallmarks of neurodegenerative diseases, such as Alzheimer’s, Parkinson’s, and Huntington’s, is the misfolding and aggregation of specific proteins (e.g., amyloid beta, alpha-synuclein, and huntingtin). Amoebae can be engineered to express proteins related to human diseases. This enables researchers to study the mechanisms underlying protein misfolding and aggregation in a simplified and controlled cellular environment. By expressing human disease-related proteins in amoebae, scientists can examine the pathological effects of these proteins on cellular function and viability, thereby providing valuable insights into disease mechanisms and potential treatment strategies (Santarriaga et al., 2015). Amoebae provide insight into how cells respond to stress. Neuronal cells in neurodegenerative diseases are often exposed to various stressors. Understanding how amoebae cope with stress can illuminate the pathways and mechanisms involved in cellular stress responses, which may be relevant to neurodegenerative conditions.
4.6 Amoeba as a host for infectious diseases studies
Amoebae, single-celled microorganisms often found in soil and water, have emerged as valuable models for the study of infectious diseases. Although they are relatively simple organisms, amoebae exhibit a surprising degree of sophistication in their interactions with pathogens, making them an excellent model system for understanding the fundamental principles of infectious disease dynamics, pathogen evolution, and host-pathogen interactions. Amoebae can serve as hosts for various pathogens, including bacteria and parasites, allowing researchers to study infectious diseases such as Legionnaires’ disease and amoebic dysentery (Shi et al., 2021). Amoebae can be infected by a wide range of pathogens including bacteria, viruses, fungi, and protozoa. Studying these interactions within amoebae provides insights into the initial stages of infection, mechanisms by which pathogens invade host cells, and how amoebae respond to these threats. Many pathogens that infect amoebae replicate within the host cell, similar to the way they infect higher organisms. This allows researchers to investigate the strategies employed by pathogens to evade the host immune system and manipulate host processes to ensure their survival and reproduction. This information can be extrapolated to understand more complex host-pathogen interactions in higher organisms. The interactions between amoebae and these pathogens shed light on the mechanisms of infection, pathogen survival, and immune evasion (Uribe-Querol and Rosales, 2020). Moreover, Amoebae can be used to study the evolution of virulence in various pathogens. As amoebae represent a simplified host environment, researchers can explore how pathogens evolve to become virulent in response to selective pressure. This can shed light on the broader patterns of virulence evolution in infectious diseases. In addition, the study of amoeba-resistant bacteria offers potential targets for developing new antibiotics (Oh et al., 2001).
4.7 Amoeba in cellular signaling pathway research
Despite their simplicity as single-celled organisms, amoebae possess conserved cellular signaling pathways that share remarkable similarities with those found in human cells. This similarity has made amoebae a valuable model for studying basic cellular functions and the roles of these pathways in human diseases (Shi et al., 2021). One example of the significance of studying these pathways in amoebae is the exploration of G protein-coupled receptors (GPCRs). GPCRs are a diverse family of cell surface receptors that play pivotal roles in a wide array of human diseases, including cardiovascular disorders and cancer. In amoebae, GPCRs are involved in crucial cellular processes, and their study has provided insights into the fundamental mechanisms underlying receptor signaling (Brewer et al., 2013; Pergolizzi et al., 2017). By understanding how these conserved pathways function in amoebae, researchers can gain a deeper understanding of their roles in human cells and their involvement in disease onset and progression. This knowledge offers a unique perspective on the potential therapeutic targets and strategies for a range of medical conditions. Consequently, the study of these conserved signaling pathways in amoebae has significant implications for advancing our understanding of human health and the development of treatments for diseases involving GPCR, such as cardiovascular disorders and cancer (Brewer et al., 2013; Pergolizzi et al., 2017).
4.8 Amoeba in drug discovery
Amoebae are incredibly adaptable and have been extensively studied in biology and genetics. One of the key advantages of using amoebae in drug screening is their ability to replicate certain aspects of cellular functions, making them an excellent surrogate for more complex organisms, such as humans. The amoeba model provides a cost-effective and efficient platform for drug screening. Researchers can test thousands of compounds for potential therapeutic effects against various diseases using amoeba-based assays (Duez et al., 1991). Amoeba-based assays involve exposing microorganisms to a wide range of chemical compounds to assess their potential therapeutic effects. These assays can be tailored to study specific diseases, and the screening process is highly versatile. Researchers can modify and optimize the assay conditions to mimic the cellular environment relevant to the disease being studied. This adaptability is a key factor that makes amoebae models invaluable for drug discovery. Thousands of chemical compounds were tested using a typical amoeba-based assay (Kangussu-Marcolino et al., 2019). These compounds include existing drugs, natural products, or new molecules that are synthesized specifically for drug discovery (Manna et al., 2010, 2013). Amoebae were exposed to these compounds, and their responses were carefully monitored and analyzed. This process allows researchers to identify compounds that have potential therapeutic effects against target diseases. Such assays can be high-throughput, enabling simultaneous screening of a large number of compounds (Harrison et al., 2015) (Figure 2). With the growing interest in natural compounds, the potential for discovering novel drugs or treatments from amoebae is high. Exploring their secondary metabolites and understanding their interaction with other microorganisms could lead to breakthroughs in the development of antimicrobial and anti-cancer therapies. Amoebae exhibit intriguing similarities with macrophages, which are immune cells responsible for the critical role of engulfing and destroying pathogens within the body (Esteban et al., 2015). Therefore, amoebae can be used to study host-pathogen interactions and immune responses (Thewes et al., 2019). The parallelism between the roles of amoebae and macrophages as phagocytes is remarkable. Both cell types are capable of engulfing and eliminating pathogens. The similarities in their cellular processes provide researchers with an opportunity to investigate host-pathogen interactions in a simplified yet informative model. Through amoeba-based studies, scientists have gained insights into how pathogens are recognized, internalized, and processed within host cells. These investigations offer a valuable perspective on the immune response, shedding light on how immune cells, including macrophages, combat infection. The findings from amoeba models can be translated into a better understanding of the molecular and cellular mechanisms underlying human immune responses, thereby contributing to the broader field of immunology. This knowledge contributes to a better understanding of infectious diseases and the development of new strategies to combat infections (Shi et al., 2021). In conclusion, the use of amoebae as models for different human diseases has proven to be a powerful tool in biomedical research. Their genetic and functional similarities with human cells allow scientists to investigate disease mechanisms, perform drug screenings, and gain valuable insights into host-pathogen interactions (Thewes et al., 2019; Samba-Louaka, 2021). As our understanding of amoebae biology and genetics continues to advance, their potential as model organisms for studying human diseases is likely to expand, contributing significantly to advancements in medical research and healthcare (Haver and Scaglione, 2021) (Figure 2).
5 Concluding and future perspective
Amoebae are a double-edged sword, offering significant ecological and biomedical benefits while posing serious health risks. Amoebae play a fascinating and multifaceted role in biology, ecology, and human health. This review sheds light on their diverse characteristics, encompassing both positive ecological contributions and potential threats to ecosystems and human well-being (Shi et al., 2021). In practical applications, amoebae have shown promise as a means of controlling plant pathogens in agriculture. Their predatory behavior towards plant pathogens offers an eco-friendly and sustainable alternative to chemical control methods, safeguarding the environment and non-target organisms (Viani et al., 2023). Furthermore, amoebae have emerged as valuable models for studying various human diseases, including cancer, neurodegenerative diseases, infectious diseases, and cellular signaling pathways. Their genetic and functional similarities to human cells make them relevant tools for investigating disease mechanisms and potential therapeutic targets (Haver and Scaglione, 2021). In wastewater management, amoebae demonstrate their potential as efficient biodegraders of pollutants, effective removal of pathogens, and contributors to sludge reduction. Their inclusion in wastewater treatment systems offers cost-effective and eco-friendly solutions, promotes sustainable methods for reducing environmental pollution, and reuses treated water. Balancing these positive and negative aspects are crucial for coexisting amoebae, and ongoing research promises advancements in utilizing their unique properties for ecological restoration, human health, and wastewater management. As we deepen our understanding of these organisms, the challenge will be to minimize their harmful impacts while maximizing their beneficial roles. Future research in amoebae biology, together with advancements in technology, offers promising potential for developing new therapeutic strategies, improving ecosystem management, and enhancing human health. Continued interdisciplinary efforts will be essential in harnessing the full potential of Amoebae in a way that benefits society as a whole.
Author contributions
SD: Validation, Visualization, Writing – original draft, Writing – review & editing. SH: Validation, Visualization, Writing – original draft, Writing – review & editing. ADe: Writing – original draft, Writing – review & editing. ADa: Writing – original draft, Writing – review & editing. LD: Writing – review & editing. SP: Writing – review & editing. KK: Writing – review & editing. MD: Writing – review & editing. AB: Writing – review & editing. DM: Writing – review & editing, Conceptualization, Funding acquisition, Supervision, Validation, Visualization, Writing – original draft.
Funding
The author(s) declare financial support was received for the research, authorship, and/or publication of this article. The author thanks the Science and Engineering Research Board (SERB) Core Research Grant (CRG/2023/005414).
Acknowledgments
We gratefully acknowledge all members of the Manna lab for helpful discussions and critical reading of the manuscript. The author also thanks the Science and Engineering Research Board (SERB) Core Research Grant (CRG/2023/005414).
Conflict of interest
The authors declare that the research was conducted in the absence of any commercial or financial relationships that could be construed as a potential conflict of interest.
Generative AI statement
The author(s) declare that no Generative AI was used in the creation of this manuscript.
Publisher’s note
All claims expressed in this article are solely those of the authors and do not necessarily represent those of their affiliated organizations, or those of the publisher, the editors and the reviewers. Any product that may be evaluated in this article, or claim that may be made by its manufacturer, is not guaranteed or endorsed by the publisher.
References
Adl, M. S., Gupta, V. S. (2006). Protists in soil ecology and forest nutrient cycling. Can. J. For. Res. 36, 1805–1817. doi: 10.1139/x06-056
Ahmed Khan, N., Baqir, H., Siddiqui, R. (2015). The immortal amoeba: a useful model to study cellular differentiation processes? Pathog. Glob. Health 109, 305–306. doi: 10.1080/20477724.2015.1103504
Anscombe, F. J., Singh, B. N. (1948). Limitation of bacteria by micro-predators in soil. Nature 161, 140–141. doi: 10.1038/161140a0
Bercu, T. E., Petri, W. A., Behm, J. W. (2007). Amebic colitis: new insights into pathogenesis and treatment. Curr. Gastroenterol. Rep. 9, 429–433. doi: 10.1007/s11894-007-0054-8
Betanzos, A., Bañuelos, C., Orozco, E. (2019). Host invasion by pathogenic amoebae: epithelial disruption by parasite proteins. Genes (Basel). 10, 123–152. doi: 10.3390/genes10080618
Blessmann, J., Ali, I. K. M., Nu, P. A. T., Dinh, B. T., Viet, T. Q. N., Van, A., et al. (2003). Longitudinal study of intestinal Entamoeba histolytica infections in asymptomatic adult carriers. J. Clin. Microbiol. 41, 4745–4750. doi: 10.1128/JCM.41.10.4745-4750.2003
Bonkowski, M., Brandt, F. (2002). Do soil protozoa enhance plant growth by hormonal effects? Soil Biol. Biochem. 34, 1709–1715. doi: 10.1016/S0038-0717(02)00157-8
Borkens, Y. (2024). The pathology of the brain eating amoeba naegleria fowleri. Indian J. Microbiol. 64, 1384–1394. doi: 10.1007/s12088-024-01218-5
Bornier, F., Zas, E., Potheret, D., Laaberki, M.-H., Coupat-Goutaland, B., Charpentier, X. (2021). Environmental free-living amoebae can predate on diverse antibiotic-resistant human pathogens. Appl. Environ. Microbiol. 87, e0074721. doi: 10.1128/AEM.00747-21
Bozue, J. A., Johnson, W. (1996). Interaction of Legionella pneumophila with Acanthamoeba castellanii: uptake by coiling phagocytosis and inhibition of phagosome-lysosome fusion. Infect. Immun. 64, 668–673. doi: 10.1128/iai.64.2.668-673.1996
Brewer, M. T., Agbedanu, P. N., Zamanian, M., Day, T. A., Carlson, S. A. (2013). Evidence for a bacterial lipopolysaccharide-recognizing G-protein-coupled receptor in the bacterial engulfment by Entamoeba histolytica. Eukaryot. Cell 12, 1433–1438. doi: 10.1128/EC.00150-13
Brock, D. A., Haselkorn, T. S., Garcia, J. R., Bashir, U., Douglas, T. E., Galloway, J., et al. (2018). Diversity of free-living environmental bacteria and their interactions with a bactivorous amoeba. Front. Cell. Infect. Microbiol. 8. doi: 10.3389/fcimb.2018.00411
Brunet, T., Albert, M., Roman, W., Coyle, M. C., Spitzer, D. C., King, N. (2021). A flagellate-to-amoeboid switch in the closest living relatives of animals. Elife 10:D380–88. doi: 10.7554/eLife.61037
Carella, E., Orusa, T., Viani, A., Meloni, D., Borgogno-Mondino, E., Orusa, R. (2022). An integrated, tentative remote-sensing approach based on NDVI entropy to model canine distemper virus in wildlife and to prompt science-based management policies. Animals 12, 1049. doi: 10.3390/ani12081049
Cervero-Aragó, S., Sommer, R., Araujo, R. M. (2014). Effect of UV irradiation (253.7 nm) on free Legionella and Legionella associated with its amoebae hosts. Water Res. 67, 299–309. doi: 10.1016/j.watres.2014.09.023
Chidebelu, P. E., Nweze, E. I. (2020). The persistence of amoebosis caused by Entamoeba histolytica in Nigeria and the role of malnutrition. Ann. Parasitol. 66, 271–282. doi: 10.17420/ap6603.265
Chow, L. M. L., Baker, S. J. (2006). PTEN function in normal and neoplastic growth. Cancer Lett. 241, 184–196. doi: 10.1016/j.canlet.2005.11.042
Clarholm, M. (1981). Protozoan grazing of bacteria in soil—impact and importance. Microb. Ecol. 7, 343–350. doi: 10.1007/BF02341429
Cooper, C. J., Fleming, R., Boman, D. A., Zuckerman, M. J. (2015). Varied clinical manifestations of amebic colitis. South. Med. J. 108, 676–681. doi: 10.14423/SMJ.0000000000000370
Daft, B. M., Visvesvara, G. S., Read, D. H., Kinde, H., Uzal, F. A., Manzer, M. D. (2005). Seasonal meningoencephalitis in Holstein cattle caused by Naegleria fowleri. J. Vet. Diagn. Invest. 17, 605–609. doi: 10.1177/104063870501700617
da Rocha-Azevedo, B., Tanowitz, H. B., Marciano-Cabral, F. (2009). Diagnosis of infections caused by pathogenic free-living amoebae. Interdiscip. Perspect. Infect. Dis. 2009, 251406. doi: 10.1155/2009/251406
da Silva, Y. G., Sampaio, G. S., Ramos, B. R. D., Freitas, Y., de, G. C., Nascimento, L., et al. (2022). Testate amoebae (Amorphea, Amoebozoa, Cercozoa) as bioindicators: a scientometric review. Acta Limnol. Bras. 34, 268–279. doi: 10.1590/s2179-975x0722
Delafont, V., Mougari, F., Cambau, E., Joyeux, M., Bouchon, D., Héchard, Y., et al. (2014). First evidence of amoebae–mycobacteria association in drinking water network. Environ. Sci. Technol. 48, 11872–11882. doi: 10.1021/es5036255
DiSalvo, S., Haselkorn, T. S., Bashir, U., Jimenez, D., Brock, D. A., Queller, D. C., et al. (2015). Burkholderia bacteria infectiously induce the proto-farming symbiosis of Dictyostelium amoebae and food bacteria. Proc. Natl. Acad. Sci. 112, E5029–E5037. doi: 10.1073/pnas.1511878112
Duez, P., Livaditis, A., Guissou, P. I., Sawadogo, M., Hanocq, M. (1991). Use of an Amoeba proteus model for in vitro cytotoxicity testing in phytochemical research. Application to Euphorbia hirta extracts. J. Ethnopharmacol. 34, 235–246. doi: 10.1016/0378-8741(91)90042-c
Dunn, J. D., Bosmani, C., Barisch, C., Raykov, L., Lefrançois, L. H., Cardenal-Muñoz, E., et al. (2017). Eat prey, live: dictyostelium discoideum as a model for cell-autonomous defenses. Front. Immunol. 8. doi: 10.3389/fimmu.2017.01906
Ehrenkaufer, G. M., Hackney, J. A., Singh, U. (2009). A developmentally regulated Myb domain protein regulates expression of a subset of stage-specific genes in Entamoeba histolytica. Cell. Microbiol. 11, 898–910. doi: 10.1111/j.1462-5822.2009.01300.x
Eichinger, L., Pachebat, J. A., Glöckner, G., Rajandream, M.-A., Sucgang, R., Berriman, M., et al. (2005). The genome of the social amoeba Dictyostelium discoideum. Nature 435, 43–57. doi: 10.1038/nature03481
Eisenberg, R. M., Hurd, L. E., Ketcham, R. B. (1989). The cellular slime mold guild and its bacterial prey: growth rate variation at the inter- and intraspecific levels. Oecologia 79, 458–462. doi: 10.1007/BF00378661
el-Fakahany, A. F., Fahmy, R. R., Mohamed, A. S. (1997). Free living amoebae as opportunistic parasites in immunocompromised hosts. J. Egypt. Soc Parasitol. 27, 515–527. doi: 10.1124/mol.52.1.172
Esteban, M. Á., Cuesta, A., Chaves-Pozo, E., Meseguer, J. (2015). Phagocytosis in teleosts. Implications of the new cells involved. Biol. (Basel). 4, 907–922. doi: 10.3390/biology4040907
Fouque, E., Yefimova, M., Trouilhé, M.-C., Quellard, N., Fernandez, B., Rodier, M.-H., et al. (2015). Morphological study of the encystment and excystment of vermamoeba vermiformis revealed original traits. J. Eukaryot. Microbiol. 62, 327–337. doi: 10.1111/jeu.12185
Fu, X., Zhong, Y., Chen, L., Ge, M., Yu, M., Sun, Y., et al. (2023). Global burden and trends of the Entamoeba infection-associated diseases from 1990 to 2019: An observational trend study. Acta Trop. 240, 106866. doi: 10.1016/j.actatropica.2023.106866
Gao, Z., Karlsson, I., Geisen, S., Kowalchuk, G., Jousset, A. (2019). Protists: puppet masters of the rhizosphere microbiome. Trends Plant Sci. 24, 165–176. doi: 10.1016/j.tplants.2018.10.011
Garcia, J. R., Larsen, T. J., Queller, D. C., Strassmann, J. E. (2019). Fitness costs and benefits vary for two facultative Burkholderia symbionts of the social amoeba, Dictyostelium discoideum. Ecol. Evol. 9, 9878–9890. doi: 10.1002/ece3.5529
Gathiram, V., Jackson, T. F. (1987). A longitudinal study of asymptomatic carriers of pathogenic zymodemes of Entamoeba histolytica. S. Afr. Med. J. 72, 669–672. doi: 10.5465/AME.1987.4275740
Ghanchi, N. K., Jamil, B., Khan, E., Ansar, Z., Samreen, A., Zafar, A., et al. (2017). Case series of naegleria fowleri primary ameobic meningoencephalitis from Karachi, Pakistan. Am. J. Trop. Med. Hyg. 97, 1600–1602. doi: 10.4269/ajtmh.17-0110
Ghosh, B., Nishida, K., Chandrala, L., Mahmud, S., Thapa, S., Swaby, C., et al. (2022). Epithelial plasticity in COPD results in cellular unjamming due to an increase in polymerized actin. J. Cell Sci. 135, 1054–1069. doi: 10.1242/jcs.258513
Grace, E., Asbill, S., Virga, K. (2015). Naegleria fowleri: pathogenesis, diagnosis, and treatment options. Antimicrob. Agents Chemother. 59, 6677–6681. doi: 10.1128/AAC.01293-15
Gray, M. A., Choy, C. H., Dayam, R. M., Ospina-Escobar, E., Somerville, A., Xiao, X., et al. (2016). Phagocytosis enhances lysosomal and bactericidal properties by activating the transcription factor TFEB. Curr. Biol. 26, 1955–1964. doi: 10.1016/j.cub.2016.05.070
Grell, V. K. G. (1988). Corallomyxa multipara n. sp., a Plasmodial Rhizopod from the East-Australian Coast. Arch. für Protistenkd. 136, 117–138. doi: 10.1016/S0003-9365(88)80001-0
Greub, G., Raoult, D. (2004). Microorganisms resistant to free-living amoebae. Clin. Microbiol. Rev. 17, 413–433. doi: 10.1128/CMR.17.2.413-433.2004
Gryseels, S., Amissah, D., Durnez, L., Vandelannoote, K., Leirs, H., De Jonckheere, J., et al. (2012). Amoebae as Potential Environmental Hosts for Mycobacterium ulcerans and Other Mycobacteria, but Doubtful Actors in Buruli Ulcer Epidemiology. PloS Negl. Trop. Dis. 6, e1764. doi: 10.1371/journal.pntd.0001764
Güémez, A., García, E. (2021). Primary amoebic meningoencephalitis by naegleria fowleri: pathogenesis and treatments. Biomolecules 11, 1320. doi: 10.3390/biom11091320
Guimaraes, A. J., Gomes, K. X., Cortines, J. R., Peralta, J. M., Peralta, R. H. S. (2016). Acanthamoeba spp. as a universal host for pathogenic microorganisms: One bridge from environment to host virulence. Microbiol. Res. 193, 30–38. doi: 10.1016/j.micres.2016.08.001
Harrison, C. F., Chiriano, G., Finsel, I., Manske, C., Hoffmann, C., Steiner, B., et al. (2015). Amoebae-based screening reveals a novel family of compounds restricting intracellular legionella pneumophila. ACS Infect. Dis. 1, 327–338. doi: 10.1021/acsinfecdis.5b00002
Haver, H. N., Scaglione, K. M. (2021). Dictyostelium discoideum as a model for investigating neurodegenerative diseases. Front. Cell. Neurosci. 15. doi: 10.3389/fncel.2021.759532
Hazra, S., Kalyan Dinda, S., Kumar Mondal, N., Hossain, S. R., Datta, P., Yasmin Mondal, A., et al. (2023). Giant cells: multiple cells unite to survive. Front. Cell. Infect. Microbiol. 13. doi: 10.3389/fcimb.2023.1220589
Hazra, S., Manna, D. (2023). “Transcriptional control in entamoeba: something old, something new,” in Pathobiology of Parasitic Protozoa: Dynamics and Dimensions (Springer Nature Singapore, Singapore), 155–173. doi: 10.1007/978-981-19-8225-5_8
Holtan, N. (1988). Amebiasis. The ancient scourge is still with us. Postgrad. Med. 83, 65–8, 72. doi: 10.1080/00325481.1988.11700302
Hwang, S.-J., Heath, R. T. (1997). The distribution of protozoa across a trophic gradient, factors controlling their abundance and importance in the plankton food web. J. Plankton Res. 19, 491–518. doi: 10.1093/plankt/19.4.491
Imam, A. M., Mahgoub, E. S. (2008). Blindness due to Acanthamoeba: first case report from Sudan. Int. J. Health Sci. (Qassim). 2, 163–166.
Jain, L., Kumar, V., Jain, S. K., Kaushal, P., Ghosh, P. K. (2023). Isolation of bacteriophages infecting Xanthomonas oryzae pv. oryzae and genomic characterization of novel phage vB_XooS_NR08 for biocontrol of bacterial leaf blight of rice. Front. Microbiol. 14. doi: 10.3389/fmicb.2023.1084025
Johnson, R. L., Rogers, J. W., Zinneman, H. H. (1968). Amebic liver abscess. Am. J. Dig. Dis. 13, 822–829. doi: 10.1007/BF02233099
Kagan, I. G. (1975). Pathogenicity of entamoeba histolytica. Infection 3, 96–98. doi: 10.1007/BF01641049
Kalra, S. K., Sharma, P., Shyam, K., Tejan, N., Ghoshal, U. (2020). Acanthamoeba and its pathogenic role in granulomatous amebic encephalitis. Exp. Parasitol. 208, 107788. doi: 10.1016/j.exppara.2019.107788
Kangussu-Marcolino, M. M., Ehrenkaufer, G. M., Chen, E., Debnath, A., Singh, U. (2019). Identification of plicamycin, TG02, panobinostat, lestaurtinib, and GDC-0084 as promising compounds for the treatment of central nervous system infections caused by the free-living amebae Naegleria, Acanthamoeba and Balamuthia. Int. J. Parasitol. Drugs Drug Resist. 11, 80–94. doi: 10.1016/j.ijpddr.2019.10.003
Kangussu-Marcolino, M. M., Morgado, P., Manna, D., Yee, H., Singh, U. (2021). Development of a CRISPR/Cas9 system in Entamoeba histolytica: proof of concept. Int. J. Parasitol. 51, 193–200. doi: 10.1016/j.ijpara.2020.09.005
Kantor, M., Abrantes, A., Estevez, A., Schiller, A., Torrent, J., Gascon, J., et al. (2018). Entamoeba histolytica: updates in clinical manifestation, pathogenesis, and vaccine development. Can. J. Gastroenterol. Hepatol. 2018, 4601420. doi: 10.1155/2018/4601420
Kin, K., Schaap, P. (2021). Evolution of multicellular complexity in the dictyostelid social amoebas. Genes (Basel). 12, 487. doi: 10.3390/genes12040487
Kliment, C. R., Nguyen, J. M. K., Kaltreider, M. J., Lu, Y., Claypool, S. M., Radder, J. E., et al. (2021). Adenine nucleotide translocase regulates airway epithelial metabolism, surface hydration and ciliary function. J. Cell Sci. 134, jcs257162. doi: 10.1242/jcs.257162
Kofman, A., Guarner, J. (2022). Infections caused by free-living amoebae. J. Clin. Microbiol. 60, e00228-21. doi: 10.1128/JCM.00228-21
Lam, C., He, L., Marciano-Cabral, F. (2019). The effect of different environmental conditions on the viability of naegleria fowleri amoebae. J. Eukaryot. Microbiol. 66, 752–756. doi: 10.1111/jeu.12719
Leal dos Santos, D., Chaúque, B. J. M., Virginio, V. G., Cossa, V. C., Pettan-Brewer, C., Schrekker, H. S., et al. (2022). Occurrence of Naegleria fowleri and their implication for health - a look under the One Health approaches. Int. J. Hyg. Environ. Health 246, 114053. doi: 10.1016/j.ijheh.2022.114053
Long, J. J., Jahn, C. E., Sánchez-Hidalgo, A., Wheat, W., Jackson, M., Gonzalez-Juarrero, M., et al. (2018). Interactions of free-living amoebae with rice bacterial pathogens Xanthomonas oryzae pathovars oryzae and oryzicola. PloS One 13, e0202941. doi: 10.1371/journal.pone.0202941
Long, J. J., Luna, E. K., Jackson, M., Wheat, W., Jahn, C. E., Leach, J. E. (2019). Interactions of free-living amoebae with the rice fungal pathogen, Rhizoctonia solani. BMC Res. Notes 12, 746. doi: 10.1186/s13104-019-4802-2
Manna, D., Dutta, P. K., Achari, B., Lohia, A. (2010). A Novel Galacto-Glycerolipid from Oxalis corniculata Kills Entamoeba histolytica and Giardia lamblia. Antimicrob. Agents Chemother. 54, 4825–4832. doi: 10.1128/AAC.00546-10
Manna, D., Ehrenkaufer, G. M., Lozano-Amado, D., Singh, U. (2020a). Entamoeba stage conversion: progress and new insights. Curr. Opin. Microbiol. 58, 62–68. doi: 10.1016/j.mib.2020.09.005
Manna, D., Grewal, J. S., Sarkar, B., Maiti, S., Lohia, A. (2013). Polyunsaturated fatty acids induce polarized submembranous F-actin aggregates and kill Entamoeba histolytica. Cytoskeleton. 70, 260–268. doi: 10.1002/cm.21105
Manna, D., Lentz, C. S., Ehrenkaufer, G. M., Suresh, S., Bhat, A., Singh, U. (2018). An NAD + -dependent novel transcription factor controls stage conversion in Entamoeba. Elife 7, e37912. doi: 10.7554/eLife.37912
Manna, D., Lozano-Amado, D., Ehrenkaufer, G., Singh, U. (2020b). The NAD+ Responsive transcription factor ERM-BP functions downstream of cellular aggregation and is an early regulator of development and heat shock response in entamoeba. Front. Cell. Infect. Microbiol. 10. doi: 10.3389/fcimb.2020.00363
Manna, D., Singh, U. (2019). Nuclear factor Y (NF-Y) modulates encystation in entamoeba via stage-specific expression of the NF-YB and NF-YC subunits. MBio. 10, e00737-19. doi: 10.1128/mBio.00737-19
Marie, C., Petri, W. A. (2014). Regulation of virulence of Entamoeba histolytica. Annu. Rev. Microbiol. 68, 493–520. doi: 10.1146/annurev-micro-091313-103550
Martinez, A. J. (1991). Infection of the central nervous system due to Acanthamoeba. Rev. Infect. Dis. 13 Suppl 5, S399–S402. doi: 10.1093/clind/13.supplement_5.s399
Mathavarajah, S., VanIderstine, C., Dellaire, G., Huber, R. J. (2021). Cancer and the breakdown of multicellularity: What Dictyostelium discoideum, a social amoeba, can teach us. Bioessays 43, e2000156. doi: 10.1002/bies.202000156
Matin, A., Siddiqui, R., Jayasekera, S., Khan, N. A. (2008). Increasing importance of Balamuthia mandrillaris. Clin. Microbiol. Rev. 21, 435–448. doi: 10.1128/CMR.00056-07
Matsubayashi, M., Suzuta, F., Terayama, Y., Shimojo, K., Yui, T., Haritani, M., et al. (2014). Ultrastructural characteristics and molecular identification of Entamoeba suis isolated from pigs with hemorrhagic colitis: implications for pathogenicity. Parasitol. Res. 113, 3023–3028. doi: 10.1007/s00436-014-3965-y
McDonald, B. A., Stukenbrock, E. H. (2016). Rapid emergence of pathogens in agro-ecosystems: global threats to agricultural sustainability and food security. Philos. Trans. R. Soc Lond. B. Biol. Sci. 371. doi: 10.1098/rstb.2016.0026
Molmeret, M., Horn, M., Wagner, M., Santic, M., Abu Kwaik, Y. (2005). Amoebae as training grounds for intracellular bacterial pathogens. Appl. Environ. Microbiol. 71, 20–28. doi: 10.1128/AEM.71.1.20-28.2005
Morgado, P., Manna, D., Singh, U. (2016). Recent advances in Entamoeba biology: RNA interference, drug discovery, and gut microbiome. F1000Research 5, 2578. doi: 10.12688/f1000research.9241.1
Nasrallah, J., Akhoundi, M., Haouchine, D., Marteau, A., Mantelet, S., Wind, P., et al. (2022). Updates on the worldwide burden of amoebiasis: A case series and literature review. J. Infect. Public Health 15, 1134–1141. doi: 10.1016/j.jiph.2022.08.013
Nguyen, H. N., Afkari, Y., Senoo, H., Sesaki, H., Devreotes, P. N., Iijima, M. (2014). Mechanism of human PTEN localization revealed by heterologous expression in Dictyostelium. Oncogene 33, 5688–5696. doi: 10.1038/onc.2013.507
Oh, Y. K., Kim, J. P., Yoon, H., Kim, J. M., Yang, J. S., Kim, C. K. (2001). Prolonged organ retention and safety of plasmid DNA administered in polyethylenimine complexes. Gene Ther. 8, 1587–1592. doi: 10.1038/sj.gt.3301516
Orusa, T., Orusa, R., Viani, A., Carella, E., Borgogno Mondino, E. (2020). Geomatics and EO data to support wildlife diseases assessment at landscape level: A pilot experience to map infectious keratoconjunctivitis in chamois and phenological trends in aosta valley (NW Italy). Remote Sens. 12, 3542. doi: 10.3390/rs12213542
Pacheco, J., Shibayama, M., Campos, R., Beck, D. L., Houpt, E., Petri, W. A., et al. (2004). In vitro and in vivo interaction of Entamoeba histolytica Gal/GalNAc lectin with various target cells: an immunocytochemical analysis. Parasitol. Int. 53, 35–47. doi: 10.1016/j.parint.2003.10.005
Padilla-Vaca, F., Anaya-Velázquez, F. (2010). Insights into Entamoeba histolytica virulence modulation. Infect. Disord. Drug Targets 10, 242–250. doi: 10.2174/187152610791591638
Padilla-Vaca, F., Ankri, S., Bracha, R., Koole, L. A., Mirelman, D. (1999). Down regulation of Entamoeba histolytica virulence by monoxenic cultivation with Escherichia coli O55 is related to a decrease in expression of the light (35-kilodalton) subunit of the Gal/GalNAc lectin. Infect. Immun. 67, 2096–2102. doi: 10.1128/IAI.67.5.2096-2102.1999
Pandit, M. A., Kumar, J., Gulati, S., Bhandari, N., Mehta, P., Katyal, R., et al. (2022). Major biological control strategies for plant pathogens. Pathog. (Basel Switzerland) 11. doi: 10.1016/j.jksus
Pergolizzi, B., Bozzaro, S., Bracco, E. (2017). G-protein dependent signal transduction and ubiquitination in dictyostelium. Int. J. Mol. Sci. 18. doi: 10.1007/s40122-017-0068-3
Petri, W. A., Mondal, D., Peterson, K. M., Duggal, P., Haque, R. (2009). Association of malnutrition with amebiasis. Nutr. Rev. 67 Suppl 2, S207–S215. doi: 10.1111/j.1753-4887.2009.00242.x
Price, C. T. D., Hanford, H. E., Al-Quadan, T., Santic, M., Shin, C. J., Da’as, M. S. J., et al. (2024). Amoebae as training grounds for microbial pathogens. MBio 15. doi: 10.1128/mbio.00827-24
Raghavan, A., Rammohan, R. (2024). Acanthamoeba keratitis – A review. Indian J. Ophthalmol. 72, 473–482. doi: 10.4103/IJO.IJO_2627_23
Rao, S., Solaymani-Mohammadi, S., Petri, W. A., Parker, S. K. (2009). Hepatic amebiasis: a reminder of the complications. Curr. Opin. Pediatr. 21, 145–149. doi: 10.1097/MOP.0b013e32831ef249
Rodríguez-Zaragoza, S. (1994). Ecology of free-living amoebae. Crit. Rev. Microbiol. 20, 225–241. doi: 10.3109/10408419409114556
Rønn, R., McCaig, A. E., Griffiths, B. S., Prosser, J. I. (2002). Impact of protozoan grazing on bacterial community structure in soil microcosms. Appl. Environ. Microbiol. 68, 6094–6105. doi: 10.1128/AEM.68.12.6094-6105.2002
Roussos, E. T., Condeelis, J. S., Patsialou, A. (2011). Chemotaxis in cancer. Nat. Rev. Cancer 11, 573–587. doi: 10.1038/nrc3078
Sagova-Mareckova, M., Boenigk, J., Bouchez, A., Cermakova, K., Chonova, T., Cordier, T., et al. (2021). Expanding ecological assessment by integrating microorganisms into routine freshwater biomonitoring. Water Res. 191, 116767. doi: 10.1016/j.watres.2020.116767
Salazar-Ardiles, C., Asserella-Rebollo, L., Andrade, D. C. (2022). Free-living amoebas in extreme environments: the true survival in our planet. BioMed. Res. Int. 2022, 2359883. doi: 10.1155/2022/2359883
Samaddar, S., Karp, D. S., Schmidt, R., Devarajan, N., McGarvey, J. A., Pires, A. F. A., et al. (2021). Role of soil in the regulation of human and plant pathogens: soils’ contributions to people. Philos. Trans. R. Soc Lond. B. Biol. Sci. 376, 20200179. doi: 10.1098/rstb.2020.0179
Samba-Louaka, A. (2021). Amoebae as targets for toxins or effectors secreted by mammalian pathogens. Toxins (Basel). 13, 526. doi: 10.3390/toxins13080526
Samba-Louaka, A., Delafont, V., Rodier, M.-H., Cateau, E., Héchard, Y. (2019). Free-living amoebae and squatters in the wild: ecological and molecular features. FEMS Microbiol. Rev. 43, 415–434. doi: 10.1093/femsre/fuz011
Santarriaga, S., Petersen, A., Ndukwe, K., Brandt, A., Gerges, N., Bruns Scaglione, J., et al. (2015). The social amoeba dictyostelium discoideum is highly resistant to polyglutamine aggregation. J. Biol. Chem. 290, 25571–25578. doi: 10.1074/jbc.M115.676247
Schaap, P. (2007). Evolution of size and pattern in the social amoebas. BioEssays 29, 635–644. doi: 10.1002/bies.20599
Schafer, K. R., Shah, N., Almira-Suarez, M. I., Reese, J. M., Hoke, G. M., Mandell, J. W., et al. (2015). Disseminated balamuthia mandrillaris infection. J. Clin. Microbiol. 53, 3072–3076. doi: 10.1128/JCM.01549-15
Sherr, E., Sherr, B. (1988). Role of microbes in pelagic food webs: A revised concept. Limnol. Oceanogr. 33, 1225–1227. doi: 10.4319/lo.1988.33.5.1225
Shi, Y., Queller, D. C., Tian, Y., Zhang, S., Yan, Q., He, Z., et al. (2021). The ecology and evolution of amoeba-bacterium interactions. Appl. Environ. Microbiol. 87, 327–337. doi: 10.1128/AEM.01866-20
Shu, L., Brock, D. A., Geist, K. S., Miller, J. W., Queller, D. C., Strassmann, J. E., et al. (2018). Symbiont location, host fitness, and possible coadaptation in a symbiosis between social amoebae and bacteria. Elife 7, arXiv:1809.01286v3. doi: 10.7554/eLife.42660
Siddiqui, R., Khan, N. A. (2015). Balamuthia mandrillaris: Morphology, biology, and virulence. Trop. Parasitol. 5, 15–22. doi: 10.4103/2229-5070.149888
Sørensen, M. E. S., Lowe, C. D., Minter, E. J. A., Wood, A. J., Cameron, D. D., Brockhurst, M. A. (2019). The role of exploitation in the establishment of mutualistic microbial symbioses. FEMS Microbiol. Lett. 366, 31. doi: 10.1093/femsle/fnz148
Storey, C. L., Williams, R. S. B., Fisher, P. R., Annesley, S. J. (2022). Dictyostelium discoideum: A model system for neurological disorders. Cells 11, 858–872. doi: 10.3390/cells11030463
Tachibana, H., Kobayashi, S., Nagakura, K., Kaneda, Y., Takeuchi, T. (2000). Asymptomatic cyst passers of Entamoeba histolytica but not Entamoeba dispar in institutions for the mentally retarded in Japan. Parasitol. Int. 49, 31–35. doi: 10.1016/s1383-5769(99)00032-x
Thewes, S., Soldati, T., Eichinger, L. (2019). Editorial: amoebae as host models to study the interaction with pathogens. Front. Cell. Infect. Microbiol. 9. doi: 10.3389/fcimb.2019.00047
Thomas, V., Casson, N., Greub, G. (2006). Criblamydia sequanensis, a new intracellular Chlamydiales isolated from Seine river water using amoebal co-culture. Environ. Microbiol. 8, 2125–2135. doi: 10.1111/j.1462-2920.2006.01094.x
Thomas, V., McDonnell, G., Denyer, S. P., Maillard, J.-Y. (2010). Free-living amoebae and their intracellular pathogenic microorganisms: risks for water quality. FEMS Microbiol. Rev. 34, 231–259. doi: 10.1111/j.1574-6976.2009.00190.x
Tillack, M., Biller, L., Irmer, H., Freitas, M., Gomes, M. A., Tannich, E., et al. (2007). The Entamoeba histolytica genome: primary structure and expression of proteolytic enzymes. BMC Genomics 8, 170. doi: 10.1186/1471-2164-8-170
Uribe-Querol, E., Rosales, C. (2020). Immune response to the enteric parasite entamoeba histolytica. Physiol. (Bethesda). 35, 244–260. doi: 10.1152/physiol.00038.2019
Usuda, D., Tsuge, S., Sakurai, R., Kawai, K., Matsubara, S., Tanaka, R., et al. (2022). Amebic liver abscess by Entamoeba histolytica. World J. Clin. cases 10, 13157–13166. doi: 10.12998/wjcc.v10.i36.13157
Van der Henst, C., Scrignari, T., Maclachlan, C., Blokesch, M. (2016). An intracellular replication niche for Vibrio cholerae in the amoeba Acanthamoeba castellanii. ISME J. 10, 897–910. doi: 10.1038/ismej.2015.165
Viani, A., Orusa, T., Borgogno-Mondino, E., Orusa, R. (2023). Snow metrics as proxy to assess sarcoptic mange in wild boar: preliminary results in aosta valley (Italy). Life 13, 987. doi: 10.3390/life13040987
Viani, A., Orusa, T., Borgogno-Mondino, E., Orusa, R. (2024). A one health google earth engine web-GIS application to evaluate and monitor water quality worldwide. Euro-Mediterranean J. Environ. Integr. doi: 10.1007/s41207-024-00528-w
Viennet, T., Rodriguez Ospina, S., Lu, Y., Cui, A., Arthanari, H., Dempsey, D. R. (2023). Chemical and structural approaches to investigate PTEN function and regulation. Methods Enzymol. 682, 289–318. doi: 10.1016/bs.mie.2022.09.007
Visvesvara, G. S., Stehr-Green, J. K. (1990). Epidemiology of free-living ameba infections. J. Protozool. 37, 25S–33S. doi: 10.1111/j.1550-7408.1990.tb01142.x
Walters, H. A., Welter, B. H., Sullivan, W. J., Temesvari, L. A. (2019). Phosphorylation of eukaryotic initiation factor-2α in response to endoplasmic reticulum and nitrosative stress in the human protozoan parasite, Entamoeba histolytica. Mol. Biochem. Parasitol. 234, 111223. doi: 10.1016/j.molbiopara.2019.111223
Wang, Y., Sun, X., Zhang, Z., Pan, B., Xu, W., Zhang, S. (2022). Revealing the early response of pear (Pyrus bretschneideri Rehd) leaves during Botryosphaeria dothideainfection by transcriptome analysis. Plant Sci. 315, 111146. doi: 10.1016/j.plantsci.2021.111146
Wilkinson, D. M. (2008). Testate amoebae and nutrient cycling: peering into the black box of soil ecology. Trends Ecol. Evol. 23, 596–599. doi: 10.1016/j.tree.2008.07.006
Wu, K., Luan, G., Xu, Y., Shen, S., Qian, S., Zhu, Z., et al. (2020). Cigarette smoke extract increases mitochondrial membrane permeability through activation of adenine nucleotide translocator (ANT) in lung epithelial cells. Biochem. Biophys. Res. Commun. 525, 733–739. doi: 10.1016/j.bbrc.2020.02.160
Keywords: amoeba, protozoa, FLA (free-living amoeba), symbiotic, predators, primary amoebic meningoencephalitis (PAM), chronic obstructive pulmonary disease (COPD)
Citation: Dinda SK, Hazra S, De A, Datta A, Das L, Pattanayak S, Kumar K, Dey MD, Basu A and Manna D (2024) Amoebae: beyond pathogens- exploring their benefits and future potential. Front. Cell. Infect. Microbiol. 14:1518925. doi: 10.3389/fcimb.2024.1518925
Received: 29 October 2024; Accepted: 20 November 2024;
Published: 18 December 2024.
Edited by:
Riccardo Orusa, Experimental Zooprophylactic Institute for Piedmont, Liguria and Valle d’Aosta (IZSTO), ItalyReviewed by:
Tommaso Orusa, Experimental Zooprophylactic Institute of Abruzzo and Molise G. Caporale, ItalyAscel Samba-Louaka, University of Poitiers, France
Annalisa Viani, Experimental Zooprophylactic Institute for Piedmont, Liguria and Valle d’Aosta (IZSPLVA), Italy
Copyright © 2024 Dinda, Hazra, De, Datta, Das, Pattanayak, Kumar, Dey, Basu and Manna. This is an open-access article distributed under the terms of the Creative Commons Attribution License (CC BY). The use, distribution or reproduction in other forums is permitted, provided the original author(s) and the copyright owner(s) are credited and that the original publication in this journal is cited, in accordance with accepted academic practice. No use, distribution or reproduction is permitted which does not comply with these terms.
*Correspondence: Dipak Manna, ZGlwYWsubWFubmFAZ20ucmttdnUuYWMuaW4=