- 1Department of Parasitology, Xiangya School of Medicine, Central South University, Changsha, China
- 2Key Laboratory of Spine and Spinal Cord Injury Repair and Regeneration of Ministry of Education, Tongji Hospital, Clinical Center for Brain and Spinal Cord Research, School of Medicine, Tongji University, Shanghai, China
- 3Department of Parasitology, School of Medicine, Northwest University, Xi’an, Shanxi, China
- 4Laboratory of Molecular Parasitology, The Key Laboratory of Arrhythmias of the Ministry of Education of China, Research Center for Translational Medicine, Shanghai East Hospital, Clinical Center for Brain and Spinal Cord Research, School of Medicine, Tongji University, Shanghai, China
- 5Department of General Manager Office, Hunan Xingchen Biotechnology Company, Yongzhou, China
Introduction: Plasmodium falciparum is the most damaging malaria pathogen and brings a heavy burden to global health. Host switching and morphological changes in P. falciparum are dependent on an effective gene expression regulatory system. C5 methylation of cytosines is a common RNA modification in eukaryotes, and the NSUN family are essential m5C modification executors. Currently, little is known about this family in Plasmodium spp. In this study, we focus on exploring the function of PfNSUN1 protein.
Methods: An efficient CRISPR/Cas9 gene editing technique was applied to construct the PfNSUN1 knockdown strain. The knockdown efficiency was confirmed by growth curves and western blot experiments. The knockdown transcriptome data was acquired to find differentially expressed genes, and target genes of PfNSUN1 protein were identified by RNA immunoprecipitation and high-throughput sequencing experiments.
Results: The efficiency of PfNSUN1 protein down-regulated was about 34%. RNA-seq data revealed that differentially expressed genes were mainly down-regulated. And there were 224, 278, 556 genes that were down-regulated with more than 2-fold changes and p-adj<0.05 at ring, trophozoite and schizont stages, respectively. PfNSUN1 protein was significantly enriched on 154 target genes, including 28S ribosomal RNA and pfap2-g5 transcription factor.
Discussion: PfNSUN1 is a crucial RNA post-transcriptional modification protein in P. falciparum. It plays a pivotal role in regulating gene expression and parasite growth by targeting 28S ribosomal RNA and pfap2-g5 transcription factor.
Introduction
Malaria is an important life-threatening parasitic disease, with 249 million people still infected and about 608,000 deaths worldwide in 2022. Cerebral malaria caused by Plasmodium falciparum is the leading contributor to malaria deaths (Muppidi et al., 2023). The parasite has a complex life cycle, which amplifies one generation every 48 h in human erythrocytes. Such rapid morphological transformation requires a highly regulated gene expression mechanism.
Posttranscriptional modification of RNA has emerged as a crucial mode of gene expression regulation (Zhao et al., 2017; Baumgarten et al., 2019; Barbieri and Kouzarides, 2020; Govindaraju et al., 2020; Sinha et al., 2021; Hao et al., 2022; Wang et al., 2022). Recent studies have revealed that dynamic and reversible RNA modifications are involved in essential biological processes such as cell development, fate determination, pathogen infection, and stress response in eukaryotes (Engel et al., 2018; Frye et al., 2018; Delaunay and Frye, 2019; Wnuk et al., 2020). C5 methylation of cytosines (5-methylcytosine or m5C) is a common modification found on various RNA molecules. In eukaryotes, the m5C modification is primarily catalyzed by members of the NOL1/NOP2/SUN (NSUN) structural domain methyltransferase family (Bohnsack et al., 2019; Tao et al., 2023). Most NSUN proteins are conserved in functions, and mutations in nsun genes have been shown to be associated with a variety of human diseases (Abbasi-Moheb et al., 2012; Khan et al., 2012).
In P. falciparum, four members of the NSUN family (NSUN1-4) have been identified, which contain conserved methylation functional domains similar to those observed in other eukaryotes (Liu et al., 2022). Functional studies on nsun1, nsun3, and nsun4 have not been reported yet. Nevertheless, disruption of NSUN2 has been demonstrated to impede the development of gametocytes in both P. falciparum and P. yoelii, and it is a crucial epigenetic regulator during the transmission of malaria (Liu et al., 2022). Therefore, the NSUN family is expected to be a promising target for malaria prevention and deserves to be intensively investigated.
In this study, a series of experiments was conducted to investigate the function of the PfNSUN1 protein. The results demonstrate that PfNSUN1 is indispensable for parasite growth and development throughout the intraerythrocytic developmental cycle (IDC). The knockdown of PfNSUN1 resulted in a number of alterations in gene expression, including several key regulators. In other eukaryotes, the NSUN1 protein has been demonstrated to be a major methyltransferase of ribosomal RNA (rRNA) (Sharma et al., 2013; Liao et al., 2022). It was found that the PfNSUN1 protein was highly enriched on some 28S rRNA genes. This suggests that the role of the PfNSUN1 protein is well-conserved and linked to ribosome biogenesis. In conclusion, our study demonstrates the crucial function of the PfNSUN1 protein in parasite growth and offers novel insights for advancing malaria research.
Materials and methods
Transgenic line construction
The plasmids used in this experiment were laboratory-modified plasmids as described previously (Shang et al., 2022). The Cas9 gene was carried by pUF1 plasmid, and the guide RNA (5′-GGTTGTTGGGAAGAAGCAAA-3′) and homologous arm sequences were carried by pL6cs plasmid. Plasmid construction is described below. First, the guide RNA was cloned into the pL6cs construct between XhoI and AvrII restriction enzyme sites. Subsequently, the C-terminal homologous arm sequences of pfnsun1 with the ty1-glms tag were cloned into AflII and AscI restriction enzyme sites. The successfully constructed plasmids were transformed into Escherichia coli XL10 for amplification and purification. Primers used to amplify homologous sequences are listed below: pfnsun1-5′HR-F: GGA TAA TGC AAT GGA TAC AC; pfnsun1-5′HR-R:GTT CAA AAT GTA TGG CAA CAT C; pfnsun1-3′HR-F:ACA AAT GGT TCT GGA GGT GAA GCA AAA GGA AAA ATA ATA ATA GAT G; and pfnsun1-3′HR-R: ATC CTT TTT ACC AAG CAC TC.
100 μg purified pL6cs-nsun1-ty1-glmS plasmids and 100 μg pUF1-Cas9-BSD plasmids were electro-transfected into fresh RBCs under the condition of 310V and 950μF, then enriched schizont-stage parasites were added into RBCs. As soon as the parasitemia reached 5% after invasion, selection drugs (2.5 nM WR99210 and 2 μg/ml Blasticidin S deaminase (BSD)) were started to add until live parasites could be found in the Giemsa solution-stained thin blood smears. Then parasites were collected and genomic DNA was extracted for PCR identification and Sanger sequencing. Once the strain was successfully constructed, it was cloned out by limiting dilution cloning as described (Fan et al., 2020).
Parasite culture
Plasmodium falciparum lines 3D7 and Pfnsun1-ty1-glmS were cultivated in vitro according to standard procedures. In brief, parasites were cultured in complete RPMI 1640 medium (Thermo Fisher Scientific, Carlsbad, CA, USA) containing 0.5% Albumax I (Thermo Fisher Scientific) and grown in media with type O+ erythrocytes at a 2% hematocrit. The incubator was set to a gas phase condition of 5% O2, 5% CO2, and 90% N2 at 37°C. To obtain tightly synchronized parasites, parasites were purified with 70%/40% Percoll–sorbitol gradients at the schizont stage. After schizonts invaded fresh RBCs for about 5 h, the resulting rings were synchronized with 5% sorbitol treatment and used for subsequent research.
Growth curve analysis
Pfnsun1-ty1-glmS strain was tightly synchronized to a 5-hour window as previously mentioned. In brief, parasites were collected by the Pecoll-sorbitol solution at schizont stage, subsequently invading new erythrocytes. 5-6 hours later, ring-stage synchronization was performed using the sorbitol solution. The strictly synchronized parasites were diluted to 0.1% parasitemia at ring stage and cultured in 6-well plate which were divided into 2 groups, i.e. with or without 5 mM glucosamine (GlcN). Parasites were cultured for four consecutive replicating cycles, and Giemsa-stained thin blood smears were collected from each cycle to count parasitemia. After three independent replications of the experiment, the data obtained were graphed using GraphPad Prism9 software.
Western blotting
The Pfnsun1-ty1-glmS strain was tightly synchronized, and ring-stage parasites were diluted to 1% parasitemia with 2% hematocrit in the presence or absence of 5 mM GlcN. Ring, trophozoite, and schizont stage samples were collected for Western blot analysis at the next life cycle. Briefly, infected RBCs were lysed by 0.15% saponin to release parasites, which were washed by PBS and resuspended in an equal volume of 2 × protein loading buffer, then heated at 100°C for 5 min. The samples were stored at – 80°C for later experiments. Proteins were separated by 8%–10% SDS-PAGE according to the molecular weight and transferred to the PVDF membrane. The primary antibodies were incubated for 1 h at RT. After three washes with PBST, membranes were incubated with the secondary antibody for 1 h at RT. After five washes, target protein signals were detected using ECL Western Blotting Kit (GE Healthcare, USA). The primary antibodies used in this study were mouse anti-ty1 (Sigma, Germany) at 1:1,000 and rabbit anti-aldolase (Abcam, England) at 1:2,000. The HRP-conjugated secondary antibodies were goat antimouse IgG (Abcam, England) and goat antirabbit IgG, which were diluted to 1:5,000.
RNA extraction, library construction, and RNA-seq data analysis
Pfnsun1-ty1-glmS parasites were tightly synchronized by Percoll and sorbitol to a 5-h window with or without 5 mM GlcN treatment as described above. Samples were collected in TRIzol at the ring (10–15 hpi), trophozoite (25–30 hpi), and schizont (40–45 hpi) stages during the next cycle, respectively. Total RNA was extracted with the kit (Zymo Research, USA) according to a standardized procedure. Library preparation for strand-specific RNA-seq was carried out by poly(A) selection with the KAPA mRNA Capture Beads (KAPA) and fragmentation to about 300–400 nucleotides (nt) in length according to the KAPA Stranded mRNA-Seq Kit (KK8421). Libraries were sequenced on an Illumina NovaSeq 6000 system to generate 150 bp pair-end reads.
RNA-seq reads were trimmed by trim-galore, then aligned to the PlasmoDB-45_Pfalciparum3D7_Genome using Hisat2. Read counts were obtained using FeatureCounts. Subsequently, DESeq2 quantified differentially expressed genes (DEGs) with the criteria of ≥2-fold alteration and p-adj < 0.05. The Gene Ontology (GO) enrichment analysis was performed on PlasmoDB (https://plasmodb.org/plasmo/).
RIP-seq and data analysis
RIP assays were performed as previously described (Fan et al., 2020). Briefly, about 5 × 109 synchronized ring-stage parasites were collected and lysed by saponin. The resulting parasite pellet was lysed under nondenaturing conditions (50 mM Tris-Cl at pH 7.4, 150 mM NaCl, 1 mM EDTA, 1 mM EGTA, 1% Triton X-100, 1% NP-40, 1× proteinase inhibitors, 0.2 U/μl RNase inhibitor) for 2 h at 4°C with rotation. The supernatant was collected and incubated with 10 µg mouse anti-ty1 antibodies for 3 h, then incubated overnight with protein G magnetic beads at 4°C. The complex was washed twice with IPP500 (500 mM NaCl, 10 mM Tris-Cl at pH 8.0, 0.05% NP-40, 1 × proteinase inhibitor, 0.2 U/µl RNase inhibitor) and once with PBS, then RNA was eluted by TRIzol reagent and extracted by the phenol-chloroform method. The RNA was directly used to prepare strand-specific RNA-seq libraries without poly(A) enrichment. Libraries were sequenced on an Illumina NovaSeq 6000 system using 150 bp pair-end reads.
For RIP-seq data analysis, reads were removed with trim-galore and aligned to the genome by Hisat2. Samtools was used to sort reads. Peaks (q-value cut-off < 0.05) were identified using the macs2 call peak command, by comparing the control and the group with the ty1 antibody, using default settings. Enrichment heatmaps and profile plots were generated using the deepTools computeMatrix and plotHeatmap tools. Peak annotation was carried out using ChIPseeker in RStudio.
Results
Generation of pfnsun1 transgenic parasite line
Pfnsun1 (PF3D7_0704200) ORF contains 4,263 bases, encoding a protein of approximately 141.2 kDa in molecular weight and containing one NOP2 structural domain. In order to compare the homology within the NSUN family, we analyzed the amino acid sequences of PfNSUN1 to PfNSUN4, along with other common Plasmodium NSUN1 proteins. Our findings showed that sequences of PfNSUN1 had low similarity to PfNSUN2-4 but was conserved among different Plasmodium species (Figure 1A). To determine the function of pfnsun1, we attempted to disrupt the pfnsun1 gene. However, following three unsuccessful transfection attempts, it was concluded that the gene might be indispensable. Consequently, we opted to construct a knockdown strain. About 1 kb bases before and after the stop codon of the pfnsun1 gene were selected as homologous arm sequences, and three tandem ty1 tags and a glmS sequence were cloned into the plasmid. The constructed plasmids were electro-transferred into wild-type parasites, which were then cultured for about 3 weeks with WR and BSD drug selection until live parasites were observed by microscopy (Figure 1B). To confirm successful transfection, we designed a forward primer P1, upstream of the 5′ homologous arm, a reverse primer P2 within the glmS sequence, and another reverse primer P3 within the 3′ homologous arm sequence, respectively. The sequence lengths of wild-type and transgenic strains were verified using the P1+P2 and P1+P3 primers. Agarose gel electrophoresis results confirmed the successful generation of the pfnsun1-ty1-glmS parasite strain (Figure 1C).
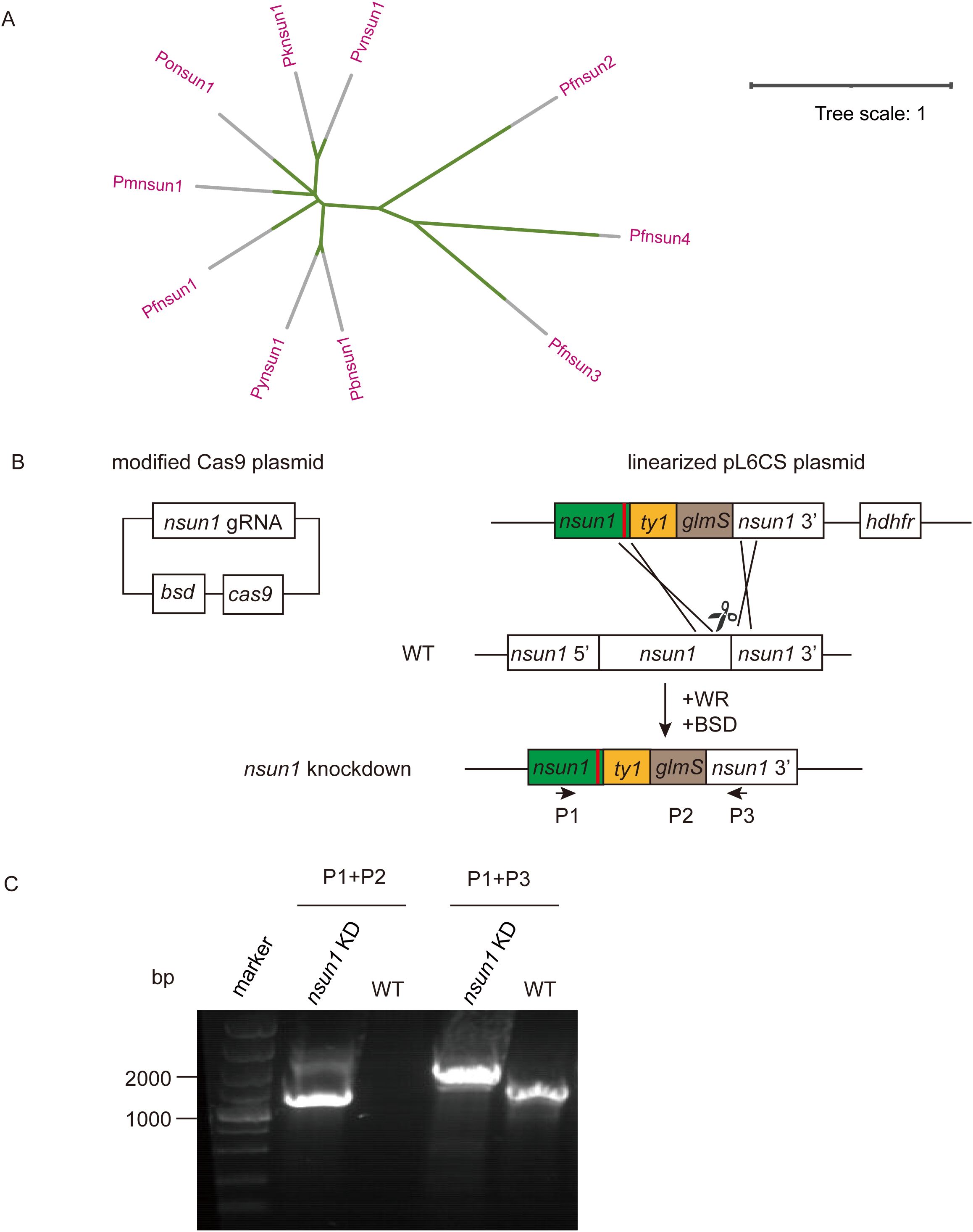
Figure 1. Generation and characterization of pfnsun1-ty1-glmS knockdown strain. (A) Phylogenetic trees of NSUN protein family orthologs in Plasmodium spp. (B) Schematic representation of transgenic line pfnsun1-ty1-glmS construction. Cotransfection of plasmids pUF1-BSD-cas9 and pL6CS-hDHFR-pfnsun1-ty1-glmS leads to gene integration. (C) PCR validation of the pfnsun1-ty1-glmS line.
Pfnsun1 is required for parasite development in the intraerythrocytic developmental cycle
To verify the knockdown effect of the pfnsun1-ty1-glmS transgenic strain, we performed a Western blot assay. The addition of 5 mM GlcN had no significant impact on the growth of wild-type P. falciparum parasites as confirmed by previous studies (Liu et al., 2020; Shang et al., 2021b). Thus, we collected the ring, trophozoite and schizont stage samples in the second growth cycle with or without GlcN to extract protein after synchronizing the parasites. The results showed insignificant changes in protein levels at ring and trophozoite stages but a significant decrease at the schizont stage (Figure 2A). The gray value of protein electrophoresis with GlcN treatment at the schizont stage decreased by approximately 34% compared to the untreated group (Figure 2B). To investigate the importance of PfNSUN1 protein in the intraerythrocytic developmental cycle, we performed growth curve experiment. We minimized the growth window of the transgenic parasite line to 5 h after rigorous synchronization. When parasites reached the early trophozoite stage, we diluted the parasitemia to 0.1% and continued cultivating for four growth cycles with or without GlcN and counted parasitemia. The results showed that at the fourth cycle, parasitemia of the group without GlcN treatment reached an average of 19.7%, while parasitemia of the group with drug treatment reduced to 12.9%, indicating an approximate 35% decrease in growth efficiency (Figure 2C). These results suggest that the pfnsun1 gene plays an important role in the intraerythrocytic developmental cycle.
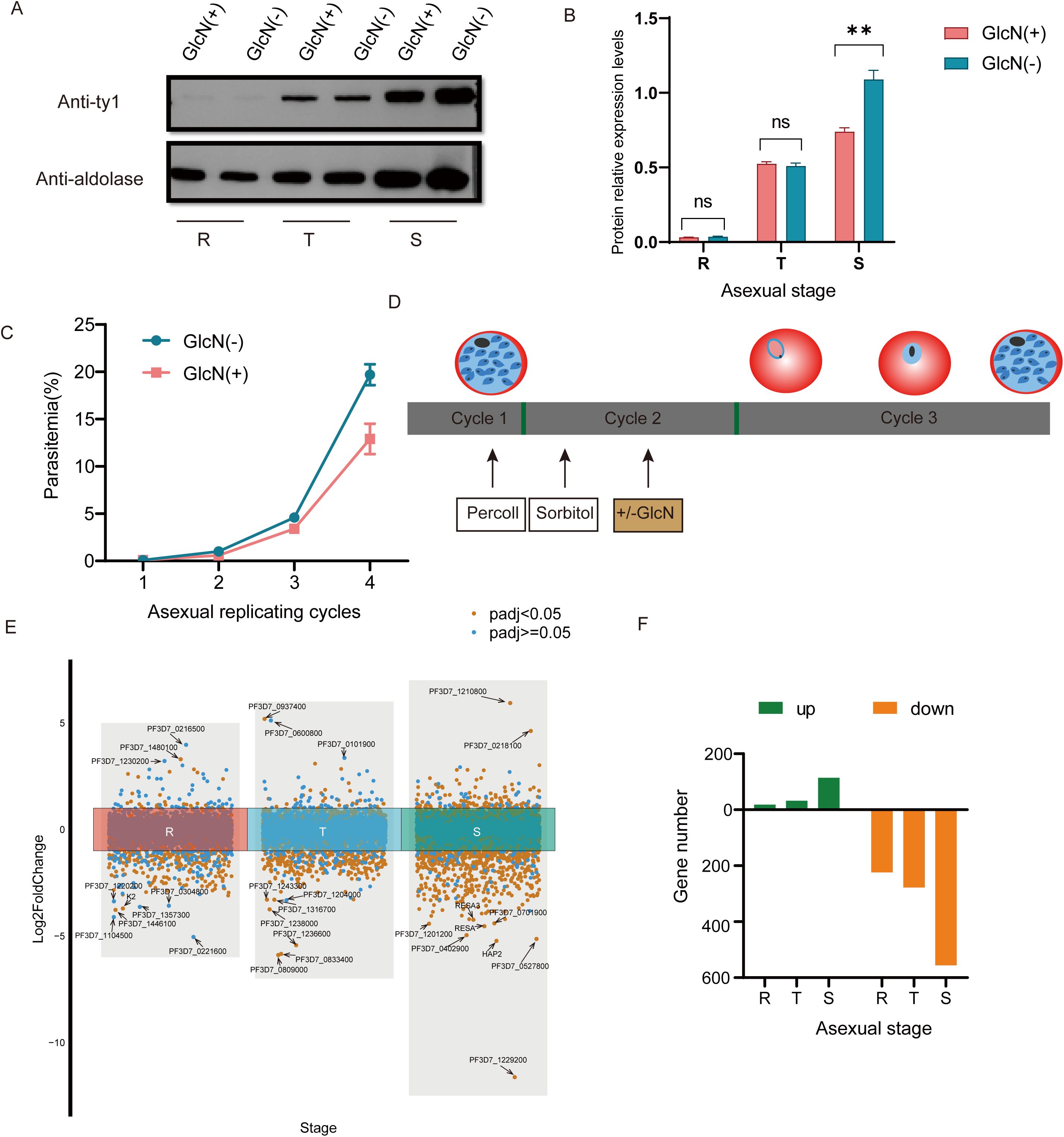
Figure 2. PfNSUN1 protein is required during intraerythrocytic development. (A) Western blot of total protein extracts from ring (R), trophozoite (T), and schizont (S) stages from the pfnsun1-ty1-glmS strain with or without GlcN. (B) Gray value analysis of Western blot experiment. **P< 0.01, ‘ns’: not significant (unpaired two-tailed Student’s t-test). (C) Growth curve assay of the pfnsun1-ty1-glmS strain with or without GlcN in the culture (n = 3, bars are SD). (D) Schematic of transcriptome samples collected. The strain experienced Percoll and sorbitol synchronization and was collected at the third cycle with or without GlcN. (E) Scatterplot of all genes in the RNA-seq data. Ten genes with the strongest changes are labeled. (F) Histogram displaying the number of up or downregulated DEGs.
Pfnsun1 knockdown altered the global transcriptome
To explore the function of PfNSUN1 protein as an epigenetic regulator, we performed strict synchronization and divided the samples into GlcN-added and non-GlcN-added groups. Transcriptome samples were collected in the subsequent growth cycle (Figure 2D). RNA-seq data revealed significant transcriptomic changes following pfnsun1 knockdown. Specifically, there were only 18, 32, and 114 upregulated genes, but 224, 278, and 556 downregulated DEGs at the ring, trophozoite, and schizont stages, respectively (Supplementary Table S1). We highlighted 10 genes with the greatest changes at each stage and found that most of the genes were antigenic variant genes or genes with unknown functions. Notably, K2 was significantly downregulated at the ring stage, and hap2, resa, and resa3 were significantly downregulated at the schizont stage. In light of these findings, PfNSUN1 may be regarded as a positive regulator that facilitates gene expression (Figures 2E, F).
To clarify the genes affected by pfnsun1 knockdown, we took the intersection of down-regulated differentially expressed genes (DEGs) at ring, trophozoite and schizont stages. This analysis revealed 12 genes with consistently decreased expression across all stages (Figure 3A). These 12 genes included sir2a, dip13, alv7, and imc20. Sir2a is an important regulator in P. falciparum and is critical for maintaining a stable heterochromatin environment and exclusive expression of antigenic variant genes (Petter et al., 2011; Mancio-Silva et al., 2013) (Figure 3B). Given that the schizont stage exhibited the highest number of downregulated DEGs, we selected these genes for GO enrichment analysis. The analysis indicated that these genes impact multiple pathways, including host entry, movement within the host environment, biological process involved in symbiotic interaction, obsolete pathogenesis, actin cytoskeleton organization, protein phosphorylation, peptidyl−serine modification, etc. (Figure 3C). To ensure comprehensive pathway identification by GO analysis, we also performed KEGG enrichment analysis on these genes and found that these genes were involved in the regulation of amino sugar and nucleotide sugar metabolism, glycerophospholipid metabolism, glycerolipid metabolism, fatty acid biosynthesis, and other pathways (Figure 3D). To ascertain the impact of PfNSUN1 on parasite growth, we investigated the downregulation of eba, msp, rap, rh, and ron invasion genes. Our findings revealed that the transcripts of eba and rh family members exhibited a downregulation of over 4-fold (Figure 3E). It was plausible that PfNSUN1 influenced the expression of these invasion-related genes. In conclusion, these findings indicate that the reduction in parasitemia may be associated with conserved biological processes, such as invasion and metabolism.
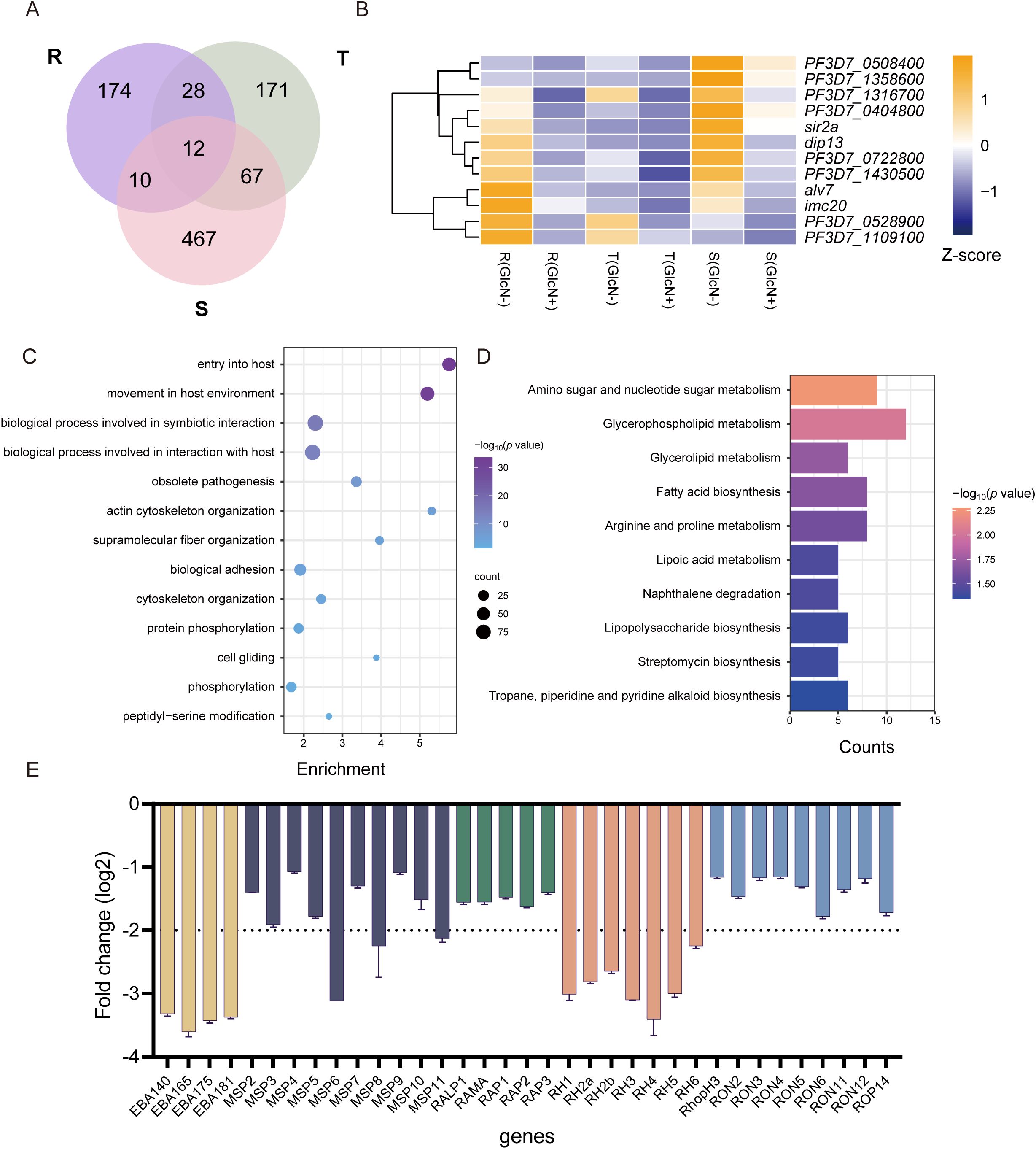
Figure 3. Functional analysis of downregulated DEGs in gene expression regulation. (A) Venn diagram showing genes downregulated at R, T, and S stages. (B) Heatmap showing the 12 downregulated gene expressing levels of the pfnsun1-ty1-glmS strain with or without GlcN across the R, T, and S stages. (C) Enriched Gene Ontology (biological processes) terms for the DEGs in the pfnsun1 knockdown line at the schizont stage. (D) Enriched KEGG terms for the DEGs in the pfnsun1 knockdown line at the schizont stage. (E) The log2 fold change (GlcN(+)/GlcN(-)) of down-regulated invasion genes at schizont stage.
PfNSUN1 binds on 28S ribosomal RNA
Pfnsun1 is an RNA methylation factor that influences gene expression by modifying RNA. Therefore, we collected a ring stage sample of the pfnsun1-ty1-glmS strain to identify its direct-binding RNAs by RIP-seq experiments. The results showed that RNAs bound by the PfNSUN1 protein were distributed across all 14 chromosomes, as illustrated in the peak diagram (Figures 4A, B). There were 154 target genes confirmed after aligning to the genome (Supplementary Table S2). In combination with RNA-seq data, our findings indicated that these genes were predominantly down-regulated, thereby substantiating the hypothesis that PfNSUN1 exerted a positive regulatory effect on gene expression (Figure 4C). GO enrichment analysis showed that these genes were involved in pathways including cytoadherence to the microvasculature, biological adhesion, response to xenobiotic stimulus, modulation by symbiont of host process, evasion of host immune response, response to host immune response, response to host defenses, response to host, response to external biotic stimulus, and response to defenses of other organisms (Figure 4D). These results showed that PfNSUN1 is related in the response between parasite and host.
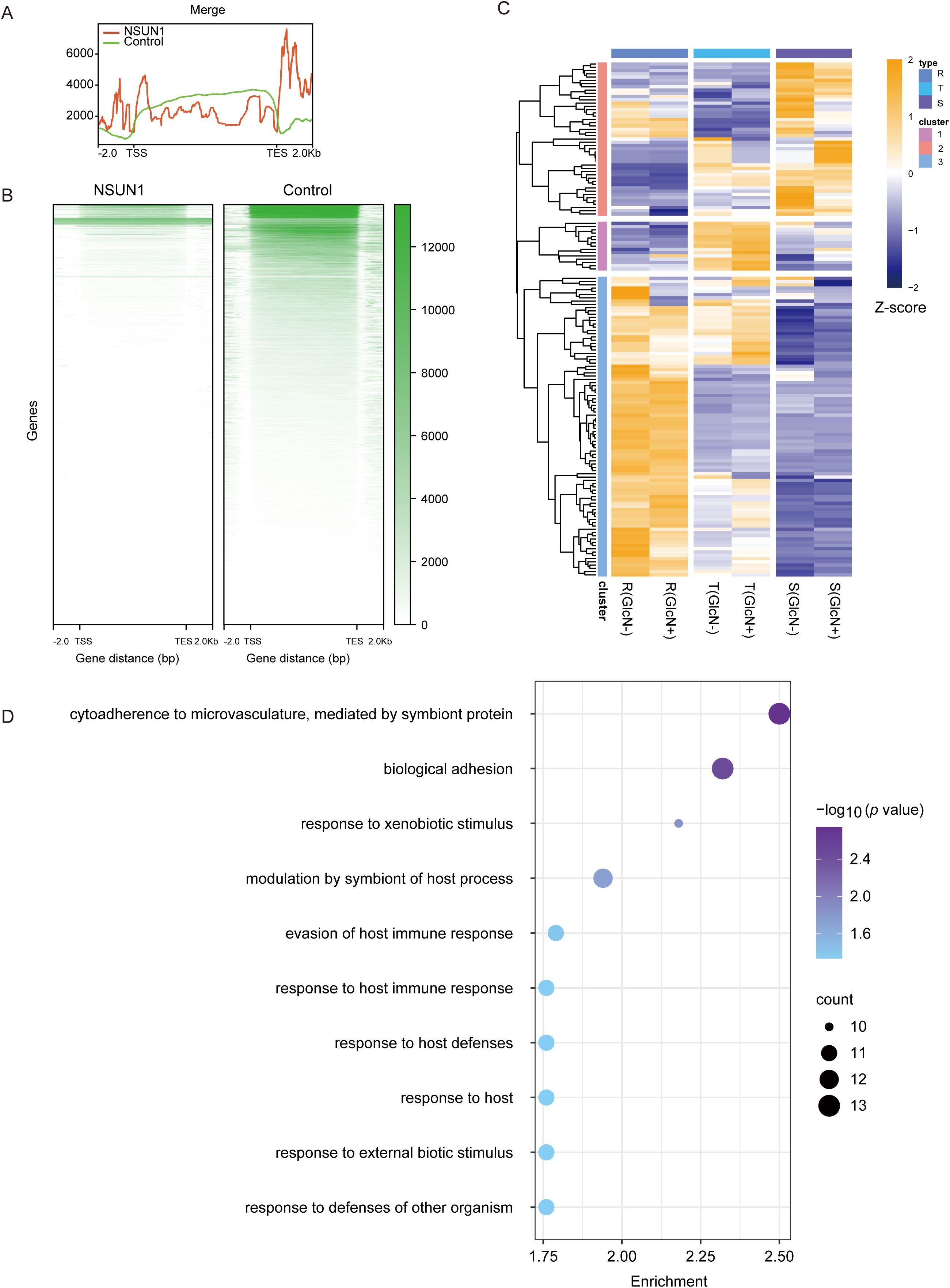
Figure 4. Target gene analysis of PfNSUN1 protein-enriched. (A) The average profile of RIP/control enrichment at gene coding sequence. (B) Heatmap showing RIP/control fold enrichment at gene coding sequence. TSS, translation start site; TES, translation end site. (C) Expressing heatmap of target genes obtained from RIP-seq at ring stage. (D) Enriched Gene Ontology (biological processes) terms for the target genes obtained from RIP-seq.
To validate target genes on which PfNSUN1 protein has a significant effect, we took intersections of target genes obtained from RIP-seq data with down-regulated DEGs at different stages. The down-regulated DEGs at ring, trophozoite, and schizont stages had 14, 12, and 11 same genes with PfNSUN1 protein target genes, respectively (Figures 5A–C). At ring stage, pfnsun1 mainly regulates cct, eg5, irp, suv3, m712, emp3, ap2-g5, p52, etc. The functions of suv3 and m712 are unknown. PfCCT has a key regulatory function in the second and rate-limiting step of the de novo phosphatidylcholine biosynthesis, which is essential for parasite survival (Izrael et al., 2020). PfEG5 is a molecular motor that cross-links microtubules, similar to other organisms (Cook et al., 2021). PfIRP regulates parasite environment homeostasis by binding iron response elements (Hodges et al., 2005). Pfemp3 encodes erythrocyte membrane protein 3, which appears on the cytoplasmic surface of the host cell membrane in the later stages and is associated with membrane skeleton and cytoadherence (Waterkeyn et al., 2000). An important factor affected by pfnsun1 is pfap2-g5, which belongs to the largest ApiAP2 transcription factor family in P. falciparum. A previous study revealed that pfap2-g5 is a transcriptional repressor that co-regulates gametocyte development with pfap2-g (Kafsack et al., 2014; Poran et al., 2017; Shang et al., 2021a) (Figures 5D, E). Additionally, we found that other ApiAP2 transcription factors showed a decrease in transcript level after pfnsun1 knockdown, such as ap2-o, PF3D7_0420300, PF3D7_1107800, etc. (Supplementary Figure S1). PfP52 is a member of the 6-cysteine family, which can ensure that sporozoites enter hepatocytes (Arredondo and Kappe, 2017). Target genes such as xl1 were downregulated at the schizont stage (Figure 5D). PfXL1 is possibly associated with adhesion between parasites and erythrocytes (Spillman et al., 2016). Noteworthy, PfNSUN1 knockdown caused some ncRNA down-regulated at trophozoite stage, most of them were 28S rRNA, such as PF3D7_0112700, PF3D7_0532000, and PF3D7_0726000 (Figures 5D, E). The results indicated that PfNSUN1 is involved in ribosome biogenesis, which is consistent with human NSUN1 protein (Liao et al., 2022).
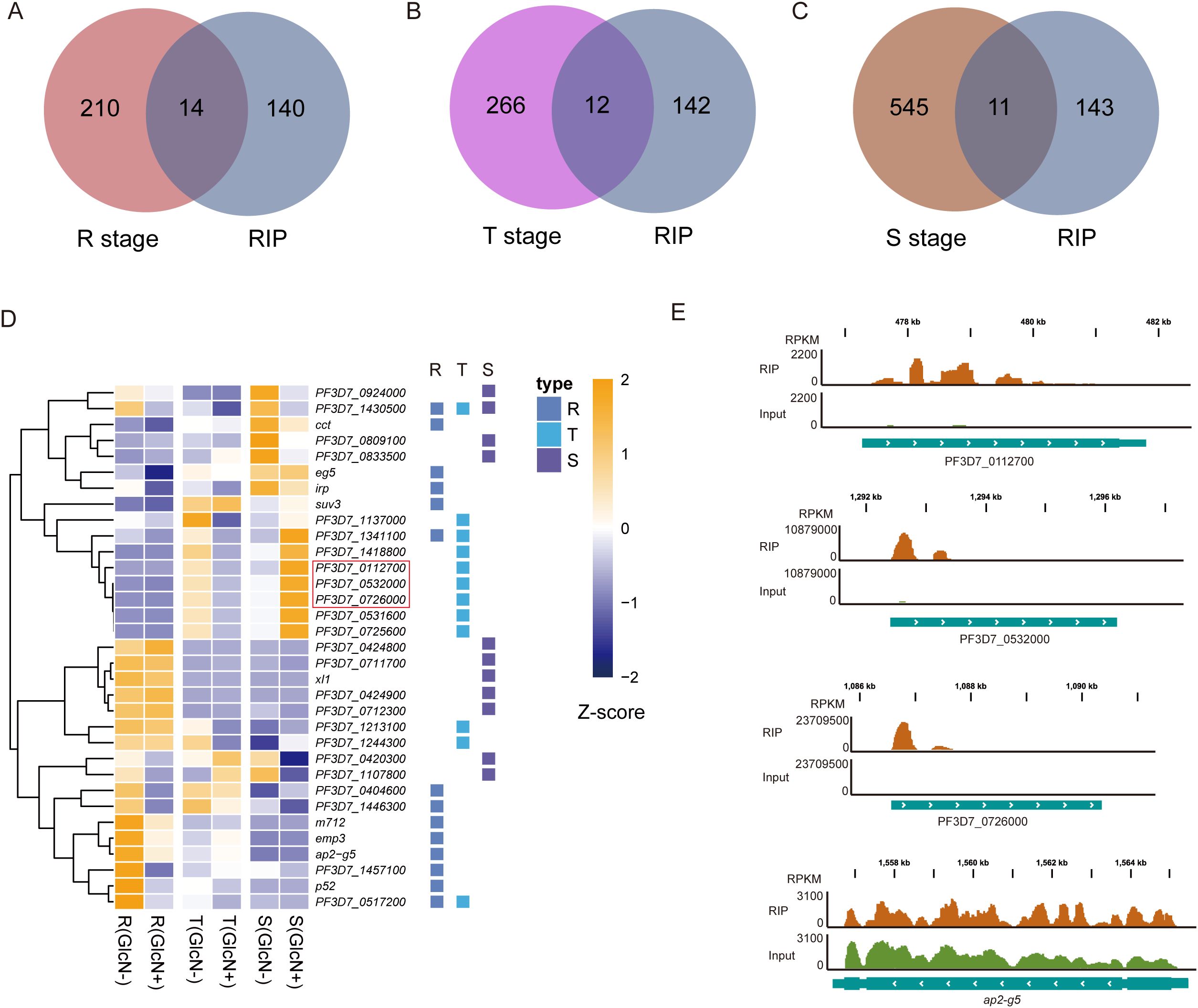
Figure 5. The PfNSUN1 protein modifies the 28S rRNA and pfap2-g5 transcription factor. (A–C) Venn diagrams showing the intersections between target genes from RIP-seq data and downregulated genes at the R, T, and S stages. (D) Heatmap showing downregulated target gene expressing levels of pfnsun1-ty1-glmS strain with or without GlcN at ring, trophozoite, and schizont stages. 28S rRNAs are marked with a red box. (E) Track view showing the enrichment signals of the PfNSUN1 protein on 28S rRNA and pfap2-g5 transcription factor.
Discussion
The NSUN family is an important m5C-modified factor in eukaryotes and plays an important role in gene expression regulation. Recent studies have increasingly focused on this family, particularly concerning human-related tumorigenesis and progression, such as hepatocellular carcinoma and bladder cancer (Awah et al., 2021; Wang et al., 2023; Zhang et al., 2023; Gu et al., 2024). A recent study demonstrated that NOP2/NSUN1 catalyzes the deposition of m5C at position 4447 on 28S rRNA and regulates ribosome biogenesis through noncatalytic complex formation with box C/D snoRNPs (Liao et al., 2022). In yeast, the NSUN protein homolog, NOP2, has the ability of m5C modification on 25S rRNA (Sharma et al., 2013). In Plasmodium spp., four genes (NSUN1–4) are predicted to contain the NOP2 functional domain. Currently, the RNA modification map of NSUN2 is the only one that has been characterized. Disrupting the gene encoding NSUN2 in P. yoelii results in a complete loss of gametocyte generation, while P. falciparum shows very low gametocyte productivity (Liu et al., 2022). Thus, the NSUN family is indispensable for parasite growth and transmission, contributing to gene regulatory diversity.
In this study, we focus on exploring the role of pfnsun1 in gene expression regulation. Despite several unsuccessful knockout attempts, we constructed an inducible knockdown system. The knockdown efficacy of this strain reached only about 34%, which was not a perfect knockdown strain, but we already could observe a genome-wide effect brought by PfNSUN1. The majority of DEGs exhibited down-regulation, while a subset demonstrated up-regulation. Since pfnsun1 knockdown altered the expression level of regulatory factors such as sir2a, pfap2-g5, pfap2-o, etc., it is unclear whether pfnsun1 regulates these genes directly or indirectly. In addition, we obtained 154 target genes directly bound by the PfNSUN1 protein. In other eukaryotes, the NSUN1 protein was identified to modify 28S rRNA and 25S rRNA (Sharma et al., 2013; Liao et al., 2022). In this study, PfNSUN1 protein was significantly enriched at several genes encoding 28S rRNA, suggesting it is conserved in regulating ribosome biogenesis. Furthermore, we found that PfNSUN1 protein was highly enriched at the activated var gene, indicating a potential association with parasite virulence (Scherf et al., 2008; Maier et al., 2009). Transcriptional data show a high transcript abundance of pfnsun1 at the ring stage, trophozoite stage, gametocyte V and ookinete stage (López-Barragán et al., 2011) (Supplementary Figure S2). Thus, PfNSUN1 protein may be involved in other critical life processes, such as gametocyte production, by regulating m5C modification of pfap2-g5, though this speculation requires further verification.
In summary, our data reveal that PfNSUN1 protein is required during IDC in P. falciparum, playing a significant role in regulating ribosome biogenesis and gene expression. These findings provide new insights into malaria control and potential drug discovery.
Data availability statement
The datasets presented in this study can be found in online repositories. The high-throughput sequencing data of this study have been deposited in Gene Expression Omnibus (GEO) database under accession number GSE254642 and GSE254643/Supplementary Material.
Ethics statement
The manuscript presents research on animals that do not require ethical approval for their study.
Author contributions
RT: Formal analysis, Investigation, Methodology, Validation, Visualization, Writing – original draft, Writing – review & editing. YF: Conceptualization, Funding acquisition, Investigation, Methodology, Writing – original draft, Writing – review & editing. BL: Writing – review & editing, Investigation, Methodology, Validation. QJ: Methodology, Resources, Writing – review & editing. XC: Methodology, Investigation, Validation, Writing – review & editing. ZZ: Conceptualization, Formal analysis, Writing – review & editing. LS: Writing – review & editing, Funding acquisition. XS: Conceptualization, Data curation, Funding acquisition, Software, Visualization, Writing – original draft, Writing – review & editing.
Funding
The author(s) declare financial support was received for the research, authorship, and/or publication of this article. This study was supported by the National Natural Science Foundation of China (#32200450 and #82202550), Natural Science Foundation of Hunan Province, China (Grant no. 2023JJ40798) and Shanghai Municipal health Commission of Research Project (Grant no. 202240012).
Acknowledgments
We thank the staff at Zhang Qingfeng’s group at Tongji University who helped with experiments in this research.
Conflict of interest
Author QJ is employed by Hunan Xingchen Biotechnology Company.
The remaining authors declare that the research was conducted in the absence of any commercial or financial relationships that could be construed as a potential conflict of interest.
Publisher’s note
All claims expressed in this article are solely those of the authors and do not necessarily represent those of their affiliated organizations, or those of the publisher, the editors and the reviewers. Any product that may be evaluated in this article, or claim that may be made by its manufacturer, is not guaranteed or endorsed by the publisher.
Supplementary material
The Supplementary Material for this article can be found online at: https://www.frontiersin.org/articles/10.3389/fcimb.2024.1474229/full#supplementary-material
Supplementary Figure 1 | Heatmap of transcription levels of ApiAP2 transcription factor family with or without GlcN.
Supplementary Figure 2 | Dynamic changes of pfnsun1 transcript abundance (TPM) during the whole life history (López-Barragán et al., 2011).
References
Abbasi-Moheb, L., Mertel, S., Gonsior, M., Nouri-Vahid, L., Kahrizi, K., Cirak, S., et al. (2012). Mutations in NSUN2 cause autosomal-recessive intellectual disability. Am. J. Hum. Genet. 90, 847–855. doi: 10.1016/j.ajhg.2012.03.021
Arredondo, S. A., Kappe, S. H. I. (2017). The s48/45 six-cysteine proteins: mediators of interaction throughout the Plasmodium life cycle. Int. J. Parasitol. 47, 409–423. doi: 10.1016/j.ijpara.2016.10.002
Awah, C. U., Winter, J., Mazdoom, C. M., Ogunwobi, O. O. (2021). NSUN6, an RNA methyltransferase of 5-mC controls glioblastoma response to temozolomide (TMZ) via NELFB and RPS6KB2 interaction. Cancer Biol. Ther. 22, 587–597. doi: 10.1080/15384047.2021.1990631
Barbieri, I., Kouzarides, T. (2020). Role of RNA modifications in cancer. Nat. Rev. Cancer 20, 303–322. doi: 10.1038/s41568-020-0253-2
Baumgarten, S., Bryant, J. M., Sinha, A., Reyser, T., Preiser, P. R., Dedon, P. C., et al. (2019). Transcriptome-wide dynamics of extensive m6A mRNA methylation during Plasmodium falciparum blood-stage development. Nat. Microbiol. 4, 2246–2259. doi: 10.1038/s41564-019-0521-7
Bohnsack, K. E., Höbartner, C., Bohnsack, M. T. (2019). Eukaryotic 5-methylcytosine (m5C) RNA methyltransferases: mechanisms, cellular functions, and links to disease. Genes (Basel) 10, 102. doi: 10.3390/genes10020102
Cook, A. D., Roberts, A. J., Atherton, J., Tewari, R., Topf, M., Moores, C. A. (2021). Cryo-EM structure of a microtubule-bound parasite kinesin motor and implications for its mechanism and inhibition. J. Biol. Chem. 297, 101063. doi: 10.1016/j.jbc.2021.101063
Delaunay, S., Frye, M. (2019). RNA modifications regulating cell fate in cancer. Nat. Cell Biol. 21, 552–559. doi: 10.1038/s41556-019-0319-0
Engel, M., Eggert, C., Kaplick, P. M., Eder, M., Röh, S., Tietze, L., et al. (2018). The role of m(6)A/m-RNA methylation in stress response regulation. Neuron 99, 389–403.e389. doi: 10.1016/j.neuron.2018.07.009
Fan, Y., Shen, S., Wei, G., Tang, J., Zhao, Y., Wang, F., et al. (2020). Rrp6 Regulates Heterochromatic Gene Silencing via ncRNA RUF6 Decay in Malaria Parasites. mBio 11, e01110–e01120. doi: 10.1128/mBio.01110-20
Frye, M., Harada, B. T., Behm, M., He, C. (2018). RNA modifications modulate gene expression during development. Science 361, 1346–1349. doi: 10.1126/science.aau1646
Govindaraju, G., Kadumuri, R. V., Sethumadhavan, D. V., Jabeena, C. A., Chavali, S., Rajavelu, A. (2020). N(6)-Adenosine methylation on mRNA is recognized by YTH2 domain protein of human malaria parasite Plasmodium falciparum. Epigenet. Chromatin 13, 33. doi: 10.1186/s13072-020-00355-7
Gu, X., Li, P., Gao, X., Ru, Y., Xue, C., Zhang, S., et al. (2024). RNA 5-methylcytosine writer NSUN5 promotes hepatocellular carcinoma cell proliferation via a ZBED3-dependent mechanism. Oncogene 43, 624–635. doi: 10.1038/s41388-023-02931-z
Hao, W., Dian, M., Zhou, Y., Zhong, Q., Pang, W., Li, Z., et al. (2022). Autophagy induction promoted by m(6)A reader YTHDF3 through translation upregulation of FOXO3 mRNA. Nat. Commun. 13, 5845. doi: 10.1038/s41467-022-32963-0
Hodges, M., Yikilmaz, E., Patterson, G., Kasvosve, I., Rouault, T. A., Gordeuk, V. R., et al. (2005). An iron regulatory-like protein expressed in Plasmodium falciparum displays aconitase activity. Mol. Biochem. Parasitol. 143, 29–38. doi: 10.1016/j.molbiopara.2005.05.004
Izrael, R., Marton, L., Nagy, G. N., Pálinkás, H. L., Kucsma, N., Vértessy, B. G. (2020). Identification of a nuclear localization signal in the Plasmodium falciparum CTP: phosphocholine cytidylyltransferase enzyme. Sci. Rep. 10, 19739. doi: 10.1038/s41598-020-76829-1
Kafsack, B. F. C., Rovira-Graells, N., Clark, T. G., Bancells, C., Crowley, V. M., Campino, S. G., et al. (2014). A transcriptional switch underlies commitment to sexual development in malaria parasites. Nature 507, 248–252. doi: 10.1038/nature12920
Khan, M. A., Rafiq, M. A., Noor, A., Hussain, S., Flores, J. V., Rupp, V., et al. (2012). Mutation in NSUN2, which encodes an RNA methyltransferase, causes autosomal-recessive intellectual disability. Am. J. Hum. Genet. 90, 856–863. doi: 10.1016/j.ajhg.2012.03.023
Liao, H., Gaur, A., McConie, H., Shekar, A., Wang, K., Chang, J. T., et al. (2022). Human NOP2/NSUN1 regulates ribosome biogenesis through non-catalytic complex formation with box C/D snoRNPs. Nucleic Acids Res. 50, 10695–10716. doi: 10.1093/nar/gkac817
Liu, H., Cui, X.-Y., Xu, D.-D., Wang, F., Meng, L.-W., Zhao, Y.-M., et al. (2020). Actin-related protein Arp4 regulates euchromatic gene expression and development through H2A.Z deposition in blood-stage Plasmodium falciparum. Parasites Vectors 13, 314. doi: 10.1186/s13071-020-04139-6
Liu, M., Guo, G., Qian, P., Mu, J., Lu, B., He, X., et al. (2022). 5-methylcytosine modification by Plasmodium NSUN2 stabilizes mRNA and mediates the development of gametocytes. Proc. Natl. Acad. Sci. U.S.A. 119, e2110713119. doi: 10.1073/pnas.2110713119
López-Barragán, M. J., Lemieux, J., Quiñones, M., Williamson, K. C., Molina-Cruz, A., Cui, K., et al. (2011). Directional gene expression and antisense transcripts in sexual and asexual stages of Plasmodium falciparum. BMC Genomics 12, 587. doi: 10.1186/1471-2164-12-587
Maier, A. G., Cooke, B. M., Cowman, A. F., Tilley, L. (2009). Malaria parasite proteins that remodel the host erythrocyte. Nat. Rev. Microbiol. 7, 341–354. doi: 10.1038/nrmicro2110
Mancio-Silva, L., Lopez-Rubio, J. J., Claes, A., Scherf, A. (2013). Sir2a regulates rDNA transcription and multiplication rate in the human malaria parasite Plasmodium falciparum. Nat. Commun. 4, 1530. doi: 10.1038/ncomms2539
Muppidi, P., Wright, E., Wassmer, S. C., Gupta, H. (2023). Diagnosis of cerebral malaria: Tools to reduce Plasmodium falciparum associated mortality. Front. Cell Infect. Microbiol. 13. doi: 10.3389/fcimb.2023.1090013
Petter, M., Lee, C. C., Byrne, T. J., Boysen, K. E., Volz, J., Ralph, S. A., et al. (2011). Expression of P. falciparum var genes involves exchange of the histone variant H2A.Z at the promoter. PloS Pathog. 7, e1001292. doi: 10.1371/journal.ppat.1001292
Poran, A., Notzel, C., Aly, O., Mencia-Trinchant, N., Harris, C. T., Guzman, M. L., et al. (2017). Single-cell RNA sequencing reveals a signature of sexual commitment in malaria parasites. Nature 551, 95–99. doi: 10.1038/nature24280
Scherf, A., Lopez-Rubio, J. J., Riviere, L. (2008). Antigenic variation in Plasmodium falciparum. Annu. Rev. Microbiol. 62, 445–470. doi: 10.1146/annurev.micro.61.080706.093134
Shang, X., Shen, S., Tang, J., He, X., Zhao, Y., Wang, C., et al. (2021a). A cascade of transcriptional repression determines sexual commitment and development in Plasmodium falciparum. Nucleic Acids Res. 49, 9264–9279. doi: 10.1093/nar/gkab683
Shang, X., Wang, C., Fan, Y., Guo, G., Wang, F., Zhao, Y., et al. (2022). Genome-wide landscape of ApiAP2 transcription factors reveals a heterochromatin-associated regulatory network during Plasmodium falciparum blood-stage development. Nucleic Acids Res. 50, 3413–3431. doi: 10.1093/nar/gkac176
Shang, X., Wang, C., Shen, L., Sheng, F., He, X., Wang, F., et al. (2021b). PfAP2-EXP2, an essential transcription factor for the intraerythrocytic development of Plasmodium falciparum. Front. Cell Dev. Biol. 9. doi: 10.3389/fcell.2021.782293
Sharma, S., Yang, J., Watzinger, P., Kötter, P., Entian, K. D. (2013). Yeast Nop2 and Rcm1 methylate C2870 and C2278 of the 25S rRNA, respectively. Nucleic Acids Res. 41, 9062–9076. doi: 10.1093/nar/gkt679
Sinha, A., Baumgarten, S., Distiller, A., McHugh, E., Chen, P., Singh, M., et al. (2021). Functional characterization of the m(6)A-dependent translational modulator PfYTH.2 in the human malaria parasite. mBio 12, e00661–e00621. doi: 10.1128/mBio.00661-21
Spillman, N. J., Dalmia, V. K., Goldberg, D. E. (2016). Exported Epoxide Hydrolases Modulate Erythrocyte Vasoactive Lipids during Plasmodium falciparum Infection. mBio 7, e01538–16. doi: 10.1128/mBio.01538-16
Tao, Y., Felber, J. G., Zou, Z., Njomen, E., Remsberg, J. R., Ogasawara, D., et al. (2023). Chemical proteomic discovery of isotype-selective covalent inhibitors of the RNA methyltransferase NSUN2. Angew Chem. Int. Ed. Engl. 62, e202311924. doi: 10.1002/anie.202311924
Wang, L., Wu, J., Liu, R., Chen, W., Pang, Z., Zhou, F., et al. (2022). Epitranscriptome profiling of spleen mRNA m(6)A methylation reveals pathways of host responses to malaria parasite infection. Front. Immunol. 13. doi: 10.3389/fimmu.2022.998756
Wang, N., Chen, R. X., Deng, M. H., Wei, W. S., Zhou, Z. H., Ning, K., et al. (2023). m(5)C-dependent cross-regulation between nuclear reader ALYREF and writer NSUN2 promotes urothelial bladder cancer Malignancy through facilitating RABL6/TK1 mRNAs splicing and stabilization. Cell Death Dis. 14, 139. doi: 10.1038/s41419-023-05661-y
Waterkeyn, J. G., Wickham, M. E., Davern, K. M., Cooke, B. M., Coppel, R. L., Reeder, J. C., et al. (2000). Targeted mutagenesis of Plasmodium falciparum erythrocyte membrane protein 3 (PfEMP3) disrupts cytoadherence of malaria-infected red blood cells. EMBO J. 19, 2813–2823. doi: 10.1093/emboj/19.12.2813
Wnuk, M., Slipek, P., Dziedzic, M., Lewinska, A. (2020). The roles of host 5-methylcytosine RNA methyltransferases during viral infections. Int. J. Mol. Sci. 21, 8176. doi: 10.3390/ijms21218176
Zhang, H., Zhai, X., Liu, Y., Xia, Z., Xia, T., Du, G., et al. (2023). NOP2-mediated m5C Modification of c-Myc in an EIF3A-Dependent Manner to Reprogram Glucose Metabolism and Promote Hepatocellular Carcinoma Progression. Res. (Wash D C) 6, 184. doi: 10.34133/research.0184
Keywords: Plasmodium falciparum, 5-methylcytosine, RNA modification, regulation, 28S ribosomal RNA
Citation: Tang R, Fan Y, Lu B, Jiang Q, Cheng X, Zhang Z, Shen L and Shang X (2024) The RNA m5C methyltransferase NSUN1 modulates human malaria gene expression during intraerythrocytic development. Front. Cell. Infect. Microbiol. 14:1474229. doi: 10.3389/fcimb.2024.1474229
Received: 01 August 2024; Accepted: 29 August 2024;
Published: 07 October 2024.
Edited by:
Gaoqian Feng, Nanjing Medical University, ChinaReviewed by:
Shigang Yin, The Affiliated Hospital of Southwest Medical University, ChinaZhenkui Li, University of South China, China
Copyright © 2024 Tang, Fan, Lu, Jiang, Cheng, Zhang, Shen and Shang. This is an open-access article distributed under the terms of the Creative Commons Attribution License (CC BY). The use, distribution or reproduction in other forums is permitted, provided the original author(s) and the copyright owner(s) are credited and that the original publication in this journal is cited, in accordance with accepted academic practice. No use, distribution or reproduction is permitted which does not comply with these terms.
*Correspondence: Xiaomin Shang, eG1zaGFuZ0Bjc3UuZWR1LmNu
†These authors have contributed equally to this work