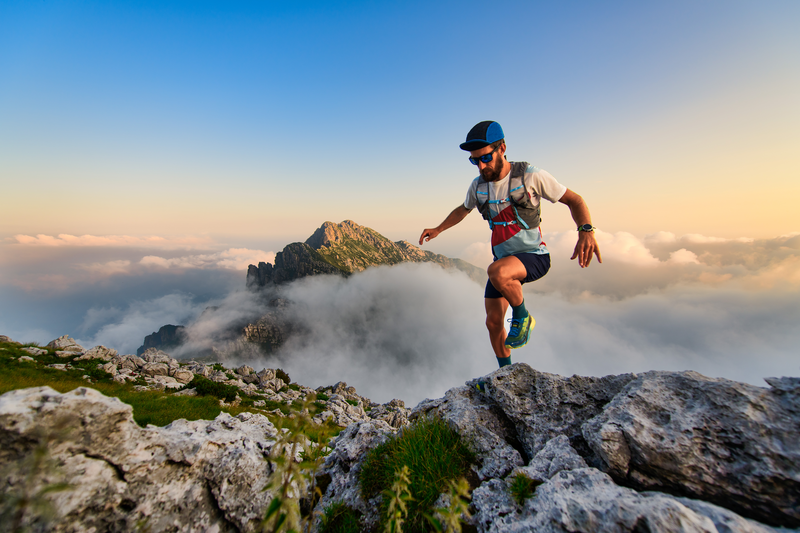
95% of researchers rate our articles as excellent or good
Learn more about the work of our research integrity team to safeguard the quality of each article we publish.
Find out more
REVIEW article
Front. Cell. Infect. Microbiol. , 26 November 2024
Sec. Intestinal Microbiome
Volume 14 - 2024 | https://doi.org/10.3389/fcimb.2024.1458033
This article is part of the Research Topic New Insights into Gut Microbiota in Colorectal Cancer View all 3 articles
Sex differences in colorectal cancer (CRC) has received considerable research attention recently, particularly regarding the influence of sex hormones and the intestinal microbiota. Estrogen, at the genetic and epigenetic levels, directly inhibits CRC cell proliferation by enhancing DNA mismatch repair, regulating miRNAs, blocking the cell cycle, and modulating ion channels. However, estradiol’s activation of GPER promotes oncogene expression. Conversely, androgen contributes to epigenetic dysregulation and CRC progression via nuclear receptors while inducing apoptosis through membrane receptors. Specific gut microorganisms produce genotoxins and oncogenic metabolites that damage colonic cell DNA and contribute to cancer induction. Regarding the tumor microenvironment, estrogen mitigates intestinal inflammation, reverses immunosuppression, increases gut microbiome diversity and commensal bacteria abundance, and decreases pathogen enrichment. On the contrary, androgen disrupts intestinal microecology, diminish immunotherapy efficacy, and exacerbate colonic inflammation and tumor growth. The impact of estrogen and androgen is closely tied to their receptor status, elucidating their dual roles in CRC pathogenesis. This review comprehensively discusses the direct and indirect effects of sex hormones and the intestinal microbiota on CRC, considering environmental factors such as diet and lifestyle to propose novel prevention and treatment strategies.
Colorectal cancer (CRC), a common malignant tumor of the digestive system, has exhibited an upward trend in morbidity and mortality in recent years (Siegel et al., 2024). Men typically display a higher incidence rate and poorer prognosis (Rubin et al., 2020; Baraibar et al., 2023). In the comprehensive investigation of CRC pathogenesis and prevention strategies, sex differences have emerged as a significant factor. Growing evidence indicates that variations in sex hormone levels, particularly estrogen and androgen, along with the intestinal microbiota, play a crucial role in the sexual dimorphism of CRC (Abancens et al., 2020; Salvador et al., 2023; Chang et al., 2024). Estrogen, for instance, may exert an inhibitory effect on CRC development in certain scenarios, whereas testosterone could stimulate the proliferation and metastasis of CRC cells (Barzi et al., 2013; Haziman et al., 2019; Song et al., 2021a). Furthermore, sex hormones may indirectly modulate colorectal carcinogenesis by influencing the intestinal microenvironment (Jiang et al., 2021; Matarrese et al., 2021). However, the levels of sex hormones are influenced by numerous factors such as genetic background, lifestyle choices, and environmental conditions, thereby increasing the complexity of CRC pathogenesis (Zhang et al., 2019). Consequently, the role of sex hormones in the development of CRC remains controversial. the intestinal microbiota, a vast and intricate ecosystem within the human body, significantly impacts host health. Recent findings highlight a strong link between the intestinal microbiota and sex disparities in CRC (Lin et al., 2019; Koyande et al., 2022; Yang et al., 2023). On one hand, there are notable differences in the composition and function of the intestinal microbiota between males and females, potentially affecting immune function and metabolic processes of the gut mucosa, consequently influencing CRC risk (Fan et al., 2021). On the other hand, the intestinal microbiota can impact the host’s sensitivity and resistance to chemotherapeutic agents, which may contribute to sex-specific variations in CRC treatment outcomes and prognosis (Lin et al., 2019). Importantly, various factors like sex hormone levels, dietary habits, and lifestyle choices can influence sex differences in the intestinal microbiota (Song et al., 2020c). Therefore, the relationship between the intestinal microbiota and sex disparities in CRC likely involves multiple complex interactions.
The recent exploration of concepts like the micro-genderome and the sex hormone-gut microbiome axis has highlighted the intricate interplay between sex hormones and the gut microbiota (Wallis et al., 2017; Ma and Li, 2019; Vemuri et al., 2019; Calcaterra et al., 2022). However, the precise mechanisms governing the interactions among CRC sexual dimorphism, sex hormone signaling, and the intestinal microbiota remain incompletely understood. This review delves into the bidirectional relationship between sex hormone signaling and the intestinal microbiota, with a focus on the direct effects (genetic and epigenetic alterations) and indirect pathways (modulation of inflammation and immune microenvironment) of sex hormones and the intestinal microbiota on CRC. Additionally, non-biological sex differences (such as diet and lifestyle) in the intestinal microbiota in CRC will be briefly discussed for comprehensive understanding. This comprehensive analysis is important for deciphering the pathogenesis of CRC and devising targeted prevention and treatment approaches.
Sex differences in the gut microbiota composition have been observed in both clinical samples and animal models. Research has shown that females generally exhibit higher microbial α-diversity and a higher Firmicutes/Bacteroidetes (F/B) ratio (Hokanson et al., 2024). However, the higher microbial α-diversity in women may be attributed to their relatively lower Bacteroidetes abundance, as evidence suggests a negative association between Bacteroidetes abundance and α-diversity (Thackray, 2019; Zhang et al., 2022). Factors like geographic location can also impact the F/B ratio (Manor et al., 2020). While summarizing sex-based differences in bacterial taxa abundance is challenging due to inconsistent findings across studies, it can be inferred that estradiol and testosterone play a role in maintaining gut microbiota diversity. Certain bacteria have been linked to sex hormones, with men having high serum testosterone levels showing enrichment for specific genera like Ruminococcus, Prevotella, Fusobacterium, Dorea, Acinetobacter, and Megamonas, while women with higher serum estradiol levels exhibit positive correlations with Bacteroidetes phyla, Akkermansia and Ruminococcus (del Castillo-Izquierdo et al., 2022). Additionally, the use of hormonal contraceptives has been found to alter gut microbiota composition in women (Sinha et al., 2019). For example, Oral contraceptives are associated with an increased abundance of species such as Bacteroides caccae, Coprobacillus unclassified, and Rothia mucilaginosa (Sinha et al., 2019). Bacteroides represent a significant group of microorganisms in the intestine (Magne et al., 2020), while Rothia mucilaginosa has been closely linked to the development of Crohn’s disease (Gevers et al., 2014). Previous studies, along with recent epidemiological evidence, have consistently indicated that the use of antiandrogen oral contraceptives reduces the risk of CRC, suggesting a potential protective role for estrogen (Bosetti et al., 2009; Bennink et al., 2024). However, there remains a lack of comprehensive research regarding the relationship between the duration of oral contraceptive use and the risk of side effects, highlighting the need for more high-quality evidence to support its availability. Animal studies have further explored the bidirectional relationship between sex hormones and gut microbiota, revealing significant differences in microbial populations between male and female mice. For instance, certain genera like Allobaculum, Anaeroplasma, and Erwinia were more abundant in males, while females had higher levels of Ruminococcus, Dorea, and Coprococcus (Org et al., 2016). Gonadectomy results in dysbiosis of the microflora (Org et al., 2016; del Castillo-Izquierdo et al., 2022; Song et al., 2023b; Cross et al., 2024). Specifically, in mice fed high-fat and high-sugar diets, ovariectomized (OVX) mice showed decreased abundance of Akkermansia, while orchiectomized (ORX) mice exhibited increased abundance of the Ruminococcacea family (Org et al., 2016). Microbial diversity and F/B ratios were notably reduced in ORX_mice treated with azoxymethane/dextran sodium sulfate (AOM/DSS) (Song et al., 2023b). The alterations in microbiota composition and diversity were significantly reversed with estrogen or testosterone replacement (Org et al., 2016; Song et al., 2023b). Furthermore, ovariectomy also led to increased fecal β-glucuronidase activity (Cross et al., 2024). The apparently contradictory findings on gut microbiome composition between humans and mice suggest that genetic variability among strains and environmental factors (such as diet and exposure to carcinogens) may obscure sex differences.
Estrogen is a key regulator of the gut microbiota, promoting the growth of bacteria that produce short-chain fatty acids (SCFAs). These SCFAs, such as butyric acid, are important metabolites resulting from gut microbe fermentation, crucial for maintaining intestinal homeostasis (Sun et al., 2018). Once produced in the colon, SCFAs are quickly absorbed by colonic cells and enter the citric acid cycle in mitochondria to generate ATP, providing energy to the cells (Koh et al., 2016; Dalile et al., 2019). In addition, SCFAs have anti-inflammatory properties, stimulate reactive oxygen species production, improve intestinal barrier function, modulate immune responses, and exhibit anti-CRC activity (Parada Venegas et al., 2019; Huang et al., 2023). Intestinal alkaline phosphatase (IAP), an antimicrobial peptide regulated by gut microbes, is up-regulated by estrogen, reducing Proteobacteria abundance and lipopolysaccharide biosynthesis to prevent chronic enteritis (Kaliannan et al., 2018). These findings suggest that sex hormone signaling can influence gut microbiota composition and diversity (Figure 1). However, studies on sex-related differences in the gut microbiota have yielded inconsistent results due to variations in study populations, methods, geography, and diet, posing a challenge in the field of gut microbiome research.
Figure 1. Bidirectional communication between sex hormones and the intestinal microbiota. Sex hormones (estrogen and androgen) play a crucial role in shaping the composition and diversity of the intestinal microbiota. Estrogen specifically supports the growth of SCFAs-producing bacteria and increases the expression of antimicrobial peptides to decrease the presence of pathogens. Conversely, the gut microbiota also influences sex hormone levels by expressing enzymes like β-glucuronidase and certain bacterial enzymes, as well as through interactions with the gut-brain axis. E1, estrone; E2, estradiol; E1S, estrone sulfate; E2S, estradiol sulfate; HSD, hydroxysteroid dehydrogenase; IAP, intestinal alkaline phosphatase; LPS, lipopolysaccharide; SCFAs, short chain fatty acids; STS, steroid sulfatase.
Not only sex hormone signaling alters the intestinal microbiota composition and diversify, but also the latter participate in the circulation and degradation of sex hormones (Figure 1). Certain bacteria, particularly in the gut microbiota of premenopausal women, possess genes involved in both the biosynthesis and degradation of sex hormones. These gut bacteria have the ability to metabolize sex hormones and their precursors (del Castillo-Izquierdo et al., 2022). Gut microbial β-glucuronidase is a key enzyme responsible for converting conjugated estrogens and androgens into their active forms (Hu et al., 2023). Through the action of β-glucuronidase, biologically active free sex hormones are reabsorbed via the enterohepatic circulation, thereby maintaining the body’s sex hormone levels and downstream physiological effects (Hu et al., 2023). Imbalances in the intestinal microbiota can disrupt the activity of β-glucuronidase, leading to a disruption in the breakdown of conjugated sex hormones and a decrease in free sex hormone levels, potentially resulting in various diseases (Hu et al., 2023). A study examining the impact of gut microbiota on intestinal androgen metabolism compared the levels of testosterone and dihydrotestosterone in the distal intestines of mice with normal gut microbiota and germ-free mice (Collden et al., 2019). The results revealed significantly higher levels of free dihydrotestosterone in the distal gut and feces of young adult males. In contrast, germ-free mice exhibited elevated levels of glucuronidated testosterone and dihydrotestosterone but lower levels of free dihydrotestosterone in the distal intestine (Collden et al., 2019). In addition, changes in intestinal flora appear to trigger androgen deficiency, causing metabolic disorders that lead to early death (Harada et al., 2020). These findings suggest a link between free estrogen and testosterone levels in the blood or gut and the presence of gut microbiota capable of metabolizing sex steroid hormones, implying that clinical testing of the abundance of specific bacteria in the feces may be able to reflect serum sex hormone levels.
The synthesis and metabolism of sex hormones involve a complex series of enzymatic reactions. Recent evidence suggests that specific bacterial enzymes, such as steroid sulfatase (STS), hydroxysteroid dehydrogenase (HSD), and steroid-17,20-desmolase, play a role in sex hormone biosynthesis alongside β-glucuronidase (Kwa et al., 2016; Thériault and Lin, 2019; Anbar et al., 2021). The conversion of estrone sulfate (E1S) and estradiol sulfate (E2S) to free estrone and estradiol is facilitated by STS. HSD17B1, HSD17B7, and HSD17B12 can convert estrone to estradiol, while HSD17B2 can metabolize estradiol back to estrone (Thomas and Potter, 2013). These findings indicate that the intestinal microbiota may impact estrogen bioavailability. Testosterone deficiency has been linked to depression-like behavior (Hauger et al., 2022). Studies have shown that Mycobacterium neoaurum, isolated from the feces of depressed patients with testosterone deficiency, can degrade testosterone in vitro (Li et al., 2022). In vivo experiments in rats confirmed that M.neoaurum reduced brain and serum testosterone levels, leading to depressive behavior, with 3β-HSD identified as the key enzyme responsible for degrading testosterone (Li et al., 2022). Moreover, certain bacteria in human feces have been found to metabolize estrogens and androgens (Rizzetto et al., 2018). While these studies support the role of bacterial enzymes in sex hormone metabolism, further research is needed to fully understand the complete bacterial pathways involved and their impact on human physiology. Beyond enzyme expression, gut microbes can influence sex hormone levels by directly affecting gonadal function (Shobeiri et al., 2022). Some gut microbes may interfere with endogenous sex hormone biosynthesis by modulating the hypothalamic-pituitary-gonadal axis via the gut-brain axis (So and Savidge, 2021; Hao et al., 2022; Hokanson et al., 2024).
Recent studies have indicated that the gut microbiota may influence the absorption of (phyto)estrogens, which in turn affects the ability of estrogens to bind to ERβ in the intestinal mucosa and counteract the activity of ERα, thereby playing a beneficial role in the prevention of colorectal neoproliferative lesions (Kumari et al., 2024). Consistent with this, estrogen primarily affects colonocytes through ERβ; notably, ERβ knockout in female mice results in decreased gut microbiota diversity and an increase in immune-damaging bacteria (Ibrahim et al., 2019; Ma et al., 2022). The gradual loss of ERβ during CRC development exacerbates the decrease in gut microbiota diversity and the enrichment of pathogens, such as the Bacteroidetes genus Prevotellaceae (Ibrahim et al., 2019). These findings suggest that the gut microbiota serves as a regulator of estrogen status, and that ERβ may inhibit the progression of colitis and colon cancer by modulating the gut microbiota. The human gut microbiota regulates estrogen metabolism primarily through the ‘estrogenome’—a collection of bacterial genes that encode enzymes such as β-glucuronidase, which activate estrogen and influence circulating levels. However, there is currently no evidence to suggest that changes in the gut microbiota affect the expression of ERα or ERβ.
Overall, sex hormones regulate the intestinal microbiota composition and diversity, which in turn can influence sex hormones levels (Figure 1; Box 1). This bidirectional communication is crucial in understanding sex differences in CRC. The following sections will delve into the direct effects and indirect pathways through which the sex hormone-gut microbiome axis affects the progression of CRC.
Box 1. Bidirectional communication between sex hormones and the intestinal microbiota.
In the classical pathway, sex steroid hormones bind to nuclear receptors in the cytoplasm, forming ligand-receptor complexes that induce conformational changes in the receptor, leading to its translocation to the nucleus. There, the receptor interacts with specific DNA sequences to selectively regulate gene transcription. In addition to these classical genomic mechanisms, sex steroid hormones can activate signal transduction pathways through non-genomic signaling mechanisms that function independently of their nuclear role (Wilkenfeld et al., 2018). Non-genomic signaling typically occurs on a much faster time scale and is often referred to as fast signaling. Rapid signals generally involve the translocation of ribonucleic acids to the plasma membrane, where they can directly or indirectly activate kinase pathways, resulting in a variety of physiological effects (Simoncini and Genazzani, 2003; Norman et al., 2004; Wilkenfeld et al., 2018). It is crucial to emphasize that non-genomic pathways can also lead to genomic effects. Consequently, many reported genomic effects mediated by sex steroid hormones may actually be partially the indirect result of rapid non-genomic signaling. The complex and diverse structure-function relationships between sex steroid hormones and their nuclear and membrane-associated receptors, along with the capacity of the same receptor to elicit both genomic and rapid responses, complicate the understanding of the role of sex steroid receptors in CRC (Norman et al., 2004).
ERα and ERβ are classical nuclear transcription factors characterized by six domains, which include a DNA-binding domain and a ligand-binding domain (Acconcia et al., 2017; Khan et al., 2022). Upon activation by estrogen, the ligand, both ERα and ERβ undergo conformational changes, homodimerize, and subsequently bind to the estrogen response element located in the promoter regions of target genes. This binding modulates gene transcription, ultimately influencing cell growth, differentiation, and apoptosis (Mauvais-Jarvis et al., 2013). Generally, estrogen exerts its effects on CRC primarily through ERβ-mediated genomic signaling. Certain CRC types show mutation accumulation due to mismatch repair (MMR) system failure, notably loss of MLH1 function (Lizardo et al., 2020). Estrogen has been found to inhibit MMR-proficient CRC while promoting MMR-deficient tumors (Honma et al., 2023). Estradiol enhances MLH1 expression in CRC cells, heightens sensitivity to 5-FU, and synergistically inhibits tumor growth (Jin et al., 2010; Lu et al., 2017). It also suppresses certain miRNAs (miR-135b, miR-31, and miR-155) expression and upregulates hMLH1 expression in CRC tissues or cells, regulating cancer cell differentiation, proliferation, and apoptosis (He et al., 2012). The impact of estradiol on miRNAs in various CRC cell lines seems to be associated with the expression level of ERβ (He et al., 2012). The circadian system affects cancer progression differently in males and females (Zhou et al., 2022; Levi et al., 2024). miR-34a, a CRC suppressor, notably inhibits the expression of the clock genes per2 and Bmal1. While estradiol significantly reduces the proliferative and migratory activity of DLD1 cells, it does not have a substantial effect on miR-34a levels (Moravcik et al., 2023). However, estradiol seems to hinder CRC growth by modulating high-fat diet (HFD)-induced changes in the clock genes Bmal1 and Npas2 via ERβ (Hases et al., 2020). Furthermore, when combined with progesterone or 5-FU, estradiol promotes ERβ expression in CRC cells, reduces the expression of the cell cycle markers CCND1/3, ultimately leading to cell cycle arrest in the sub-G1 phase and apoptosis (Mahbub, 2022; Mahbub et al., 2022). Changes in ion transporter activity are one of the epigenetic alterations identified in CRC. Estrogens are recognized as regulators of epithelial ion channels and influence the function of the voltage-gated K+ channel (KCNQ1:KCNE3) and the CFTR Cl- channel (Anderson et al., 2019; Abancens et al., 2020). Both KCNQ1 and CFTR are downregulated in CRC (Anderson et al., 2019; Abancens et al., 2020). KCNQ1 inhibits the Wnt/β-catenin pathway, thereby suppressing CRC cell proliferation and metastasis (Abancens et al., 2020). The absence of CFTR results in mucus blockage, inflammation, and microbial dysbiosis (Anderson et al., 2019). These findings suggest that estradiol directly inhibits CRC cell proliferation and induces apoptosis primarily through mechanisms such as promoting DNA mismatch repair, modulating miRNA and clock genes, arresting the cell cycle, and regulating ion channels (Figure 2). It is important to note that further validation of these results is necessary in future studies, considering variations in study methodologies, types of CRC cell lines, and concentrations and exposure times of estradiol.
Figure 2. Estrogen-induced genetic and epigenetic alterations in CRC. Estradiol inhibits CRC cell proliferation by promoting DNA mismatch repair, regulating miRNAs, blocking the cell cycle, and modulating ion channels. However, in conditions of ERβ deficiency and hypoxia, estradiol can activate non-genomic signaling via GPER, thereby promoting oncogene expression. ASNS, asparagine synthase. ATM, Ataxia telangiectasia mutated; CRC, colorectal cancer; CTGF, connective tissue growth factor; E2, estradiol/17β-estradiol; ERβ, estrogen receptor beta; GPER, G protein-coupled estrogen receptor; HFD, high-fat diet; MMR, mismatch repair; dMMR, deficient MMR; pMMR, proficient MMR; P4, progesterone; STS, steroid sulfatase.
In the absence of ERβ, estradiol initiates rapid signaling events primarily through the membrane receptor GPER, which contributes to pro-tumor effects in CRC (Figure 2). Typically, GPER signaling occurs via transactivation of the epidermal growth factor receptor. In addition to these rapid signaling events, GPER also regulates the FOS gene by activating signaling pathways involving cAMP, PI3K, and ERK, which promotes the formation of the transcription factor AP-1 and indirectly influences transcriptional activity (Arterburn and Prossnitz, 2023). As CRC advances, ERβ levels decrease while GPER remains expressed, making it a key player in estradiol’s impact on CRC. Clinical evidence indicates that high GPER expression is linked to a worse prognosis only in female patients with advanced CRC, not affecting survival in early-stage female patients or male patients at any stage (Bustos et al., 2017; Gilligan et al., 2017; Abancens et al., 2022; Lu et al., 2023). Under normoxic levels, estradiol inhibits the proliferation and migration of ERβ-negative CRC cells. However, under hypoxic conditions, GPER activated by estradiol promotes angiogenesis by suppressing the Ataxia telangiectasia mutated (ATM, a key MMR gene), and increasing VEGFA and HIF-1α expression (Bustos et al., 2017). Given the hypoxic nature of advanced CRC and the functional similarities between estrogen and hypoxia, this combined effect may explain the sex differences observed in female patients with advanced CRC and their lower survival rates when GPER expression is high. Furthermore, estradiol-induced GPER activation triggers centrosome amplification in HCT-116 and HT-29 cells, leading to the formation of alignment defects and lagging chromosomes that drive malignant progression (Buhler et al., 2023). Although estradiol does not affect the survival of KRAS-mutant CRC cells, these cells can adapt to nutrient scarcity by upregulating GPER and asparagine synthetase (ASNS) expression in the absence of glutamine (Lu et al., 2023). Previously mentioned as a bacterial enzyme facilitating the desulfuration of E1S and subsequent estradiol production (Thomas and Potter, 2013), STS stimulation leads to further GPER activation by estradiol, promoting connective tissue growth factor (CTGF) expression and stimulating CRC cell proliferation (Gilligan et al., 2017). Therefore, elevated STS activity is regarded as an indicator of poor prognosis in CRC. These results indicate that GPER, ATM, ASNS, and STS could serve as possible targets for therapy in CRC. However, it is also suggested that estrogen replacement therapy, tamoxifen and fulvestrant (known GPER agonists) commonly used in clinical practice, may have a negative impact on CRC prevention and treatment. Therefore, caution is advised for advanced CRC patients considering the use of these medications.
Like estrogens, androgens function through specific receptors known as androgen receptors (ARs). Structurally, ARs consist of two distinct subtypes: AR-α and AR-β. These isoforms act as ligand-activated transcription factors and exhibit differential expression in colon cancer tissue compared to healthy colon mucosa. At the mRNA level, ARs are present in both normal and colon cancer mucosa; however, at the protein level, both isoforms are expressed in normal colon mucosa. In contrast, AR-α is exclusively expressed in colon cancer, while the expression of AR-β is absent in colon cancer (Catalano et al., 2000). This indicates that the expression of AR-α in colon cancer cells may serve as a distinctive feature of CRC. Evidence suggests that testosterone may contribute to the development of CRC through multiple pathways, and serum free testosterone levels, together with carcinoembryonic antigen, are expected to serve as a potential biomarker for CRC (Roshan et al., 2016). Similar to estrogens, androgens act synergistically through intracellular ARs (iARs). iARs are DNA-binding transcription factors encoded by genes located on chromosome Xq11-12. In the general population, iARs contain varying numbers of cytosine-adenine-guanine (CAG) repeats that regulate gene expression and protein synthesis (Roshan et al., 2016). Patients with CRC tend to have longer CAG repeat lengths, increasing the risk of CRC in both males and females (Roshan et al., 2016). Epigenetic dysregulation is a susceptibility factor for tumorigenesis, with methylation of iARs genes potentially playing a role in sex differences in CRC (Xia et al., 2019; Chen et al., 2022b). An analysis of a cohort study indicated that hypomethylation of iARs in peripheral blood was associated with a higher risk of CRC, suggesting that iARs in peripheral blood could be a biomarker for CRC (Xia et al., 2019). The histone demethylase JMJD2D is an epigenetic factor that coordinates the activation of iARs. JMJD2D is highly expressed in the testis and promotes CRC progression by activating β-catenin and HIF1α to induce the expression of various oncogenes and glycolysis-related genes (Chen et al., 2022b). These findings imply that epigenetic dysregulation mediated by iARs could be a potential mechanism for testosterone-induced CRC development (Figure 3). However, there are conflicting views as well. Previous reports have shown that testosterone induces apoptosis in CRC through membrane ARs (mARs) (Gu et al., 2009, 2010, 2013). Activation of mARs, a class of G-protein-coupled receptors located on the cell surface, primarily occurs in CRC rather than in normal colonic mucosa (Roshan et al., 2016). Testosterone-albumin couplings selectively bind to mARs, inhibiting CRC cell invasion and migration while inducing apoptosis through the activation of Akt, caspase-3, and rapid actin reorganization (Gu et al., 2009, 2010, 2013). The discovery that androgens promote apoptosis in CRC cells aligns with certain clinical data suggesting that testosterone may have a potentially protective effect against CRC (Dashti et al., 2020). Overall, androgens contribute to epigenetic dysregulation and CRC progression primarily through the nuclear receptors, whereas their interaction with membrane receptors may trigger apoptosis (Figure 3). However, there is limited evidence regarding the direct impact of androgens on CRC genetics and epigenetics, necessitating further studies to validate these concepts.
Figure 3. Androgen-related genomic and non-genomic signals in CRC. Androgens (testosterone) contribute to epigenetic dysregulation and CRC progression primarily through the nuclear receptors, whereas their interaction with membrane receptors may trigger apoptosis. CAG, cytosine-adenine-guanine. CRC, colorectal cancer; iAR, intracellular androgen receptor; mAR, membrane androgen receptor.
The intestinal microbiota is categorized into probiotic (e.g., Bifidobacterium and Lactobacillus), conditionally pathogens (e.g., Enterobacter and Enterococcus), and pathogens (e.g., Salmonella, Proteus, and Escherichia coli) (Chen et al., 2022a). CRC is linked to a reduction in overall gut microbial diversity and probiotics, along with an increase in pathogens compared to healthy individuals (Geng et al., 2013; Brennan and Garrett, 2016). The predominant flora in CRC includes bacteria like Fusobacterium nucleatum, E. coli, Streptococcus gallolyticus, and Bacteroides fragilis, particularly F. nucleatum (Lin et al., 2019; Koyande et al., 2022). F. nucleatum has been found in high levels in tumor tissues with microsatellite instability-high and CpG island methylator phenotype-high (Lin et al., 2019). In addition, F. nucleatum promotes the malignant phenotype of CRC cells by producing FadA adhesin that interacts with E-cadherin in epithelial cells and triggers the expression of inflammatory cytokines, transcription factors and Wnt genes (Castellarin et al., 2012; Fan et al., 2021; Wu et al., 2022). As mentioned previously, sex hormone signaling can influence the composition and diversity of gut microbiota in healthy individuals. Recent research suggests that CRC may also exhibit sex-specific differences in gut flora composition (Koyande et al., 2022; Yang et al., 2023). Clinical samples from CRC patients revealed that male patients had higher levels of Bacteroides, Eubacterium, and Faecalibacterium, while female patients had higher levels of Bacteroides, Subdoligranulum, and Eubacterium. Additionally, Dorea and Bacteroides were identified as key bacteria associated with sex and CRC, while Blautia, Barnesiella and Anaerostipes were among the most sexually diverse bacteria in CRC (Yang et al., 2023). This indicates that males and females may possess distinct gut microbiota that either promote or inhibit CRC progression. However, it remains unclear whether these microbial imbalances contribute to CRC development or are a result of the disease. Further investigation is needed to explore the relationship between sex-related bacteria, sex hormones, and dietary habits in the context of CRC.
Microbial-induced genetic alterations that activate oncogenes or inactivate tumor suppressor genes play a significant role in colorectal carcinogenesis. Normally, gut microbes help protect the intestinal mucosa and prevent pathogen invasion by preventing DNA damage and maintaining the integrity of the intestinal barrier (Chen et al., 2022a). Dysregulation of the intestinal microecology can lead certain microorganisms to produce virulence factors and metabolites that promote the development of CRC (Figure 4). For example, some intestinal flora generate genotoxic toxins like enterotoxins and reactive oxygen species (ROS) from B. fragilis and Enterococcus faecalis, as well as the cytolethal distending toxin (CDT) produced by E. coli, which can cause DNA damage, disrupt the cell cycle, induce inflammation, and directly harm the intestinal epithelial cells (Lin et al., 2019; Chen et al., 2022a; Koyande et al., 2022; Qu et al., 2023; Wong and Yu, 2023). Additionally, bacterial metabolites such as secondary bile acids, acetaldehyde, and glucuronic acid, serve as energy sources for microbes and can accelerate CRC development by causing DNA damage, gene mutations, and the formation of oxygen radicals (Lin et al., 2019; Avuthu and Guda, 2022; Koyande et al., 2022). The genetic and epigenetic alterations triggered by these microorganisms are potential contributing factors to CRC, resulting in abnormal cell proliferation and malignant transformation of intestinal epithelial cells (Raskov et al., 2017). While there is some evidence indicating a possible causal link between gut microbiota and CRC, it remains unclear whether these bacteria act independently or in conjunction with other microorganisms to induce and advance colorectal carcinogenesis.
Figure 4. The intestinal microecological dysregulation-induced genotoxicity. Dysregulation of the intestinal microecology can lead certain microorganisms to produce virulence factors and metabolites that promote the development of colorectal cancer. CDT, cytolethal distending toxin; ROS, reactive oxygen species.
Taken together, both sex hormones and gut microbiota have dual effects on CRC. Estradiol inhibits CRC cell proliferation by mechanisms such as promoting DNA mismatch repair, regulating miRNAs and clock genes, blocking the cell cycle, and modulating ion channels. However, in conditions of ERβ deficiency and hypoxia, estradiol can activate non-genomic signaling through the membrane receptor GPER, thereby promoting oncogene expression. On the other hand, androgens contribute to epigenetic dysregulation and CRC progression primarily via nuclear receptor activation, while their interaction with membrane receptors can trigger apoptosis. Dysregulation of the intestinal microecology can result in the production of genotoxins and oncogenic metabolites, causing direct damage to colon cell DNA and promoting cancer development (Figures 2–4; Box 2). Despite indications of a potential direct causal link between sex hormone signaling, gut microbiome, and CRC, there is insufficient evidence to support the notion that the interplay between sex hormone signaling and the intestinal microbiota significantly contributes to sex-based differences in CRC. Current research predominantly focuses on exploring the impact of the sex hormone-gut microbiome axis on the colon tumor microenvironment (TME), particularly in terms of inflammation and immunity, as elaborated in the subsequent section.
Box 2. Direct effects of sex hormones and the intestinal microbiota on CRC: genetic and epigenetic alterations.
Inhibition of inflammatory signaling and pro-inflammatory cytokines expression is a key mechanism through which estrogen reduces the risk of colitis-associated cancer (CAC) (Son et al., 2019; Song et al., 2019). Previous studies have shown that ovariectomy worsens colonic inflammation and tumorigenesis in AOM/DSS model mice, while supplementation with 17β-estradiol (E2) significantly decreases inflammation and malignant progression. Mechanistically, estradiol binds to ERβ and inhibits the release of inflammatory mediators like COX-2, TNF-α, IFN-γ, and IL-6 by blocking NF-κB signaling (Armstrong et al., 2017; Son et al., 2019; Song et al., 2019; Son et al., 2020). On the other hand, estradiol enhances NLRP3 inflammasome expression, which helps reduce intestinal inflammation (Son et al., 2019). Chronic colitis can disrupt gut microbial balance and trigger colon carcinogenesis, which in turn leads to a further reduction in the gut microbiota diversity, especially in the absence of ERβ (Armstrong et al., 2017; Ibrahim et al., 2019; Lee et al., 2019; Song et al., 2020b). In the AOM/DSS model, modulating the gut microbiota with antibiotics lowered pro-inflammatory cytokines levels and colon tumorigenesis, with estradiol supplementation yielding similar results (Lee et al., 2019; Song et al., 2020b). Estradiol increased gut microbial diversity, boosted commensal bacteria levels, and reduced opportunistic pathogens and the F/B ratio in male and female mice (Song et al., 2020b). Probiotics stimulate anti-inflammatory cytokines production, while pathogens trigger the release of inflammatory mediators, pro-inflammatory toxins, and ROS (Lin et al., 2019; Fan et al., 2021; Koyande et al., 2022). In the absence of ERβ, both male and female AOM/DSS mice exhibited decreased gut microbial diversity, particularly male mice with an abundance of flora linked to cell motility and metabolism (Ibrahim et al., 2019). Carnobacterium maltaromaticum has shown significant inhibition of intestinal tumor formation in female mice but has minimal impact on male mice, indicating its potential anticancer activity in the presence of estrogen (Li et al., 2023). Estradiol is thought to enhance the attachment and colonization of C. maltaromaticum by upregulating colonic SLC3A2 expression. The colonization of C. maltaromaticum leads to a shift in gut microbiota composition, increasing beneficial butyric acid-producing bacteria like Faecalibacterium prausnitzii and Lachnispiraceae bacterium while reducing levels of opportunistic pathogens such as Bacteroides vulgatus and Muribaculum intestinale. These beneficial bacteria activate vitamin D receptor (VDR) signaling, reduce mucosal inflammation, and maintain intestinal barrier function (Li et al., 2023). These findings suggest that estrogen and ERβ contribute to a favorable gut microbiota, crucial for mitigating inflammatory responses and preventing colorectal carcinogenesis (Figure 5). Modulating the gut microbiota using antibiotics or estrogens may hold promise as a potential therapeutic approach for CAC. It is important to note that the AOM/DSS model primarily simulates CRC associated with inflammatory bowel disease. In addition to its connections to sex hormones and an imbalance in intestinal flora, the malignant progression of this cancer is also linked to a reduced rate of epithelial cell renewal and apoptosis. Furthermore, this progression is associated with a decrease exposure time to genetic mutants (Girardi et al., 2018).
Figure 5. Co-regulation of colorectal inflammatory microenvironment by sex hormones and gut microbiota. Estrogen may slow the progression of CAC by promoting a more favorable gut microecology that reduces intestinal inflammation and inhibits oxidative stress damage. This includes increasing the diversity of the gut microbiome and the abundance of probiotics while simultaneously decreasing the abundance of pathogens. On the contrary, androgen may play a role in disrupting gut microecological balance, which could exacerbate colonic inflammation and heighten the susceptibility to intestinal tumor development. AOM/DSS, azoxymethane/dextran sodium sulfate; CAC, colitis-associated cancer; E2, estradiol/17β-estradiol; ERβ, estrogen receptor-beta; KO, knockout; ORX, orchiectomized; ROS, reactive oxygen species; SCFAs, short chain fatty acids; VDR, vitamin D receptor.
Oxidative stress can lead to an increase in intracellular oxygen free radicals and ROS, which in turn can activate inflammatory responses. During the initial phases of colitis, estradiol boosts the expression of Nrf2 and its associated antioxidant enzymes HO-1, NQO1, GCLC, and GCLM, thereby reducing oxidative stress damage and inflammatory reactions (Son et al., 2019; Song et al., 2019). Nonetheless, in the absence of Nrf2, estradiol may hinder the progression of distal CRC through an ERβ-dependent pathway (Song et al., 2020a). Apart from upregulating antioxidant enzymes, estradiol also triggers the expression of neuroglobin, an oxidative stress sensor. In the absence of oxidative stress, estradiol induces apoptosis in CRC cells; however, in the presence of high oxidative stress, neuroglobin interacts with cytochrome C to inhibit the subsequent apoptotic process, thereby counteracting the pro-apoptotic impact of estradiol (Fiocchetti et al., 2015). Emerging evidence indicates that the Nrf2 genotype can influence the intestinal microbiota composition (Song et al., 2021b). B. vulgatus promotes inflammatory responses and the malignant advancement of colon adenomas in cases where gut barrier function is compromised. Treatment with AOM/DSS leads to an increase in the abundance of B. vulgatus in both male and female mice. Conversely, Nrf2 knockout results in a decrease in the abundance of the probiotic Lactobacillus murinus in male mice. Furthermore, the levels of the intestinal pathogen Akkermansia muciniophila are elevated in male mice irrespective of Nrf2 knockout (Song et al., 2021b). These findings suggest that estrogen may delay the progression of CAC by fostering a more favorable gut microbiota that helps mitigate oxidative stress damage and intestinal inflammation (Figure 5).
In comparison to estrogen signaling, androgen seems to contribute to dysregulation of the intestinal microecology, colonic inflammation, and tumor progression (Song et al., 2021a, 2023b; Wang et al., 2023). Studies using the AOM/DSS model have shown that orchiectomy in male mice significantly reduced the severity of colitis and distal colonic adenomas (Song et al., 2021a, 2023b). Additionally, mice without testes had higher gut microbial diversity and increased F/B ratios (Song et al., 2023b). When testosterone was supplemented, it led to the upregulation of inflammatory mediators like COX-2 and iNOS, as well as an increase in opportunistic pathogens such as Mucispirillum schaedleri or Akkermansia muciniphila, exacerbating colonic inflammation and submucosal invasive carcinoma (Song et al., 2021a, 2023b). Moreover, in pseudo-germ mice that received fecal samples from male mice or men, A. muciniphila was significantly enriched while the probiotic Parabacteroides goldsteinii was reduced, resulting in increased intestinal inflammation and disruption of barrier function (Wang et al., 2023). Mechanistically, the metabolites from these pathogens activate the glycerophospholipid metabolic pathway, ultimately worsening colon tumorigenesis in males (Wang et al., 2023). These findings suggest that androgen, particularly testosterone, may induce intestinal microecological dysregulation and chronic inflammation, potentially contributing to sex differences in CRC (Figure 5).
Overall, estrogens have been shown to potentially reduce intestinal inflammation and slow the progression of CAC by promoting a more favorable gut microecology. This includes increasing gut microbiome diversity and commensal bacteria abundance, while decreasing pathogen abundance. Conversely, androgen may contribute to intestinal microecological dysregulation, leading to worsened colonic inflammation and increased risk of intestinal tumor formation (Figure 5; Box 3). However, current evidence is limited, with most studies focusing on differences in strains influenced by sex hormones or sex, rather than exploring the specific molecular mechanisms underlying the interaction between sex hormones and the intestinal microbiota in relation to intestinal inflammation.
Box 3. Indirect pathways of sex hormones and the intestinal microbiota on CRC: tumor microenvironment.
It is well known that the expression of programmed death-ligand 1 (PD-L1) in tumor cells or immune cells can inhibit T cell cytotoxicity (Dammeijer et al., 2020). In both AOM/DSS and MC38 colon tumor models, there was a high presence of PD-L1-positive tumor cells, M2-like tumor-associated macrophages (TAMs), myeloid-derived suppressor cells (MDSCs), Treg cells, and cancer-associated fibroblasts (CAFs), along with a low presence of cytotoxic CD8+ T cells (Jiang et al., 2021; Kang et al., 2021; Song et al., 2022). Estradiol supplementation was found to reverse this suppressive immune microenvironment and enhance the body’s anti-tumor immune response (Jiang et al., 2021; Kang et al., 2021; Song et al., 2022). Additionally, extracellular vesicles (EVs) derived from MC38 colon tumors were also found to contribute to the immunosuppressive TME. Estradiol was shown to decrease the levels of the immunosuppressive factor TGF-β1 in EVs, ultimately inhibiting MC38 tumor growth (Jiang et al., 2021). Interestingly, different concentrations of estradiol did not impact the viability of MC38 cells or the expression of PD-L1 in these cells [96]. Furthermore, estradiol treatment before injecting MC38 cells led to a significant reduction in tumor weight, whereas treatment after cell injection had no effect on tumor growth (Song et al., 2022). Based on the discovery that estradiol reduces PD-L1 expression in colon tumor tissues to impede tumor growth, researchers also observed that pre-treatment with estradiol before αPD-L1 therapy led to alterations in the gut microbiota composition and diversity in male mice (Song et al., 2020b, 2022, 2023a). The combination of estradiol and αPD-L1 treatment resulted in increased levels of the intestinal probiotic Parabacteroides goldsteinii and Lactobacillus murinus group, while reducing the presence of the opportunistic pathogen Enterobacteriaceae family in MC38 male mice (Song et al., 2023a). However, changes in the F/B ratios varied among different models, with estradiol treatment alone or in combination with αPD-L1 increasing F/B ratios in MC38 mice, but significantly decreasing in AOM/DSS mice (Song et al., 2020b, 2023a). Despite a decrease in the relative abundance of certain commensal bacteria (PAC000664 and Phocea) and opportunistic pathogens (Pseudoflavonifractor and Neglecta) in estradiol-treated AOM/DSS male mice, the ratio of commensal bacteria to opportunistic pathogens remained elevated (Song et al., 2020b). Overall, these findings suggest that estradiol downregulates PD-L1 expression, modulates the population of infiltrating immune cells and tumor-associated cells, and fosters a gut microecological environment conducive to immune recovery. Through its interaction with the intestinal microbiota, estradiol collaborates to reverse the suppressive immune microenvironment and enhance the body’s anti-tumor immunity, ultimately hindering the progression of CRC (Figure 6).
Figure 6. Co-regulation of colorectal immune microenvironment by sex hormones and gut microbiota. Estradiol may enhance the body’s anti-tumor immunity by downregulating PD-L1 expression, modulating the population of infiltrating immune cells, and fostering an intestinal microecology that supports immune recovery to counteract the suppressive TIME. Conversely, testosterone could have a negative impact on αPD-L1 therapy, and reducing testosterone levels might change gut microbial composition and enhance the effectiveness of immunotherapy. CRC, colorectal cancer; C/O, commensal bacteria/opportunistic pathogens; E2, estradiol/17β-estradiol; EVs, extracellular vesicles; F/B, Firmicutes/Bacteroidetes; ICIs, immune checkpoint inhibitors; MDSCs, myeloid-derived suppressor cells; PD-L1, programmed death-ligand 1; TIME, tumor immune microenvironment.
Estradiol enhances the efficacy of αPD-L1, but clinical data indicates that αPD-L1 treatment is more effective in male patients than in female patients (Wang et al., 2021). In the MC38 colon tumor model, it was observed that αPD-L1 reduced testosterone levels in male mice without affecting sex hormone levels in females. αPD-L1 did not impact the gut microbiota in males, but it decreased the abundance of Lachnospiraceae in females, a group associated with positive responses to immune checkpoint inhibitors (ICIs). Mechanistically, αPD-L1 disrupted lipid metabolism and caused inflammation in testes, leading to decreased testosterone production. Moreover, the administration of antibiotic colistin during anti-PD-L1 treatment further lowered testosterone levels and increased estradiol levels in male mice, resulting in alterations in gut microbiota composition and function (Wang et al., 2021). These findings suggest that reducing testosterone levels enhances the efficacy of immunotherapy (Figure 6). The use of specific narrow-spectrum antibiotics, such as neomycin and metronidazole, alleviated ICIs-associated colitis in MC38 mice and improved the anti-tumor efficacy of αPD-L1 in male mice, while negatively affecting its efficacy in female mice. These differences were linked to sex-specific variations in the intestinal microbiota, particularly in the abundance of Muribaculaceae and Lachnospiraceae (Jing et al., 2022). The study highlights the importance of considering sex differences in the gut microbiota when using antibiotics to manage ICIs-associated colitis.
The intestinal microbiota plays a crucial role in shaping the host immune system’s development and function, which is essential for maintaining the key features of the host-microbe symbiosis (Zheng et al., 2020). Whereas the immune system is capable of sex differences, the gut microbiota is simultaneously influenced by sex hormones that control sex differences (Rizzetto et al., 2018). There is extensive communication between the gut microbiota and host immunity, with potential implications for cancer immune surveillance. For instance, the presence of F. nucleatum in the colorectal TME can directly impede the killing of tumors by NK cells and is associated with lower CD3+ T cell levels (Gur et al., 2015; Mima et al., 2015). Pathogenic bacteria like F. nucleatum, Klebsiella pneumoniae, and Peptostreptococcus anaerobius can enhance the activity of tumor-derived cytokines, leading to the recruitment of MDSCs and M2-subtype TAMs, thereby supporting CRC progression (Wong and Yu, 2023). In mice lacking both NOD2 and CYBB, the pathogen Mucispirillum schaedleri can induce Th1 cell-mediated intestinal inflammation (Caruso et al., 2019). Furthermore, inflammation-induced disruption of the gut barrier can result in the translocation of bacterial symbionts into the mucosal layer, triggering abnormal host immune responses and tissue damage (Zheng et al., 2020). In contrast, certain intestinal probiotics can stimulate T cell production, activate NK cells, boost macrophage phagocytic activity, and enhance the body’s anti-tumor immune response (Lin et al., 2019; Fong et al., 2020; Zheng et al., 2020; Koyande et al., 2022) (Figure 6). These discoveries highlight the significant role of the intestinal microbiota in cancer immune surveillance and immunotherapy, suggesting its potential for optimizing the immunotherapeutic response in CRC patients.
Collectively, estradiol may enhance the body’s anti-tumor immunity by downregulating PD-L1 expression, modulating the population of infiltrating immune cells, and fostering an intestinal microecology that supports immune recovery to counteract the suppressive tumor immune microenvironment (TIME). Conversely, testosterone could have a negative impact on αPD-L1 therapy, and reducing testosterone levels might change gut microbial composition and enhance the effectiveness of immunotherapy (Figure 6; Box 3). These results indicate that sex hormones and gut microbiome may be pivotal in CRC immunotherapy. Estradiol and specific probiotics could be valuable targets for boosting αPD-L1 efficacy. Moreover, sex-specific variations in the intestinal microbiota should be taken into account when using antibiotics to manage ICI-associated colitis.
Common research primarily focuses on the effects of endogenous sex hormones on intestinal microbiota and CRC. Notably, specific lifestyle choices and hormone therapies also influence human sex hormone levels and gut microbiota abundance. Previous studies have indicated that various dietary patterns affect estrogen levels differently in premenopausal women. Estrogen concentrations were found to be inversely associated with overall diet quality, as assessed by the Alternative Healthy Eating Index model, but not with diet quality evaluated by the Dietary Approaches to Stop Hypertension or the alternative Mediterranean Eating pattern (Hirko et al., 2016). Evidence suggests that low energy intake can adversely affect serum testosterone concentrations, and certain vitamins and minerals are crucial for testosterone synthesis (Zamir et al., 2021). Additionally, a high-cholesterol diet may lead to reduced serum testosterone levels in rats (Liu et al., 2023). However, some clinical trials have reported that micronutrients do not significantly impact free testosterone and estradiol levels (Janjuha et al., 2020). Additionally, long-term follow-up evidence from the Women’s Health Initiative does not support the addition of vegetables, fruits, and grains to a low-fat diet in menopausal women for the prevention of CRC (Manson et al., 2024). It is important to note that these findings are limited, and all experiments or trials exhibit notable methodological limitations. Phytoestrogens are present in a wide variety of foods and plants, and they can disrupt the endocrine system by interfering with the hypothalamic-pituitary-gonadal axis, which regulates estrogen secretion. The effects of phytoestrogens on the body can vary depending on the individual’s life stage. Additionally, phytoestrogens can alter levels of sex hormone-binding globulin and influence the biological activity of circulating estrogen and androgen (Domínguez-López et al., 2020). Studies have demonstrated that the phytoestrogen isoflavones stimulate the synthesis of hormone-binding globulins in hepatocellular carcinoma cells and inhibit aromatase and other enzymes involved in steroid hormone synthesis (Domínguez-López et al., 2020). Isoflavones can be converted to S-estrone by intestinal bacteria; however, not all individuals produce S-estrone. Results from trials involving individuals at high risk of CRC suggest that isoflavones may reduce insulin-growth factor, but this effect appears to occur only in those who produce estramustrol (Usui et al., 2013). In addition to the effects of dietary and exogenous hormones on sex hormone levels, hormone therapy significantly influences the onset of CRC. Research indicates that individuals undergoing postmenopausal hormone replacement therapy can reduce their risk of CRC by over 20% (Murphy et al., 2015). Clinical trials suggest that estrogen-progesterone therapy may decrease women’s absolute risk of CRC; however, the overall health risks associated with the use of estrogen plus progesterone, such as a potential increased risk of breast cancer, outweigh the benefits (Rossouw et al., 2002; Genazzani et al., 2021). While the evidence remains inconsistent, it appears that overall menopausal hormone therapy is safe for many cancer patients (Hickey et al., 2024).
The composition and diversity of the intestinal microbiota are influenced by host physiology and environmental factors. Increasing evidence suggests that environmental factors such as high-fat diet (HFD), smoking, alcohol consumption, overweight, and obesity can also impact CRC by inducing significant changes in the intestinal microbiota composition (O’Keefe, 2016; Capurso and Lahner, 2017; Haziman et al., 2019; Song and Chan, 2019; Zheng et al., 2020; Chang et al., 2024). Men, often influenced by social background factors, tend to consume more fat, smoking, and alcohol than women, contributing partially to the increased risk of CRC in men (Sun et al., 2023). Therefore, it is necessary to differentiate between the effects of dietary and lifestyle choices and true biological sexual dimorphism on CRC. A substantial body of evidence indicates that HFD can contribute to the progression of CRC by impacting the intestinal microbiota and its metabolites, as well as influencing immune cell function (Devkota et al., 2012; Cheng et al., 2016; O’Keefe, 2016; Yang et al., 2022; Song et al., 2023c; Cross et al., 2024). HFD has been shown to disrupt gut barrier function, leading to increased proliferation and metastasis of CRC cells by altering the balance of intestinal pathogens (such as Alistipes sp. Marseille-P5997) and harmful metabolites while reducing probiotics like Parabobacterides distasonis (Yang et al., 2022). Oophorectomy has been found to increase intestinal permeability and inflammation, promoting a microbiota composition associated with metabolic dysfunction, which is further exacerbated by HFD (Cross et al., 2024). Furthermore, HFD elevates levels of secondary bile acids and Bilophila wadsworthia, leading to Th1-type immune responses (Devkota et al., 2012), and decreases butyric acid levels, disrupting intestinal dendritic cell homeostasis (Cheng et al., 2016) and promoting colitis and CAC development. Additionally, HFD in conjunction with the HNF1AA98V variant activates β-catenin, contributing to colon polyp formation (Song et al., 2023c). Untimely dietary intake interacts with alcohol consumption to accelerate alcohol-associated CRC by decreasing the population of SCFAs-producing bacteria (Bishehsari et al., 2020). Dietary habits, such as high consumption of red and processed meats, low intake of dietary fiber, and alcohol consumption, are also recognized as risk factors for CRC. Preservatives in red meat, processed meats and dairy products produce chemicals during cooking. These chemicals are metabolized by intestinal bacteria to produce harmful metabolites that promote colonic inflammation and tumors (Viennois et al., 2017; Song et al., 2020c). Diets rich in fiber support a beneficial relationship between gut microbes and their hosts. Fiber fermentation generates SCFAs like butyrate, propionate, and acetate, which are crucial for the energy supply of gut microbiota and the maintenance of colonic mucosal health (O’Keefe, 2016). Exposure to alcohol and smoking, in addition to dietary habits, can result in notable alterations in the composition of the intestinal microbiota. This includes a reduction in ‘anti-inflammatory’ bacteria from the Firmicutes phylum, such as Lactococcus, Pediococcus, and Ruminococcus sp., and an increase in ‘pro-inflammatory’ bacteria like Bacteroidetes and Verrucomicrobia. These changes can disrupt mucosal barrier function, trigger inflammation, and promote bacterial translocation (Capurso and Lahner, 2017).
Taken together, the impact of dietary patterns and lifestyle on the composition and metabolism of the intestinal microbiota is significant. Environmental factors such as diet and lifestyle choices may mask differences in gut microbial composition related to sex hormone status. This makes it challenging to pinpoint the correlation between individual dietary habits, lifestyle choices, and sexual dimorphism in the intestinal microbiota. Moreover, these results suggest that high-sugar, high-fat, and low-fiber diets, along with lifestyle habits like smoking and alcohol consumption, are risk factors for CRC. These findings offer valuable insights for the prevention of this disease.
In conclusion, sex hormones shape the composition and diversity of the gut microbiota, which reciprocally influences sex hormone levels. This bidirectional communication is crucial for understanding sex disparities in CRC. Both sex hormones and the intestinal microbiota are directly important for CRC cells as they contribute to genetic and epigenetic alterations. Moreover, they indirectly influence CRC onset, progression, and therapeutic responses by modulating inflammation and the immune microenvironment. For instance, estrogen restrains CRC cell proliferation by enhancing DNA repair, miRNA regulation, and ion channel modulation. In the absence of ERβ, estradiol activates non-genomic signaling via GPER, augmenting oncogene expression. Conversely, androgens promote epigenetic dysregulation and CRC advancement through nuclear receptor activation while inducing apoptosis via membrane receptors. Dysbiosis of the gut microbiota can result in the production of genotoxins and oncogenic metabolites by certain microbes, directly damaging colonic cell DNA and promoting tumorigenesis. Regarding the TME, estrogen fosters a favorable gut microecology by enhancing microbiome diversity and commensal bacteria abundance while suppressing pathogen levels, thereby mitigating intestinal inflammation and reversing immunosuppression. Conversely, androgens may disrupt intestinal microecology, diminish αPD-L1 efficacy, and exacerbate colonic inflammation and tumor growth. It is noteworthy that diet and lifestyle choices significantly shape gut microbiome composition, potentially masking differences due to sex hormone status. These findings suggest that targeting sex hormone receptors could be a promising strategy for intervening in CRC, with the intestinal microbiota serving as a potential biomarker or prognostic indicator for CRC. Estradiol and specific probiotics may enhance αPD-L1 efficacy. Additionally, considering sex-related differences in the intestinal microbiota when using antibiotics to manage ICIs-associated colitis is crucial. Overall, these insights offer valuable guidance for leveraging hormone replacement therapy, immunotherapy, and fecal microbiota transplantation in CRC management.
However, the direct contribution of interactions between sex hormone signaling and the intestinal microbiota to sex differences in CRC lacks sufficient evidence. Existing studies often focus on strain differences related to sex hormones or sex, without elucidating the specific molecular mechanisms through which these interactions lead to inflammation and immunosuppression. Future research should prioritize investigating how the interplay between sex hormones and the intestinal microbiota affects CRC genetics and epigenetics and delve into the mechanisms by which they influence the tumor microenvironment. Epidemiological evidence on the impact of the intestinal microbiota on CRC risk in men and women primarily derives from retrospective studies on patients already diagnosed with CRC. It remains unclear whether the observed gut microbial changes are a cause or a consequence of CRC development. Prospective studies that collect detailed gut microbiome data before individuals develop CRC are essential for better understanding the long-term effects of environmental factors (i.e., diet and lifestyle) on the intestinal microbiota and their implications for CRC prevention in both sexes. Human data on the role of gut microbiome alterations in CRC treatment are limited. The effects of the intestinal microbiota on laboratory mice may differ significantly from those in humans, with mice possessing a natural wild microbiota being more resilient to environmental challenges and responding to immunotherapy in a manner closer to humans. Overall, to develop sex hormone- and gut microbiome-based therapies, a deeper comprehension of the complex interactions between sex hormones, the intestinal microbiota, and sexual dimorphism in CRC is necessary. The successful translation of these therapies into clinical practice urges standardized, rigorous preclinical and clinical intervention investigations.
ZW: Data curation, Formal analysis, Methodology, Project administration, Writing – original draft, Writing – review & editing, Software. YS: Data curation, Writing – review & editing. WH: Writing – review & editing, Software. ZJ: Methodology, Writing – review & editing. FY: Conceptualization, Funding acquisition, Project administration, Writing – review & editing. XL: Conceptualization, Funding acquisition, Methodology, Resources, Supervision, Validation, Writing – review & editing. CX: Conceptualization, Funding acquisition, Investigation, Project administration, Resources, Visualization, Writing – review & editing, Writing – original draft.
The author(s) declare financial support was received for the research, authorship, and/or publication of this article. This research was supported by the Natural Science Foundation of Sichuan, grant number 2023NSFSC1830, and the Hospital of Chengdu University of Traditional Chinese Medicine Science and Technology Development Fund, grant number 23LC04.
The authors declare that the research was conducted in the absence of any commercial or financial relationships that could be construed as a potential conflict of interest.
All claims expressed in this article are solely those of the authors and do not necessarily represent those of their affiliated organizations, or those of the publisher, the editors and the reviewers. Any product that may be evaluated in this article, or claim that may be made by its manufacturer, is not guaranteed or endorsed by the publisher.
AOM/DSS, azoxymethane/dextran sodium sulfate; ASNS, asparagine synthetase; ATM, Ataxia telangiectasia mutated; CAC, colitis-associated cancer; CAFs, cancer-associated fibroblasts; CAG, cytosine-adenine-guanine; CDT, cytolethal distending toxin; CRC, colorectal cancer; CTGF, connective tissue growth factor; ERβ, estrogen receptor-beta; E2, estradiol/17β-estradiol; E1S, estrone sulfate; E2S, estradiol sulfate; EVs, extracellular vesicles; F/B, Firmicutes/Bacteroidetes; GPER, G protein-coupled estrogen receptor; HFD, high-fat diet; HSD, hydroxysteroid dehydrogenase; IAP, intestinal alkaline phosphatase; iARs, intracellular androgen receptors; ICIs, immune checkpoint inhibitors; MDSCs, myeloid-derived suppressor cells; MMR, mismatch repair gene; mARs, membrane androgen receptors; ORX, orchiectomized; OVX, ovariectomized; PD-L1, programmed death-ligand 1; ROS, reactive oxygen species; SCFAs, short chain fatty acids; STS, steroid sulfatase; TAMs, tumor-associated macrophages; TIME, tumor immune microenvironment; TME, tumor microenvironment; VDR, vitamin D receptor.
Abancens, M., Bustos, V., Harvey, H., McBryan, J., Harvey, B. J. (2020). Sexual dimorphism in colon cancer. Front. Oncol. 10. doi: 10.3389/fonc.2020.607909
Abancens, M., Harvey, B. J., McBryan, J. (2022). GPER agonist G1 prevents wnt-induced JUN upregulation in HT29 colorectal cancer cells. Int. J. Mol. Sci. 23, 12581. doi: 10.3390/ijms232012581
Acconcia, F., Fiocchetti, M., Marino, M. (2017). Xenoestrogen regulation of ERalpha/ERbeta balance in hormone-associated cancers. Mol. Cell Endocrinol. 457, 3–12. doi: 10.1016/j.mce.2016.10.033
Anbar, H. S., Isa, Z., Elounais, J. J., Jameel, M. A., Zib, J. H., Samer, A. M., et al. (2021). Steroid sulfatase inhibitors: the current landscape. Expert Opin. Ther. Patents 31, 453–472. doi: 10.1080/13543776.2021.1910237
Anderson, K. J., Cormier, R. T., Scott, P. M. (2019). Role of ion channels in gastrointestinal cancer. World J. Gastroenterol. 25, 5732–5772. doi: 10.3748/wjg.v25.i38.5732
Armstrong, C. M., Allred, K. F., Weeks, B. R., Chapkin, R. S., Allred, C. D. (2017). Estradiol has differential effects on acute colonic inflammation in the presence and absence of estrogen receptor beta expression. Dig Dis. Sci. 62, 1977–1984. doi: 10.1007/s10620-017-4631-x
Arterburn, J. B., Prossnitz, E. R. (2023). G protein-coupled estrogen receptor GPER: molecular pharmacology and therapeutic applications. Annu. Rev. Pharmacol. Toxicol. 63, 295–320. doi: 10.1146/annurev-pharmtox-031122-121944
Avuthu, N., Guda, C. (2022). Meta-analysis of altered gut microbiota reveals microbial and metabolic biomarkers for colorectal cancer. Microbiol. Spectr. 10 (4), e0001322. doi: 10.1128/spectrum.00013-22
Baraibar, I., Ros, J., Saoudi, N., Salva, F., Garcia, A., Castells, M. R., et al. (2023). Sex and gender perspectives in colorectal cancer. ESMO Open 8, 101204. doi: 10.1016/j.esmoop.2023.101204
Barzi, A., Lenz, A. M., Labonte, M. J., Lenz, H. J. (2013). Molecular pathways: Estrogen pathway in colorectal cancer. Clin. Cancer Res. 19, 5842–5848. doi: 10.1158/1078-0432.CCR-13-0325
Bennink, H. J. T. C., van Gennip, F. A. M., Gerrits, M. G. F., Egberts, J. F. M., Gemzell-Danielsson, K., Kopp-Kallner, H. (2024). Health benefits of combined oral contraceptives - a narrative review. Eur. J. Contracept Reprod. Health Care 29, 40–52. doi: 10.1080/13625187.2024.2317295
Bishehsari, F., Engen, P. A., Voigt, R. M., Swanson, G., Shaikh, M., Wilber, S., et al. (2020). Abnormal eating patterns cause circadian disruption and promote alcohol-associated colon carcinogenesis. Cell. Mol. Gastroenterol. Hepatol. 9, 219–237. doi: 10.1016/j.jcmgh.2019.10.011
Bosetti, C., Bravi, F., Negri, E., La Vecchia, C. (2009). Oral contraceptives and colorectal cancer risk: a systematic review and meta-analysis. Hum. Reprod. Update 15, 489–498. doi: 10.1093/humupd/dmp017
Brennan, C. A., Garrett, W. S. (2016). Gut microbiota, inflammation, and colorectal cancer. Annu. Rev. Microbiol. 70, 395–411. doi: 10.1146/annurev-micro-102215-095513
Buhler, M., Fahrlander, J., Sauter, A., Becker, M., Wistorf, E., Steinfath, M., et al. (2023). GPER1 links estrogens to centrosome amplification and chromosomal instability in human colon cells. Life Sci. Alliance 6, e202201499. doi: 10.26508/lsa.202201499
Bustos, V., Nolan, Á.M., Nijhuis, A., Harvey, H., Parker, A., Poulsom, R., et al. (2017). GPER mediates differential effects of estrogen on colon cancer cell proliferation and migration under normoxic and hypoxic conditions. Oncotarget 8, 84258–84275. doi: 10.18632/oncotarget.20653
Calcaterra, V., Rossi, V., Massini, G., Regalbuto, C., Hruby, C., Panelli, S., et al. (2022). Precocious puberty and microbiota: The role of the sex hormone-gut microbiome axis. Front. Endocrinol. 13. doi: 10.3389/fendo.2022.1000919
Capurso, G., Lahner, E. (2017). The interaction between smoking, alcohol and the gut microbiome. Best Pract. Res. Clin. Gastroenterol. 31, 579–588. doi: 10.1016/j.bpg.2017.10.006
Caruso, R., Mathes, T., Martens, E. C., Kamada, N., Nusrat, A., Inohara, N., et al. (2019). A specific gene-microbe interaction drives the development of Crohn’s disease–like colitis in mice. Sci. Immunol. 4, eaaw4341. doi: 10.1126/sciimmunol.aaw4341
Castellarin, M., Warren, R. L., Freeman, J. D., Dreolini, L., Krzywinski, M., Strauss, J., et al. (2012). Fusobacterium nucleatum infection is prevalent in human colorectal carcinoma. Genome Res. 22, 299–306. doi: 10.1101/gr.126516.111
Catalano, M. G., Pfeffer, U., Raineri, M., Ferro, P., Curto, A., Capuzzi, P., et al. (2000). Altered expression of androgen-receptor isoforms in human colon-cancer tissues. Int. J. Cancer 86, 325–330. doi: 10.1002/(sici)1097-0215(20000501)86:3<325::Aid-ijc4>3.0.Co;2-g
Chang, Y.-M., Kang, Y.-R., Lee, Y.-G., Sung, M.-K. (2024). Sex differences in colonic gene expression and fecal microbiota composition in a mouse model of obesity-associated colorectal cancer. Sci. Rep. 14, 3576. doi: 10.1038/s41598-024-53861-z
Chen, M., Lin, W., Li, N., Wang, Q., Zhu, S., Zeng, A., et al. (2022a). Therapeutic approaches to colorectal cancer via strategies based on modulation of gut microbiota. Front. Microbiol. 13. doi: 10.3389/fmicb.2022.945533
Chen, Q., Peng, K., Mo, P., Yu, C. (2022b). Histone demethylase JMJD2D: A novel player in colorectal and hepatocellular cancers. Cancers 14, 2841. doi: 10.3390/cancers14122841
Cheng, L., Jin, H., Qiang, Y., Wu, S., Yan, C., Han, M., et al. (2016). High fat diet exacerbates dextran sulfate sodium induced colitis through disturbing mucosal dendritic cell homeostasis. Int. Immunopharmacol. 40, 1–10. doi: 10.1016/j.intimp.2016.08.018
Collden, H., Landin, A., Wallenius, V., Elebring, E., Fandriks, L., Nilsson, M. E., et al. (2019). The gut microbiota is a major regulator of androgen metabolism in intestinal contents. Am. J. Physiol. Endocrinol. Metab. 317, E1182–E1192. doi: 10.1152/ajpendo.00338.2019
Cross, T. L., Simpson, A. M. R., Lin, C. Y., Hottmann, N. M., Bhatt, A. P., Pellock, S. J., et al. (2024). Gut microbiome responds to alteration in female sex hormone status and exacerbates metabolic dysfunction. Gut Microbes 16, 2295429. doi: 10.1080/19490976.2023.2295429
Dalile, B., Oudenhove, L. V., Vervliet, B., Verbeke, K. (2019). The role of short-chain fatty acids in microbiota-gut-brain communication. Nat. Rev. Gastroenterol. Hepatol. 16, 461–478. doi: 10.1038/s41575-019-0157-3
Dammeijer, F., van Gulijk, M., Mulder, E. E., Lukkes, M., Klaase, L., van den Bosch, T., et al. (2020). The PD-1/PD-L1-checkpoint restrains T cell immunity in tumor-draining lymph nodes. Cancer Cell 38, 685–700 e688. doi: 10.1016/j.ccell.2020.09.001
Dashti, S. G., Viallon, V., Simpson, J. A., Karahalios, A., Moreno-Betancur, M., English, D. R., et al. (2020). Explaining the link between adiposity and colorectal cancer risk in men and postmenopausal women in the UK Biobank: A sequential causal mediation analysis. Int. J. Cancer 147, 1881–1894. doi: 10.1002/ijc.32980
del Castillo-Izquierdo, Á., Mayneris-Perxachs, J., Fernández-Real, J. M. (2022). Bidirectional relationships between the gut microbiome and sexual traits. Am. J. Physiology-Cell Physiol. 322, C1223–C1229. doi: 10.1152/ajpcell.00116.2022
Devkota, S., Wang, Y., Musch, M. W., Leone, V., Fehlner-Peach, H., Nadimpalli, A., et al. (2012). Dietary-fat-induced taurocholic acid promotes pathobiont expansion and colitis in Il10–/– mice. Nature 487, 104–108. doi: 10.1038/nature11225
Domínguez-López, I., Yago-Aragón, M., Salas-Huetos, A., Tresserra-Rimbau, A., Hurtado-Barroso, S. (2020). Effects of dietary phytoestrogens on hormones throughout a human lifespan: A review. Nutrients 12, 2456. doi: 10.3390/nu12082456
Fan, X., Jin, Y., Chen, G., Ma, X., Zhang, L. (2021). Gut microbiota dysbiosis drives the development of colorectal cancer. Digestion 102, 508–515. doi: 10.1159/000508328
Fiocchetti, M., Camilli, G., Acconcia, F., Leone, S., Ascenzi, P., Marino, M. (2015). ERbeta-dependent neuroglobin up-regulation impairs 17beta-estradiol-induced apoptosis in DLD-1 colon cancer cells upon oxidative stress injury. J. Steroid Biochem. Mol. Biol. 149, 128–137. doi: 10.1016/j.jsbmb.2015.02.005
Fong, W., Li, Q., Yu, J. (2020). Gut microbiota modulation: a novel strategy for prevention and treatment of colorectal cancer. Oncogene 39, 4925–4943. doi: 10.1038/s41388-020-1341-1
Genazzani, A. R., Monteleone, P., Giannini, A., Simoncini, T. (2021). Hormone therapy in the postmenopausal years: considering benefits and risks in clinical practice. Hum. Reprod. Update 27, 1115–1150. doi: 10.1093/humupd/dmab026
Geng, J., Fan, H., Tang, X., Zhai, H., Zhang, Z. (2013). Diversified pattern of the human colorectal cancer microbiome. Gut Pathog. 5, 2. doi: 10.1186/1757-4749-5-2
Gevers, D., Kugathasan, S., Denson, L. A., Vázquez-Baeza, Y., Van Treuren, W., Ren, B., et al. (2014). The treatment-naive microbiome in new-onset crohn’s disease. Cell Host Microbe 15, 382–392. doi: 10.1016/j.chom.2014.02.005
Gilligan, L. C., Rahman, H. P., Hewitt, A. M., Sitch, A. J., Gondal, A., Arvaniti, A., et al. (2017). Estrogen activation by steroid sulfatase increases colorectal cancer proliferation via GPER. J. Clin. Endocrinol. Metab. 102, 4435–4447. doi: 10.1210/jc.2016-3716
Girardi, B., Principi, M., Pricci, M., Giorgio, F., Iannone, A., Losurdo, G., et al. (2018). Chemoprevention of inflammation-related colorectal cancer by silymarin-, acetyl-11-keto-beta-boswellic acid-, curcumin- and maltodextrin-enriched dietetic formulation in animal model. Carcinogenesis 39, 1274–1282. doi: 10.1093/carcin/bgy104
Gu, S., Kounenidakis, M., Schmidt, E.-M., Deshpande, D., Alkahtani, S., Alarifi, S., et al. (2013). Rapid activation of FAK/mTOR/p70S6K/PAK1-signaling controls the early testosterone-induced actin reorganization in colon cancer cells. Cell. Signalling 25, 66–73. doi: 10.1016/j.cellsig.2012.08.005
Gu, S., Papadopoulou, N., Gehring, E.-M., Nasir, O., Dimas, K., Bhavsar, S. K., et al. (2009). Functional membrane androgen receptors in colon tumors trigger pro-apoptotic responses in vitro and reduce drastically tumor incidence in vivo. Mol. Cancer 8, 114. doi: 10.1186/1476-4598-8-114
Gu, S., Papadopoulou, N., Nasir, O., Föller, M., Alevizopoulos, K., Lang, F., et al. (2010). Activation of membrane androgen receptors in colon cancer inhibits the prosurvival signals akt/bad in vitro and in vivo and blocks migration via vinculin/actin signaling. Mol. Med. 17, 48–58. doi: 10.2119/molmed.2010.00120
Gur, C., Ibrahim, Y., Isaacson, B., Yamin, R., Abed, J., Gamliel, M., et al. (2015). Binding of the fap2 protein of fusobacterium nucleatum to human inhibitory receptor TIGIT protects tumors from immune cell attack. Immunity 42, 344–355. doi: 10.1016/j.immuni.2015.01.010
Hao, Y. A., Feng, Y. N., Yan, X. W., Chen, L., Zhong, R. Q., Tang, X. F., et al. (2022). Gut microbiota-testis axis: FMT improves systemic and testicular micro-environment to increase semen quality in type 1 diabetes. Mol. Med. 28, 45. doi: 10.1186/s10020-022-00473-w
Harada, N., Minami, Y., Hanada, K., Hanaoka, R., Kobayashi, Y., Izawa, T., et al. (2020). Relationship between gut environment, feces-to-food ratio, and androgen deficiency-induced metabolic disorders. Gut Microbes 12, 1817719. doi: 10.1080/19490976.2020.1817719
Hases, L., Archer, A., Indukuri, R., Birgersson, M., Savva, C., Korach-Andre, M., et al. (2020). High-fat diet and estrogen impacts the colon and its transcriptome in a sex-dependent manner. Sci. Rep. 10, 16160. doi: 10.1038/s41598-020-73166-1
Hauger, R. L., Saelzler, U. G., Pagadala, M. S., Panizzon, M. S. (2022). The role of testosterone, the androgen receptor, and hypothalamic-pituitary-gonadal axis in depression in ageing Men. Rev. Endocrine Metab. Disord. 23, 1259–1273. doi: 10.1007/s11154-022-09767-0
Haziman, A. A., Ravinderan, S., Thangavelu, T., Thomas, W. (2019). A novel role for estrogen-induced signaling in the colorectal cancer gender bias. Irish J. Med. Sci. 188, 389–395. doi: 10.1007/s11845-018-1867-1
He, Y. Q., Sheng, J. Q., Ling, X. L., Fu, L., Jin, P., Yen, L., et al. (2012). Estradiol regulates miR-135b and mismatch repair gene expressions via estrogen receptor-beta in colorectal cells. Exp. Mol. Med. 44, 723–732. doi: 10.3858/emm.2012.44.12.079
Hickey, M., Basu, P., Sassarini, J., Stegmann, M. E., Weiderpass, E., Nakawala Chilowa, K., et al. (2024). Managing menopause after cancer. Lancet 403, 984–996. doi: 10.1016/s0140-6736(23)02802-7
Hirko, K. A., Spiegelman, D., Barnett, J. B., Cho, E., Willett, W. C., Hankinson, S. E., et al. (2016). Dietary patterns and plasma sex hormones, prolactin, and sex hormone–binding globulin in premenopausal women. Cancer Epidemiol. Biomarkers Prev. 25, 791–798. doi: 10.1158/1055-9965.Epi-15-1019
Hokanson, K. C., Hernández, C., Deitzler, G. E., Gaston, J. E., David, M. M. (2024). Sex shapes gut–microbiota–brain communication and disease. Trends Microbiol. 32, 151–161. doi: 10.1016/j.tim.2023.08.013
Honma, N., Arai, T., Matsuda, Y., Fukunaga, Y., Muramatsu, M., Ikeda, S., et al. (2023). Estrogen receptor-beta gene cytosine-adenine (ESR2-CA) repeat polymorphism in postmenopausal colon cancer. Int. J. Mol. Sci. 24, 4502. doi: 10.3390/ijms24054502
Hu, S., Ding, Q., Zhang, W., Kang, M., Ma, J., Zhao, L. (2023). Gut microbial beta-glucuronidase: a vital regulator in female estrogen metabolism. Gut Microbes 15, 2236749. doi: 10.1080/19490976.2023.2236749
Huang, C., Deng, W., Xu, H. Z., Zhou, C., Zhang, F., Chen, J., et al. (2023). Short-chain fatty acids reprogram metabolic profiles with the induction of reactive oxygen species production in human colorectal adenocarcinoma cells. Comput. Struct. Biotechnol. J. 21, 1606–1620. doi: 10.1016/j.csbj.2023.02.022
Ibrahim, A., Hugerth, L. W., Hases, L., Saxena, A., Seifert, M., Thomas, Q., et al. (2019). Colitis-induced colorectal cancer and intestinal epithelial estrogen receptor beta impact gut microbiota diversity. Int. J. Cancer 144, 3086–3098. doi: 10.1002/ijc.32037
Janjuha, R., Bunn, D., Hayhoe, R., Hooper, L., Abdelhamid, A., Mahmood, S., et al. (2020). Effects of dietary or supplementary micronutrients on sex hormones and IGF-1 in middle and older age: A systematic review and meta-analysis. Nutrients 12, 1457. doi: 10.3390/nu12051457
Jiang, L., Fei, H., Yang, A., Zhu, J., Sun, J., Liu, X., et al. (2021). Estrogen inhibits the growth of colon cancer in mice through reversing extracellular vesicle-mediated immunosuppressive tumor microenvironment. Cancer Lett. 520, 332–343. doi: 10.1016/j.canlet.2021.08.011
Jin, P., Lu, X. J., Sheng, J. Q., Fu, L., Meng, X. M., Wang, X., et al. (2010). Estrogen stimulates the expression of mismatch repair gene hMLH1 in colonic epithelial cells. Cancer Prev. Res. (Phila) 3, 910–916. doi: 10.1158/1940-6207.CAPR-09-0228
Jing, N., Wang, L., Zhuang, H., Ai, C., Jiang, G., Liu, Z. (2022). Sex-biased immune responses to antibiotics during anti-PD-L1 treatment in mice with colon cancer. J. Immunol. Res. 2022, 9202491. doi: 10.1155/2022/9202491
Kaliannan, K., Robertson, R. C., Murphy, K., Stanton, C., Kang, C., Wang, B., et al. (2018). Estrogen-mediated gut microbiome alterations influence sexual dimorphism in metabolic syndrome in mice. Microbiome 6, 205. doi: 10.1186/s40168-018-0587-0
Kang, C., Song, C. H., Kim, N., Nam, R. H., Choi, S. I., Yu, J. E., et al. (2021). The enhanced inhibitory effect of estrogen on PD-L1 expression following nrf2 deficiency in the AOM/DSS model of colitis-associated cancer. Front. Oncol. 11. doi: 10.3389/fonc.2021.679324
Khan, M. Z. I., Uzair, M., Nazli, A., Chen, J. Z. (2022). An overview on Estrogen receptors signaling and its ligands in breast cancer. Eur. J. Med. Chem. 241, 114658. doi: 10.1016/j.ejmech.2022.114658
Koh, A., De Vadder, F., Kovatcheva-Datchary, P., Backhed, F. (2016). From dietary fiber to host physiology: short-chain fatty acids as key bacterial metabolites. Cell 165, 1332–1345. doi: 10.1016/j.cell.2016.05.041
Koyande, N., Gangopadhyay, M., Thatikonda, S., Rengan, A. K. (2022). The role of gut microbiota in the development of colorectal cancer: a review. Int. J. Colorectal Dis. 37, 1509–1523. doi: 10.1007/s00384-022-04192-w
Kumari, N., Kumari, R., Dua, A., Singh, M., Kumar, R., Singh, P., et al. (2024). From gut to hormones: unraveling the role of gut microbiota in (Phyto)Estrogen modulation in health and disease. Mol. Nutr. Food Res. 68, e2300688. doi: 10.1002/mnfr.202300688
Kwa, M., Plottel, C. S., Blaser, M. J., Adams, S. (2016). The intestinal microbiome and estrogen receptor-positive female breast cancer. J. Natl. Cancer Inst 108, djw029. doi: 10.1093/jnci/djw029
Lee, J. G., Eun, C. S., Jo, S. V., Lee, A. R., Park, C. H., Han, D. S. (2019). The impact of gut microbiota manipulation with antibiotics on colon tumorigenesis in a murine model. PloS One 14, e0226907. doi: 10.1371/journal.pone.0226907
Levi, F. A., Okyar, A., Hadadi, E., Innominato, P. F., Ballesta, A. (2024). Circadian regulation of drug responses: toward sex-specific and personalized chronotherapy. Annu. Rev. Pharmacol. Toxicol. 64, 89–114. doi: 10.1146/annurev-pharmtox-051920-095416
Li, Q., Chan, H., Liu, W. X., Liu, C. A., Zhou, Y., Huang, D., et al. (2023). Carnobacterium Maltaromaticum boosts intestinal vitamin D production to suppress colorectal cancer in female mice. Cancer Cell 41, 1450–1465 e1458. doi: 10.1016/j.ccell.2023.06.011
Li, D., Liu, R., Wang, M., Peng, R., Fu, S., Fu, A., et al. (2022). 3β-Hydroxysteroid dehydrogenase expressed by gut microbes degrades testosterone and is linked to depression in males. Cell Host Microbe 30, 329–339.e325. doi: 10.1016/j.chom.2022.01.001
Lin, C., Cai, X., Zhang, J., Wang, W., Sheng, Q., Hua, H., et al. (2019). Role of gut microbiota in the development and treatment of colorectal cancer. Digestion 100, 72–78. doi: 10.1159/000494052
Liu, L., Zhang, M., Jiang, F., Luo, D., Liu, S., Su, Y., et al. (2023). High cholesterol diet–induced testicular dysfunction in rats. Hormones 22, 685–694. doi: 10.1007/s42000-023-00472-4
Lizardo, D. Y., Kuang, C., Hao, S., Yu, J., Huang, Y., Zhang, L. (2020). Immunotherapy efficacy on mismatch repair-deficient colorectal cancer: From bench to bedside. Biochim. Biophys. Acta (BBA) - Rev. Cancer 1874, 188447. doi: 10.1016/j.bbcan.2020.188447
Lu, J., Jin, P., Gao, W., Wang, D., Sheng, J. (2017). Estrogen enhances mismatch repair by induction of MLH1 expression via estrogen receptor-β. Oncotarget 8, 38767–38779. doi: 10.18632/oncotarget.16351
Lu, L., Zhang, Q., Shen, X., Zhen, P., Marin, A., Garcia-Milian, R., et al. (2023). Asparagine synthetase and G-protein coupled estrogen receptor are critical responders to nutrient supply in KRAS mutant colorectal cancer. bioRxiv. 05, 539577. doi: 10.1101/2023.05.05.539577
Ma, Z. S., Li, W. (2019). How and why men and women differ in their microbiomes: medical ecology and network analyses of the microgenderome. Adv. Sci. (Weinh) 6, 1902054. doi: 10.1002/advs.201902054
Ma, Y., Liu, T., Li, X., Kong, A., Xiao, R., Xie, R., et al. (2022). Estrogen receptor beta deficiency impairs gut microbiota: a possible mechanism of IBD-induced anxiety-like behavior. Microbiome 10, 160. doi: 10.1186/s40168-022-01356-2
Magne, F., Gotteland, M., Gauthier, L., Zazueta, A., Pesoa, S., Navarrete, P., et al. (2020). The firmicutes/bacteroidetes ratio: A relevant marker of gut dysbiosis in obese patients? Nutrients 12, 1474. doi: 10.3390/nu12051474
Mahbub, A. A. (2022). 17beta-estradiol enhances 5-fluorouracil anti-cancer activities in colon cancer cell lines. Med. Sci. (Basel) 10, 62. doi: 10.3390/medsci10040062
Mahbub, A. A., Aslam, A., Elzubier, M. E., El-Boshy, M., Abdelghany, A. H., Ahmad, J., et al. (2022). Enhanced anti-cancer effects of oestrogen and progesterone co-therapy against colorectal cancer in males. Front. Endocrinol. (Lausanne) 13. doi: 10.3389/fendo.2022.941834
Manor, O., Dai, C. L., Kornilov, S. A., Smith, B., Price, N. D., Lovejoy, J. C., et al. (2020). Health and disease markers correlate with gut microbiome composition across thousands of people. Nat. Commun. 11, 5206. doi: 10.1038/s41467-020-18871-1
Manson, J. E., Crandall, C. J., Rossouw, J. E., Chlebowski, R. T., Anderson, G. L., Stefanick, M. L., et al. (2024). The women’s health initiative randomized trials and clinical practice. Jama 331, 1748–1760. doi: 10.1001/jama.2024.6542
Matarrese, P., Mattia, G., Pagano, M. T., Pontecorvi, G., Ortona, E., Malorni, W., et al. (2021). The sex-related interplay between TME and cancer: on the critical role of estrogen, microRNAs and autophagy. Cancers (Basel) 13, 3287. doi: 10.3390/cancers13133287
Mauvais-Jarvis, F., Clegg, D. J., Hevener, A. L. (2013). The role of estrogens in control of energy balance and glucose homeostasis. Endocr. Rev. 34, 309–338. doi: 10.1210/er.2012-1055
Mima, K., Sukawa, Y., Nishihara, R., Qian, Z. R., Yamauchi, M., Inamura, K., et al. (2015). Fusobacterium nucleatumand T cells in colorectal carcinoma. JAMA Oncol. 1, 653–661. doi: 10.1001/jamaoncol.2015.1377
Moravcik, R., Olejarova, S., Zlacka, J., Herichova, I. (2023). Effect of miR-34a on the expression of clock and clock-controlled genes in DLD1 and Lovo human cancer cells with different backgrounds with respect to p53 functionality and 17beta-estradiol-mediated regulation. PloS One 18, e0292880. doi: 10.1371/journal.pone.0292880
Murphy, N., Strickler, H. D., Stanczyk, F. Z., Xue, X., Wassertheil-Smoller, S., Rohan, T. E., et al. (2015). A prospective evaluation of endogenous sex hormone levels and colorectal cancer risk in postmenopausal women. J. Natl. Cancer Inst 107, djv210. doi: 10.1093/jnci/djv210
Norman, A. W., Mizwicki, M. T., Norman, D. P. G. (2004). Steroid-hormone rapid actions, membrane receptors and a conformational ensemble model. Nat. Rev. Drug Discovery 3, 27–41. doi: 10.1038/nrd1283
O’Keefe, S. J. D. (2016). Diet, microorganisms and their metabolites, and colon cancer. Nat. Rev. Gastroenterol. Hepatol. 13, 691–706. doi: 10.1038/nrgastro.2016.165
Org, E., Mehrabian, M., Parks, B. W., Shipkova, P., Liu, X., Drake, T. A., et al. (2016). Sex differences and hormonal effects on gut microbiota composition in mice. Gut Microbes 7, 313–322. doi: 10.1080/19490976.2016.1203502
Parada Venegas, D., de la Fuente, M. K., Landskron, G., Gonzalez, M. J., Quera, R., Dijkstra, G., et al. (2019). Short chain fatty acids (SCFAs)-mediated gut epithelial and immune regulation and its relevance for inflammatory bowel diseases. Front. Immunol. 10. doi: 10.3389/fimmu.2019.00277
Qu, R., Zhang, Y., Ma, Y., Zhou, X., Sun, L., Jiang, C., et al. (2023). Role of the gut microbiota and its metabolites in tumorigenesis or development of colorectal cancer. Adv. Sci. (Weinh) 10, e2205563. doi: 10.1002/advs.202205563
Raskov, H., Burcharth, J., Pommergaard, H. C. (2017). Linking gut microbiota to colorectal cancer. J. Cancer 8, 3378–3395. doi: 10.7150/jca.20497
Rizzetto, L., Fava, F., Tuohy, K. M., Selmi, C. (2018). Connecting the immune system, systemic chronic inflammation and the gut microbiome: The role of sex. J. Autoimmun 92, 12–34. doi: 10.1016/j.jaut.2018.05.008
Roshan, M. H. K., Tambo, A., Pace, N. P. (2016). The role of testosterone in colorectal carcinoma: pathomechanisms and open questions. EPMA J. 7, 22. doi: 10.1186/s13167-016-0071-5
Rossouw, J. E., Anderson, G. L., Prentice, R. L., LaCroix, A. Z., Kooperberg, C., Stefanick, M. L., et al. (2002). Risks and benefits of estrogen plus progestin in healthy postmenopausal women: principal results From the Women’s Health Initiative randomized controlled trial. JAMA 288, 321–333. doi: 10.1001/jama.288.3.321
Rubin, J. B., Lagas, J. S., Broestl, L., Sponagel, J., Rockwell, N., Rhee, G., et al. (2020). Sex differences in cancer mechanisms. Biol. Sex Dif. 11, 17. doi: 10.1186/s13293-020-00291-x
Salvador, A. C., Huda, M. N., Arends, D., Elsaadi, A. M., Gacasan, C. A., Brockmann, G. A., et al. (2023). Analysis of strain, sex, and diet-dependent modulation of gut microbiota reveals candidate keystone organisms driving microbial diversity in response to American and ketogenic diets. Microbiome 11, 220. doi: 10.1186/s40168-023-01588-w
Shobeiri, P., Kalantari, A., Teixeira, A. L., Rezaei, N. (2022). Shedding light on biological sex differences and microbiota-gut-brain axis: a comprehensive review of its roles in neuropsychiatric disorders. Biol. Sex Dif. 13, 12. doi: 10.1186/s13293-022-00422-6
Siegel, R. L., Giaquinto, A. N., Jemal, A. (2024). Cancer statistic. CA Cancer J. Clin. 74, 12–49. doi: 10.3322/caac.21820
Simoncini, T., Genazzani, A. R. (2003). Non-genomic actions of sex steroid hormones. Eur. J. Endocrinol. 148, 281–292. doi: 10.1530/eje.0.1480281
Sinha, T., Vila, A. V., Garmaeva, S., Jankipersadsing, S. A., Imhann, F., Collij, V., et al. (2019). Analysis of 1135 gut metagenomes identifies sex-specific resistome profiles. Gut Microbes 10, 358–366. doi: 10.1080/19490976.2018.1528822
So, S. Y., Savidge, T. C. (2021). Sex-bias in irritable bowel syndrome: linking steroids to the gut-brain axis. Front. Endocrinol. 12. doi: 10.3389/fendo.2021.684096
Son, H. J., Kim, N., Song, C. H., Lee, S. M., Lee, H. N., Surh, Y. J. (2020). 17beta-Estradiol reduces inflammation and modulates antioxidant enzymes in colonic epithelial cells. Korean J. Intern. Med. 35, 310–319. doi: 10.3904/kjim.2018.098
Son, H. J., Sohn, S. H., Kim, N., Lee, H. N., Lee, S. M., Nam, R. H., et al. (2019). Effect of estradiol in an azoxymethane/dextran sulfate sodium-treated mouse model of colorectal cancer: implication for sex difference in colorectal cancer development. Cancer Res. Treat 51, 632–648. doi: 10.4143/crt.2018.060
Song, M., Chan, A. T. (2019). Environmental factors, gut microbiota, and colorectal cancer prevention. Clin. Gastroenterol. Hepatol. 17, 275–289. doi: 10.1016/j.cgh.2018.07.012
Song, M., Chan, A. T., Sun, J. (2020c). Influence of the gut microbiome, diet, and environment on risk of colorectal cancer. Gastroenterology 158, 322–340. doi: 10.1053/j.gastro.2019.06.048
Song, C. H., Kim, N., Hee Nam, R., In Choi, S., Hee Son, J., Eun Yu, J., et al. (2020a). 17beta-Estradiol strongly inhibits azoxymethane/dextran sulfate sodium-induced colorectal cancer development in Nrf2 knockout male mice. Biochem. Pharmacol. 182, 114279. doi: 10.1016/j.bcp.2020.114279
Song, C. H., Kim, N., Lee, S. M., Nam, R. H., Choi, S. I., Kang, S. R., et al. (2019). Effects of 17beta-estradiol on colorectal cancer development after azoxymethane/dextran sulfate sodium treatment of ovariectomized mice. Biochem. Pharmacol. 164, 139–151. doi: 10.1016/j.bcp.2019.04.011
Song, C. H., Kim, N., Nam, R. H., Choi, S. I., Jang, J. Y., Choi, J., et al. (2023a). Anti-PD-L1 antibody and/or 17beta-estradiol treatment induces changes in the gut microbiome in MC38 colon tumor model. Cancer Res. Treat 55, 894–909. doi: 10.4143/crt.2022.1427
Song, C. H., Kim, N., Nam, R. H., Choi, S. I., Jang, J. Y., Kim, J. W., et al. (2022). Combination treatment with 17beta-estradiol and anti-PD-L1 suppresses MC38 tumor growth by reducing PD-L1 expression and enhancing M1 macrophage population in MC38 colon tumor model. Cancer Lett. 543, 215780. doi: 10.1016/j.canlet.2022.215780
Song, C. H., Kim, N., Nam, R. H., Choi, S. I., Jang, J. Y., Lee, H. N. (2023b). Changes in gut microbiome upon orchiectomy and testosterone administration in AOM/DSS-induced colon cancer mouse model. Cancer Res. Treat 55, 196–218. doi: 10.4143/crt.2022.080
Song, C. H., Kim, N., Nam, R. H., Choi, S. I., Lee, H. N., Surh, Y. J. (2020b). 17beta-Estradiol supplementation changes gut microbiota diversity in intact and colorectal cancer-induced ICR male mice. Sci. Rep. 10, 12283. doi: 10.1038/s41598-020-69112-w
Song, C.-H., Kim, N., Nam, R. H., Choi, S. I., Yu, J. E., Nho, H., et al. (2021a). Testosterone strongly enhances azoxymethane/dextran sulfate sodium-induced colorectal cancer development in C57BL/6 mice. Am. J. Cancer Res. 11, 3145–3162.
Song, C. H., Kim, N., Nam, R. H., Choi, S. I., Yu, J. E., Nho, H., et al. (2021b). Changes in microbial community composition related to sex and colon cancer by nrf2 knockout. Front. Cell Infect. Microbiol. 11. doi: 10.3389/fcimb.2021.636808
Song, H., Sontz, R. A., Vance, M. J., Morris, J. M., Sheriff, S., Zhu, S., et al. (2023c). High-fat diet plus HNF1A variant promotes polyps by activating β-catenin in early-onset colorectal cancer. JCI Insight 8, e167163. doi: 10.1172/jci.insight.167163
Sun, Q. F., Hu, Y. Z., Yu, C. Q., Guo, Y., Pei, P., Yang, L., et al. (2023). Healthy lifestyle and life expectancy free of major chronic diseases at age 40 in China. Nat. Hum. Behav. 7, 1542–1550. doi: 10.1038/s41562-023-01624-7
Sun, M., Wu, W., Chen, L., Yang, W., Huang, X., Ma, C., et al. (2018). Microbiota-derived short-chain fatty acids promote Th1 cell IL-10 production to maintain intestinal homeostasis. Nat. Commun. 9, 3555. doi: 10.1038/s41467-018-05901-2
Thackray, V. G. (2019). Sex, microbes, and polycystic ovary syndrome. Trends Endocrinol. Metab. 30, 54–65. doi: 10.1016/j.tem.2018.11.001
Thériault, J. F., Lin, S. X. (2019). The dual sex hormone specificity for human reductive 17β-hydroxysteroid dehydrogenase type 7: Synergistic function in estrogen and androgen control. J. Steroid Biochem. Mol. Biol. 186, 61–65. doi: 10.1016/j.jsbmb.2018.09.012
Thomas, M. P., Potter, B. V. (2013). The structural biology of oestrogen metabolism. J. Steroid Biochem. Mol. Biol. 137, 27–49. doi: 10.1016/j.jsbmb.2012.12.014
Usui, T., Tochiya, M., Sasaki, Y., Muranaka, K., Yamakage, H., Himeno, A., et al. (2013). Effects of natural S-equol supplements on overweight or obesity and metabolic syndrome in the Japanese, based on sex and equol status. Clin. Endocrinol. 78, 365–372. doi: 10.1111/j.1365-2265.2012.04400.x
Vemuri, R., Sylvia, K. E., Klein, S. L., Forster, S. C., Plebanski, M., Eri, R., et al. (2019). The microgenderome revealed: sex differences in bidirectional interactions between the microbiota, hormones, immunity and disease susceptibility. Semin. Immunopathol. 41, 265–275. doi: 10.1007/s00281-018-0716-7
Viennois, E., Merlin, D., Gewirtz, A. T., Chassaing, B. (2017). Dietary emulsifier–induced low-grade inflammation promotes colon carcinogenesis. Cancer Res. 77, 27–40. doi: 10.1158/0008-5472.Can-16-1359
Wallis, A., Butt, H., Ball, M., Lewis, D. P., Bruck, D. (2017). Support for the microgenderome invites enquiry into sex differences. Gut Microbes 8, 46–52. doi: 10.1080/19490976.2016.1256524
Wang, L., Jiang, G., Jing, N., Liu, X., Zhuang, H., Zeng, W., et al. (2021). Downregulating testosterone levels enhance immunotherapy efficiency. Oncoimmunology 10, 1981570. doi: 10.1080/2162402X.2021.1981570
Wang, L., Tu, Y. X., Chen, L., Zhang, Y., Pan, X. L., Yang, S. Q., et al. (2023). Male-biased gut microbiome and metabolites aggravate colorectal cancer development. Adv. Sci. (Weinh) 25, e2206238. doi: 10.1002/advs.202206238
Wilkenfeld, S. R., Lin, C., Frigo, D. E. (2018). Communication between genomic and non-genomic signaling events coordinate steroid hormone actions. Steroids 133, 2–7. doi: 10.1016/j.steroids.2017.11.005
Wong, C. C., Yu, J. (2023). Gut microbiota in colorectal cancer development and therapy. Nat. Rev. Clin. Oncol. 20, 429–452. doi: 10.1038/s41571-023-00766-x
Wu, Z., Ma, Q., Guo, Y., You, F. (2022). The role of fusobacterium nucleatum in colorectal cancer cell proliferation and migration. Cancers 14, 5350. doi: 10.3390/cancers14215350
Xia, T., Sun, H., Huang, H., Bi, H., Pu, R., Zhang, L., et al. (2019). Androgen receptor gene methylation related to colorectal cancer risk. Endocrine Connections 8, 979–987. doi: 10.1530/ec-19-0122
Yang, X., Li, P., Qu, Z., Zhuang, J., Wu, Y., Wu, W., et al. (2023). Gut bacteria and sex differences in colorectal cancer. J. Med. Microbiol. 72, 1706. doi: 10.1099/jmm.0.001706
Yang, J., Wei, H., Zhou, Y., Szeto, C.-H., Li, C., Lin, Y., et al. (2022). High-fat diet promotes colorectal tumorigenesis through modulating gut microbiota and metabolites. Gastroenterology 162, 135–149.e132. doi: 10.1053/j.gastro.2021.08.041
Zamir, A., Ben-Zeev, T., Hoffman, J. R. (2021). Manipulation of dietary intake on changes in circulating testosterone concentrations. Nutrients 13, 3375. doi: 10.3390/nu13103375
Zhang, F., Aschenbrenner, D., Yoo, J. Y., Zuo, T. (2022). The gut mycobiome in health, disease, and clinical applications in association with the gut bacterial microbiome assembly. Lancet Microbe 3, E969–E983. doi: 10.1016/S2666-5247(22)00203-8
Zhang, J., Yin, W., Li, P., Hu, C., Wang, L., Li, T., et al. (2019). Interaction between diet- and exercise-lifestyle and phthalates exposure on sex hormone levels. J. Hazardous Materials 369, 290–298. doi: 10.1016/j.jhazmat.2019.02.011
Zheng, D., Liwinski, T., Elinav, E. (2020). Interaction between microbiota and immunity in health and disease. Cell Res. 30, 492–506. doi: 10.1038/s41422-020-0332-7
Keywords: colorectal cancer, estrogens, androgens, intestinal microbiota, direct and indirect effects
Citation: Wu Z, Sun Y, Huang W, Jin Z, You F, Li X and Xiao C (2024) Direct and indirect effects of estrogens, androgens and intestinal microbiota on colorectal cancer. Front. Cell. Infect. Microbiol. 14:1458033. doi: 10.3389/fcimb.2024.1458033
Received: 01 July 2024; Accepted: 04 November 2024;
Published: 26 November 2024.
Edited by:
Shaobo Mo, Fudan University, ChinaReviewed by:
Giuseppe Losurdo, University of Bari Medical School, ItalyCopyright © 2024 Wu, Sun, Huang, Jin, You, Li and Xiao. This is an open-access article distributed under the terms of the Creative Commons Attribution License (CC BY). The use, distribution or reproduction in other forums is permitted, provided the original author(s) and the copyright owner(s) are credited and that the original publication in this journal is cited, in accordance with accepted academic practice. No use, distribution or reproduction is permitted which does not comply with these terms.
*Correspondence: Xueke Li, MjAxNzIwMjA0MDA0NkB3aHUuZWR1LmNu; Chong Xiao, eGlhb2Nob25nQHN0dS5jZHV0Y20uZWR1LmNu
Disclaimer: All claims expressed in this article are solely those of the authors and do not necessarily represent those of their affiliated organizations, or those of the publisher, the editors and the reviewers. Any product that may be evaluated in this article or claim that may be made by its manufacturer is not guaranteed or endorsed by the publisher.
Research integrity at Frontiers
Learn more about the work of our research integrity team to safeguard the quality of each article we publish.