- 1International Centre of Insect Physiology and Ecology (icipe), Nairobi, Kenya
- 2African Regional Postgraduate Program in Insect Science (ARPPIS), University of Ghana, Legon, Accra, Ghana
- 3Department of Zoology, The University of Bamenda, Bambili, Cameroon
- 4Unit of Environmental Sciences and Management, North-West University, Potchefstroom, South Africa
Fall armyworm (FAW), Spodoptera frugiperda is a generalist pest known to feed on more than 300 plant species, including major staple crops such as rice, maize and sorghum. Biological control of FAW using a combination of a major indigenous egg parasitoid Telenomus remus and entomopathogenic fungi was explored in this study. Metarhizium anisopliae strains (ICIPE 7, ICIPE 41, and ICIPE 78) and Beauveria bassiana ICIPE 621 which demonstrated effectiveness to combat the pest, were evaluated through direct and indirect fungal infection to assess their pathogenicity and virulence against T. remus adults, S. frugiperda eggs and their effects on T. remus parasitism rates. Metarhizium anisopliae ICIPE 7 and ICIPE 78 exhibited the highest virulence against T. remus adults with LT50 values >2 days. ICIPE 7 induced the highest T. remus mortality rate (81.40 ± 4.17%) following direct infection with dry conidia. Direct fungal infection also had a significant impact on parasitoid emergence, with the highest emergence rate recorded in the M. anisopliae ICIPE 7 treatment (42.50 ± 5.55%), compared to the control ± (83.25 ± 5.94%). In the indirect infection, the highest concentration of 1 x 109 conidia ml-1 of ICIPE 78 induced the highest mortality (100 ± 0.00%) of T. remus adults, and the highest mortality (51.25%) of FAW eggs, whereas the least FAW egg mortality (15.25%) was recorded in the lowest concentration 1 x 105 conidia ml-1 of ICIPE 41. The number of parasitoids that emerged and their sex ratios were not affected by the different fungal strain concentrations except in ICIPE 7 at high dose. This study showed that potential combination of both M. anisopliae and B. bassiana with T. remus parasitoid can effectively suppress FAW populations.
1 Introduction
Fall armyworm (FAW), Spodoptera frugiperda J.E. Smith (Lepidoptera: Noctuidae) is a highly polyphagous pest that preferentially affects several agricultural crops such as maize, sorghum, and rice (Day et al., 2017). The pest spread from its native regions in America over the past few years, and currently an invasive species in Africa and East Asia countries (Hruska, 2019). Within sub-Saharan Africa, the pest was initially reported in the West African countries of Nigeria, Togo, and Benin in 2016, but has since spread to virtually all other countries (Goergen et al., 2016). FAW populations in Africa consist of both corn strain and rice strain and were estimated to cause maize yield losses ranging from 8.3 to 20.6 million tons per year according to a study conducted in 12 African maize-producing countries (Prasanna et al., 2018). These significant losses highly impact the diet of many residents in Africa because maize is reported to account for almost half of all calories and proteins consumed in Eastern and Southern Africa (Macauley, 2015).
Several methods for controlling FAW have been proposed but most have had little impact, with the efficacy of some solutions being questionable due to their inadequate assessment (Harrison et al., 2019). Although FAW has been the subject of extensive scientific research in its native range in America, chemical control methods continue to dominate its management, while the adoption of biological control strategies remain limited globally (Wyckhuys et al., 2024). Since the advent of FAW in African countries, insecticides have been commonly used as an emergency management approach to halt pest distribution and minimize damage in maize fields. Some of the pesticides used in the control of S. frugiperda include extremely dangerous methomyl, methyl parathion, endosulfan, and lindane (Bateman et al., 2018). In some parts of the world, certain pesticides, such as endosulfan and lindane, have been banned (Roşca et al., 2023). Biological control agents, such as fungal parasitoids and biopesticides are promising and sustainable alternatives. Therefore, appropriate and effective control strategies should be implemented in order to be able to combat this pest (Abbas et al., 2022).
The development and application of entomopathogens as biocontrol agents have led to several successes and have been commercialized to suppress insect pests. Over 50 entomopathogenic fungi, viruses, nematodes, and bacteria are currently commercialized and utilized as microbial biopesticides (Morales-Reyes et al., 2018). In the quest to design more sustainable and ecofriendly pest control strategies, some key potent fungal-based biopesticides have been identified as effective in managing both FAW eggs and neonates (Akutse et al., 2019), as well as the adults (Akutse et al., 2020a). The investigators observed that Metarhizium anisopliae s.l., ICIPE 78, ICIPE 40 and ICIPE 20 were most effective than the other tested strains in decreasing egg hatchability, achieving reductions by 87, 83 and, 79.5%, respectively. Additionally, these fungal strains also caused significant mortality to the neonates, following treatment with fungus after emergence. Specifically, M. anisopliae s.l. strains, ICIPE 41 and ICIPE 7 were the most effective, with larval mortality rates of 96.49% and 93.66% respectively. ICIPE 78 and ICIPE 7 which have been already commercialized as Achieve® and Tickoff® against spider mites and ticks, respectively by Real IPM in partnership with International Centre of Insect Physiology and Ecology (icipe) (Akutse et al., 2020b), could therefore be used as a potential ovicidal biopesticide to suppress FAW population in Africa (Akutse et al., 2019). Another study also demonstrated that B. bassiana s.l. ICIPE 621 and M. anisopliae s.l. ICIPE 7 outperformed all the other strains by causing 100% mortality of the FAW adults (Akutse et al., 2020).
In addition, some parasitoids such as Cotesia icipe, Chelonus curvimaculatus, Telenomus remus, Charops ater, Palexorista zonata, Trichogramma chilonis and Coccygidium luteum were also locally were identified to be effective in managing FAW egg and larvae (Sisay et al., 2019). Therefore, assessing how these effective biopesticides interact with parasitoids is crucial for enhancing FAW control within an integrated pest management (IPM) framework in Africa. This study, therefore, focused on evaluating the non-target impacts of the identified potent commercial biopesticides (M. anisopliae ICIPE 7, ICIPE 78, ICIPE 41, and B. bassiana ICIPE 621) on FAW egg parasitoid T. remus.
2 Materials and methods
2.1 Fall armyworm rearing
A total of 450 S. frugiperda larvae were collected from maize farms in Homa Bay and Siaya counties (−0.61401° Latitude, 34.09095° Longitude; 1215 m a.s.l.) from April-July 2017. They were maintained in small cages (30 × 30 × 30 cm) under laboratory conditions with a temperature of 25 ± 2 °C, relative humidity of 70 ± 10% and a 12-hour light: 12-hour dark photoperiod. The larvae collected from the field were reared on a semisynthetic diet until they pupated (Akutse et al., 2020a).
The pupae (about 50 per cage) were kept in sleeved Perspex cages (40 × 40 × 45 cm) until the adults emerged. The moths were fed with 10% honey solution, absorbed in cotton wool balls. The colony was then maintained on maize seedlings for more than 10 generations prior to the bioassays. Female moths (about 50 pairs) were released for oviposition and potted maize plants introduced into the cages. After oviposition, the plants were transferred in a separate ventilated cage (50 × 50 × 60 cm) after 24 hours’ exposure to monitor egg hatchability. Three days after hatching, the infested plants were transferred to clean cages (50 × 50 × 60 cm) with lids covered in fine netting for ventilation. To absorb excess moisture, the cages were lined with a paper towel. Fresh maize leaves were fed to the larvae every day until they pupated. The pupae were carefully removed from the cages, using a fine camel hair brush and transferred to sleeved Perspex cages (40 × 40 × 45 cm) until the moths emerged.
2.2 Telenomus remus parasitoids rearing
A colony of T. remus was established from a group of 15 wasps (1:2 males: females) sourced from parasitized eggs of FAW collected during a field survey in Yatta (01.23044°S; 37.45789°E). They were kept in glass vials in the rearing room at icipe’s Animal Rearing and Quarantine Unit (ARQU), at a room temperature of 25 ± 1°C, 12-hour light:12-hour dark photoperiod, and 60-70% relative humidity (RH) (Chepkemoi et al., 2023). The parasitoids were reared on newly laid FAW egg masses, mounted onto rectangular manila cards (1 cm x 5 cm) with white glue and introduced to the wasps for parasitism. The exposure date was noted on the back of each card to track and estimate the expected emergence time of the parasitoids (Kibii, 2021). Additionally, a piece of plain paper (2 cm x 1 cm) coated with honey was placed inside the glass vial as nourishment for the emerged parasitoids. Glass vials holding the parasitoids were stored on the laboratory shelves. About 3-4 days after exposure, the eggs darkened, indicating that they had been parasitized. The parasitized egg cards were then transferred to a separate clean glass vial, where they were incubated at room temperature to monitor emergence of the parasitoids. The parasitoids emerged after a period of about 6-13 days. This process was repeated to maintain the colony, which continued for 16 generations before commencing the bioassays (Kibii, 2021).
2.3 Fungal strains culture and conidia viability assessment
The fungal strains used in this study were sourced from the icipe’s Arthropod Germplasm Centre. The origins and details of the fungal strains (three Metarhizium and one Beauveria) are outlined in Table 1. Metarhizium anisopliae s.l. ICIPE 7, ICIPE 78, and ICIPE 41 strains were cultured on Sabouraud dextrose agar (SDA), while B. bassiana s.l. ICIPE 621 was cultured on Potato dextrose agar (PDA) in Petri dishes (9 cm diameter). The inoculated plates were incubated in complete darkness at a temperature of 25 ± 2°C. After three weeks, the conidia were harvested from surface cultures and suspended in 10 ml distilled water with 0.05% Triton X‐100 in universal bottles (30 ml), each containing three glass beads. The suspension was vortexed for 5 min at about 700 rpm to break the conidial clumps and create a consistent, homogeneous mixture (Akutse et al., 2013). Conidial concentrations were quantified using a hemocytometer under a light microscope. Prior to the bioassays, the conidial suspensions were adjusted to a concentration of 1 × 109 conidia ml-1, and other concentrations were achieved through serial dilution (Akutse et al., 2013). Conidia viability was determined by spread-plating 0.1 ml of 3 x 106 conidia ml-1 onto 9 cm Petri dishes containing SDA or PDA medium. A sterile microscope cover slip (2 x 2 cm) was placed on top of the agar in each plate. Plates were sealed with parafilm and incubated in complete darkness at 25 ± 2°C and examined after 18 - 20 hours. The percentage germination was calculated by counting 100 randomly selected conidia on the area covered by each coverslip under the light microscope (400x magnification) (Oliveira et al., 2015). Conidia was considered to have germinated if the length of the germ tube was at least twice the diameter of the conidium (Oliveira et al., 2015). Each plate represented a replicate and each strain had four replicates and replicated over time (Chepkemoi et al., 2023).
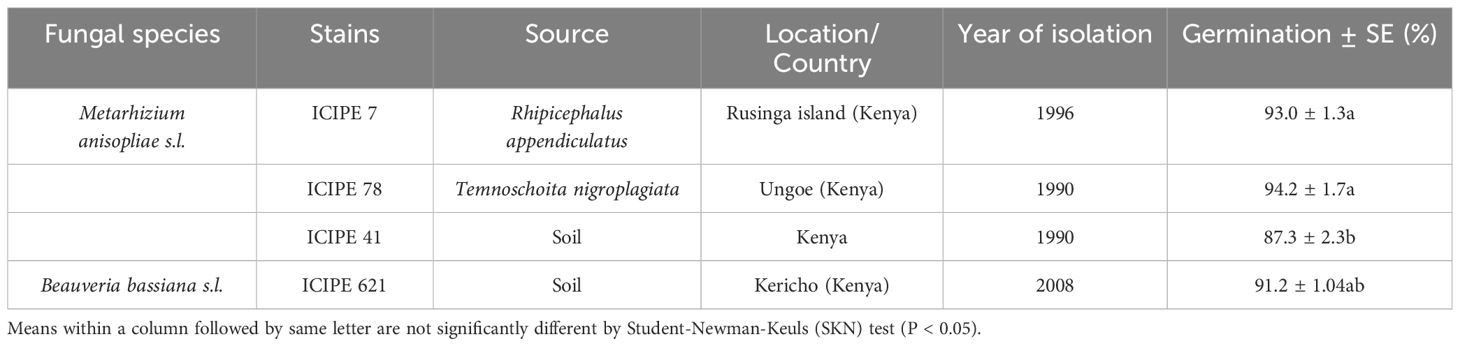
Table 1. Identity of fungal strains screened against Telenomus remus for their virulence assessment under laboratory conditions.
2.4 Direct effects of Metarhizium anisopliae and Beauveria bassiana strains on Telenomus remus
The virulence of four entomopathogenic fungal (EPF) isolates M. anisopliae s.l. strains (ICIPE 7, ICIPE 78, ICIPE 41) and B. bassiana ICIPE 621 s.l was evaluated against T. remus adults. For each fungal treatment, 20 1-day-old T. remus adults (ratio of 1:2/male: female) were infected with 1 g of dry conidia using velvet-coated plastic jars (150 × 80 mm) (Chepkemoi et al., 2023). Twenty (20) adult parasitoids (ratio of 1:2 - males: females) were exposed to the device for 3 min to collect spores. Control treatments were introduced to fungus-free velvet plastic jars. After being exposed for 3 min, the parasitoids that were contaminated were placed in clean 40 ml glass vials and fed with honey. Mortality was recorded daily for 7 days by counting the dead parasitoids. Mycosis in the dead insects was evaluated, with the presence of conidia and hyphae on the cadavers confirming that mortality was due to fungal infection. A completely randomized design was employed to arrange all treatments with each treatment, including the controls, replicated four times over time (Opisa et al., 2018).
Twenty adult parasitoids (ratio of 1:2 - males: females) were exposed to 50 freshly (1-day old) laid FAW eggs for 24 hours after the parasitoids had been contaminated with the fungus. The eggs were then transferred to separate glass vials and monitored until the adult parasitoids emerged. FAW egg mortality and emergence of adult parasitoids were recorded. Additionally, emergence rates of both FAW and adult parasitoids, parasitism rates and sex ratio of the parasitoids were documented. Adult parasitoids were kept in separate vials and fed with honey. The mortality, fecundity, oviposition/parasitism rate, and egg hatchability of the infected parasitoids were also evaluated. The experiment was replicated four times under a completely randomized design (Chepkemoi et al., 2023).
2.5 Indirect effects of Metarhizium anisopliae and Beauveria bassiana strains on Telenomus remus
A hundred freshly laid eggs (1-day old) of S. frugiperda were sprayed directly with 10 ml at different concentrations (1.0 x 105, 1.0 x 106, 1.0 x 107, 1.0 x 108 and 1.0 x 109 conidia ml-1) of M. anisopliae strains (ICIPE 7, ICIPE 78, ICIPE 41) and B. bassiana ICIPE 621 using the Burgerjon’s (1956) spray tower, before being transferred to potted maize plants (Chepkemoi et al., 2023). Control treatments were sprayed with sterile distilled water containing 0.05% Triton X-100. After spraying, the eggs were air-dried then transferred to clean glass vials. Twenty of T. remus parasitoids with a male: female ratio of 1:2, were then introduced into each of the glass vials containing sprayed FAW eggs to assess parasitism. The experiment was replicated four times and arranged in a complete randomized design (Chepkemoi et al., 2023). The bioassays were maintained at temperature of 25-27°C, 50-70% relative humidity, and 12-hour light: 12-hour dark photoperiod. After 24 hours, the sprayed eggs were transferred to clean vials and T. remus adults were provided with honey. The mortality rate of the parasitoids was recorded daily until all individuals died. Dead parasitoids were surface sterilized and placed in Petri dishes lined with damp sterilized filter paper to promote fungal growth on the surface of the cadaver. The mycosis rate was determined by observing fungal development on the surface of the cadavers. Mortality of the FAW eggs and parasitism rates were evaluated (Chepkemoi et al., 2023).
2.6 Data analysis
FAW egg and adult T. remus mortality data were corrected for natural mortality using Abbott’s formula (Abbott, 1925). The data’s normality was tested using Barlett’s test before being subjected to one‐way analyses of variance (ANOVA). The variances of the percentage mortalities were calculated using a binomial generalized linear model with logit link function (Atkinson, 2015). When the treatments showed significant differences (P < 0.05), the means were separated using the Student–Newman–Keuls (SNK) test et al., 20. The lethal time for 50% mortality (LT50) was analyzed by Generalized Linear Model (GLM), using the function ‘dose. p’ from the MASS library, to estimate the lethal time to 50% mortality (LT50) (Opisa et al., 2018). The parasitism rate was calculated as the percentage of the number of parasitoid adults relative to the total number of host eggs and then analyzed using ANOVA. Mycosis data were analyzed using one‐way ANOVA, while the sex ratios assessed using chi-squared test (χ2 test) (Chepkemoi et al., 2023). All data analyses were performed using R (version 3.2.5) statistical software packages (R Core Team, 2018).
3 Results
3.1 Direct effects of entomopathogenic fungal strains on Telenomus remus and FAW eggs
Significant differences were observed in mortality of T. remus adults among all the treatments seven days’ post-treatment (F = 10.16; df = 3, 12; P = 0.001) (Table 2). Beauveria bassiana ICIPE 621 recorded the lowest adult mortality, whereas M. anisopliae ICIPE 7 recorded the highest mortality (Table 2). The strains of M. anisopliae ICIPE 7 and ICIPE 78 were identified as the most virulent, with LT50 less than 2 days. In contrast, M. anisopliae ICIPE 41 and B. bassiana ICIPE 621 had LT50 values of 2.52 and 2.89 days, respectively (Table 2). Calculated LT50 values for the strains indicate that, overall, the M. anisopliae strains induced quicker mortality with lower LT50 values compared to B. bassiana strain. The percentage of mycosis of the cadavers ranged from 16.25 – 47.50% with significant differences observed among the strains (F = 6.306; df = 4, 15; P = 0.003) (Table 2). The fungal strains did not significantly impact FAW eggs hatchability after exposure to the infected T. remus adults seven days’ post-treatment (F = 5.487; df = 3, 12; P = 0.632) (Table 2).
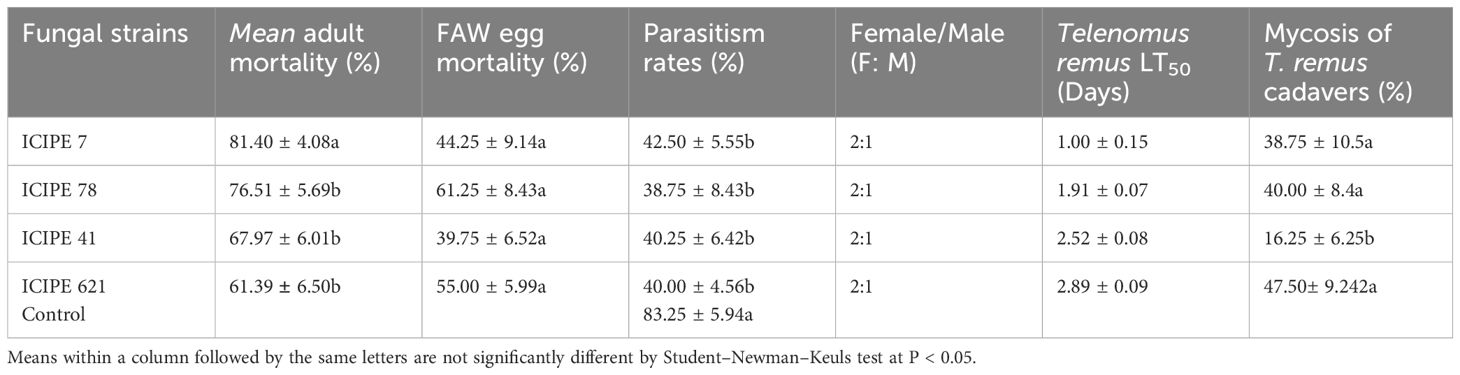
Table 2. Percentage of mortality of Telenomus remus adults; LT50 and FAW eggs induced mortality by the different fungal strains after direct infection.
Furthermore, the number of emerged T. remus adults was significantly influenced by fungal species infection and showed variability among the different strains (F = 9.357; df = 4, 15; P < 0.001) (Table 2). The control treatment had the highest parasitoid emergence rate at 83.25%, while the lowest was recorded in M. anisopliae ICIPE 78 at 38.75% (Table 2). The sex ratios of the emerged parasitoids were similar across all treatments and did not differ significantly (P > 0.05, χ2 test).
3.2 Indirect effects of entomopathogenic fungal strains on Telenomus remus adults
3.2.1 Cumulative mortalities of Telenomus remus adults and FAW eggs after indirect exposure to FAW eggs infected with different strains concentrations
Significant differences in mortality of T. remus adults were evident/obtained in all the concentrations of M. anisopliae ICIPE 41 (F = 14.83; df = 4,15; P < 0.001), ICIPE 7 (F = 14.35; df = 4, 15; P < 0.001), ICIPE 78 (F = 16.34; df = 4,15; P < 0.001) and B. bassiana ICIPE 621 (F = 14.77; df = 4, 15; P < 0.001) strains, respectively, seven days’ post-treatment. Among all the strains, the highest concentration of 1 x 109 conidia ml-1 recorded the highest T. remus adult mortality with ICIPE 41, ICIPE 7, ICIPE 78 and B. bassiana ICIPE 621 recording 62.50%, 87.50%, 100.00% and 77.50%, respectively (Figure 1). Also, significant differences in mycosed cadavers were noted among all the concentrations (F = 15.64; df = 4, 15; P < 0.001) with mycosis ranged from 18.75% to 78.75%.
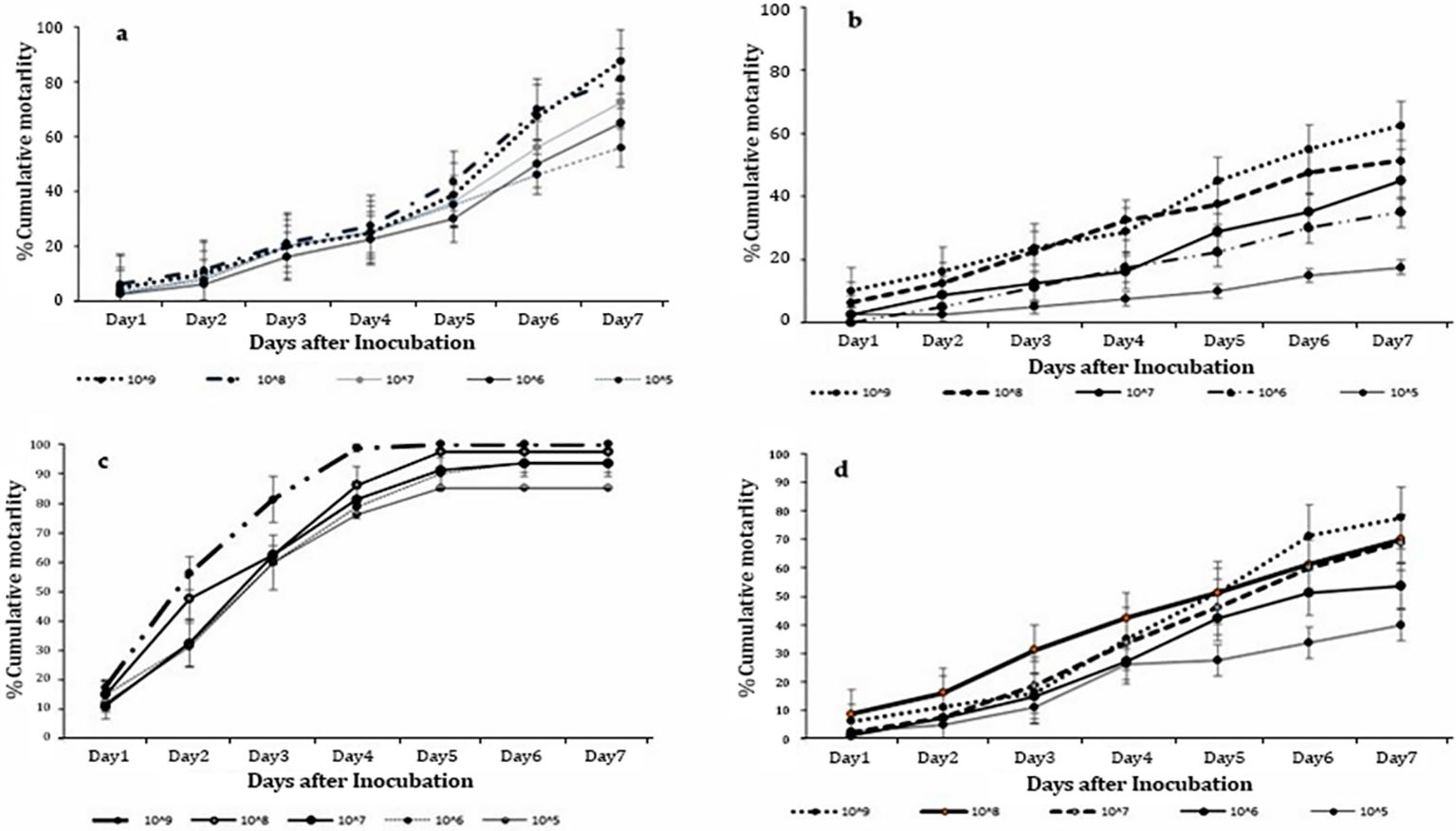
Figure 1. Cumulative mortalities of Telenomus remus adults indirectly exposed to Metarhizium anisopliae s.l. concentrations: (A) ICIPE 7, (B) ICIPE 41, (C) ICIPE 78, and (D) Beauveria bassiana ICIPE 621 s.l.
Furthermore, significant differences in FAW egg mortality were observed among all the M. anisopliae concentrations of M. anisopliae ICIPE 41 (F = 2.77; df = 4, 15; P < 0.001), ICIPE 7 (F = 7.063; df = 4, 15; P < 0.001), and ICIPE 78 (F = 2.304; df = 4, 15; P < 0.001) strains, respectively, seven days post-treatment. In contrast, B. bassiana ICIPE 621, did not show significant effects across the various tested concentrations (F = 4.79; df = 4, 15; P = 0.3). Among all the strains, the highest concentration led to the highest FAW larval adult mortality, with ICIPE 41, ICIPE 7, and ICIPE 78 recording 26.25%, 49.25% and 51.25%, respectively (Table 3).
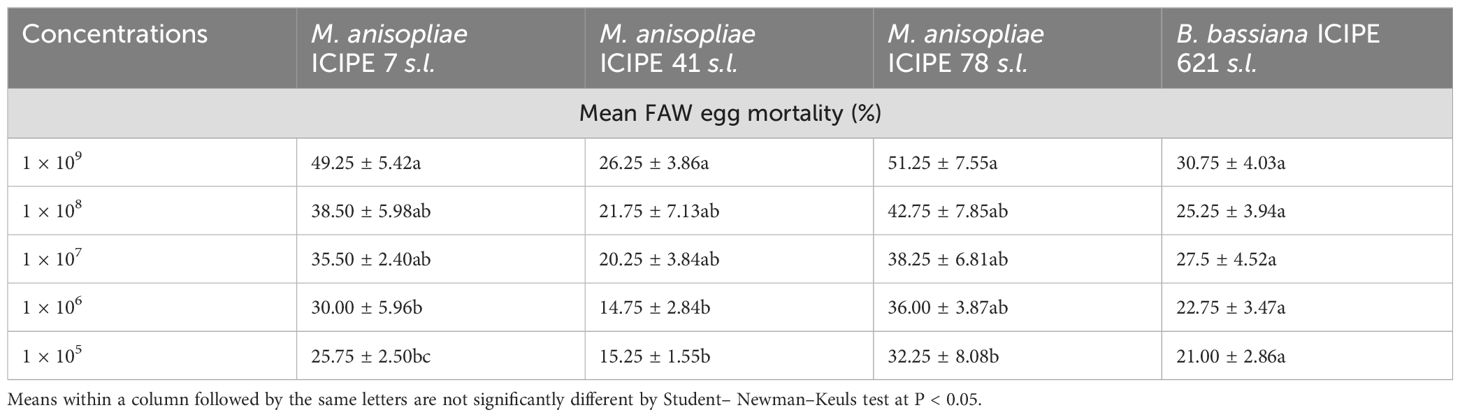
Table 3. Mean FAW egg mortality after infection with the different fungal isolates at the various concentrations.
3.2.2 Effect of entomopathogenic fungi concentrations on parasitoid emergence
The average emergence of T. remus adults was not significantly impacted by M. anisopliae and B. bassiana strains, with ICIPE 41 (F = 1.415; df = 5, 18; P = 0.266), ICIPE 78 (F = 0.913; df = 5, 18; P = 0.495) and B. bassiana ICIPE 621 (F = 1.197; df = 5, 18; P = 0.35) showing no significant differences. However, ICIPE 7 showed a significant effect (F = 3.174; df = 5, 18; P = 0.0315). Additionally, the sex ratios of the F1 progeny of T. remus did not differ significantly across the different fungal strain treatments when compared to the controls (Table 4).
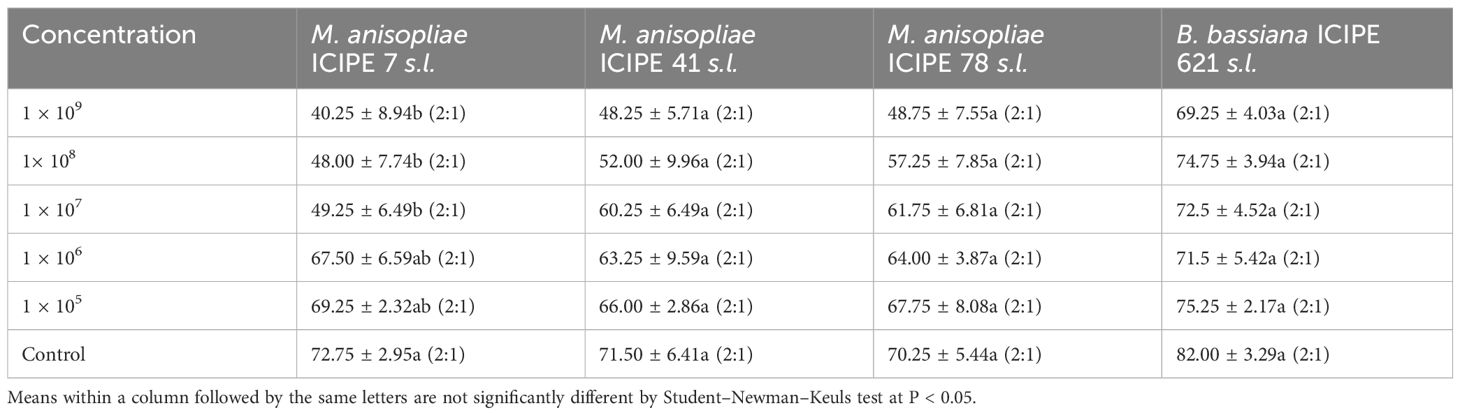
Table 4. Effects of the various fungal strain concentrations on the emergence of Telenomus remus (parasitism rates) in the laboratory (Mean ± SE (%)) and their female/male (F:M) proportion.
4 Discussion
Our study showed that there was a direct effect of EPF on T. remus, causing adult mortality to T. remus when exposed to the different dry conidia of entomopathogenic fungi isolates. Upon direct application, M. anisopliae and B. bassiana strains exhibited pathogenicity towards T. remus adults with ICIPE 7 inducing the highest adult mortality while B. bassiana ICIPE 621 resulted in the least mortality rate. Metarhizium anisopliae strains have generally demonstrated higher virulence than B. bassiana strain in several studies. Previous reports by Martinez et al. (2020) recorded a lower parasitoid Coptera haywardi (Hymenoptera: Diapriidae) infection by B. bassiana strain through a direct application into the soil under semi-natural conditions, when both biological control agents were applied in combination to manage Anastrepha obliqua (Macquart) (Diptera: Tephritidae) fruit flies. The authors observed a significantly lower mortality of A. obliqua under semi-protected conditions as compared to the laboratory (Martinez et al., 2020). The mortality reduction could be attributed to the fact that environmental factors like temperature, solar radiation and humidity have been shown to diminish B. bassiana survival and potency (Robert et al., 2011). This low infection rate allows flexible adjustment of application periods for both parasitoid and fungus in the field, resulting in minimal or no parasitoid infection, as described with the use of B. bassiana and the parasitoid Diadegma semiclausum (Hellen) (Hymenoptera: Ichneumonidae) in controlling Plutella xylostella (L.) (Lepidoptera: Plutellidae) (Monnerat et al., 2002). Presa-Parra et al. (2021) similarly observed that direct infection of M. anisopliae resulted in significant 40% mortality rate in Cephalonomia stephanoderis Betrem (Hymenoptera: Bethylidae) adults, coffee berry borer (Hypothenemus hampei) parasitoid. Another study also recorded 90% mortality in Diadegma semiclausum (Hellen), a parasitoid of P. xylostella, after infection by the fungus Zoophthora. radicans (Abbas, 2020). However, other reports have also shown that Monochamus alternatus (Coleoptera: Cerambycidae), adult pine sawyers were successfully infected with B. bassiana at lethal dose simply by walking across fungus- contaminated non-woven fabric strips with a concentration of 3.5 x 108 conidia cm -2 (Shimazu, 2004).
The strains also had varying killing speeds with the most virulent strains which recorded LT50 less than 2 days being M. anisopliae strains. Despite the fact that all entomopathogenic fungi have a similar infection mechanism, variation in virulence among strains could be due to factors such as proteases, lipases and chitinases, responsible for cuticle destruction and insect penetration (Ortiz-Urquiza and Keyhani, 2013). Previous research by Migiro et al. (2010) also found that entomopathogenic fungi from different species or strains within the same genera caused varying mortality rates in Liriomyza huidobrensis (Blanchard) (Diptera: Agromyzidae), a pea leafminer.
Metarhizium anisopliae ICIPE 78 recorded the highest conidial transfer from adult T. remus parasitoid to S. frugiperda eggs, followed by B. bassiana ICIPE 621. In contrast, M. anisopliae ICIPE 41 recorded the lowest FAW egg mortality rate, indicating less effective conidial transfer and fungal impact. The transfer of fungal conidia by a non-target insect, such as T. remus to the FAW eggs is known as entomopathogenic vectoring. During this process, T. remus parasitoid successfully transmitted some degree of the different fungal strains of the fungi to FAW eggs causing significant mortalities. In previous studies, B. bassiana, B. brongniartii and M. anisopliae were vectored by species of collembolans (Fernaldella fimetaria, (Lepidoptera: Geometridae), Hestina assimilis (Lepidoptera: Nymphalidae), and Proisotoma minuta (Collembola: Isotomidae), which ended up causing mortality on mealworms, Tenebrio molitor (L.) (Coleoptera: Tenebrionidae) (Dromph, 2003). This level of entomopathogenic fungi vectoring (entomovectoring) combined with parasitism showed positive results in the management of FAW as previously reported with Cotesia icipe (Hymenoptera: Braconidae) by Chepkemoi et al. (2023). In the current study, parasitism of T. remus on FAW eggs was highest in samples exposed in the controls, but no significant difference in parasitism was recorded among the different fungal strains. This parasitism extent of T. remus on FAW, unaffected by other external biopesticides was corroborated by a laboratory study conducted by Laminou et al. (2020), where it was reported that T. remus parasitized averagely 78% of FAW eggs, whereas Trichogrammatoidea sp. (Hymenoptera: Trichogrammatidae) parasitized only 25%. Additionally, T. remus was capable to parasitize FAW egg clusters, even when the eggs were completely covered with scales, whereas Trichogrammatoidea sp. could only parasitize eggs that were not covered. Upon parasitization of FAW eggs, T. remus emergence was the highest in controls as compared to those exposed to different fungal strain treatments.
To assess the most effective entomopathogenic fungal concentrations for inducing ovicidal effects on S. frugiperda eggs and their subsequent impact on T. remus adults, our results indicated a general reduction in the lethal efficacy of all the four strains, as the concentrations decreased from 1 × 109 conidia ml-1 to 1 × 105 conidia ml-1. The highest mortality rate of S. frugiperda eggs was observed in M. anisopliae ICIPE 78, followed by M. anisopliae ICIPE 7 and B. bassiana ICIPE 621, whereas least egg mortality was observed in M. anisopliae ICIPE 41. Our results collaborate with those described by Akutse et al. (2013) who showcased ovicidal effects on S. frugiperda after exposure to entomopathogenic fungi in a laboratory study using a single discriminating concentration (1 x 108 conidia ml-1) of M. anisopliae ICIPE 41 and ICIPE 7 which caused 97.5% and 96% mortality rates of eggs and neonates, outperforming strains ICIPE 78, ICIPE 40 and ICIPE 20, which caused mortalities of 87.0%, 83.0% and 79.5%, respectively. A field trial of biocontrol-based IPM on FAW included two treatments: one spray of Bacillus thuringiensis (NBAIR-BT25) and another of M. anisopliae (NBAIR Ma-35) at 2.0 x108 cfu/g. The trials achieved ovicidal effects of 76% and 71.64% on egg masses, respectively (Varshney et al., 2021). The two treatments induced larval mortalities of 80% and 74.4%, respectively, over a period of 60 days’ after application (Varshney et al., 2021). Idrees et al. (2022) reported that B. bassiana strains, when applied at a concentration of 1 x 109 conidia ml-1, resulted in a 96% mortality rate among 2nd instar FAW larvae. Other studies by Anand and Tiwary (2009) showed that, both B. bassiana and M. anisopliae demonstrated ovicidal effects on other species of Spodoptera Guenée, viz., S. litura (Lepidoptera: Noctuoidae). Additionally, these fungi showed efficacy against other lepidopterans including Phthorimaea operculella Zeller (Lepidoptera: Gelechiidae) potato tuber moth, with Khorrami et al. (2018) reporting a 63% larval mortality.
Exposure of T. remus parasitoids to infected FAW eggs showed that higher EPF’s concentrations led to significant mortality among adult parasitoids compared to lower concentrations. Beauveria bassiana ICIPE 621 strain at the concentration of 1 x 109 conidia ml-1, recorded the highest T. remus adult mortality rate, whereas lowest adult mortality was recorded in M. anisopliae ICIPE 78 at the concentration 1 x 105 conidia ml-1. More research have shown that M. anisopliae has the ability to infect Diglyphus isaea Walker (Hymenoptera: Eulophidae), emphasizing the need for careful application, to prevent negative impacts on beneficial parasitoids (Migiro et al., 2010).
Emergence of T. remus F1 progeny was not affected by the different fungal strains showing that the parasitoid mortality occurred after parasitizing the FAW eggs. This is an indication that these strains could be effectively combined with T. remus parasitoid for FAW management. These findings also highlight that even though increased concentrations may increase parasitoid mortality, careful timing is crucial in such way that the females’ parasitoids will have parasitized the hosts before dying. Ferreira Agüero and Neves (2014) similarly observed that Telenomus podisi (Hymenoptera: Scelionidae) parasitism rates on Euschistus heros eggs, treated with M. anisopliae strain. Rashki et al. (2009) also found that releasing the parasitoids before applying entomopathogenic fungi resulted in reduced impact on the parasitoids, in aphid control (Hemiptera: Aphididae). Additionally, Abbas (2020) also evaluated the effect of B. bassiana on the emergence and longevity of Aphidius matricariae (Haliday), the peach aphid, Myzus persicae (Sulzer) parasitoid and reported that the longevity and sex ratios of mummies treated with fungus were similar to untreated ones, four days’ post treatment. Therefore, timing of application for both the parasitoid and the fungus plays a crucial role in their interactions. Some field research have also demonstrated that integrating low dose of EPF with multiple parasitoid species can be an effective pest management strategy (Abbas, 2020).
There was no significant effect on the sex ratios of the emerged T. remus adult parasitoids from both the direct and indirect fungal applications when compared to controls. Moreover, all the tested concentrations resulted in a predominance of female progeny in the F1 generation, which is crucial for parasitoid reproduction. Further research is thus necessary to investigate the lifespan and survival rates of the F1 progenies. Potrich et al. (2009) found no variations in the sex ratios of Trichogramma pretiosum emerging from Ephestia kuehniella (Lepidoptera: Pyralidae) eggs treated with M. anisopliae (Unioeste 22 strain), when compared to control. Furthermore, Polanczyk et al. (2010) reported that M. anisopliae had no effect on the sex ratios of Trichogramma atopovirilia (Hymenoptera: Trichogrammatidae) even when diet was contaminated and eggs immersed in conidial solutions. While studying the effects of M. brunneum on controlling Spodoptera littoralis (Boisduval) (Lepidoptera: Noctuidae) larvae (Miranda-Fuentes et al. 2020) and its interaction with the parasitoid Hyposoter didymator (Thunberg) (Hymenoptera: Ichneumonidae) in melon plants, Miranda-Fuentes et al. (2021) found that, high concentrations of the fungus decreased the longevity of the parasitoids, but did not affect the productive capacity of the females three days post- treatment.
5 Conclusions
Our findings indicated that the autodissemination process of the fungal inoculum, whether vectored by the FAW eggs to the parasitoids or from the parasitoids to the eggs, along with varying the fungal concentrations, could have detrimental effects on the parasitoids. The parasitoids infected with M. anisopliae strains exhibited a higher conidial transfer to their hosts, leading to increased FAW egg mortality. Moreover, results from indirect application indicated that conidia concentrations, which were sublethal to parasitized S. frugiperda eggs, did not adversely affect the performance and emergence of T. remus adults. This suggests that both control agents could be effectively used concurrently, with an appropriate time of release. To accurately assess compatibility, more field studies are required to provide enough information on how the two biocontrol agents perform in a non-controlled environment. Further research is needed to explore more effective application methods to enhance the impact of the two biocontrol agents in field conditions.
Data availability statement
The original contributions presented in the study are included in the article/supplementary material. Further inquiries can be directed to the corresponding author.
Ethics statement
The manuscript presents research on animals that do not require ethical approval for their study.
Author contributions
JC: Conceptualization, Formal Analysis, Methodology, Validation, Writing – original draft, Writing – review & editing. KF: Conceptualization, Resources, Supervision, Validation, Writing – review & editing. FA: Conceptualization, Data curation, Software, Validation, Writing – review & editing. JM: Investigation, Methodology, Validation, Writing – review & editing. KA: Conceptualization, Formal Analysis, Funding acquisition, Investigation, Methodology, Project administration, Resources, Supervision, Validation, Writing – original draft, Writing – review & editing.
Funding
The author(s) declare that financial support was received for the research, authorship, and/or publication of this article. This research was funded by the UK’s Foreign, Commonwealth and Development Office (FCDO) (B2329A-DFID-FAW and B2291A - FCDO - BIOPESTICIDE) through the International Centre of Insect Physiology and Ecology (icipe).
Acknowledgments
The authors gratefully acknowledge the icipe core funding provided by the Swedish International Development Cooperation Agency (Sida); the Swiss Agency for Development and Cooperation (SDC); the Australian Centre for International Agricultural Research (ACIAR); the Norwegian Agency for Development Cooperation (Norad); the German Federal Ministry for Economic Cooperation and Development (BMZ); and the Government of the Republic of Kenya. The first author was supported through the Dissertation and Research Internship Program (DRIP) of icipe. We are also thankful to Sospeter Wafula, Jane Kimemia and Levi Ombura for their technical assistance. The views expressed herein do not necessarily reflect the official opinion of the donors.
Conflict of interest
The authors declare that the research was conducted in the absence of any commercial or financial relationships that could be construed as a potential conflict of interest.
Publisher’s note
All claims expressed in this article are solely those of the authors and do not necessarily represent those of their affiliated organizations, or those of the publisher, the editors and the reviewers. Any product that may be evaluated in this article, or claim that may be made by its manufacturer, is not guaranteed or endorsed by the publisher.
References
Abbas, A., Ullah, F., Hafeez, M., Han, X., Dara, M. Z. N., Gul, H., et al. (2022). Biological control of fall armyworm, Spodoptera frugiperda. Agronomy 12, 2704. doi: 10.3390/agronomy12112704
Abbas, M. (2020). Interactions between entomopathogenic fungi and entomophagous insects. Adv. Entomol. 08, 130–146. doi: 10.4236/ae.2020.83010
Abbott, W. S. (1925). A method of computing the effectiveness of an insecticide. J. Econ. Entomol. 18, 265–267. doi: 10.1093/jee/18.2.265a
Ferreira Agüero, M. A., Neves, O. J. (2014). Selectivity of Metarhizium anisopliae to Telenomus podisi (Hymenoptera: Scelionidae). Investigación. Agraria. 16, 21–28.
Akutse, K. S., Khamis, F. M., Ambele, F. C., Kimemia, J. W., Ekesi, S., Subramanian, S. (2020a). Combining insect pathogenic fungi and a pheromone trap for sustainable management of the fall armyworm, Spodoptera frugiperda (Lepidoptera: Noctuidae). J. Invertebrate. Pathol. 177, 107477. doi: 10.1016/j.jip.2020.107477
Akutse, K. S., Kimemia, J. W., Ekesi, S., Khamis, F. M., Ombura, O. L., Subramanian, S. (2019). Ovicidal effects of entomopathogenic fungal isolates on the invasive fall armyworm Spodoptera frugiperda (Lepidoptera: Noctuidae). J. Appl. Entomol. 143, 626–634. doi: 10.1111/jen.12634
Akutse, K. S., Maniania, N. K., Fiaboe, K. K. M., Van den Berg, J., Ekesi, S. (2013). Endophytic colonization of Vicia faba and Phaseolus vulgaris (Fabaceae) by fungal pathogens and their effects on the life-history parameters of Liriomyza huidobrensis (Diptera: Agromyzidae). Fungal Ecol. 6, 293–301. doi: 10.1016/j.funeco.2013.01.003
Akutse, K. S., Subramanian, S., Maniania, K. N., Dubois, T., Ekesi, S. (2020b). Biopesticide research and product development in Africa for sustainable agriculture and food security – Experiences from the International Centre of Insect Physiology and Ecology (icipe). Front. Sustain. Food Syst. 4. doi: 10.3389/fsufs.2020.563016
Anand, R., Tiwary, B. N. (2009). Pathogenicity of entomopathogenic fungi to eggs and larvae of Spodoptera litura, the common cutworm. Biocontrol Sci. Technol. 9 (9), 919–929. doi: 10.1080/09583150903205069
Atkinson, A. C. (2015). “Optimal experimental design,” in International Encyclopedia of the Social & Behavioral Sciences, 2nd ed., (Netherlands: Elsevier) 256–262. doi: 10.1016/B978-0-08-097086-8.42041-6
Bateman, M. L., Day, R. K., Luke, B., Edgington, S., Kuhlmann, U., Cock, M. J. (2018). Assessment of potential biopesticide options for managing fall armyworm (Spodoptera frugiperda) in Africa. J. Appl. Entomol. 142, 805–819. doi: 10.1111/jen.12565
Burgerjon, A. (1956). Pulverisation de poudrage au laboratoire par des préparations pathogènes insecticides. Annales des Épiphyties 4, 677–688.
Chepkemoi, J., Fening, K. O., Ambele, F. C., Munywoki, J., Akutse, K. S. (2023). Direct and indirect infection effects of four potent fungal isolates on the survival and performance of fall armyworm larval parasitoid Cotesia icipe. Sustainability 15, 3250. doi: 10.3390/su15043250
Day, R., Abrahams, P., Bateman, M., Beale, T., Clottey, V., Cock, M., et al. (2017). Fall armyworm: Impacts and implications for Africa. Outlooks. Pest Manage. 28 (5), 196–201. doi: 10.1564/v28_oct_02
Dromph, K. M. (2003). Collembolans as vectors of entomopathogenic fungi. Pedobiologia. 47 (3), 245–256. doi: 10.1078/0031-4056-00188
Goergen, G., Kumar, P. L., Sankung, S. B., Togola, A., Tamò, M. (2016). First report of outbreaks of the fall armyworm Spodoptera frugiperda (J E Smith) (Lepidoptera, Noctuidae), a new alien invasive pest in West and Central Africa. PloS One 11, 1–9. doi: 10.1371/journal.pone.0165632
Harrison, R. D., Thierfelder, C., Baudron, F., Chinwada, P., Midega, C., Schaffner, U., et al. (2019). Agro-ecological options for fall armyworm (Spodoptera frugiperda JE Smith) management: Providing low-cost, smallholder-friendly solutions to an invasive pest. J. Environ. Manage. 243, 318–330. doi: 10.1016/j.jenvman.2019.05.011
Hruska, A. J. (2019). Fall armyworm (Spodoptera frugiperda) management by smallholders. CABI Reviews 043, 0–3. doi: 10.1079/PAVSNNR201914043
Idrees, A., Afzal, A., Qadir, Z. A., Li, J. (2022). Bioassays of Beauveria bassiana isolates against the fall armyworm, Spodoptera frugiperda. J. Fungi. 8, 717. doi: 10.3390/jof8070717
Khorrami, F., Mehrkhou, F., Mahmoudian, M., Ghosta, Y. (2018). “Pathogenicity of three different entomopathogenic fungi, Metarhizium anisopliae IRAN 2252, Nomuraea rileyi IRAN 1020C and Paecilomyces tenuipes IRAN 1026C against the potato tuber moth, Phthorimaea operculella Zeller (Lepidoptera: Gelechiidae),” in Potato Research. (Switzerland: Springer) doi: 10.1007/s11540-018-9378-z
Kibii, M. J. (2021). Diversity and performance of egg parasitoids occurring on fall armyworm (Spodoptera frugiperda J.E. Smith) on maize in Kenya (Doctoral dissertation (University of Nairobi).
Laminou, S. A., Ba, M. N., Karimoune, L., Doumma, A., Muniappan, R. (2020). Parasitism of locally recruited EGG parasitoids of the fall armyworm in Africa. Insects. 11 (7), 430. doi: 10.3390/insects11070430
Macauley, H. (2015). Cereal crops: Rice, maize, millet, sorghum, wheat. (Ghana: University of Cape Coast) 21–23. doi: 10.4324/9781315560625-26
Martinez, B., Olga, T., Jorge, C., Jorge, L., Pablo, G., Jaime, M., et al. (2020). Interaction between Beauveria bassiana (Hypocreales: Cordycipitaceae) and Coptera haywardi (Hymenoptera: Diapriidae) for the management of Anastrepha obliqua (Diptera: Tephritidae). J. Insect Sci. 20 (2), 6. doi: 10.1093/jisesa/ieaa010
Migiro, L., Maniania, N. M., Chabi-Olaye, A., Van den Berg, J. (2010). Pathogenicity of entomopathogenic fungi Metarhizium anisopliae and Beauveria bassiana (Hypocreales: Clavicipitaceae) isolates to the adult pea leafminer (Diptera: Agromyzidae) and prospects of an autoinoculation device for infection in the field. Environ. Entomol. 39, 468–475. doi: 10.1603/EN09359
Miranda-Fuentes, P., Quesada-Moraga, E., Aldebis, H. K., Yousef-Naef, M. (2020). Compatibility between the endoparasitoid Hyposoter didymator and the entomopathogenic fungus Metarhizium brunneum: a laboratory simulation for the simultaneous use to control Spodoptera littoralis. Pest Manage. Sci. 76, 1060–1070. doi: 10.1002/ps.5616
Miranda-Fuentes, P., Yousef-Yousef, M., Valverde-García, P., Rodríguez-Gómez, I., Garrido-Jurado, I., Quesada-Moraga, E. (2021). Entomopathogenic fungal endophyte-mediated tritrophic interactions between Spodoptera littoralis and its parasitoid Hyposoter didymator. Journal of Pest Science. 94 (4), 933–945. doi: 10.1007/s10340-021-01344-3
Monnerat, R. G., Kirk, A. A., Bordat, E. D. (2002). Biology of Diadegma sp. (Hymenoptera: Ichneumonidae), a parasitoid of Plutella xylostella (L.) (Lepidoptera: Yponomeutidae), from Reunion Island. 2002. Neotropical. Entomol. 31, 271–274. doi: 10.1590/S1519-566X2002000200015
Morales-Reyes, Z., Martín-López, B., Moleón, M., Mateo-Tomás, P., Botella, F., Margalida, A., et al. (2018). Farmer perceptions of the ecosystem services provided by scavengers: what, who, and to whom. Conserv. Lett. 11, e12392. doi: 10.1111/conl.12392
Oliveira, D. ,. G., Pauli, G., Gabriel, M. M., Delaibera, I. (2015). A protocol for determination of conidial viability of the fungal entomopathogens Beauveria bassiana and Metarhizium anisopliae from commercial products. J. Microbiol. Methods 119, 44–52. doi: 10.1016/j.mimet.2015.09.021
Opisa, S., du Plessis, H., Akutse, K. S., Fiaboe, K. K. M., Ekesi, S. (2018). Effects of Entomopathogenic fungi and Bacillus thuringiensis-based biopesticides on Spoladea recurvalis (Lepidoptera: Crambidae). J. Appl. Entomol. 142, 617–626. doi: 10.1111/jen.12512
Ortiz-Urquiza, A., Keyhani, N. O. (2013). Action on the surface: Entomopathogenic fungi versus the insect cuticle. Insects 4, 357–374. doi: 10.3390/insects4030357
Polanczyk, R. A., Pratissoli, D., Dalvi, L. P., Grecco, E. D., Franco, C. R. (2010). Efeito de Beauveria bassiana (Bals.) Vuillemin e Metarhizium anisopliae (Metsch.) Sorokin nos parámetros biologi-cos de Trichogramma atopovirilia Oatman &; Platner 1983 (Hymenoptera: trichogrammatidae). Sci. Agrotechnol. 34, 1412–1416. doi: 10.1590/S1413-70542010000600008
Potrich, M., Alves, L. F., Haas, J., Da Silva, E. R., Daros, A., Pietrowski, V., et al. (2009). Seletividade de Beauveria bassiana e Metarhizium anisopliae a Trichogramma pretiosum Riley (Hymenoptera: Trichogrammatidae). Neotropical. Entomol. 38, 822–826. doi: 10.1590/S1519-566X2009000600016
Prasanna, B., Huesing, J. E., Eddy, R., Peschke, V. M. (2018). Fall armyworm in Africa: A guide for integrated pest management (Mexico, CDMX: CIMMYT, First Edit), 45–62.
Presa-Parra, E., Hernández-Rosas, F., Bernal, J. S., Valenzuela-González, J. E., Martínez-Tlapa, J., Birke, A. (2021). Impact of Metarhizium robertsii on adults of the parasitoid Diachasmimorpha longicaudata and parasitized Anastrepha ludens larvae. Insects 12, 125. doi: 10.3390/insects12020125
Rashki, M., Kharazi-Pakdel, A., Allahyari, H., Alphen, J. (2009). Interactions among the entomopathogenic fungus, Beauveria bassiana (Ascomycota: Hypocreales), the parasitoid, Aphidius matricariae (Hymenoptera: Braconidae), and its host, Myzus persicae (Homoptera: Aphididae). Biol. Control. 50, 324–328. doi: 10.1016/j.biocontrol.2009.04.016
R Core Team (2018). R: A Language and Environment for Statistical Computing (Vienna, Austria: R Foundation for Statistical Computing). Available online at: https://www.R-project.org/ (Accessed 23 June 2021).
Roşca, M., Mihalache, G., Stoleru, V. (2023). “Banned pesticides with high persistence: The impact of their use in agriculture and their removal by microbial biodegradation,” in Microbial Bioremediation. Eds. Bhat, R. A., Butnariu, M., Dar, G. H., Hakeem, K. R. (Springer, Cham). doi: 10.1007/978-3-031-18017-0_3
Robert, D. A., Andrew, S. B., Simon, B., Krijn, P. P., Matthew, B. T. (2011). Comparative growth kinetics and virulence of four different isolates of entomopathogenic fungi in the house fly (Musca domestica L.). J. Invertebrate. Pathol. 107, 179–184. doi: 10.1016/j.jip.2011.04.004
Shimazu, M. (2004). A novel technique to inoculate conidia of entomopathogenic fungi and its application for investigation of susceptibility of the Japanese pine sawyer, Monochamus alternatus, to Beauveria bassiana. Appl. Entomol. Zool. 9 (3), 485–490. doi: 10.1303/aez.2004.485
Sisay, B., Simiyu, J., Mendesil, E., Likhayo, P., Ayalew, G., Mohamed, S., et al. (2019). Fall armyworm, Spodoptera frugiperda infestations in East Africa: Assessment of damage and parasitism. Insects 10, p.195. doi: 10.3390/insects10070195
Varshney, R., Poornesha, B., Raghavendra, A., Lalitha, Y., Apoorva, V., Ramanujam, B., et al. (2021). Biocontrol-based management of fall armyworm, Spodoptera frugiperda (J. E. Smith) (Lepidoptera: Noctuidae) on Indian maize. J. Plant Dis. Prot. 28, 87–95. doi: 10.1007/s41348-021-00453-7
Keywords: biological control, Beauveria bassiana, fall armyworm, Metarhizium anisopliae, Telenomus remus, insect-microbe interactions
Citation: Chepkemoi J, Fening KO, Ambele FC, Munywoki J and Akutse KS (2024) Effects of four potent entomopathogenic fungal isolates on the survival and performance of Telenomus remus, an egg parasitoid of fall armyworm. Front. Cell. Infect. Microbiol. 14:1445156. doi: 10.3389/fcimb.2024.1445156
Received: 06 June 2024; Accepted: 26 August 2024;
Published: 12 September 2024.
Edited by:
Junaid Ali Siddiqui, Guizhou University, ChinaReviewed by:
Bartholomew Saanu Adeleke, Olusegun Agagu University of Science and Technology, NigeriaMohamed Abdel-Raheem, National Research Centre (Egypt), Egypt
Carlos Gava, Embrapa Semiárido, Brazil
Copyright © 2024 Chepkemoi, Fening, Ambele, Munywoki and Akutse. This is an open-access article distributed under the terms of the Creative Commons Attribution License (CC BY). The use, distribution or reproduction in other forums is permitted, provided the original author(s) and the copyright owner(s) are credited and that the original publication in this journal is cited, in accordance with accepted academic practice. No use, distribution or reproduction is permitted which does not comply with these terms.
*Correspondence: Komivi Senyo Akutse, kakutse@icipe.org