- 1College of Veterinary Medicine, Gansu Agricultural University, Lanzhou, China
- 2College of Chemistry and Life Sciences, Gansu Minzu Normal University, Gannan, China
- 3Innovation Center for Traditional Chinese Veterinary Medicine, College of Veterinary Medicine, China Agricultural University, Beijing, China
- 4Zhangye Wanhe Animal Husbandry Industry Technology Development Co., Ltd, Zhangye, China
Object: To investigate the effects of Shen Qi Bu Qi Powder (SQBQP) on the average daily gain, blood indexes, gastrointestinal microflora, and serum metabolites of calves.
Methods: A total of 105 calves were randomly assigned to three groups (n = 35 per group): the control group (C, fed with a basal diet for 21 days) and two treatment groups (SQBQP-L and SQBQP-H, fed with the basal diet supplemented with 15 and 30 g/kg of SQBQP), respectively for 21 days. The active components of SQBQP were identified using LC-MS/MS. Serum digestive enzymes and antioxidant indices were determined by ELISA kits and biochemical kits, respectively. Serum differential metabolites were analyzed by liquid chromatography-mass spectrometry/mass spectrometry (LC-MS/MS), while flora in rumen fluid and fecal were analyzed by 16S rDNA sequencing. Further correlation analysis of gastrointestinal flora and serum metabolites of SQBQP-H and C groups were performed with Spearman’s correlation.
Results: The principal active components of SQBQP mainly includes polysaccharides, flavonoids, and organic acids. Compared to the control group (C), calves in the SQBQP-H (high dose) and SQBQP-L (low dose) groups showed a significant increase in serum amylase (AMS) levels (P<0.001), while lipase content significantly decreased (P<0.05). Additionally, the average daily gain, T-AOC, and cellulase content of calves in the SQBQP-H group significantly increased (P<0.05). Proteobacteria and Succinivibrio in the rumen flora of the SQBQP-H group was significantly lower than that of the C group (P<0.05). The relative abundance of Proteobacteria, Actinobacteria, Candidatus_Saccharibacteria, Deinococcus_Thermus, Cyanobacteria, and Succinivibrio in the SQBQP-H group was significantly increased (P<0.05), while the relative abundance of Tenericutes and Oscillibacter was significantly decreased (P<0.05). Serum metabolomics analysis revealed 20 differential metabolites, mainly enriched in amino acid biosynthesis, β-alanine metabolism, tyrosine, and tryptophan biosynthesis metabolic pathways (P<0.05). Correlation analysis results showed that Butyrivibrio in rumen flora and Oscillibacter_valericigenes in intestinal flora were significantly positively correlated with average daily gain, serum biochemical indexes, and differential metabolite (-)-Epigallocatechin (R>0.58, P<0.05).
Conclusion: SQBQP can promote calves weight gain and enhance health by modulating gastrointestinal flora and metabolic processes in the body.
1 Introduction
In light of the rapid expansion of the beef cattle breeding industry in China, the intensification degree is on the rise. The off-site fattening mode has emerged as the dominant paradigm in this context. Factors such as transportation, driving, vaccination stimuli, and heat stress negatively impact calves, leading to reduce immunity and growth performance, and increase morbidity and mortality (Deters and Hansen, 2022). To support the “Antibiotic Reduction Action” initiative by the Ministry of Agriculture and Rural Affairs of China and to promote the healthy, green, and sustainable development of the beef cattle industry, it is crucial to conduct research on the efficacy of Chinese veterinary compounds for improving calf health and growth.
Shen Qi Bu Qi Powder (SQBQP) is a self-made prescription by us, which primarily comprises natural Chinese herbal medicines, including Radix Astragali and Rhizoma Atractylodis macrocephalae and so on. The prescription has the effects of strengthening the stomach, replenishing qi, and consolidating the surface. Previous research conducted by us found that SQBQP could improve the immune index of 3-month-old calves, and could not effect on the liver and kidney function. The active ingredients of traditional Chinese medicine (TCM) regulate the immune level of animals by affecting the antioxidant level (Huo et al., 2022), digestive enzyme activity (Liu et al., 2023d; Long et al., 2020), rumen, and intestinal microbial flora (Che et al., 2022; Chen et al., 2023), thus achieving the desired health care effect. Some studies found that TCM was rich in active ingredients such as polysaccharides, flavonoids, and saponins (Zhu and Yu, 2018), which play an important role in bacteriostasis (Shi et al., 2022), anti-inflammatory (Lin et al., 2015), promoting growth (Liu et al., 2023b), and improving immunity effects (Liu et al., 2019). The medicinal and food-related TCM like Radix Astragali and Rhizoma Atractylodis macrocephalae have demonstrated extensive applications in breeding industry, including the rearing of aquatic animals, poultry, pigs, sheep, cows, and rabbits (Salazar et al., 2019; Swelum et al., 2021; Zou et al., 2022). The serum antioxidant index is a crucial indicator for assessing oxidative stress in animals. The active ingredients present in Radix Astragali and Rhizoma Atractylodis macrocephalae include polysaccharides, organic acids, and flavonoids, which have been demonstrated to effectively enhance the activity of antioxidant enzymes such as superoxide dismutase (SOD) and glutathione peroxidase (GSH-Px) in serum. These ingredients have been shown to reduce the damage caused by oxidative free radicals to cell membranes and to maintain intestinal barrier function (Hao et al., 2020; Wang et al., 2021). Serum digestive enzyme activity is a key indicator of the animal’s digestion and absorption abilities. TCM practices have been shown to enhance the activity of digestive enzymes, including pepsin, trypsin, and amylase, thus facilitating the nutrient digestion and absorption and improving the production performance (Harikrishnan et al., 2022). The rumen microbial flora plays an important regulatory role in the nutrition and metabolism of ruminants. The active ingredients in TCM have been shown to enhance feed degradation and fermentation, thereby improving the feed utilization by regulating the structure of gastrointestinal microbial flora (Kholif and Olafadehan, 2021; Harikrishnan et al., 2022; Rabee et al., 2024). These ingredients selectively promote the growth of beneficial bacteria, inhibit harmful bacteria, maintain rumen environment stability, and enhance body immunity (Liu et al., 2023b; Ma et al., 2023). Analyzing serum metabolites provides a deeper understanding of the effects of TCM on the animal metabolism and elucidates the comprehensive multi-component, multi-channel, and multi-target mechanisms of TCM action (Liu et al., 2023a).
In this study, SQBQP was used to feed calves, and its effects on the growth performance, serum antioxidant capacity, digestive enzyme activity, rumen and intestinal microflora, and serum metabolism of calves were analyzed. The aim was to systematically investigate the mechanisms of promoting calf health and weight gain of SQBQP, thereby support its effective application in the healthy breeding of calves.
2 Materials and methods
2.1 Preparation of Shen Qi Bu Qi powder
Radix Astragali, Radix Codonopsis pilosulae, Radix Saposhnikoviae divaricatae, and Rhizoma Atractylodis macrocephalae were crushed. Then they were passed through a 100-mesh sieve and mixed in proportion to create the final preparation.
2.2 LC-MS/MS analysis of SQBQP samples
The active components of SQBQP were subjected to analysis by Waters 2D UPLC (Waters, USA) in conjunction with a Q Exactive high-resolution mass spectrometer (Thermo Fisher Scientific, USA). Chromatographic conditions were as follows: the chromatographic column used was a Hypersil GOLD a Q column (100*2.1 mm, 1.9 μm, Thermo Fisher Scientific, USA). The mobile phases employed were 0.1% formic acid in water (liquid A) and 100% acetonitrile containing 0.1% formic acid (liquid B). The elution gradients were as follows: The mobile phases were as follows: 0-2 min, 5% B; 2-22 min, 5%-95% B; 22-27 min, 95% B; 27.1-30 min, 5% B. The flow rate was 0.3 mL/kg. The column temperature was maintained at 40°C, the flow rate was set at 0.3 mL/min, and the injection volume was fixed at 5 μL. Mass spectrometry: The primary and secondary mass spectrometry data were collected using a Q Exactive mass spectrometer (Thermo Fisher Scientific, USA). The mass-to-charge ratio range for mass spectrometry scanning was 150-1500, with a primary resolution of 70,000, an AGC of 1e6, and a maximum injection time (IT) of 100 ms. The top three parent ions were selected for fragmentation, and secondary information was subsequently collected. The secondary resolution was 35,000, the AGC was 2e5, the maximum injection time (IT) was 50 ms, and the stepped voltage was set to 20, 40, and 60 eV. The settings for the ion source (ESI) were as follows: the sheath gas flow rate was 40, the auxiliary gas flow rate was 10, the spray voltage (kV) was 3.80 for positive ion mode, and 3.20 for negative ion mode, the capillary temperature was 320°C, and the temperature of the auxiliary gas heater was 350°C. The TCMSP database was used to compare the results of LC-MS/MS detection.
2.3 Experimental animals
A total of 105 three-month-old Simmental hybrid bull calves with a mean body weight of 122.55 ± 15.01 kg were randomly assigned to three groups, with 35 calves in each group. There was no significant difference in the body weight of the calves among the three groups. This experiment was conducted at the beef cattle farm of Wanhe Grass Livestock Industry Science and Technology Development Co., Ltd. in Zhangye City, Gansu Province, China. Calves in the control group (C) were fed with a basal diet daily (Table 1) for 21 days. Calves in the SQBQP-L group were fed with a basal diet supplemented with 15 g/kg of SQBQP for 21 days, and calves in the SQBQP-H group were fed with a basal diet supplemented with 30 g/kg of SQBQP for 21 days. The calves were fed twice daily, at 7:00-8:00 am and 2:30-3:30 pm, taking food and water freely.
2.4 Sample collection
At the end of the experiment, the blood (10 mL) was collected from the tail vein of all experimental animals before morning feeding. The serum was separated by centrifugation at 3500 rpm for 15 mins and stored at -80°C. Six calves were randomly selected from each group for ruminal fluid collection, which was filtered and stored in a refrigerator at -80°C. Additionally, 14 calves were randomly selected from each group, and approximately 5 g of fresh feces were collected and stored at -80°C.
2.5 Average daily gain and daily feed intake of calves
Initial and final weights were recorded before and after the test and the daily weight gain was calculated.
2.6 Detection of serum antioxidant and digestive enzyme indexes
Four Enzyme-linked immunosorbent assay kits (Shanghai Enzyme-linked Biological Co., Ltd.) were used to determine amylase (AMS, YJ921025), cellulase (Cellulase, YJ551029), protease (Protease, YJ361025), and lipase (Lipase, YJ295405) in calf serum. In addition, serum total antioxidant capacity (T-AOC, A015-2-1), total superoxide dismutase (T-SOD, A001-1-2), malondialdehyde (MDA, A003-1), catalase (CAT, A007-1-1), and reduced glutathione (GSH, A006-2-1) activities were measured using the biochemical kits (Nanjing Jiancheng Bioengineering Institute, Nanjing, China).
2.7 Detection of rumen fluid and fecal samples
A total of six rumen fluid samples and fourteen fecal samples were selected from each group for the gastrointestinal flora detection. For PCR amplification, 30 ng of qualified genomic DNA and corresponding fusion primers were used to configure the PCR reaction system and set the PCR reaction parameters. The PCR amplification products were purified using Agencourt AMPure XP magnetic beads, dissolved in the elution buffer, labeled, and the library construction was completed. The Agilent 2100 Bioanalyzer was used to determine the fragment range and concentration of the library. The qualified library was sequenced on a sequencer based on the size of the inserted fragment. After data filtering, the remaining high-quality clean data is used for post-analysis. Through the overlapping relationship between reads, reads are spliced into tags. Tags were clustered into OTUs and compared with the database and species annotation. Based on the OTU and annotation results, sample species complexity analysis and intergroup species difference analysis were performed.
2.8 Serum metabolomics analysis
A total of six serum samples were selected from each group for detecting serum metabolites. Metabolites were separated and detected by Waters 2D UPLC (Waters, USA) and Q Exactive high-resolution mass spectrometer (Thermo Fisher Scientific, USA). The chromatographic column used was a BEH C18 chromatographic column (1.7 μm 2.1 * 100 mm, Waters, USA). In the positive ion mode, the mobile phase consisted of 0.1% aqueous formic acid solution (A) and 100% methanol (B) containing 0.1% formic acid. In the negative ion mode, the mobile phase consisted of 10 mM ammonium formate aqueous solution (A) and 95% methanol (B) containing 10 mM ammonium formate. The following gradient was used for elution: 0-1 min, 2% B solution; 1~9 min, 2%~98% B solution; 9~12 min, 98% B solution; 12~12. 1 min, 98% B solution~2% B solution 12. 1~15 min, 2% B liquid. The flow rate was 0.35 mL/min, the column temperature was 45°C and the injection volume was 5 μL. Q Exactive mass spectrometer (Thermo Fisher Scientific, USA) was used to collect primary and secondary mass spectrometry data. The MS scan mass-to-nucleus ratio range was 70-1050, the first order resolution was 70,000, the AGC was 3e6 and the maximum injection time (IT) was 100 ms. According to the parent ion strength, Top3 was selected for fragmentation, and secondary information was collected. The secondary resolution was 17,500, the AGC was 1e5, the maximum injection time (IT) was 50 ms and the fragmentation energy (stepped once) was set to 20, 40, 60 eV. The ion source (ESI) parameters were set as follows: Sheath gas flow rate was 40, auxiliary gas flow rate was 10, spray voltage (| KV |) was 3.80 in positive ion mode, 3.20 in negative ion mode, capillary temperature was 320°C, auxiliary gas heater temperature was 350°C.
2.9 Statistical methods
Statistical analysis was performed using the GraphPad. Prism. 9.5 software. The data were presented in the form of X ± SEM, and T-test and one-way analysis of variance were used for group comparison. P<0.05 indicated a significant difference, and P<0.01 indicated an extremely significant difference.
3 Results
3.1 LC-MS/MS analysis of SQBQP sample analysis
The TCMSP database was used to compare the results of LC-MS/MS detection. The results showed that 27 active components were identified in SQBQP, including polysaccharides, amino acids, flavonoids, nucleic acids, organic acids, and alcohols. The top three components were polysaccharides, flavonoids, and organic acids (Figure 1, Table 2).
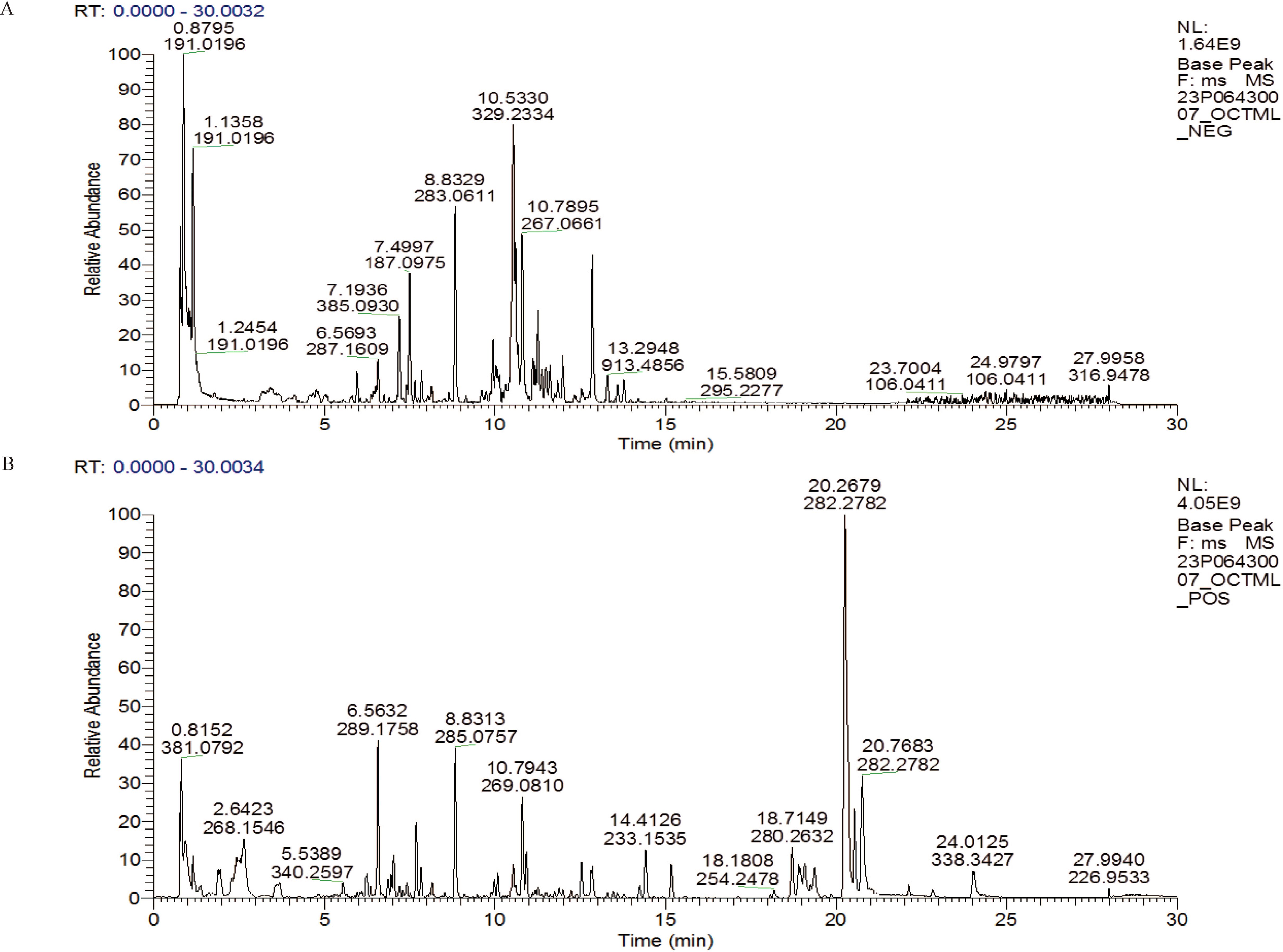
Figure 1. LC-MS/MS total ion chromatogram in SQBQP. (A) Negative ion mode diagram; (B) Positive ion mode diagram.
3.2 Effects of the SQBQP on the average daily gain and the daily feed intake of calves
The average daily gain (ADG) of calves in the SQBQP-H group was significantly higher than that in the C group (Figure 2A). There was no significant change in the daily feed intake of calves between groups (Figure 2B). It was concluded that SQBQP could significantly increase the average daily gain of calves (P<0.05).
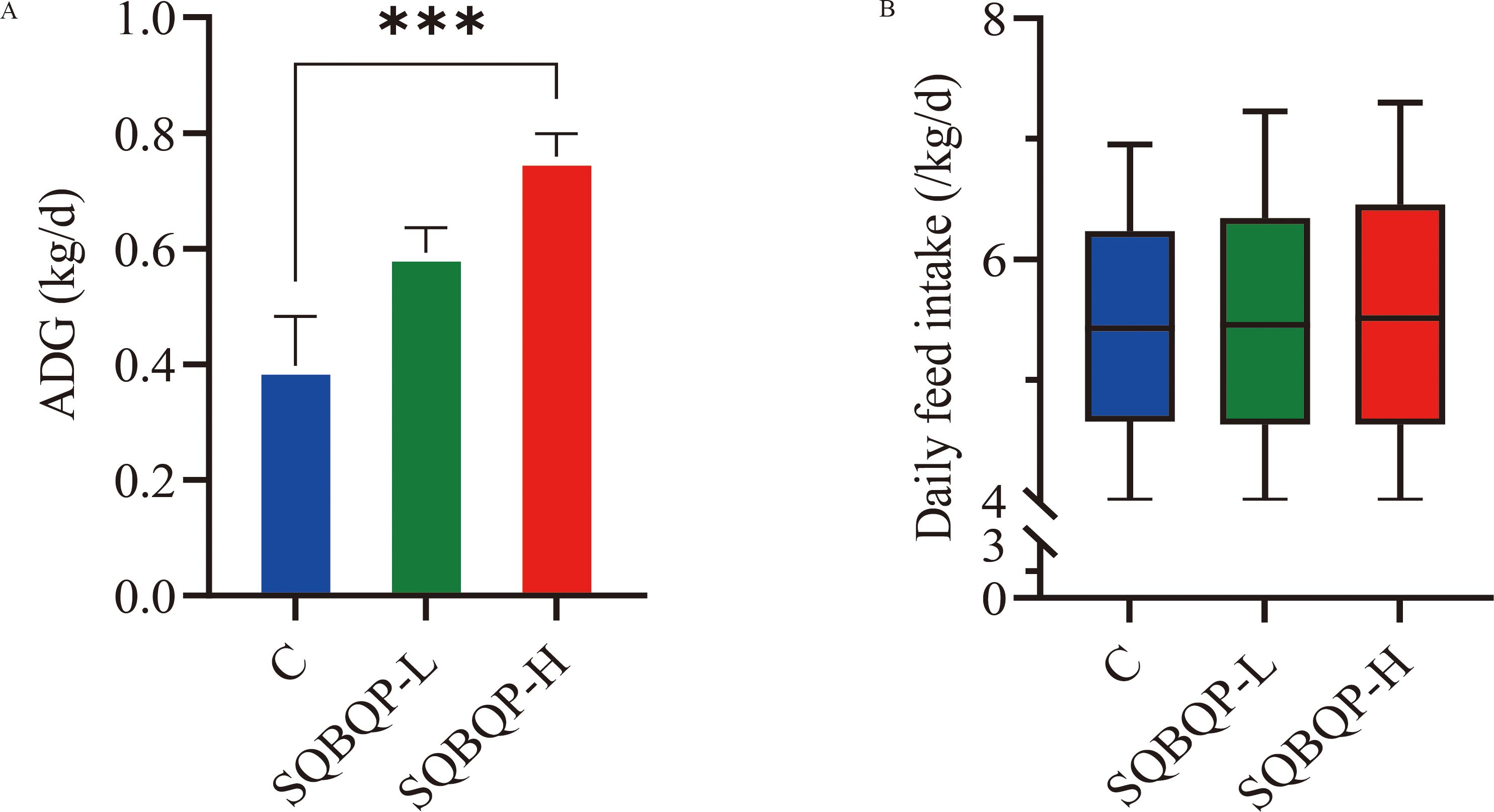
Figure 2. Effects of SQBQP on the average daily gain and daily feed intake of calves. (A) Average daily gain, (B) Daily feed intake. The date are expressed as SEM ± mean (n=35) and asterisks indicate significant differences (∗∗∗P<0.001).
3.3 Effects of the SQBQP on the antioxidant indexes of the serum of calves
By measuring the antioxidant indices such as GSH, CAT, and T-SOD in the calf serum, it was found that compared with the control group (C), the GSH content in the SQBQP-L group was significantly increased (P<0.01), and the T-AOC content in the SQBQP-H group was significantly increased (P<0.001). Both CAT and T-SOD showed an increasing trend, but the difference was not significant (P>0.05). MDA showed a decreasing trend, but the difference was not significant (P>0.05). These results indicated that SQBQP could improve the antioxidant capacity of calves (Figures 3A–E).
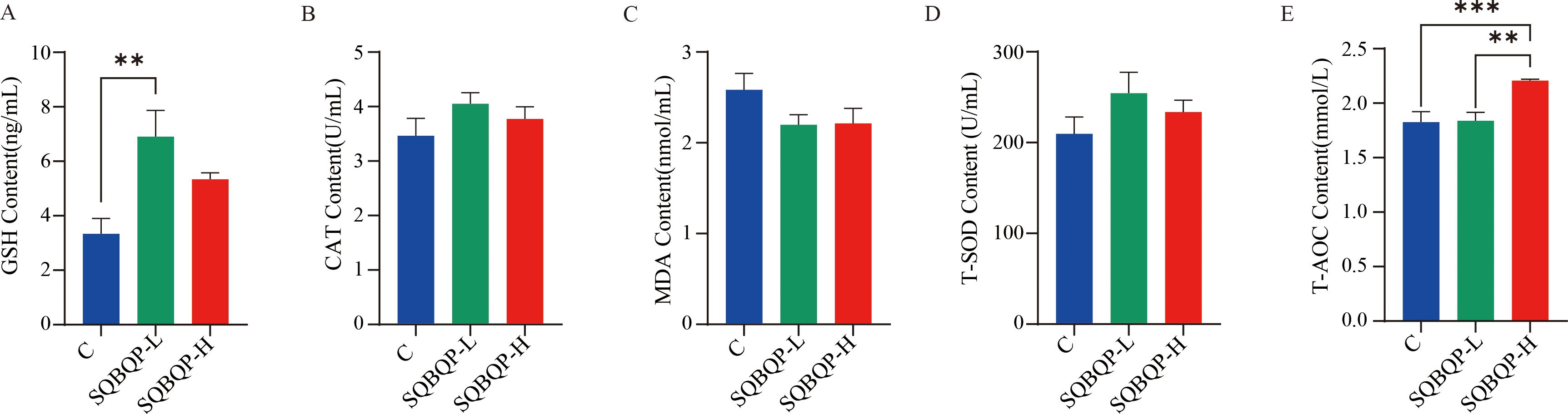
Figure 3. Effect of SQBQP on the serum antioxidants in calves. (A) GSH content, (B) CAT content, (C) MDA content, (D) T-SOD content, (E) T-AOC content. The data were expressed as SEM ± mean (n=6), and the asterisk indicated that there were significant differences according to one-way analysis of variance (∗∗P<0.01, ∗∗∗P<0.001).
3.4 Effects of the SQBQP on serum digestive enzymes of calves
The contents of AMS, lipase, cellulase, and protease digestive enzymes in the calf serum were measured. Compared to the control group (C), the contents of cellulase (P<0.05) and AMS (P<0.001) were significantly increased in the SQBQP-H group. The content of cellulase in SQBQP-L group had an increasing trend, but the difference was not significant (P>0.05). A significant decrease in the lipase content (P<0.05) was observed in both the SQBQP-L and SQBQP-H groups. The results demonstrated that SQBQP significantly enhanced the digestive capacity of calves (Figures 4A–D).
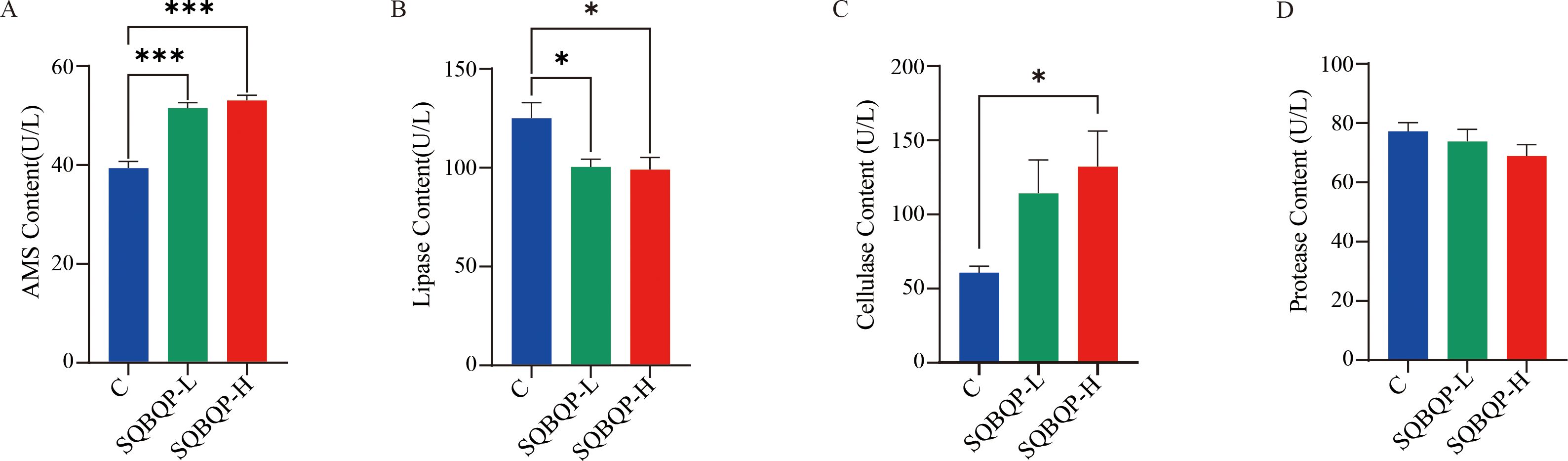
Figure 4. Effects of SQBQP on serum digestive enzymes in calves. (A) AMS content, (B) Lipase content, (C) Cellulase content, (D) Protease content. The data were expressed as the standard error of the mean SEM ± mean (n=6), with the asterisk indicating that there were significant differences according to one-way analysis of variance (∗P<0.05, ∗∗∗P<0.001).
3.5 Effects of SQBQP-H on the rumen and intestinal flora of calves
3.5.1 Effects of SQBQP-H on α and β diversity, phylum and genus level richness of rumen microbiota in calves
The total number of operational taxonomic units (OUT) in group C was 1265, while the total number of OUTs in group SQBQP-H was 1146. Principal coordinate analysis (PCoA) demonstrated a certain degree of similarity between the SQBQP-H group and the C group (Figures 5A, B). The analysis of α and β diversity accurately reflected the species and structural diversity of the rumen microbial community. Compared to the C group, there were no significant difference in the rumen microbial Chao1, ACE, Shannon, and Simpson indexes of calves in the SQBQP-H group (Figures 5C–F, P>0.05). Furthermore, no significant differences were observed between the two groups in the core microbiota at the phylum and genus levels (Figures 5G, H, P>0.05). However, it was observed that the SQBQP-H group exhibited a significant reduction in the relative abundance of Succinivibrio and Butyrivibrio at the genus level (Figure 5H, P<0.05). The detection of rumen microbial flora revealed that SQBQP down-regulated the relative abundance of certain microbial flora without affecting the core microbial flora in the rumen of calves. It resulted in maintaining a dynamic balance of rumen microbial flora and promoting forage digestion and absorption in the rumen.
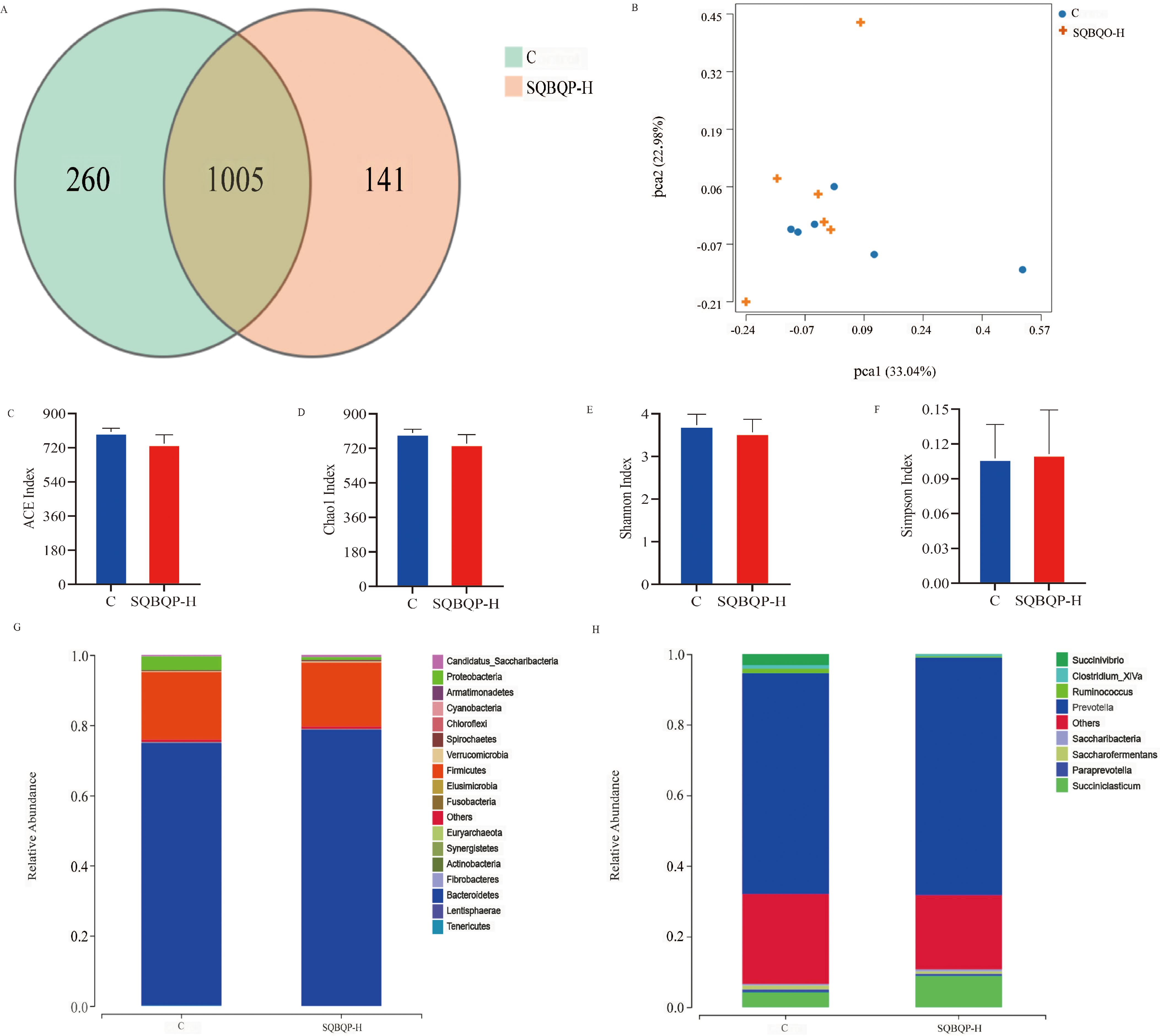
Figure 5. Effects of SQBQP-H on the rumen microflora composition in calves. (A) Venn diagram, (B) PCoA diagram, (C) Chao 1 index, (D) ACE index, (E) Shannon index, (F) Simpson index, (G) Phylum level, (H) Genus level (n=6).
3.5.2 Effects of SQBQP-H on α and β diversity, phylum and genus richness of intestinal flora in calves
The total number of operational taxonomic units (OUT) in group C was 1646, while the total number of OUTs in group SQBQP-H was 1688. Principal coordinate analysis (PCoA) demonstrated a certain degree of similarity between the SQBQP-H group and the C group (Figures 6A, B). The α and β diversity analysis of the two groups accurately reflected the species and structural diversity of the intestinal microbial community. Compared to the C group, there were no significant differences in the Chao1, ACE, Shannon, and Simpson indexes of rumen microorganisms in the SQBQP-H group (Figures 6C–F, P>0.05). However, the relative abundance of Proteobacteria, Actinobacteria, Candidatus_Saccharibacteria, Deinococcus_Thermus, and Cyanobacteria at the phylum level in TOP10 of the SQBQP-H group was significantly increased compared to the C group (Figure 6G, P<0.05). Conversely, the relative abundance of Tenericutes was significantly decreased (Figure 6G, P<0.05). At the genus level, the relative abundance of Succinivibrio in the TOP10 of the SQBQP-H group was significantly increased, while that of Oscillibacter was significantly decreased (Figure 6H, P<0.05). The results demonstrated that SQBQP-H not only maintained the steady state of rumen microbial flora in calves but also reduced the relative abundance of harmful intestinal flora. It increased the growth and reproduction of beneficial flora, promoted the digestion and absorption of nutrients, and improved the energy utilization and average daily gain.
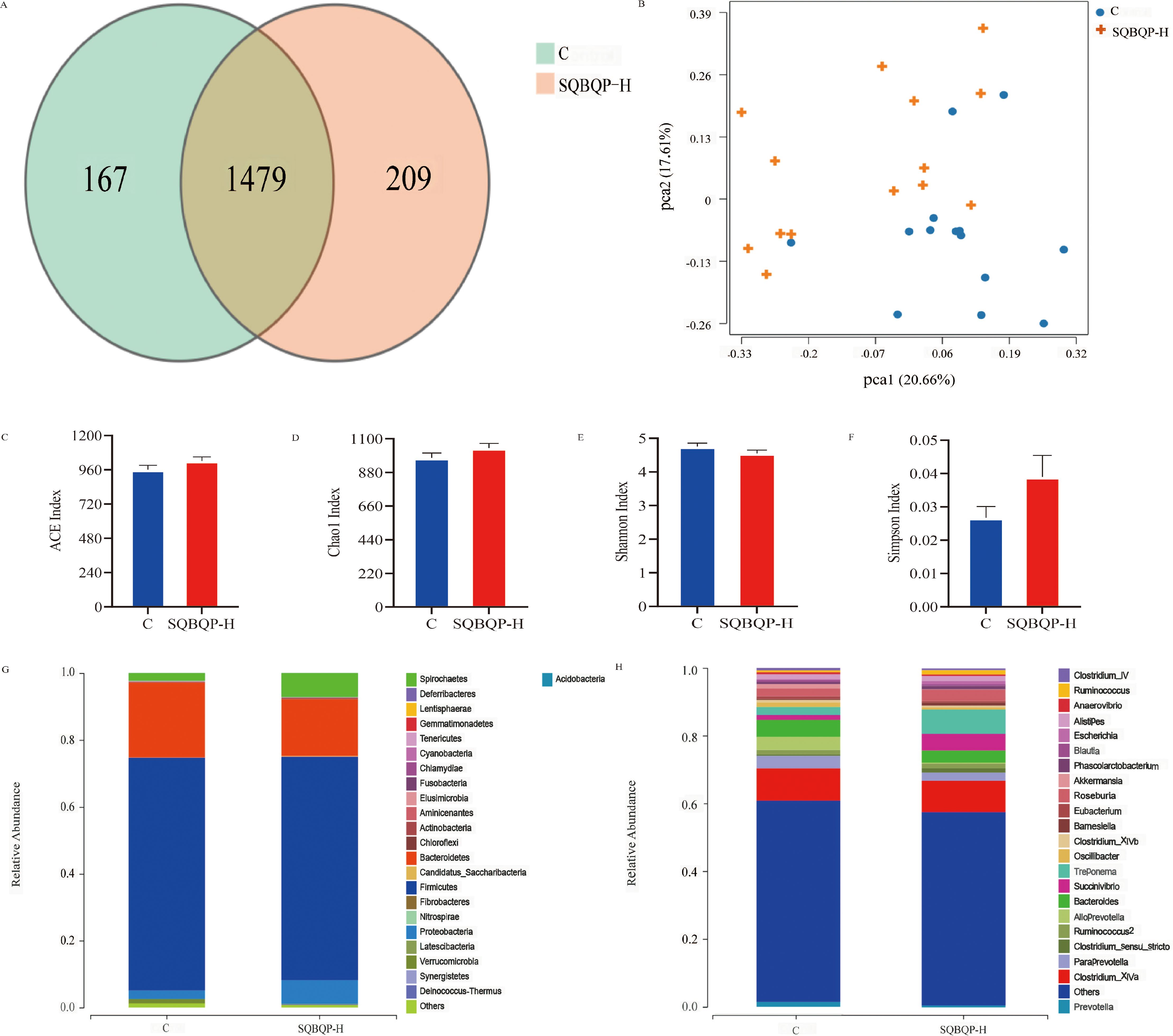
Figure 6. Effects of SQBQP-H on the composition of intestinal flora in calves. (A) Venn diagram, (B) PCoA diagram, (C) Chao 1 index, (D) ACE index, (E) Shannon index, (F) Simpson index, (G) Phylum level, (H) Genus level (n=14).
3.6 Effects of SQBQP-H on serum metabolites of calves
Partial least squares discriminant analysis (PLS-DA) revealed a clear distinction between the two groups (Figure 7A), indicating that SQBQP-H significantly altered the serum metabolite profile. The heat map demonstrated the differential metabolites aggregated between the control group (C) and the SQBQP-H group, revealing significant metabolic differences. Furthermore, the overall distribution of differential metabolites is illustrated in the volcano plot (Figure 7B). In both positive and negative ion modes, a total of 150 metabolites exhibited significant changes. Among these, 108 metabolites were up-regulated, such as (-)-Epigallocatechin, Trans-cinnamaldehyde, and Trans-picerol. Additionally, 42 metabolites were down-regulated, such as arginine and Glycine-DL-phenylalanine.
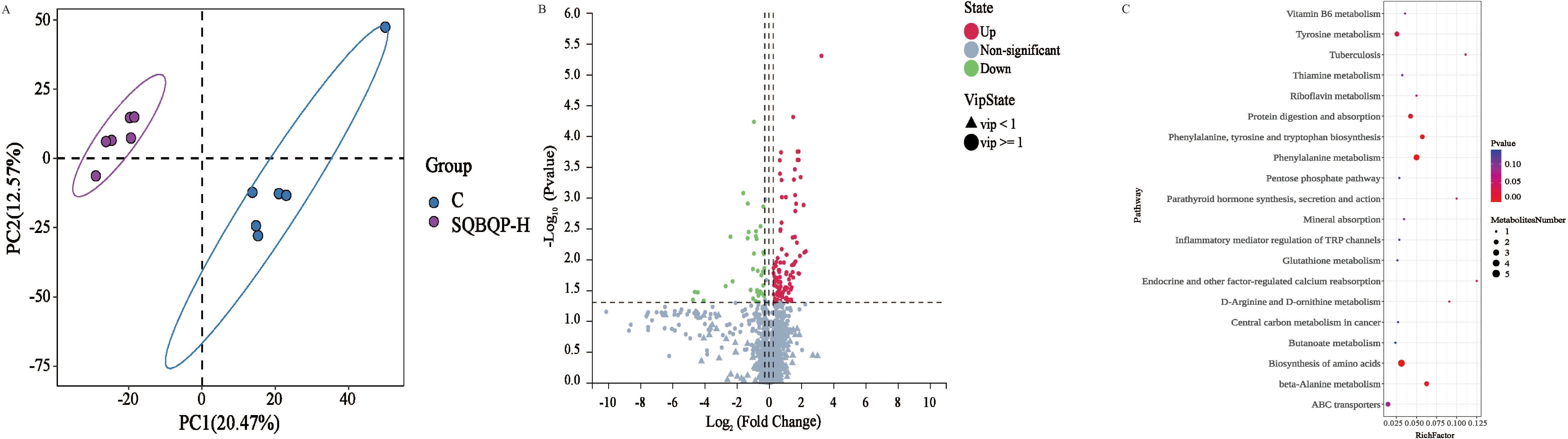
Figure 7. Effects of SQBQP-H on the serum metabolites in calves. (A) PLS-DA score plot of orthogonal partial least squares discriminant analysis of serum metabolites in the C and SQBQP-H groups, (B) Volcano map, each point represents a metabolite. The significantly upregulated metabolites were indicated by red dots, while the downregulated metabolites were indicated by green dots, and the metabolites with no significant difference were indicated by grey, (C) Kyoto Encyclopedia of Genes and Genomes (KEGG)-enriched serum differential metabolites. The color of the spot indicates the P value. The greater the degree of red, the more significant the enrichment. The size of the spots represents the number of different metabolites that are enriched (n=6).
The impact of SQBQP on the metabolic pathway of calves was investigated using KEGG pathway analysis. The top20 metabolic pathways of calves in the SQBQP-H group were found to be affected, as illustrated in Figure 7C. The analysis results revealed that SQBQP could regulate the serum metabolic pathways in calves including amino acid biosynthesis, β-alanine metabolism, phenylalanine, tyrosine and tryptophan biosynthesis, protein digestion and absorption, ABC transporters, and other metabolic pathways.
3.7 Correlation analysis between gastrointestinal flora and average daily gain and serum indexes
The Spearman correlation method was employed to further investigate the relationship between gastrointestinal flora and average daily gain (ADG) and serum indexes. Overall, the correlation between gastrointestinal microbiota and ADG and serum indexes exhibited changes following the SQBQP intervention in calves (Figures 8A, B). The SQBQP intervention influenced weight gain and serum antioxidant and digestive enzyme indexes by regulating the homeostasis of gastrointestinal microbiota in calves. The study found that the reduction in Succinivibrio in the rumen microbial community of calves following the SQBQP intervention was negatively correlated with ADG (R<-0.6, P<0.05) (Figure 8A). Additionally, in SQBQP-treated calves, Oscillibacter was negatively correlated with CAT, T-AOC (R<-0.6, P<0.05), ADG, GSH, and Cellulase (R<-0.5, P> 0.05). Furthermore, Oscillibacter was found to be positively correlated with lipase (R>0.6, P<0.05) (Figure 8B). The results demonstrated that SQBQP could significantly enhance the average daily gain and serum indices of calves by regulating the relative abundance of Succinivibrio in rumen bacteria and Oscillibacter in intestinal bacteria. This further indicated that SQBQP could be employed as a traditional Chinese medicine compound to promote the growth and development of calves.
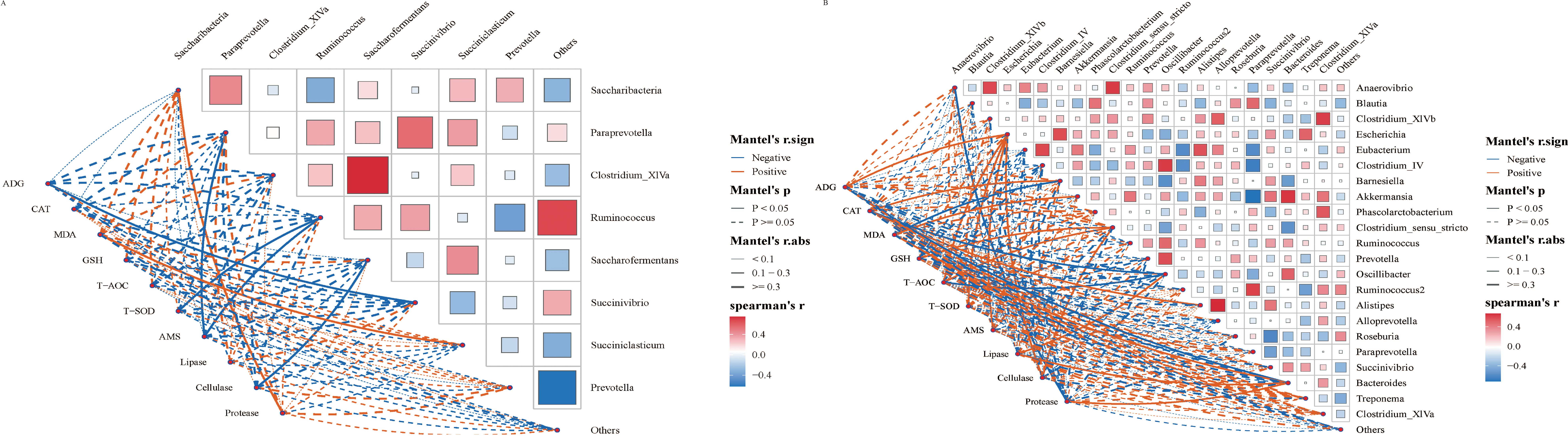
Figure 8. Correlation analysis between gastrointestinal flora and average daily gain and serum indexes. (A) Correlation between rumen flora and average daily gain and serum indicators, (B) Correlation between intestinal flora and average daily gain and serum indicators. The red and blue lines represent positive correlation and negative correlation, respectively. The thicker the line is, the stronger the correlation is. P<0.05 is the solid line.
3.8 Correlation analysis between rumen flora and intestinal flora
The correlation analysis results between the level of rumen flora and intestinal flora in calves were illustrated in Figure 9. It was demonstrated that Succinivibrio in the intestinal flora exhibited a positive correlation with Prevotella in the rumen flora (R>0.4, P>0.05). This data indicated a correlation between different bacteria in the gastrointestinal tract of calves, suggesting that one bacteria can indirectly influence the growth of another bacteria in the different internal environments.
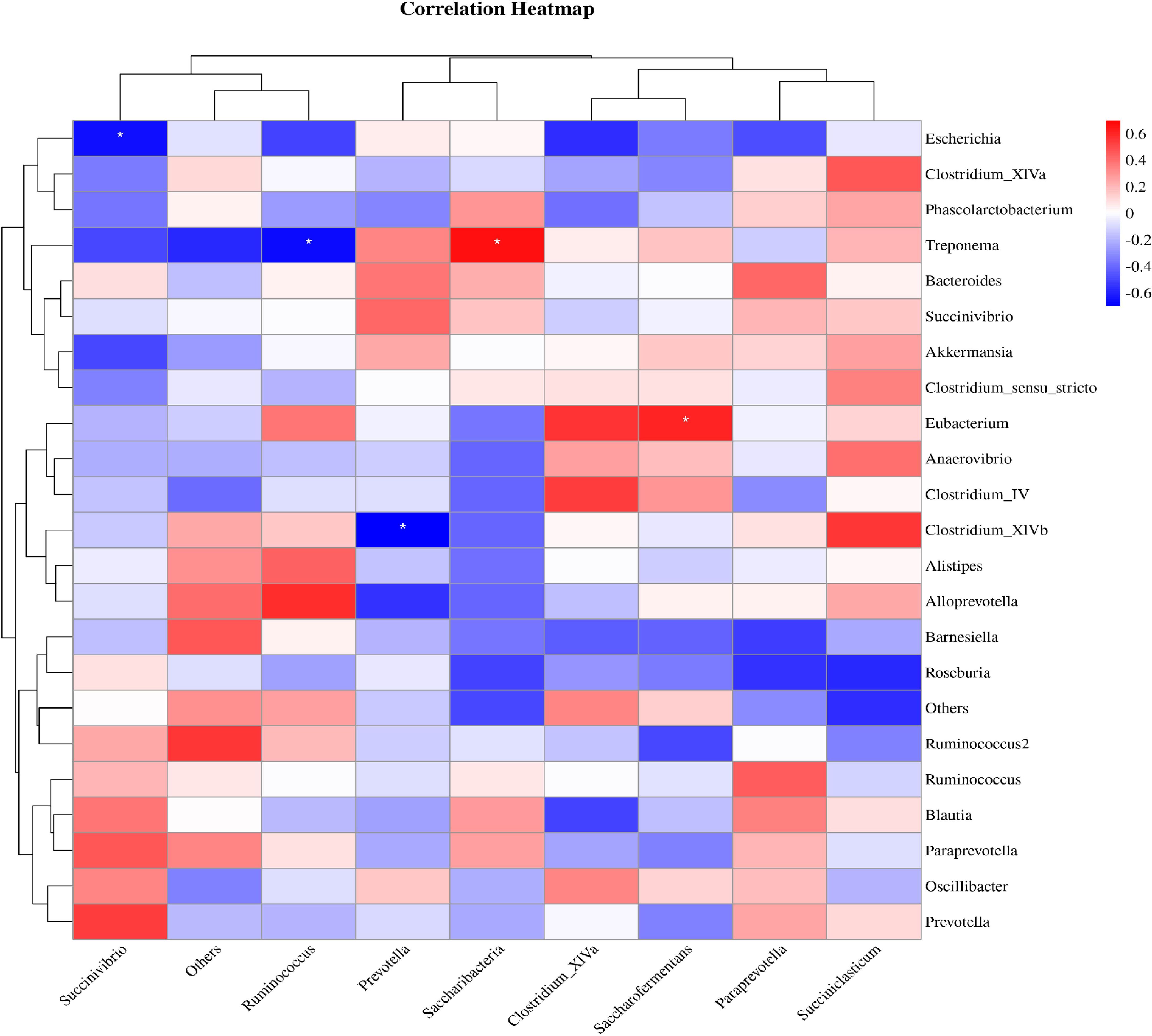
Figure 9. Correlation analysis between rumen flora and intestinal flora. Red indicates a positive correlation, blue indicates a negative correlation, and * represents P<0.05.
3.9 Correlation analysis between serum metabolites and average daily gain and serum indexes
The results of correlation analysis between serum metabolites, average daily gain (ADG), and serum indices showed that metabolites were correlated with weight gain and serum indices after the SQBQP intervention (Figure 10). This study found that the serum metabolite (-)-Epigallocatechin was positively correlated with T-AOC, AMS, cellulase, GSH, ADG, and CAT (R>0.58, P<0.05). Trans-cinnamaldehyde was found to be positively correlated with T-AOC, AMS, cellulase, GSH, T-SOD and ADG (R>0.58, P<0.05). Trans-piceatannol was positively correlated with T-AOC, cellulase, AMS, GSH, ADG, and CAT (R>0.6, P<0.05). P-hydroxyphenylacetic acid demonstrated a positive correlation with lipase, protease, and malondialdehyde (R>0.4, P<0.05) while exhibiting a negative correlation with ADG, AMS, T-AOC, and cellulase (R<-0.55, P<0.05). Gly-DL-phenylalanine was found to be negatively correlated with AMS, cellulase, T-SOD, ADG, T-AOC, and GSH (R<-0.6, P<0.05). The results indicated that SQBQP could significantly enhance ADG and serum indices of calves by regulating the content of various metabolites. Consequently, SQBQP can be employed as a traditional Chinese medicine compound to enhance the growth and development of calves.
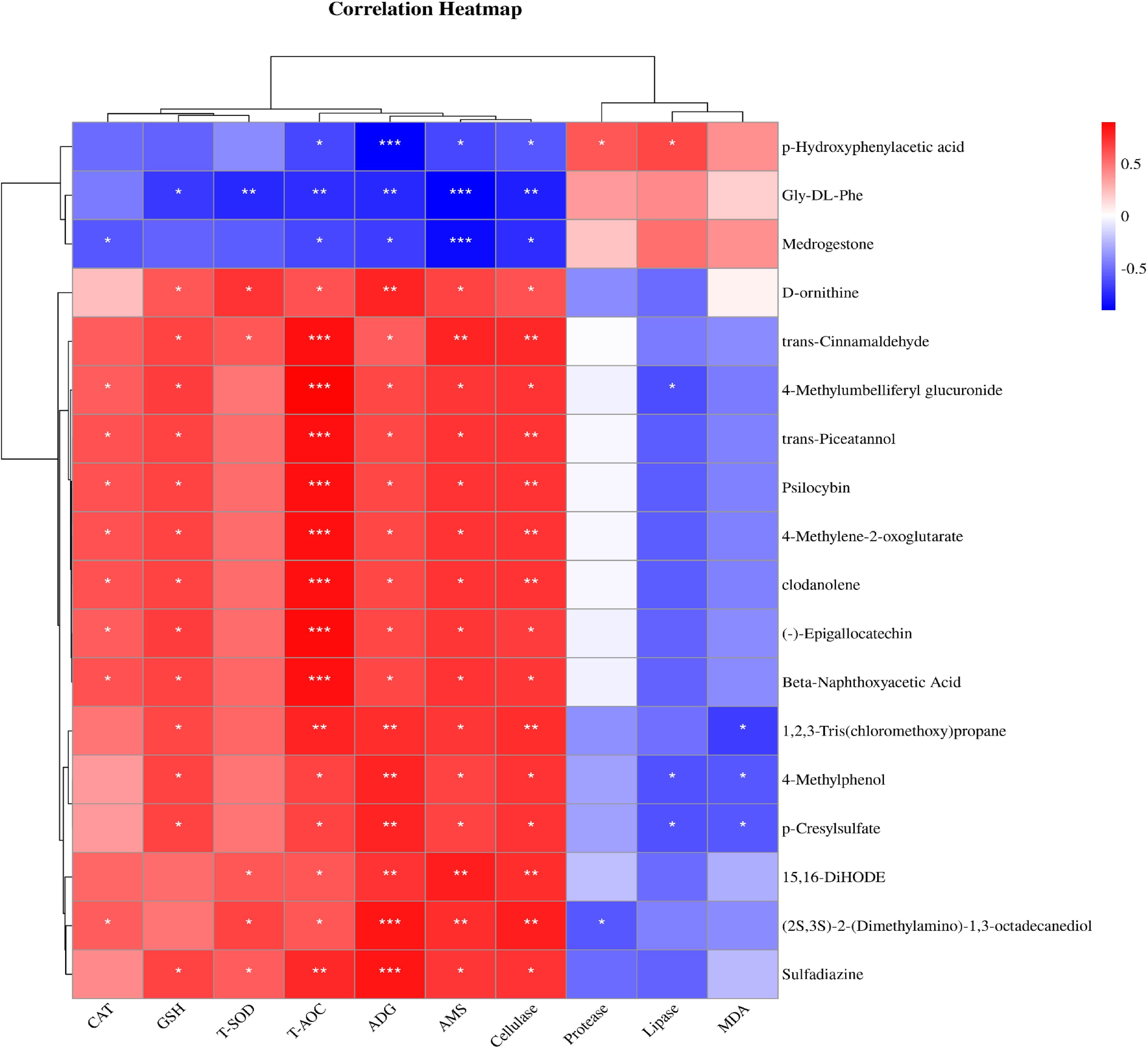
Figure 10. Correlation analysis between serum metabolites and average daily gain and serum indexes. Red represents positive correlation, green represents negative correlation, * represents P<0.05, ** represents P<0.01, *** represents P<0.001.
3.10 Correlation analysis between serum metabolites and gastrointestinal flora
The correlation analysis results between differential metabolites in the calf serum and the level of rumen flora (Figure 11A). Twenty differential metabolites were strongly correlated with rumen flora (R>0.6, P<0.05). Metabolites such as (-)-Epigallocatechin, Trans-cinnamaldehyde, and Trans-picerol were negatively correlated with Butyrivibrio. Additionally, P-hydroxyphenylacetic acid was positively correlated with Butyrivibrio. The correlation analysis results between serum differential metabolites and intestinal flora species levels in calves (Figure 11B). Twenty differential metabolites were strongly correlated with the intestinal flora (R>0.6, P<0.05). Metabolites such as (-)-Epigallocatechin, Trans-cinnamaldehyde, and Trans-piceatannol were negatively correlated with Oscillibacter_valericigenes in the intestinal flora. In contrast, Glycine-DL-phenylalanine was positively correlated with Oscillibacter_valericigenes. These results indicate that SQBQP can regulate the abundance of gastrointestinal microflora in calves and indirectly affect the expression of serum metabolites.
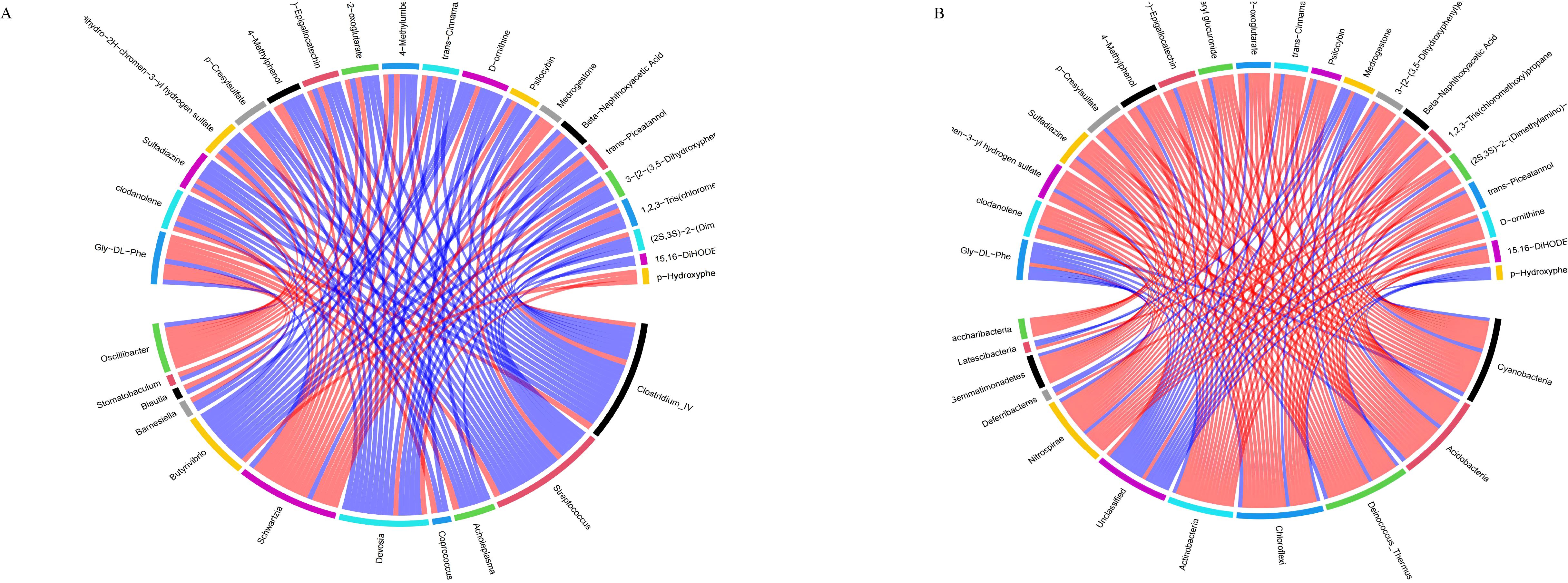
Figure 11. Correlation analysis between differential metabolites in serum and gastrointestinal flora. (A) correlation between serum differential metabolites and rumen flora, (B) Correlation between serum differential metabolites and intestinal flora; red and blue lines represent positive and negative correlations, respectively.
4 Discussion
In the cattle production, some factors, including transportation, extreme temperatures, driving, and vaccination stimuli, can induce the stress response in calves, which in turn reduces their disease resistance of calves. Therefore it is of great importance to screen TCM or compound for calf healthcare according to the requirements of the ‘antibiotic reduction action’ in animal husbandry production proposed by China, to ensure the health of calves and improve breeding efficiency. The advantages of TCM include the green, natural, and non-resistant properties. This study analyzed the effects of SQBQP on ADG, blood indexes, gastrointestinal microflora, and serum metabolites of 3-month-old calves based on clinical practice. LC-MS/MS was employed to identify and quantify the active components present in SQBQP. The results showed that the primary substances in SQBQP mainly includes polysaccharides, flavonoids, and organic acids. It was demonstrated that astragalus polysaccharide (Jia et al., 2019), codonopsis pilosula polysaccharide (Gao et al., 2020), atractylodes macrocephala polysaccharide (Li et al., 2022), and saposhnikovia divaricata polysaccharide (Fan et al., 2023) could enhance the antioxidant and antibacterial capabilities. Flavonoids have been shown to confer various health benefits, exhibiting antibacterial, anti-inflammatory, anti-tumor, and antioxidant effects (Tagousop et al., 2018; Calis et al., 2020; Zeng et al., 2022). Organic acids have the effects of anti-tumor, anti-inflammatory, and free radical scavenging (Wei et al., 2013; Erukainure et al., 2017). Although the components of TCM are complex and the mechanisms of action are diverse, the evidence of their curative effect is irrefutable. TCMs such as Radix Astragali and Rhizoma Atractylodis macrocephalae, are rich in polysaccharide active ingredients (Zeng et al., 2019; Chen et al., 2020). These ingredients significantly enhance the antioxidant capacity of calves and promote their growth and development, particularly in improving the average daily weight gain of calves.
The redox state is a significant indicator of the overall health status of the body. Animals, including livestock and poultry, can rely on a variety of antioxidant enzymes to regulate the redox balance. This study found that after the administration of SQBQP on calves, there was a significant increase in the serum GSH content, a increase tendency of CAT and T-SOD content, and a decrease tendency of MDA content. These findings indicate that SQBQP can enhance the antioxidant capacity of calves. The active ingredients in TCM, such as polysaccharides and flavonoids, can activate the antioxidant system, increase the activity of antioxidant enzymes, alleviate oxidative stress and enhance the body’s antioxidant capacity by increasing serum levels of CAT, GSH, T-SOD and T-AOC and decreasing levels of MDA. (Chen et al., 2019b; Zeng et al., 2019; Wang et al., 2022b). Some studies have shown that the flavonoid eupatilin has a range of pharmacological activities, including anticancer, anti-inflammatory, antioxidant, neuroprotective, anti-allergic, and cardioprotective properties (Lu et al., 2023, 2024). Other studies have shown that ferulic acid, a naturally occurring compound with antioxidant and antimicrobial properties, improves calf growth performance by enhancing the calf’s antioxidant capacity (Peña-Torres et al., 2021). The experiment results demonstrated that SQBQP enhanced the antioxidant capacity of calves and effectively alleviated the oxidative stress.
Digestive enzymes are produced by various tissues and organs of animals and transported to target organs to decompose and digest specific substances. Cellulose is produced in the rumen of calves to break down cellulose and provide energy. Proteases, amylases, and lipases are present in the gastrointestinal tract, where they can decompose and digest nutrients, provide energy for daily requirements. The results of this study indicate that the feeding calves with SQBQP can significantly increase the activity of cellulase and amylase, while the activity of lipase is significantly reduced. Some previous research has shown that adding sunflower shells to the diet can significantly increase the amylase activity in bovine serum (Kondrashova et al., 2021). In this study, SQBQP significantly increased serum amylase activity in calves but decreased lipase activity. This suggests that serum determination can be used instead of material determination in target organs, warranting further research. Adding Bacillus subtilis to piglet diets has been shown to significantly increase the activity of amylase and lipase in the ileum (Deng et al., 2020). Substituting corn with wheat in the diet of beef cattle significantly enhances the activity of amylase, lipase, protease, and cellulase in the gastrointestinal tract (Liu et al., 2016). Naringenin affects lipid metabolism in serum primarily by directly inhibiting pancreatic lipase activity (Liu et al., 2022). In contrast, ferulic acid may indirectly affect serum digestive enzyme function by improving intestinal health and enhancing antioxidant capacity (Gong et al., 2019). The findings demonstrate that SQBQP can enhance the digestive and absorptive capabilities of calves, thereby promoting their growth by influencing serum levels of digestive enzymes.
The complex microbial flora in the gastrointestinal tract of ruminants plays a crucial role in maintaining homeostasis, regulating energy metabolism, and activating intestinal immunity (Dill-McFarland et al., 2019). By regulating feed composition, it can enhance feed utilization and animal growth performance (Jiang et al., 2021; Nguse et al., 2022; Chen et al., 2019a). This study demonstrated that SQBQP significantly impacted the regulation of rumen and intestinal microbial communities in calves and notably increased their ADG. The dominant phyla in all test samples were Bacteroidetes, Firmicutes, and Proteobacteria. In the SQBQP-H group, the abundance of Firmicutes in rumen fluid and feces decreased, while the relative abundance of Bacteroidetes in the rumen increased. Furthermore, the overall relative abundance of Firmicutes and Bacteroidetes was higher than that in the control group (C group). Both Firmicutes and Bacteroidetes are key players in the fermentation of fiber and the degradation of carbohydrates, producing various cellulases to hydrolyze macromolecular compounds such as cellulose and sugar (Jami et al., 2014; Xie et al., 2023). This process promotes fat deposition, milk fat production, and the digestion and absorption of nutrients (de Melo et al., 2023; Song et al., 2023). SQBQP has been shown to maintain the homeostasis of the rumen environment in calves by regulating the relative abundance of Bacteroidetes and Firmicutes. The increased relative abundance of Proteobacteria in the intestinal microflora of the SQBQP-H group was linked to the degradation and fermentation of biopolymers. Additionally, the study revealed that Actinobacteria, Candidatus_Saccharibacteria, Deinococcus_Thermus, and Cyanobacteria were markedly elevated in the intestinal tract of the SQBQP-H group, with Actinobacteria playing a pivotal role in the biodegradation of lignocellulose (Briggs et al., 2021). The study also found that the relative abundance of Prevotella in the rumen of the SQBQP-H group was higher than that in the C group, whereas its abundance in the intestine was lower. Flavonoids have been shown to enhance the decomposition and utilization of plant polysaccharides by increasing the relative abundance of Prevotell (Ghimire et al., 2022). This suggests that the increased abundance of Prevotella in the rumen of calves in the SQBQP-H group facilitates feed digestion and utilization. Conversely, the relative abundance of Succinivibrio in the rumen bacteria of the SQBQP-H group was significantly reduced, while its abundance in the intestinal bacteria was considerably increased. This indicates a competitive relationship between Prevotella and Succinivibrio in the rumen, where an increase in Prevotella inhibits Succinivibrio growth, a dynamic reversed in the intestine. Succinivibrio could ferment a diverse range of sugars, primarily producing acetic acid and succinic acid, with minor amounts of formic acid and lactic acid (Liu et al., 2023c). Correlation analysis results suggest a positive relationship between the abundance of Prevotella in rumen flora and Succinivibrio in intestinal flora, indicating similar roles in the different organ environments. Furthermore, correlation analysis results between gastrointestinal flora, ADG of calves, and serum indices revealed that SQBQP intervention reduced the relative abundance of Succinivibrio in the rumen and significantly increased the ADG of calves. After SQBQP intervention, the relative abundance of Oscillibacter in the intestine was reduced, while the serum levels of CAT and T-AOC significantly increased, and the lipase levels decreased. These findings demonstrate that SQBQP exerts a positive regulatory effect on the rumen and intestinal flora of calves. An increase in the relative abundance of beneficial flora resulted in an enhanced feed digestion and absorption, subsequently improving ADG, antioxidant capacity, and the digestive efficiency in calves.
Alterations in the serum metabolite concentrations have a direct impact on the health status of animals (Guasch-Ferré et al., 2016; Wang et al., 2020; Wu et al., 2022). In this study, three down-regulated and 17 up-regulated differential serum metabolites were identified, exhibiting strong correlations with ADG and serum indicators. Among these metabolites, (-)-Epigallocatechin, Trans-cinnamaldehyde, and Trans-piceatannol were positively correlated with T-AOC, AMS, cellulase, GSH, ADG, and CAT. Conversely, hydroxyphenylacetic acid and Glycine-DL-phenylalanine were negatively correlated with these indicators. These findings suggest that alterations in the concentration of these metabolites may significantly enhance the ADG and serum indicators of calves. Numerous prospective studies have investigated the correlation between gastrointestinal microbiota and serum metabolites in both humans and animals (Zhang et al., 2021; Wang et al., 2022a). Our correlation analysis of rumen flora and serum metabolites revealed that (-)-Epigallocatechin, Trans-cinnamaldehyde, and Trans-piceatannol were negatively correlated with Butyrivibrio. Additionally, P-hydroxyphenylacetic acid was positively correlated with Butyrivibrio. Similarly, the analysis between intestinal flora and serum metabolism indicated that metabolites such as (-)-Epigallocatechin, Trans-cinnamaldehyde, and Trans-piceatannol were negatively correlated with Oscillibacter valericigenes in the intestinal flora, while Glycine-DL-phenylalanine was positively correlated with Oscillibacter valericigenes. This study found that differential metabolites showed a correlation with their corresponding gastrointestinal microbial flora in terms of significant up-regulation or down-regulation. Numerous studies have demonstrated that these metabolites can enhance animal growth performance and promote gastrointestinal health. For instance, β-naphthyloxyacetic acid is an auxin metabolite that stimulates mammalian guanylate cyclase activity. (-)-Epigallocatechin (Umme et al., 2021), Trans-cinnamaldehyde (Wang et al., 2022c), and Trans-pistol have been shown to possess anti-inflammatory and antioxidant properties, while Trans-cinnamaldehyde also exhibits antibacterial activity (Wu et al., 2020). D-ornithine, a key metabolite for energy conversion, has been observed to increase the body weight of young goats (Wang et al., 2023). SQBQP, a traditional Chinese medicine compound, has demonstrated antioxidant, anti-inflammatory, and antibacterial properties. This study found that SQBQP intervention in calves significantly up-regulate the content of these metabolites in calf serum, reduced the relative abundance of Butyrivibrio in rumen bacteria, and Oscillibacter_valericigenes in intestinal bacteria. Further studies revealed that SQBQP can up-regulate calf serum metabolites, affect various metabolic pathways, including amino acid biosynthesis, β-alanine metabolism, phenylalanine, tyrosine and tryptophan biosynthesis, protein digestion and absorption, and ABC transporter pathways. Amino acid biosynthesis plays a pivotal role in protein synthesis, energy provision, and participation in the metabolic pathways. Through conjugated and independent pathways, organisms can convert inorganic substances into amino acids, regulate by feedback inhibition and gene regulation. Prenatal nutrient supplementation significantly affected β-alanine metabolism in pregnant cows (Schalch Junior et al., 2022). Moreover, yam has been found to regulate abnormal fecal flora caused by diarrhea in rats and participate in β-alanine metabolism, as well as phenylalanine, tyrosine, and tryptophan biosynthesis (Zhang et al., 2023). Protein digestion and absorption, along with ABC transporters are the crucial metabolic pathways for calf gastrointestinal health (Huang et al., 2020). Tyrosine metabolism is also vital for the normal metabolism of perinatal dairy cows (Lopreiato et al., 2023; Zhao et al., 2024). Combining weight gain, serum partial indicators, microbiome, metabolomics, and their correlation analysis, it was confirmed that SQBQP promotes gastrointestinal health and increases the average daily gain of calves by regulating the abundance of gastrointestinal flora, serum metabolites, and metabolic pathways.
5 Conclusion
The study demonstrated that SQBQP could enhance the ADG of calves, improve serum indices, regulate the relative abundance of gastrointestinal flora, and influence the serum metabolite changes in calves. Further correlation analysis of the gastrointestinal microbiome and metabolomics revealed that SQBQP could enhance the body weight and antioxidant capacity of calves by regulating the relative abundance of gastrointestinal microbial flora, including Succinivibrio, Butyrivibrio, and Oscillibacter_valericigenes, and the levels of metabolites such as Trans-cinnamaldehyde, (-)-Epigallocatechin, Trans-piceatannol, P-hydroxyphenylacetic acid, and Glycine-DL-phenylalanine. Moreover, the study identified specific bacterial species, namely Butyrivibrio and Oscillibacter_valericigenes, and key metabolites including Trans-cinnamaldehyde, (-)-Epigallocatechin, Trans-piceatannol, P-hydroxyphenylacetic acid, and Glycine-DL-phenylalanine, which were found to promote gastrointestinal health in calves. These results provided the preliminary evidence of the potential of SQBQP as a functional feed additive. However, further systematic research and long-term monitoring are needed to fully evaluate the potential for the widespread use.
Data availability statement
The datasets used and analyzed during the current study are available from the corresponding author upon reasonable request.
Ethics statement
The animal study was approved by animal ethics committee of Gansu Agricultural University. The study was conducted in accordance with the local legislation and institutional requirements.
Author contributions
HY: Conceptualization, Data curation, Formal Analysis, Investigation, Methodology, Software, Writing – original draft, Writing – review & editing. JR: Data curation, Formal Analysis, Investigation, Methodology, Writing – review & editing. PJ: Formal Analysis, Funding acquisition, Project administration, Resources, Supervision, Validation, Writing – review & editing. XSZ: Data curation, Investigation, Methodology, Software, Writing – review & editing. ZM: Conceptualization, Data curation, Investigation, Methodology, Software, Writing – review & editing. CL: Data curation, Investigation, Methodology, Software, Writing – review & editing. NZ: Data curation, Investigation, Methodology, Software, Writing – review & editing. TM: Data curation, Investigation, Methodology, Software, Writing – review & editing. XPZ: Methodology, Project administration, Resources, Supervision, Validation, Writing – review & editing. YH: Funding acquisition, Project administration, Resources, Supervision, Visualization, Writing – review & editing. YW: Funding acquisition, Methodology, Project administration, Resources, Visualization, Writing – review & editing.
Funding
The author(s) declare that financial support was received for the research, authorship, and/or publication of this article. This research was supported by the Key R&D Projects of Ningxia Science and Technology Department (2023BCF01039), Gansu Provincial Technology Innovation Guidance Plan-East-West Cooperation (23CXNA0016), the National Natural Science Foundation of China (32260899), Gansu Agricultural University Youth Mentor Support Fund (GAU-QDFC-2021-04), by the China Agriculture Research System of MOF and MARA (CARS-37), by the Fuxi talent project of Gansu Agricultural University (Gaufx-02y05).
Acknowledgments
We thank all authors for their contributions and support.
Conflict of interest
Author XZ was employed by Zhangye Wanhe Animal Husbandry Industry Technology Development Co.
The authors declare that the research was conducted in the absence of any commercial or financial relationships that could be construed as a potential conflict of interest.
The author(s) declared that they were an editorial board member of Frontiers, at the time of submission. This had no impact on the peer review process and the final decision.
Publisher’s note
All claims expressed in this article are solely those of the authors and do not necessarily represent those of their affiliated organizations, or those of the publisher, the editors and the reviewers. Any product that may be evaluated in this article, or claim that may be made by its manufacturer, is not guaranteed or endorsed by the publisher.
References
Briggs, J. A., Grondin, J. M., Brumer, H. (2021). Communal living: glycan utilization by the human gut microbiota. Environ. Microbiol. 23, 15–35. doi: 10.1111/1462-2920.15317
Calis, Z., Mogulkoc, R., Baltaci, A. K. (2020). The roles of flavonols/flavonoids in neurodegeneration and neuroinflammation. Mini Rev. Med. Chem. 20, 1475–1488. doi: 10.2174/1389557519666190617150051
Che, Q. Y., Luo, T. T., Shi, J. H., He, Y. H., Xu, D. L. (2022). Mechanisms by which traditional Chinese medicines influence the intestinal flora and intestinal barrier. Front. Cell Infect. Microbiol. 12. doi: 10.3389/fcimb.2022.863779
Chen, S. Y., Deng, F., Jia, X., Liu, H., Zhang, G. W., Lai, S. J. (2019a). Gut microbiota profiling with differential tolerance against the reduced dietary fiber level in rabbits. Sci. Rep. 91, 1–9. doi: 10.1038/s41598-018-36534-6
Chen, F., Huang, G., Yang, Z., Hou, Y. (2019b). Antioxidant activity of Momordica charantia polysaccharide and its derivatives. Int. J. Biol. Macromol. 138, 673–680. doi: 10.1016/j.ijbiomac.2019.07.129
Chen, G., Li, Z. Q., Liu, S. L., Tang, T., Chen, Q. H., Yan, Z. M., et al. (2023). Fermented Chinese herbal medicine promoted growth performance, intestinal health, and regulated bacterial microbiota of weaned piglets. Anim. (Basel). 13, 476. doi: 10.3390/ani13030476
Chen, L., Long, R., Huang, G., Huang, H. (2020). Extraction and antioxidant activities in vivo of pumpkin polysaccharide. Ind. Crops Prod. 146, 112199. doi: 10.1016/j.indcrop.2020.112199
de Melo, H. S. A., Ítavo, L. C. V., de Castro, A. P., Ítavo, C. C. B. F., de Araújo Caldas, R., Mateus, R. G., et al. (2023). Effect of whole oilseeds in the diet on bacterial diversity in the solid fraction of the ruminal content of steers. Trop. Anim. Health Prod. 55, 32. doi: 10.1007/s11250-022-03442-x
Deng, B., Wu, J., Li, X., Zhang, C., Men, X., Xu, Z. (2020). Effects of Bacillus subtilis on growth performance, serum parameters, digestive enzyme, intestinal morphology, and colonic microbiota in piglets. AMB Express. 10, 212. doi: 10.1186/s13568-020-01150-z
Deters, E. L., Hansen, S. L. (2022). Long-distance transit alters the liver and skeletal muscle physiology of beef cattle. Animal 16, 100555. doi: 10.1016/j.animal.2022.100555
Dill-McFarland, K. A., Weimer, P. J., Breaker, J. D., Suen, G. (2019). Diet influences early microbiota development in dairy calves without long-term impacts on milk production. Appl. Environ. Microbiol. 85, e02141–e02118. doi: 10.1128/AEM.02141-18
Erukainure, O. L., Hafizur, R. M., Choudhary, M. I., Adhikari, A., Mesaik, A. M., Atolani, O., et al. (2017). Anti-diabetic effect of the ethyl acetate fraction of Clerodendrum volubile: protocatechuic acid suppresses phagocytic oxidative burst and modulates inflammatory cytokines. BioMed. Pharmacother. 86, 307–315. doi: 10.1016/j.biopha.2016.12.035
Fan, H. T., Sun, M., Li, J., Zhang, S. F., Tu, G. Z., Liu, K. C., et al. (2023). Structure characterization and immunomodulatory activity of a polysaccharide from Saposhnikoviae Radix. Int. J. Biol. Macromol. 233, 123502. doi: 10.1016/j.ijbiomac.2023.123502
Gao, Z. Z., Zhang, C., Jing, L. R., Feng, M., Li, R., Yang, Y. (2020). The structural characterization and immune modulation activities comparison of Codonopsis pilosula polysaccharide (CPPS) and selenizing CPPS (sCPPS) on mice in vitro and vivo. Int. J. Biol. Macromol. 160, 814–822. doi: 10.1016/j.ijbiomac.2020.05.149
Ghimire, S., Cady, N. M., Lehman, P., Peterson, S. R., Shahi, S. K., Rashid, F., et al. (2022). Dietary isoflavones alter gut microbiota and lipopolysaccharide biosynthesis to reduce inflammation. Gut Microbes 14, 2127446. doi: 10.1080/19490976.2022.2127446
Gong, L. X., Wang, H. N., Wang, T. X., Liu, Y. L., Wang, J., Sun, B. G. (2019). Feruloylated oligosaccharides modulate the gut microbiota in vitro via the combined actions of oligosaccharides and ferulic acid. J. Funct. Foods. 60, 103453. doi: 10.1016/j.jff.2019.103453
Guasch-Ferré, M., Hruby, A., Toledo, E., Clish, C. B., Martínez-González, M. A., Salas-Salvadó, J., et al. (2016). Metabolomics in prediabetes and diabetes: A systematic review and meta-analysis. Diabetes Care 39, 833–846. doi: 10.2337/dc15-2251
Hao, X. Y., Wang, P. J., Ren, Y. S., Liu, G. T., Zhang, J. X., Leury, B., et al. (2020). Effects of Astragalus membranaceous roots supplementation on growth performance, serum antioxidant and immune response in finishing lambs. Asian-Australas J. Anim. Sci. 33, 965–972. doi: 10.5713/ajas.19.0295
Harikrishnan, R., Devi, G., Doan, H. V., Tapingkae, W., Balasundaram, C., Arockiaraj, J., et al. (2022). Changes in immune gene expression, immune response, digestive enzymes -antioxidant status, and growth of catla (Catla catla) fed with Astragalus polysaccharides against Edwardsiellosis disease. Fish Shellfish Immunol. 121, 418–436. doi: 10.1016/j.fsi.2022.01.022
Huang, M. Z., Cui, D. A., Wu, X. H., Hui, W., Yan, Z. T., Ding, X. Z., et al. (2020). Serum metabolomics revealed the differential metabolic pathway in calves with severe clinical diarrhea symptoms. Anim. (Basel). 10, 769. doi: 10.3390/ani10050769
Huo, X. Q., Gu, Y., Zhang, Y. L. (2022). The discovery of multi-target compounds with anti-inflammation activity from traditional Chinese medicine by TCM-target effects relationship spectrum. J. Ethnopharmacol. 293, 115289. doi: 10.1016/j.jep.2022.115289
Jami, E., White, B. A., Mizrahi, I. (2014). Potential role of the bovine rumen microbiome in modulating milk composition and feed efficiency. PloS One 9, e85423. doi: 10.1371/journal.pone.0085423
Jia, N., Qiao, H. R., Zhu, W., Zhu, M. H., Meng, Q. H., Lu, Q., et al. (2019). Antioxidant, immunomodulatory, oxidative stress inhibitory, and iron supplementation effect of Astragalus membranaceus polysaccharide-iron (III) complex on iron-deficiency anemia mouse model. Int. J. Biol. Macromol. 132, 213–221. doi: 10.1016/j.ijbiomac.2019.03.196
Jiang, C. X., Ding, L. M., Dong, Q. M., Wang, X. J., Wei, H. Y., Hu, C. S., et al. (2021). Effects of root extracts of three traditional Chinese herbs as dietary supplements on dry matter intake, average daily gain, rumen fermentation, and ruminal microbiota in early weaned yak calves. Anim. Feed Sci. Technol. 278. doi: 10.1016/j.anifeedsci.2021.115002
Kholif, A. E., Olafadehan, O. A. (2021). Essential oils and phytogenic feed additives in ruminant diet: chemistry, ruminal microbiota and fermentation, feed utilization and productive performance. Phytochem. Rev. 206, 1–22. doi: 10.1007/s11101-021-09739-3
Kondrashova, K., Shejjda, E., Shajahmetova, E., Lebedev, S., Miroshnikov, S., Duskaev, G., et al. (2021). PSVII-22 Changes in biochemical composition of saliva and blood of cattle after sunflower husk included in the diet. J. Anim. Sci. 3, 338–339. doi: 10.1093/jas/skab235.622
Li, W. Y., Zhou, X. Y., Xu, S. W., Cao, N., Li, B. X., Chen, W. B., et al. (2022). Lipopolysaccharide-induced splenic ferroptosis in goslings was alleviated by polysaccharide of atractylodes macrocephala koidz associated with proinflammatory factors. Poult Sci. 101, 101725. doi: 10.1016/j.psj.2022.101725
Lin, Y. C., Chang, C. W., Wu, C. R. (2015). Anti-nociceptive, anti-inflammatory and toxicological evaluation of Fang-Ji-Huang-Qi-Tang in rodents. BMC Complem. Altern. Med. 15, 10. doi: 10.1186/s12906-015-0527-5
Liu, S., Han, Z., Trivett, A. L., Lin, H., Hannifin, S., Yang, D., et al. (2019). Cryptotanshinone has curative dual anti-proliferative and immunotherapeutic effects on mouse Lewis lung carcinoma. Cancer Immunol. Immunother. 68, 1059–1071. doi: 10.1007/s00262-019-02326-8
Liu, J., Pang, Y. Q., Yang, H. X., Huo, D. N., Liu, X. X., Chen, X. X. (2023a). Gut microbiota and urine metabolomics based exploration of coreopsis tinctoria nutt. tea on type 2 diabetic mellitus. Arab J. Chem. 16, 105037. doi: 10.1016/j.arabjc.2023.105037
Liu, S., Wang, K. J., Lin, S. Z., Zhang, Z. D., Cheng, M., Hu, S. S., et al. (2023b). Comparison of the effects between tannins extracted from different natural plants on growth performance, antioxidant capacity, immunity, and intestinal flora of broiler chickens. Antioxid. (Basel Switzerland). 12, 441. doi: 10.3390/antiox12020441
Liu, S., Xiao, G. X., Wang, Q., Tian, J. P., Feng, X., Zhang, Q. Y., et al. (2023c). Effects of dietary Astragalus membranaceus and Codonopsis pilosula extracts on growth performance, antioxidant capacity, immune status, and intestinal health in broilers. Front. Vet. Sci. 10. doi: 10.3389/fvets.2023.1302801
Liu, C. W., Zeng, H. Z., Jiang, R. G., Wang, K. F., Ouyang, J., Wen, S., et al. (2023d). Effects of mulberry leaf fu tea on the intestines and intestinal flora of goto-kakizaki type 2 diabetic rats. Foods 12, 4006. doi: 10.3390/foods12214006
Liu, H., Zhao, H., Che, J. J., Yao, W. J. (2022). Naringenin protects against hypertension by regulating lipid disorder and oxidative stress in a rat model. Kidney Blood Press Res. 47, 423–432. doi: 10.1159/000524172
Liu, Y. F., Zhao, H. B., Liu, X. M., You, W., Cheng, H. J., Wan, F. C., et al. (2016). Substitution of wheat for corn in beef cattle diets: digestibility, digestive enzyme activities, serum metabolite contents and ruminal fermentation. Asian-Australas J. Anim. Sci. 29, 1424–1431. doi: 10.5713/ajas.15.0866
Long, L. N., Kang, B. J., Jiang, Q., Chen, J. S. (2020). Effects of dietary Lycium barbarum polysaccharides on growth performance, digestive enzyme activities, antioxidant status, and immunity of broiler chickens. Poult Sci. 99, 744–751. doi: 10.1016/j.psj.2019.10.043
Lopreiato, V., Alharthi, A. S., Liang, Y. S., Elolimy, A. A., Bucktrout, R., Socha, M. T., et al. (2023). Influence of cobalt source, folic acid, and rumen-protected methionine on performance, metabolism, and liver tissue one-carbon metabolism biomarkers in peripartal holstein cows. Anim. (Basel). 13, 2107. doi: 10.3390/ani13132107
Lu, Y. L., Li, D., Huang, Y. Y., Sun, Y. Y., Zhou, H. M., Ye, F. R., et al. (2023). Pretreatment with eupatilin attenuates inflammation and coagulation in sepsis by suppressing JAK2/STAT3 signaling pathway. J. Inflammation Res. 16, 1027–1042. doi: 10.2147/jir.s393850
Lu, Y. Y., Min, Q. Q., Zhao, X. Y., Li, L., Zhao, G. J., Dong, J. Z. (2024). Eupatilin attenuates doxorubicin-induced cardiotoxicity by activating the PI3K-AKT signaling pathway in mice. Mol. Cell. Biochem. 479, 869–880. doi: 10.1007/s11010-023-04769-1
Ma, K. Y., Bai, T. T., Hu, P. F., Zhao, M., Xiu, Z., Surilige, et al. (2023). Sanwei sandalwood decoction improves the function of the gut microbiota in heart failure. Front. Microbiol. 14. doi: 10.3389/fmicb.2023.1236749
Nguse, M., Yang, Y., Fu, Z. L., Xu, J. C., Ma, L., Bu, D. P. (2022). Phyllanthus emblica (Amla) fruit powder as a supplement to improve preweaning dairy calves’ health: effect on antioxidant capacity, immune response, and gut bacterial diversity. Biol. (Basel). 11, 1753. doi: 10.3390/biology11121753
Peña-Torres, E. F., Dávila-Ramírez, J. L., Peña-Ramos, E. A., Valenzuela-Melendres, M., Pinelli-Saavedra, A., Avendaño-Reyes, L., et al. (2021). Effects of dietary ferulic acid on growth performance, carcass traits and meat quality of heifers. J. Sci. Food Agric. 101, 548–554. doi: 10.1002/jsfa.10666
Rabee, A. E., Mohamed M Ghandour, M., Sallam, A., Elwakeel, E. A., Mohammed, R. S., Sabra, E. A., et al. (2024). Rumen fermentation and microbiota in Shami goats fed on condensed tannins or herbal mixture. BMC Vet. Res. 20, 35. doi: 10.1186/s12917-024-03887-2
Salazar, L. F. L., Nero, L. A., Campos-Galvão, M. E. M., Cortinhas, C. S., Acedo, T. S., Tamassia, L. F. M., et al. (2019). Effect of selected feed additives to improve growth and health of dairy calves. PloS One 14, e0216066. doi: 10.1371/journal.pone.0216066
Schalch Junior, F. J., Polizel, G. H. G., Cançado, F. A. C. Q., Fernandes, A. C., Mortari, I., Pires, P. R. L., et al. (2022). Prenatal supplementation in beef cattle and its effects on plasma metabolome of dams and calves. Metabolites 12, 347. doi: 10.3390/metabo12040347
Shi, Q. Q., Li, X., Zhu, D. J., Jiang, J. J., Li, X. G. (2022). Comprehensive analysis of the antibacterial and anti-hepatoma activity of metabolites from jujube fruit. Food Biosci. 47. doi: 10.1016/j.fbio.2021.101511
Song, C., Zhang, T. T., Xu, D. H., Zhu, M. M., Mei, S. H., Zhou, B. J., et al. (2023). Impact of feeding dried distillers’ grains with solubles diet on microbiome and metabolome of ruminal and cecal contents in Guanling yellow cattle. Front. Microbiol. 14. doi: 10.3389/fmicb.2023.1171563
Swelum, A. A., Hashem, N. M., Abdelnour, S. A., Taha, A. E., Ohran, H., Khafaga, A. F., et al. (2021). Effects of phytogenic feed additives on the reproductive performance of animals. Saudi J. Biol. Sci. 28, 5816–5822. doi: 10.1016/j.sjbs.2021.06.045
Tagousop, C. N., Tamokou, J. D., Ekom, S. E., Ngnokam, D., Voutquenne-Nazabadioko, L. (2018). Antimicrobial activities of flavonoid glycosides from Graptophyllum granulosa and their mechanism of antibacterial action. BMC Complem. Altern. Med. 18, 252. doi: 10.1186/s12906-018-2321-7
Umme, H., Kandagalla, S., Sharath, B. S., Jyothsna, K., Manjunatha, H. (2021). Network pharmacology approach uncovering pathways involved in targeting Hsp90 through curcumin and epigallocatechin to control inflammation. Curr. Drug Discovery Technol. 18, 127–138. doi: 10.2174/1570163816666191210145652
Wang, M. W., Huang, H. J., Wang, L., Yang, H. S., He, S. W., Liu, F., et al. (2021). Herbal extract mixture modulates intestinal antioxidative capacity and microbiota in weaning piglets. Front. Microbiol. 12. doi: 10.3389/fmicb.2021.706758
Wang, Y., Liu, X. J., Chen, J. B., Cao, J. P., Li, X., Sun, C. D. (2022a). Citrus flavonoids and their antioxidant evaluation. Crit. Rev. Food Sci. Nutr. 62, 3833–3854. doi: 10.1080/10408398.2020.1870035
Wang, Y., Nan, X. M., Zhao, Y. G., Jiang, L. S., Wang, H., Zhang, F., et al. (2022b). Changes in the profile of fecal microbiota and metabolites as well as serum metabolites and proteome after dietary inulin supplementation in dairy cows with subclinical mastitis. Front. Microbiol. 13. doi: 10.3389/fmicb.2022.809139
Wang, H. X., Peng, Z. H., Sun, H. M. (2022c). Antifungal activities and mechanisms of trans-cinnamaldehyde and thymol against food-spoilage yeast Zygosaccharomyces rouxii. J. Food Sci. 87, 1197–1210. doi: 10.1111/1750-3841.16075
Wang, D. D., Tang, G. F., Li, Y. Y., Yu, J. J., Lei, X. J., Cao, Y. C., et al. (2023). Differences in serum metabolome profile explain individual variations in the growth performance of young goats. J. Proteomics. 288, 104982. doi: 10.1016/j.jprot.2023.104982
Wang, H. Y., Xu, R. Y., Zhang, H., Su, Y., Zhu, W. Y. (2020). Swine gut microbiota and its interaction with host nutrient metabolism. Anim. Nutr. 6, 410–420. doi: 10.1016/j.aninu.2020.10.002
Wei, G., Guan, Y., Yin, Y., Duan, J., Zhou, D., Zhu, Y. R., et al. (2013). Anti-inflammatory effect of protocatechuic aldehyde on myocardial ischemia/reperfusion injury in vivo and in vitro. Inflammation 36, 592–602. doi: 10.1007/s10753-012-9581-z
Wu, Z. A., Du, Z. Z., Tian, Y. Y., Liu, M., Zhu, K. L., Zhao, Y. F., et al. (2022). Inulin accelerates weight loss in obese mice by regulating gut microbiota and serum metabolites. Front. Nutr. 9. doi: 10.3389/fnut.2022.980382
Wu, J. R., Zhong, W. J., Chen, Z. D., Zhu, B. Q., Jiang, Y. Y., Wierzbicki, P. M. (2020). The protective impact of Trans-Cinnamaldehyde (TCA) against the IL-1b induced inflammation in vitro osteoarthritis model by regulating PI3K/AKT pathways. Folia Histochem. Cytobiol. 58, 264–271. doi: 10.5603/FHC.a2020.0025
Xie, K. L., Chang, S. H., Ning, J., Guo, Y. R., Zhang, C., Yan, T. T., et al. (2023). Dietary supplementation of Allium mongolicum modulates rumen-hindgut microbial community structure in Simmental calves. Front. Microbiol. 14. doi: 10.3389/fmicb.2023.1174740
Zeng, P., Li, J., Chen, Y. L., Zhang, L. J. (2019). The structures and biological functions of polysaccharides from traditional Chinese herbs. Prog. Mol. Biol. Transl. Sci. 163, 423–444. doi: 10.1016/bs.pmbts.2019.03.003
Zeng, X., Li, J. X., Lyu, X., Chen, J., Chen, X., Guo, S. (2022). Untargeted metabolomics reveals multiple phytometabolites in the agricultural waste materials and medicinal materials of codonopsis pilosula. Front. Plant Sci. 12. doi: 10.3389/fpls.2021.814011
Zhang, Q., Zhang, X., Wang, Q., Chen, S. Q. (2023). Dioscoreae rhizoma starch improves chronic diarrhea by regulating the gut microbiotas and fecal metabolome in rats. Food Sci. Nutr. 11, 6271–6287. doi: 10.1002/fsn3.3567
Zhang, Q., Zhang, Y. M., Zeng, L., Chen, G. W., Zhang, L., Liu, M. F. (2021). The role of gut microbiota and microbiota-related serum metabolites in the progression of diabetic kidney disease. Front. Pharmacol. 12. doi: 10.3389/fphar.2021.757508
Zhao, X. Y., Wang, Y. X., Wang, L. Y., Sun, S. Q., Li, C. Y., Zhang, X. W., et al. (2024). Differences of serum glucose and lipid metabolism and immune parameters and blood metabolomics regarding the transition cows in the antepartum and postpartum period. Front. Vet. Sci. 11. doi: 10.3389/fvets.2024.1347585
Zhu, D. P., Yu, B. (2018). Synthesis of the diverse glycosides in traditional Chinese medicine. Chin. J. Chem. 368, 681–691. doi: 10.1002/cjoc.201800156
Keywords: calves, average daily gain, serum indicators, gastrointestinal flora, serum metabolites, correlation analysis
Citation: Yang H, Ren J, Ji P, Zhang X, Mai Z, Li C, Zhao N, Ma T, Zhu X, Hua Y and Wei Y (2024) Investigating the regulatory effect of Shen Qi Bu Qi powder on the gastrointestinal flora and serum metabolites in calves. Front. Cell. Infect. Microbiol. 14:1443712. doi: 10.3389/fcimb.2024.1443712
Received: 04 June 2024; Accepted: 02 August 2024;
Published: 23 August 2024.
Edited by:
Jianzhao Liao, South China Agricultural University, ChinaReviewed by:
Tao Zhang, Beijing University of Agriculture, ChinaLei Liu, Hunan Agricultural University, China
Shuwei Dong, Yulin University, China
Copyright © 2024 Yang, Ren, Ji, Zhang, Mai, Li, Zhao, Ma, Zhu, Hua and Wei. This is an open-access article distributed under the terms of the Creative Commons Attribution License (CC BY). The use, distribution or reproduction in other forums is permitted, provided the original author(s) and the copyright owner(s) are credited and that the original publication in this journal is cited, in accordance with accepted academic practice. No use, distribution or reproduction is permitted which does not comply with these terms.
*Correspondence: Peng Ji, amlwQGdzYXUuZWR1LmNu; Yanming Wei, d2VpeW1AZ3NhdS5lZHUuY24=; Yongli Hua, aHVheW9uZ2xpMjAwNEAxNjMuY29t