- 1Infectious Diseases and Immunity in Global Health Program, Research Institute of McGill University Health Centre, Montréal, QC, Canada
- 2Department of Pathology and Microbiology, Faculty of Veterinary Medicine, Université de Montréal, Saint-Hyacinthe, QC, Canada
- 3Department of Microbiology and Immunology, McGill University, Montréal, QC, Canada
The study of extracellular vesicles has become an incredibly important field of study, but the inherent heterogeneity of these vesicles continues to make their study challenging. The genetic variability and well-documented protocols for the growth and vesicle isolation from Leishmania parasites provide a unique opportunity to compare the heterogeneity of different populations secreted by Leishmania clones. Leishmania mexicana was cultured on solid SDM agar plates and 8 clonal colonies were selected. The EVs collected from the liquid cultures of these 8 clones were assessed by NTA, TEM, and proteomic analysis. We found that all 8 clonal L. mexicana cultures were visually indistinguishable from each other and had similar growth rate, and these physical similarities extended to their EVs. However, proteomic analysis reveals that the EVs collected have unique protein profiles compared to each other and EVs isolated from a heterogeneous liquid culture of L. mexicana. We selected 3 clonal EVs for further mouse infection experiments and found that EVs from CL7 L. mexicana consistently caused reduced footpad swelling in C57BL6 mice footpads compared to EVs from CL1, CL8, and heterogenous L. mexicana. This trend was not observed when infecting Balb/C mice and C57BL6 with the parasites alone, with only CL1 L. mexicana causing significantly increased infection in Balb/c mice. Our results together show that EVs isolated from different clonal colonies of L. mexicana have distinct differences in protein cargo which can lead to varying outcomes on Leishmania infection. Further evaluation will be needed to determine the underlying mechanisms behind this and verify that differences observed in infectivity are directly caused by variations between our L. mexicana clones, especially genetic sequencing and immunoblotting to validate our results.
Introduction
Extracellular vesicles (EVs) are important vehicles for cell-cell communication and are constitutively secreted by both bacteria and eukaryotes (van Niel et al., 2018). They carry many biologically active macromolecules, ranging from nucleic acids to proteins, from their cell of origin and deliver these to a recipient cell to induce the desired physiological change (Thery et al., 2006). EVs have been of great interest due to their importance in intercellular communication, especially in the context of immune and tumor cell research and have recently been established to play an important role in pathogen-host interaction (Dong et al., 2019). Understanding the mechanisms of EV biogenesis and release/uptake have already been proven to be key to understanding human disease progression and diagnosis, but the study of EVs in pathogens and parasites is still a developing field of research.
Leishmania spp. are protozoan parasites that are the causative agents of the neglected tropical disease Leishmaniasis (Mann et al., 2021). These parasites specifically infect the macrophages of mammalian hosts and have evolved several mechanisms to evade the host immune response involving a variety of immune-modulating virulence factors (Olivier et al., 2012). Our lab has extensively studied these immunomodulatory virulence factors, especially GP63, and our research along with that of others in the field has revealed the important role of Leishmania EVs as vehicles for the delivery of Leishmania virulence factors to target mammalian host cells. Leishmania EVs are constitutively secreted and can also be induced by 37C temperature shock (Silverman et al., 2010a; Silverman et al., 2010b; Hassani et al., 2011). These EVs are loaded with common virulence factors like GP63 and EF1a and have profound immunomodulatory effects on mammalian macrophages. Leishmania EVs are also constitutively secreted within the midgut of its Sandfly vector and are therefore present during initial infection (Atayde et al., 2015). This co-inoculation causes heightened infection severity.
There are many distinct classes of EVs, including ectosomes, microvesicles, apoptotic bodies, exosomes, exomeres, and more (Colombo et al., 2014). Most recent EV research has focused on exosomes, a class of EVs ranging from 30 to 140nm in diameter. Exosomes originate from the endocytic pathway and first form as intraluminal vesicles within multivesicular bodies (MVB). Eventually, these vesicles are released once the MVB fuses with the plasma membrane. It was this distinct biogenesis pathway with directed cargo sorting that made exosomes particularly attractive to study as the main vehicles for intercellular communication. However, it has been shown that exosomes cannot be defined by consistent biomarkers or a conveniently distinct cellular origin and have instead begun to be classified based on their small size (sEVs) (Ramirez et al., 2018). This heterogeneity of EVs extends further to the subpopulations of EVs that can be secreted by not only different cell types but even individual cells within a single population (Willms et al., 2018).
The study of EV heterogeneity at the single cell level is a relatively recent development and often involve the use of expensive and/or custom-made microfluidic systems (Bordanaba-Florit et al., 2021). However, Leishmania spp. present an alternative novel method of shedding light on the variance of EVs. Leishmania can be grown in liquid cultures and can also be plated onto solid media. When plated, Leishmania forms clonal colonies like many bacteria cultures (Pacheco RS et al., 1990). By selecting clonal colonies of Leishmania, genetically identical liquid cultures of Leishmania can be expanded and EVs can be collected from these cultures. Following this methodology, 8 different clones of Leishmania mexicana, a species with great genetic heterogeneity (Berzunza-Cruz et al., 2000), were selected and the EVs released by these clones were collected using differential ultracentrifugation and compared based on their characteristics and effects on Leishmania infection. Sample enrichment was omitted in order to assess the entire population of clonal EVs rather than a single type of EVs alone.
Methods
Parasite culture and plating
L. mexicana m379 was cultured in 26C liquid Schneider’s Drosophila Medium (Gibco-BRL, Grand Island, NY) supplemented with 10% heat-inactivated standard fetal bovine serum (FBS) (Sigma-Aldrich), 2 mM L-glutamine, 100 U/ml penicillin, and 100 µl/ml streptomycin (complete Schneider’s). To make solid SDM agar, un-supplemented SDM was mixed with 2.5% agar solution and heat inactivated FBS in a ratio of 5:4:1, then this mixture was heated to 56C and poured into 100mm petri dishes to a thickness of 5mm and cooled. 2*10^6 log phase (3-4 day old liquid culture) L. mexicana promastigotes were then spread onto the SDM-agar plates and incubated at 26C for 10 days. Individual colonies were then carefully picked under magnification and cultured in liquid SDM. The resulting liquid cultures were then frozen after 3-4 days. Subsequent culturing of frozen L. mexicana clones was limited to a maximum of 2 passages.
EV purification
Purification of Leishmania EVs was performed according to standard methods for mammalian cells (Thery et al., 2006; Hassani et al., 2011). L. mexicana stationary phase promastigotes (day 6-8) were washed twice with PBS to remove SDM media and especially FBS, resuspended in vesicle-free RPMI 1640 medium (Life Technologies, Rockville, MD) without FBS and phenol red at a concentration of 2-4*10^8 promastigotes/ml, and incubated for 4 hours at 37°C for the release of EVs in the culture medium. At the end of a 4-hour incubation, the sample was centrifuged at 4000 RPM to remove parasites and large debris and the supernatant was filtered through a 0.45 µm syringe filter, followed by a 0.20 µm syringe filter. Next, EVs were pelleted by 2 rounds of 1-hour centrifugation at 100 000 g at 4C and resuspended in exosome buffer (137 mM NaCl, 20 mM Hepes, pH 7.5). Resulting EV samples were aliquoted and frozen at -80C, with each aliquot being thawed no more than twice. EV protein content was determined using Thermo Fisher MicroBCA kit according to manufacturer instructions.
Transmission electron microscopy
In vitro purified L. mexicana clone EVs were coated directly onto carbon-coated copper grids by dropping 5 ul on to the grid and allowing to dry for 2 minutes. The grids were then dried using the edge of a Kimwipe and fixed with 1% glutaraldehyde in 0.1 M sodium cacodylate buffer for 1 minute, washed 3 times in miliQ water, and stained with 1% uranyl acetate for 1 minute. After drying, grids were visualized in the FEI Tecnai G2 Spirit Twin 120 kV Cryo-TEM and images were taken with the Gatan Ultrascan 4000 4k x 4k CCD Camera System Model 895. Microscopy and sample preparation was performed at the Facility for Electron Microscopy Research at McGill University.
Nanoparticle tracking analysis
EV samples were initially diluted by 1:100 in exosome buffer and NTA was performed using the NanoSight NS500 (Malvern Panalytical). Samples were loaded into the machine at a minimum initial volume of 500 uL. Focus and camera level were then adjusted based on the first sample before standard script was run (camera level was unchanged for subsequent samples). Three 30 second videos were taken using the standard camera and analysis was performed using the NTA software to determine particle quantity and size as well as to perform statistical analysis.
LC/MS-MS
LC-MS/MS was performed as previously described at the Institute de Recherches Cliniques de Montréal (IRCM, University of Montreal, Canada) (Olivier et al., 2012; Atayde et al., 2015; Douanne et al., 2020). EVs were diluted in ultrapure water and protein content was determined using the microBCA protein assay kit (Thermo Scientific) to account for the high dilution ratio (1:20). Protein concentration was determined using an Infinite 200 Pro spectrophotometer (Tecan) and interpolation with a BSA standard curve. EV protein was then precipitated with 15% trichloroacetic acid (TCA)/acetone and processed for LC-MS/MS analysis, utilizing 3ug of protein from each sample of clonal EVs and Ht clonal EVs. After precipitation, in solution digestion was performed by the addition of trypsin at a ratio of 1:25 protease: protein. After an overnight incubation at 37°C, the reactions were quenched by the addition of formic acid to a final concentration of 0.2% and cleaned with C18 Zip Tip pipette tips (Millipore, Billerica, MA), before mass spectrometry analysis. Extracted peptides were injected onto a Zorbax Extended-C18 desalting column (Agilent, Santa Clara, CA) and subsequent chromatography was performed using the Easy-LLC II system (Proxeon Biosystems, Odense, Denmark) and coupled to the LTQ Orbitrap Velos (ThermoFisher Scientific, Bremen, Germany) equipped with a Proxeon nanoelectrospray ion source to perform mass spectrometry.
Proteomic analysis
Individual sample tandem mass spectrometry spectra were peak listed using the Distiller version 2.1.0.0 software (www.matrixscience.com/distiller) with the following parameters: minimum mass set to 500 Da, maximum mass set to 6,000 Da, no grouping of MS/MS spectra, precursor charge set to auto, minimum number of fragment ions set to 5, and peak picking parameters set at 1 for signal noise ratio and at 0.3 for correlation threshold. The peak-listed data was then searched against the NCBI database with the Mascot software version 2.3.02 (Matrix Science, London, UK). Mascot was set up to search the L. mexicana database (txid5665, 17 265 proteins) with a fragment ion mass tolerance of 0.50 Da and a parent ion tolerance of 1.5 Da. Carbamidomethyl was specified in both search engines as a fixed modification. Oxidation of methionine residues was specified in Mascot as a variable modification. Scaffold software version 4.0.6.1 (Proteome Software Inc., Portland, OR) was used to validate MS/MS peptide and protein identifications. Identification of peptides was accepted if it could be established at greater than 95.0% probability. Identification of proteins was accepted if it could be established at greater than 95.0% probability and contained at least 2 identified peptides. Proteins that contained similar peptides and could not be differentiated based on MS/MS analysis alone were grouped to satisfy the principles of parsimony. The final number of peptides per protein was represented by the average of the biological replicas after normalization to the total number of peptides. Unidentified proteins were removed from analysis. Unique and shared proteins were compared between different L. mexicana clones using UpSet (Lex et al., 2014). Gene ontology (GO) annotations were attributed using Panther by converting L. mexicana uniprot accession IDs to Leishmania major to functionally classify each protein (Mi et al., 2019). L. mexicana interacting EV proteins were mapped using STRING, searching against L. major as the STRING database lacks L. mexicana (Szklarczyk et al., 2019). The full STRING network was used with interactions determined based on evidence and a medium minimum interaction score (0.400). Clusters were generated using k-means clustering with 4 clusters and interactions were determined based on textmining, experiments, databases, co-expression, neighborhood, gene fusion, and co-occurence.
Mice infections
All experiments with mice were carried out in pathogen-free housing and in accordance with the regulations of the Canadian Council of Animal Care, approved by the McGill University Animal Care Committee. Female C57BL6 mice (6 to 8 weeks old) were infected in the right hind footpad with 5*106 stationary phase L. mexicana Ht promastigotes and 5 µg of in vitro purified L. mexicana Ht, CL1, 7, and 8 EVs. Female C57BL6 mice were also infected in the right hind footpad with 5*106 L. mexicana Ht, CL1, 7, and 8 promastigotes without vesicle co-inoculation. This infection was also performed using male BALB/c mice (6 to 8 weeks old). Disease progression was monitored by measuring footpad swelling weekly with a metric caliper, up to 10 weeks post-infection. At the end of each experiment, footpads were imaged. Statistical analysis was performed using Graphpad Prism 8.
Results
L. mexicana clone EVs have similar size and morphology
Without further enrichment, the EV samples collected for this study are comprised of multiple types of EVs, mostly a mixture of exosomes and microvesicles after filtration. NTA was performed on these EVs isolated from 8 different L. mexicana cultures grown from clonal colonies, as well as an Ht L. mexicana culture, using the NS500. The average concentration, mean particle diameter, and mode particle diameter were recorded (Table 1) and the particle size distribution of each EV population was graphed using the Nanosight NTA software (Figure 1). Particle concentration of each L. mexicana clone EV and the Ht EVs were compared, with CL2 having the lowest particle concentration and Ht having the highest (Figure 2A). However, no significant difference in particle concentration was found between the clones or Ht. Mean particle size (Figure 2B) and proportional size distribution (Figure 2C) were also compared, with no significant difference found in either comparison, though CL2 EVs had much lower mean and median particle size. TEM imaging was also performed to confirm successful EV isolation (Figure 1). Well defined EVs were identified in all samples, demonstrating a circular shape with the signature cupped form due to sample drying. The EVs were visually indistinguishable between samples.
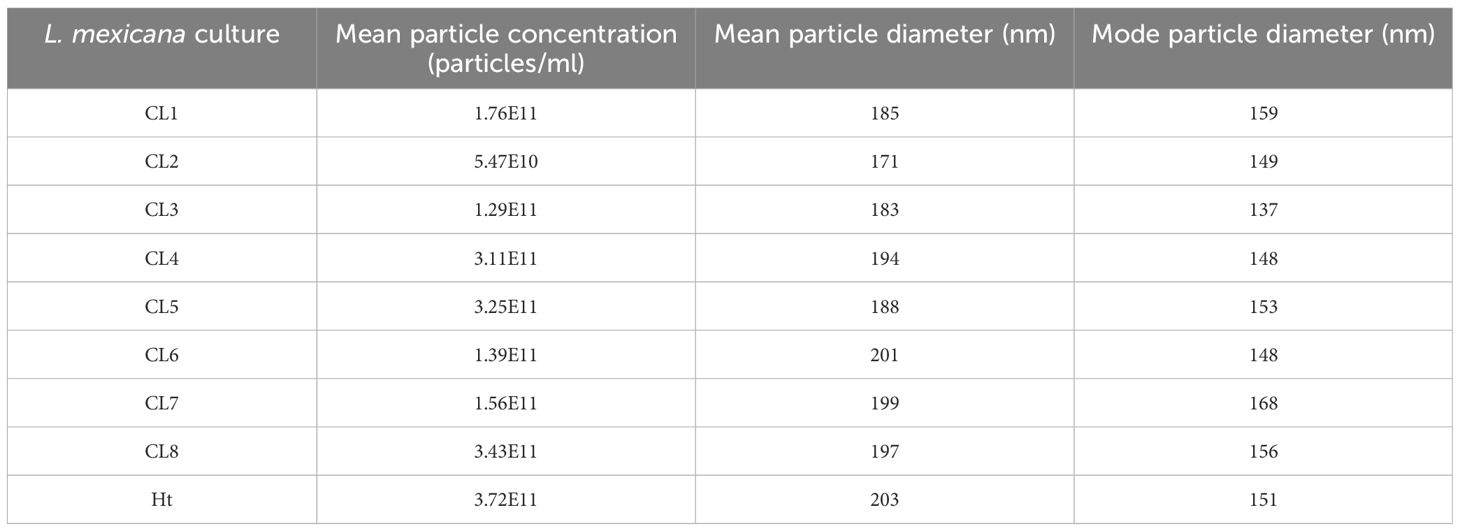
Table 1. Mean concentration, mean particle diameter, and mode particle diameter of EVs isolated from L. mexicana Clones and Ht. Stats were determined by the NS500 and NTA software from Malvern Panalytical from 3 separate videos for each sample.
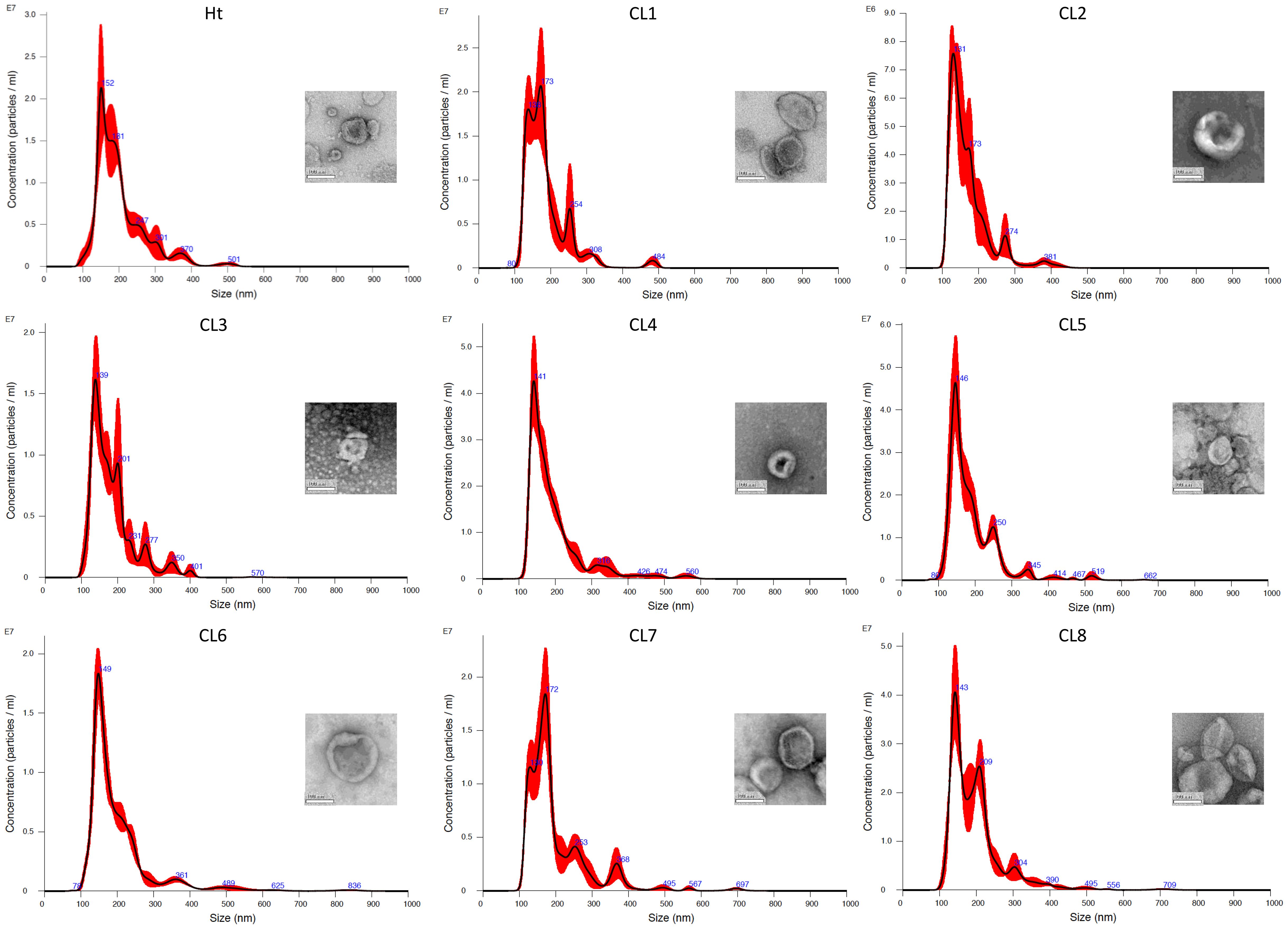
Figure 1. Particle diameter distribution of EVs isolated from each L. mexicana clone and Ht graphed by Malvern Panalytical NTA software. Example TEM images of the EVs are also provided, each having been taken at 30 000x magnification, with the scale bar representing 100nm.
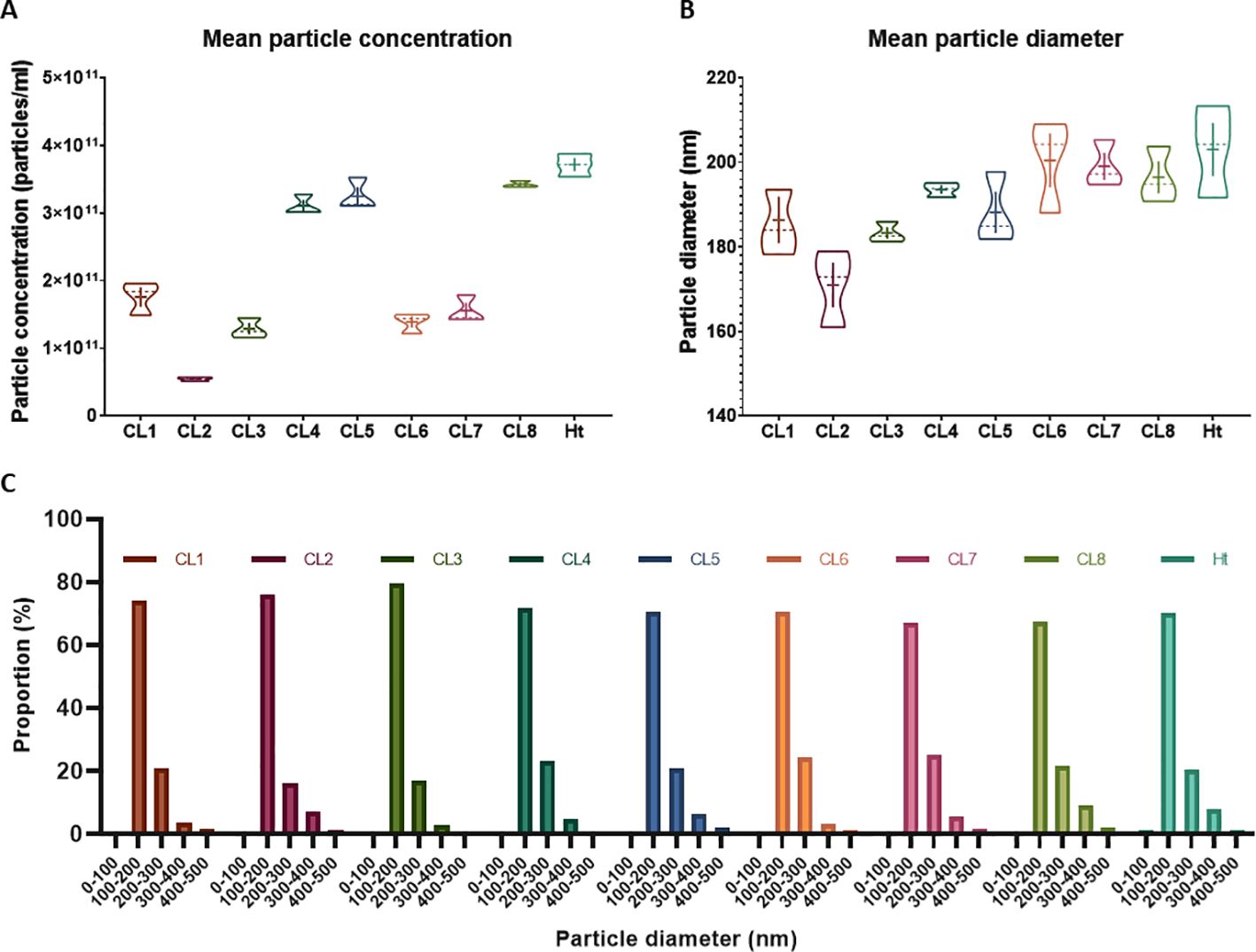
Figure 2. Mean particle concentration and size were compared between the L. mexicana clones and Ht L. mexicana using violin plots (A, B). The solid line represents the mean while the dotted line represents the median. Proportional size distribution of EVs isolated from each L. mexicana clone and Ht was also graphed and to compare overall variations between the EV populations (C). Particle concentration is represented as proportional to the total EV concentration of each sample.
EV protein content varies between different L. mexicana clones
A total of 253 proteins were identified across all 8 L. mexicana clonal EVs and the Ht EVs. These proteins were analyzed using Scaffold 4 (Supplementary Table 1). Unique and shared proteins between the 8 L. mexicana clones and Ht L. mexicana was visualized using an UpSet plot (Figure 3). Surprisingly few proteins were identified in EVs collected from the Ht L. mexicana culture, having the lowest number of individual proteins identified, followed by CL3 and 7. Ht L. mexicana and CL3 also had the most proteins uniquely absent, at 11 and 9 respectively, as well as sharing 15 proteins absent from all other groups (Table 2). This was unexpected considering that the Ht L. mexicana was the original progenitor strain, and thus we expected its EVs to contain most if not all proteins found in other clonal EVs. Surprisingly, despite CL7 having the next fewest individual proteins identified, there were only 3 proteins, long-chain-fatty-acid-CoA ligase, surface protein amastin, and amastin-like protein, uniquely absent from CL7 compared to all other groups, showing that there was strong overlap between CL7 and other clones altogether. CL8 EVs also uniquely lacked a cytochrome C protein, which is an important cell respiratory component.
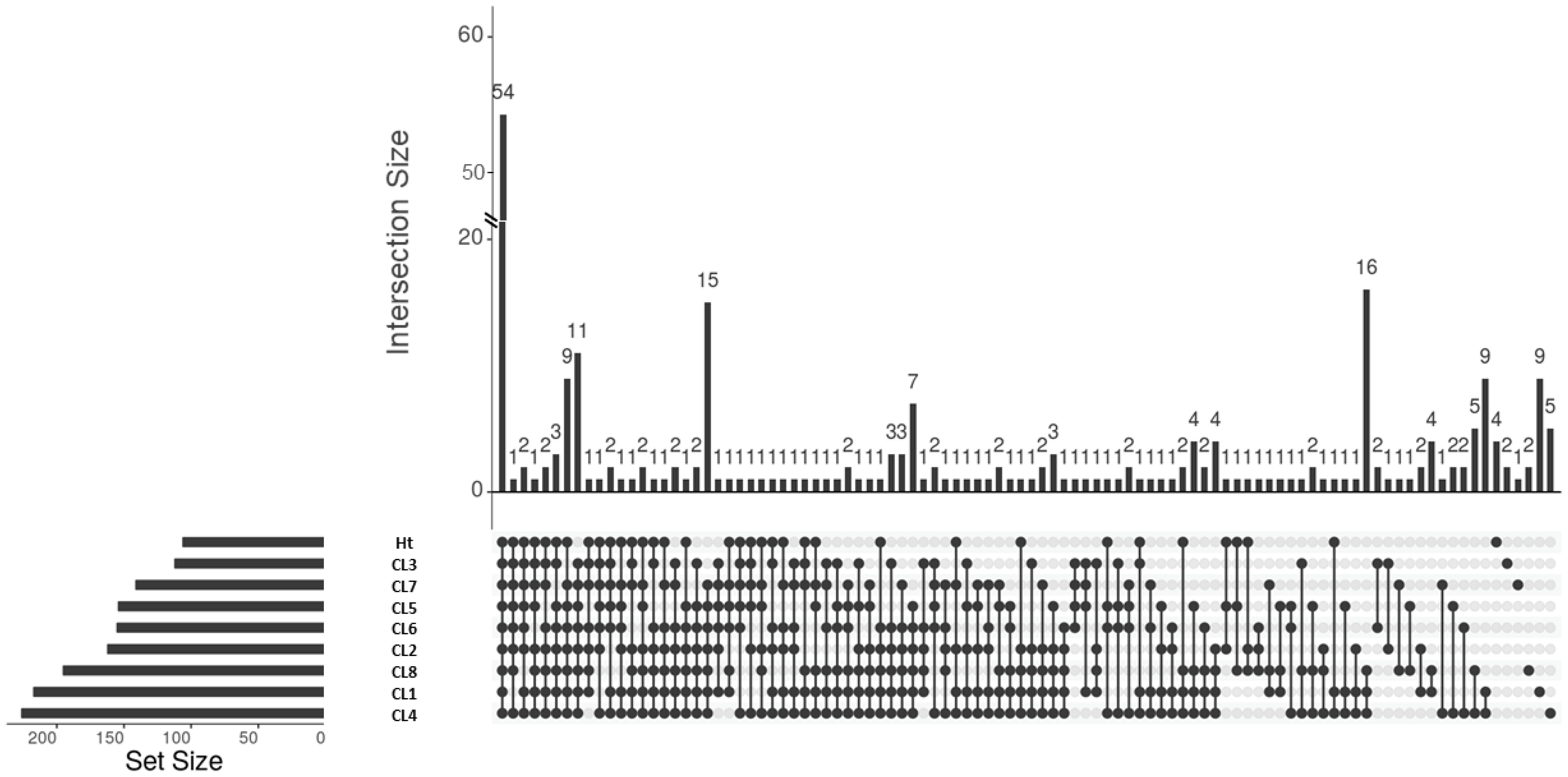
Figure 3. UpSet plot representing the shared and unique proteins present in L. mexicana clones and Ht. The legend is found below the plot, where each dot represents the presence of the proteins in that sample and the line representing that these proteins are shared between all samples with a dot. Samples are ordered from least unique proteins to most.
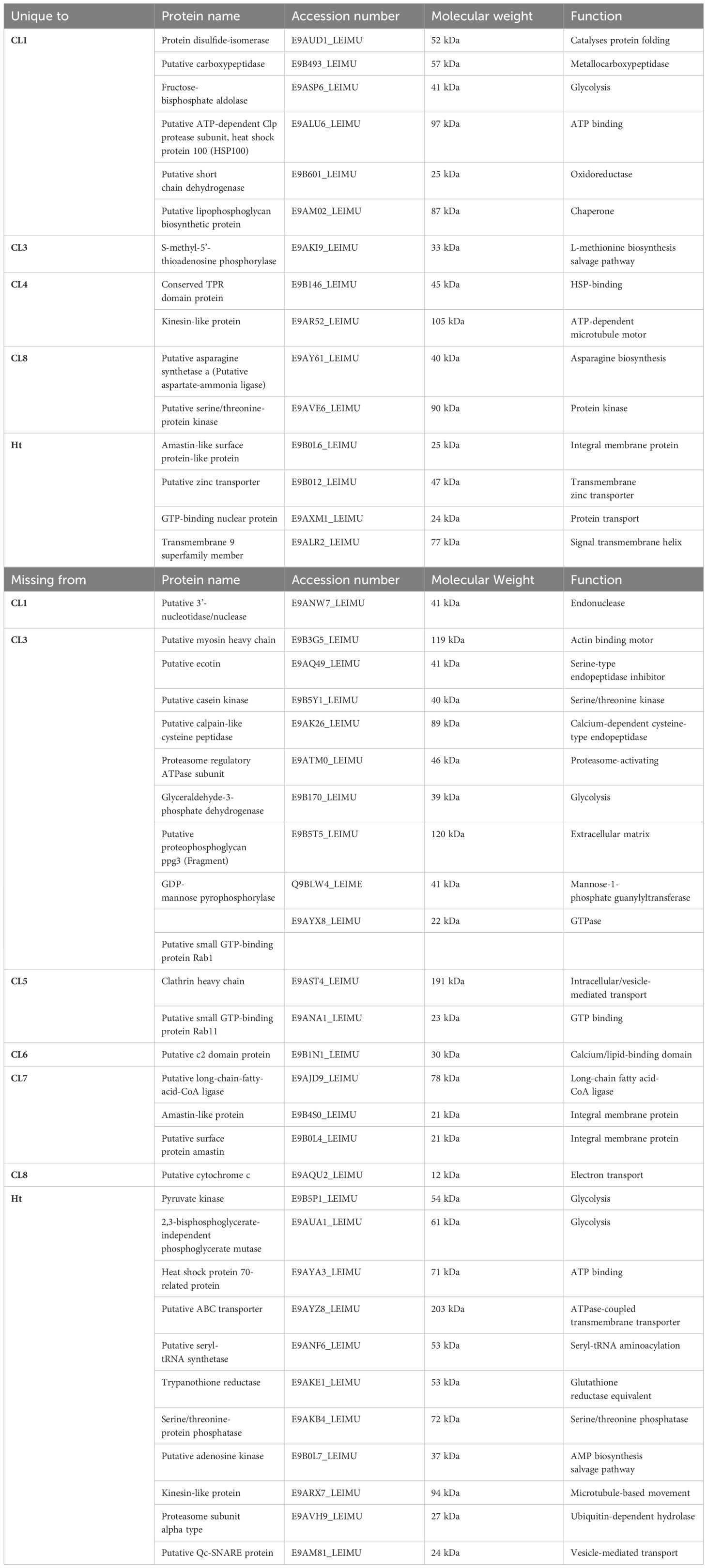
Table 2. Proteins categorized based on being present in only a single L. mexicana clone (Top) or missing from only a single L. mexicana clone (Bottom).
EV protein abundance varies between different L. mexicana clones
Overall protein abundance was measured in total spectra score using scaffold and compared between the 8 clones (Figure 4). From this comparison, it is evident that CL1, 4, and 8 EVs generally contain more proteins compared to other clones, with the blue line representing average total spectrum count of all clones. This higher protein abundance is especially clear when representing this data using a Z-score heatmap with all 253 identified proteins (Figure 5). To better display typically high abundance proteins found in Leishmania, a heatmap was also generated with the top 50 most abundant proteins across all clonal and Ht EVs, with this limited scope also allowing visualization of total spectra rather than Z-score (Figure 6). CL7 EVs contained the lowest amount of GP63, HSP70, HSP83-1, plasma membrane ATPase, EF1a, and EF2 (Figure 6; Supplementary Table 1). CL3 EVs had lower overall protein abundance but a significantly higher quantity of clathrin heavy chain. CL1 EVs contained the highest amount of actin, GP63, EF1a, and EF2 amongst the clones. Although Ht EVs contained fewer unique proteins, overall protein abundance was higher than EVs from other L. mexicana clones, having the highest amount of HSP70, GP63, EF1a, and tubulin beta. CL8 EVs contained the highest amount of receptor-type adenylate cyclase A. The combined low abundance and low number of proteins identified in CL3 EVs suggested that there was material loss during the processing or analysis of this sample, resulting in its exclusion from further analysis. However, CL3 EVs contained by far the most clathrin heavy chain despite their low overall protein abundance.
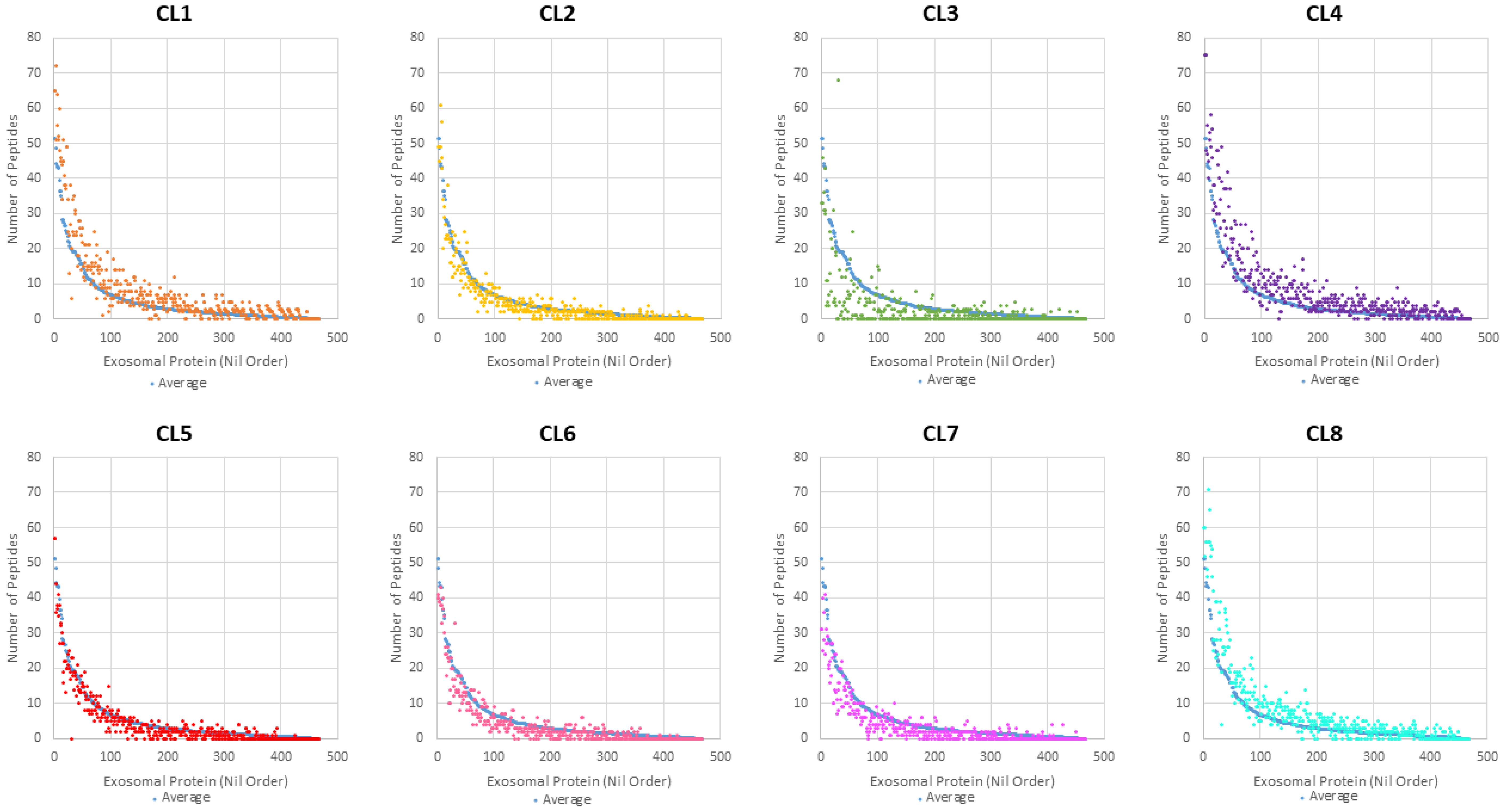
Figure 4. Overall protein abundance of each L. mexicana clone was plotted and compared, with the blue dots representing the baseline. Each dot represents an individual protein and the spectrum count associated with that protein.
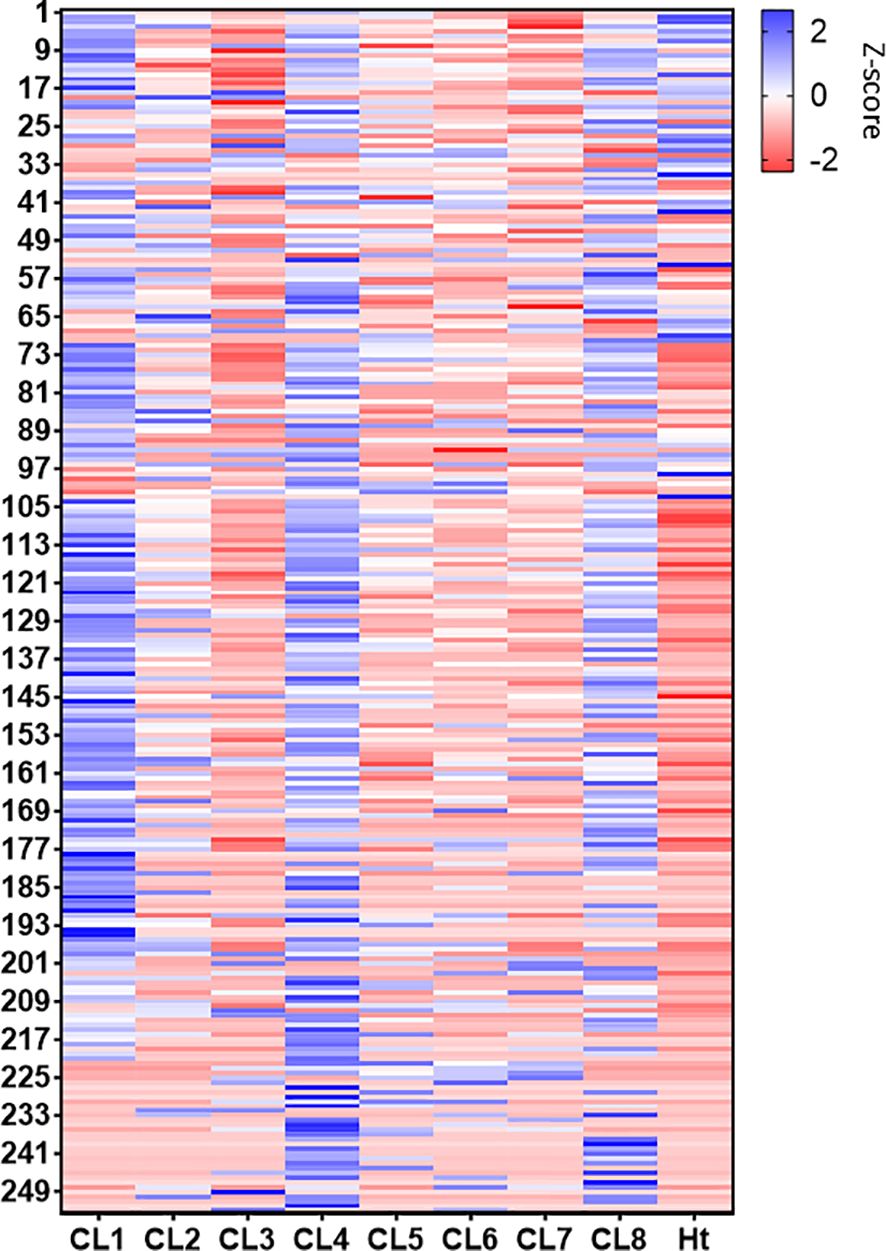
Figure 5. Heatmap generated representing all proteins identified (253) in the L. mexicana clones and Ht using Z-score. For protein identities, see Supplementary Materials (Supplementary Table 1).
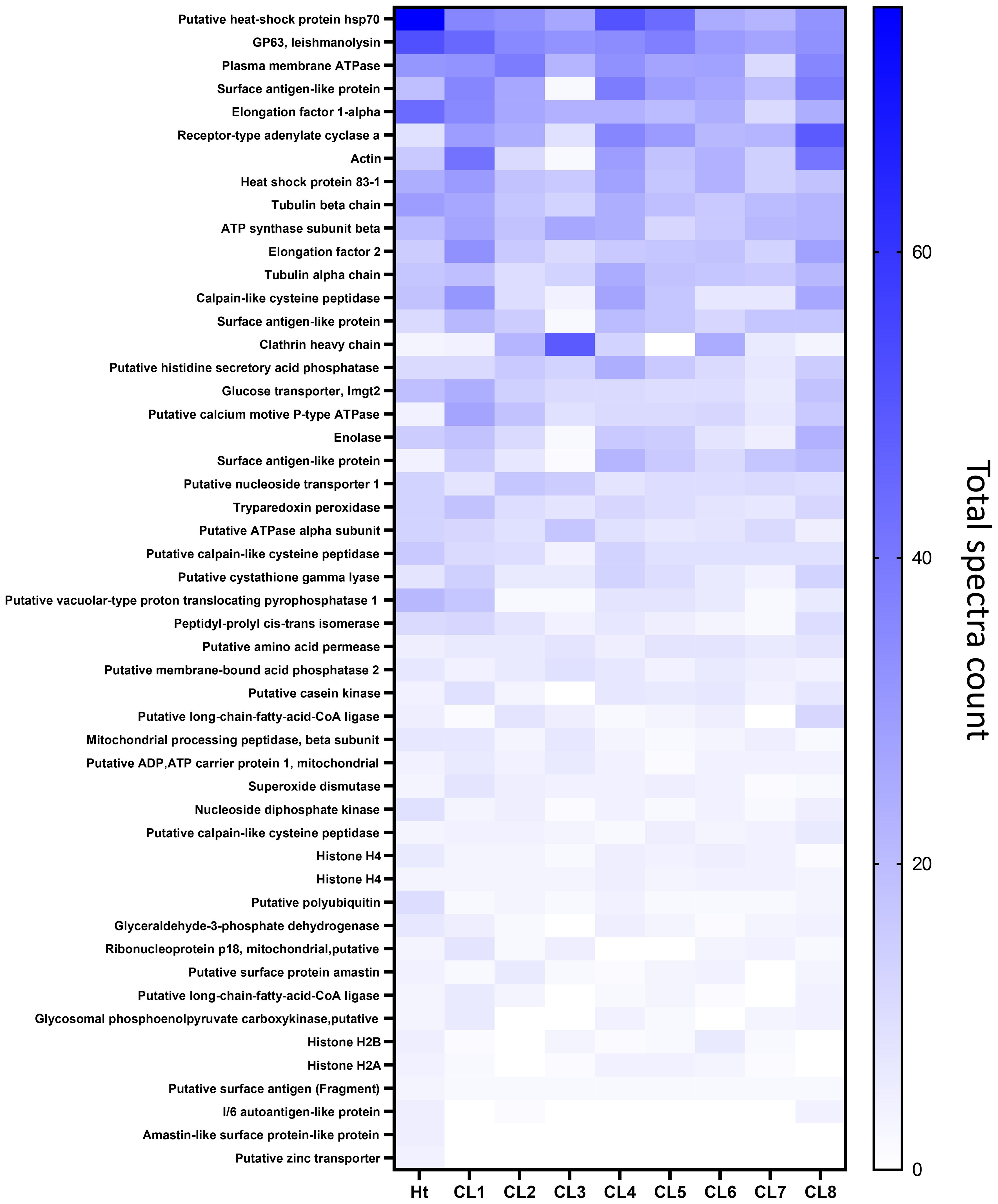
Figure 6. Heatmap generated representing the top 50 most abundant proteins identified in the L. mexicana clones and Ht using total spectrum count and ordered by Ht.
Overall protein function does not vary between clone EVs
Gene ontology (GO) annotations were attributed using Panther and represented using percentages for each clone and Ht L. mexicana EVs (Figure 7). Annotations for biological processes are visualized on the left and molecular function on the right. Cellular and metabolic processes were the most common biological processes annotated to L. mexicana EVs. Although localization and establishment of localization appeared as distinct categories during the GO search, these categories overlapped entirely. When searching by molecular function, there was strong overlap with the very broad molecular function category which encompassed almost all proteins with a hypothesized mechanism of action. There was no significant variation in EV protein content when looking at these broader GO terms between the different clones compared. However, proteins related to enzyme regulation were entirely absent in CL7 EVs. The 51 shared proteins between all groups are visualized in a STRING diagram to show hypothesized protein interactions (Figure 8; Table 3). We found 4 functional protein clusters shared between all L. mexicana clones and Ht L. mexicana: purine ribonucleoside triphosphate metabolism (Atayde et al., 2015), GTP-binding (Silverman et al., 2010a), one-carbon metabolism (Mann et al., 2021), and stress response (Dong et al., 2019). Several Leishmania virulence factors, like GP63, EF1α, and enolase, fall under the purine ribonucleoside triphosphate metabolism cluster, while important cytoskeletal proteins, like actin and tubulin, fall under the GTP-binding cluster.
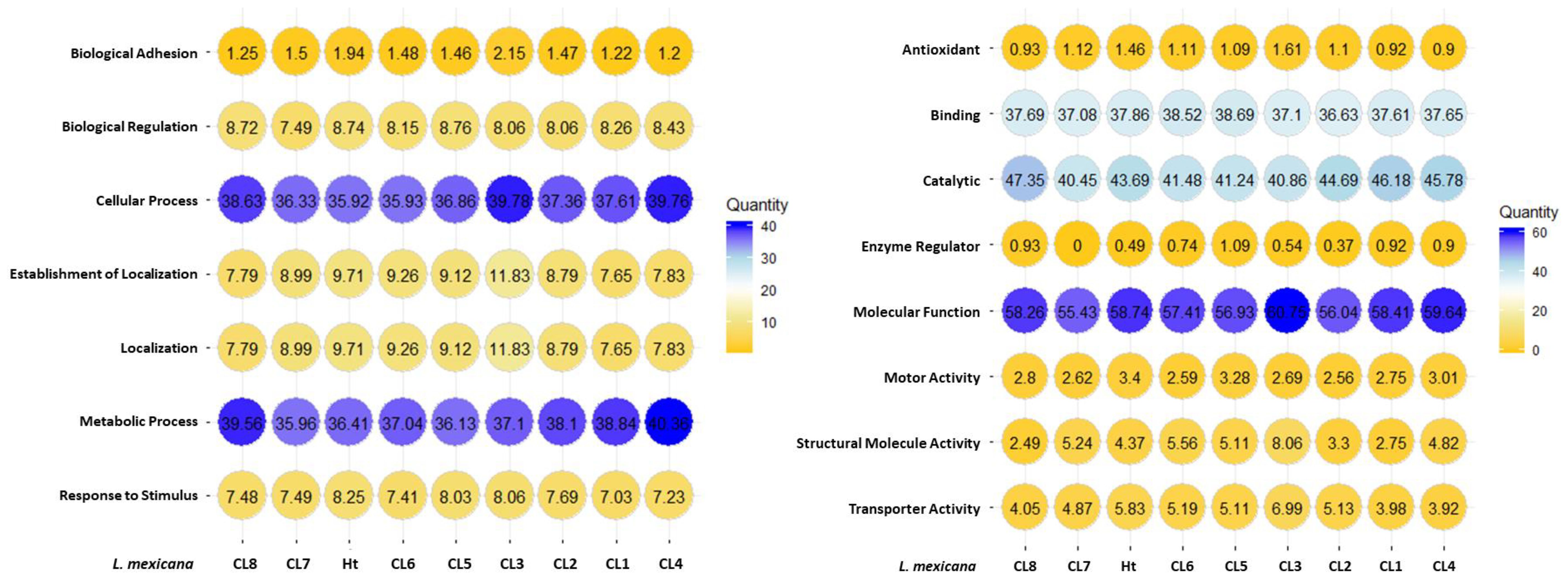
Figure 7. Gene ontology performed for searching for terms related to biological processes and molecular functions. Number present in circles represents the percentage of proteins of that specific category (Eg. Biological adhesion) compared to all proteins identified in the sample. The color legend indicates the spectrum count.
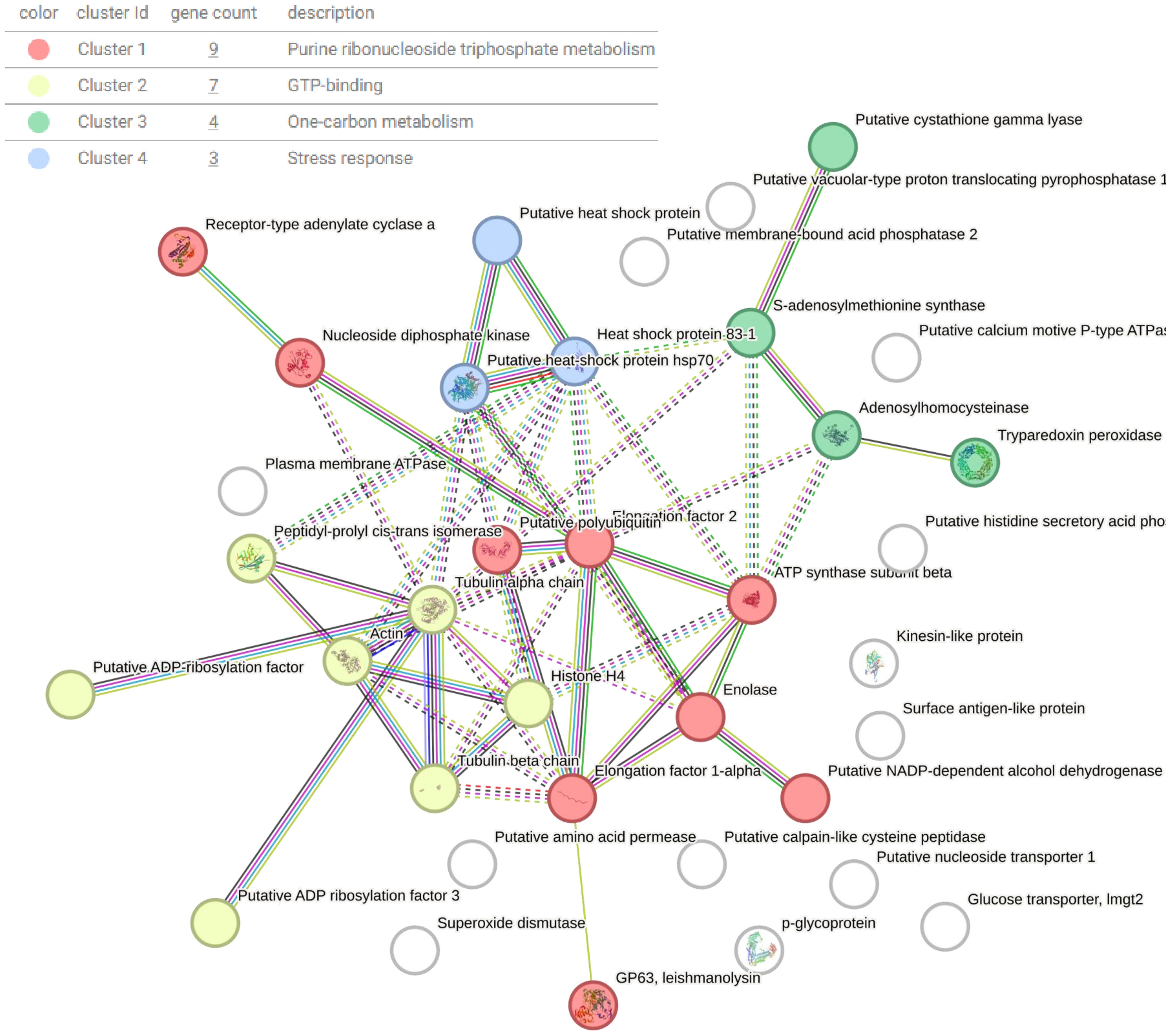
Figure 8. STRING diagram representing interactions between shared proteins present in all EVs isolated from L. mexicana clones and Ht. Proteins are clustered based on function using k-means clustering. Lines represent a direct relationship between proteins, with dotted lines representing the edge of a cluster.
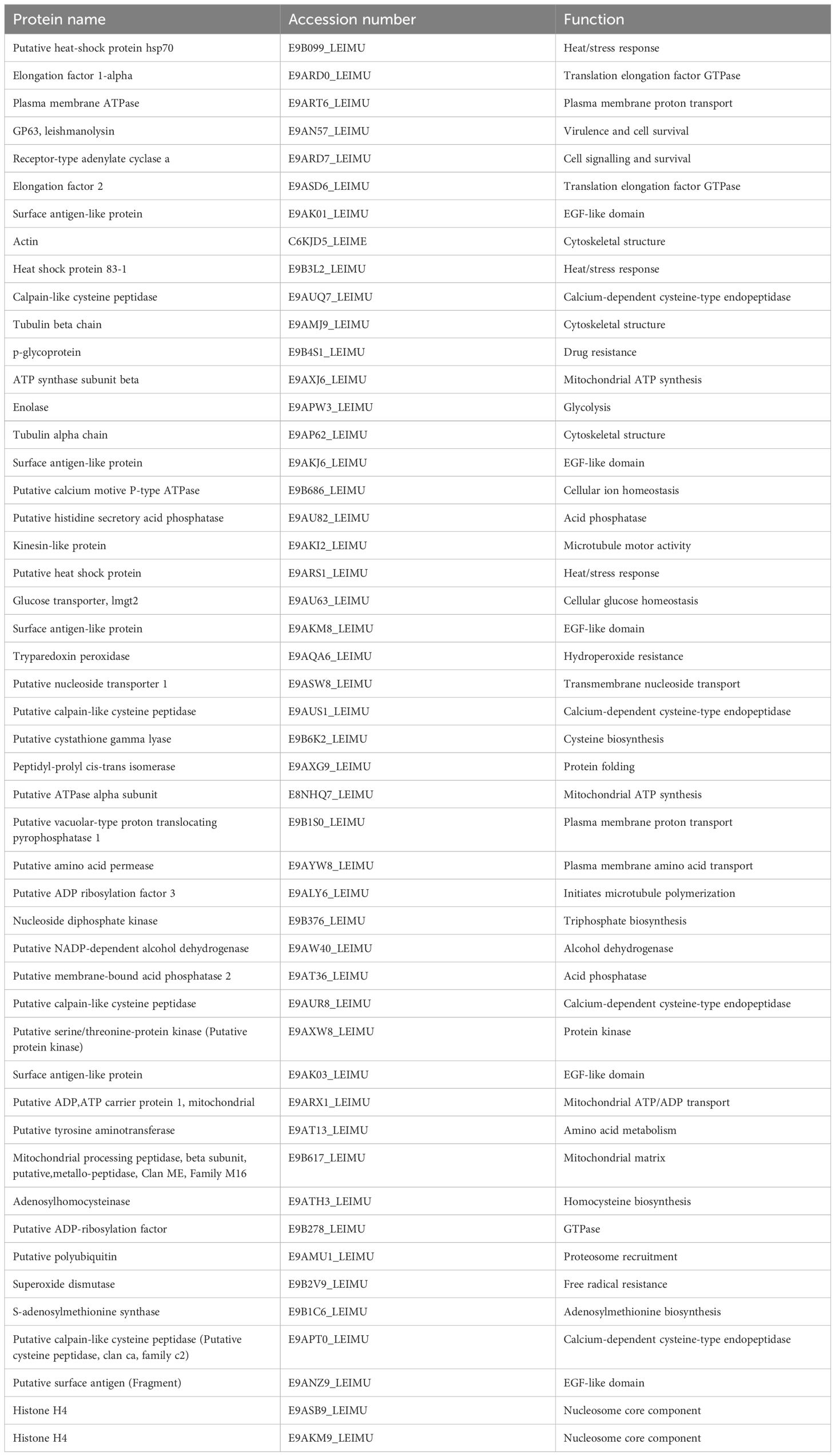
Table 3. All proteins shared between all L. mexicana clones and Ht. Proteins were present with at least 2 peptides and 95% confidence, as determined by Scaffold4.
Vesicles isolated from selected clones exhibit varied infectious capability compared to parasite virulence alone
The infectious capability of L. mexicana clone EVs was tested using a C57BL6 murine footpad infection model, measuring the subsequent footpad swelling to determine infection severity (Figure 9A). The co-inoculation of Ht L. mexicana along with Ht and CL1 EVs significantly worsened the footpad infection compared to Ht L. mexicana alone at all time points measured, consistently causing a 0.2mm increase in footpad swelling at the start of infection and increasing to a 0.3mm difference by week 6 of the infection. CL8 EVs co-inoculation actually reduced footpad swelling at the start of infection compared to Ht and CL1 EVs, even showing an inhibitory effect at week 2. However, the infection proceeded as expected from week 3 onwards, causing around a 0.1mm increase in swelling compared to Ht alone. Strikingly, CL7 EVs did not cause significantly increased footpad swelling compared to Ht alone. The infectious capability of the L, mexicana clone parasites was tested as well, omitting EV co-inoculation (Figure 9B). Surprisingly, the infectious capability of the parasites themselves differed from what was seen with the EVs. Although CL1 EVs did not augment infection more than Ht EVs, CL1 parasites alone caused more severe infection during the first 4 weeks compared to Ht parasites, although footpad swelling was only significantly greater at weeks 1 and 3. The other selected clones did not cause significantly altered infection overall compared to Ht. We also assessed parasite infectious capability using Balb/c mice (Figure 9C). The resulting footpad swelling was significantly greater compared to C57BL6 infection. With the Balb/c model, we found that all L. mexicana clones caused similar infection to Ht L. mexicana with the exception of CL1, which caused significantly more footpad swelling at almost all timepoints measured (weeks 1, 4, 6, and 8 post-infection). CL7 only caused reduced footpad swelling at week 4.
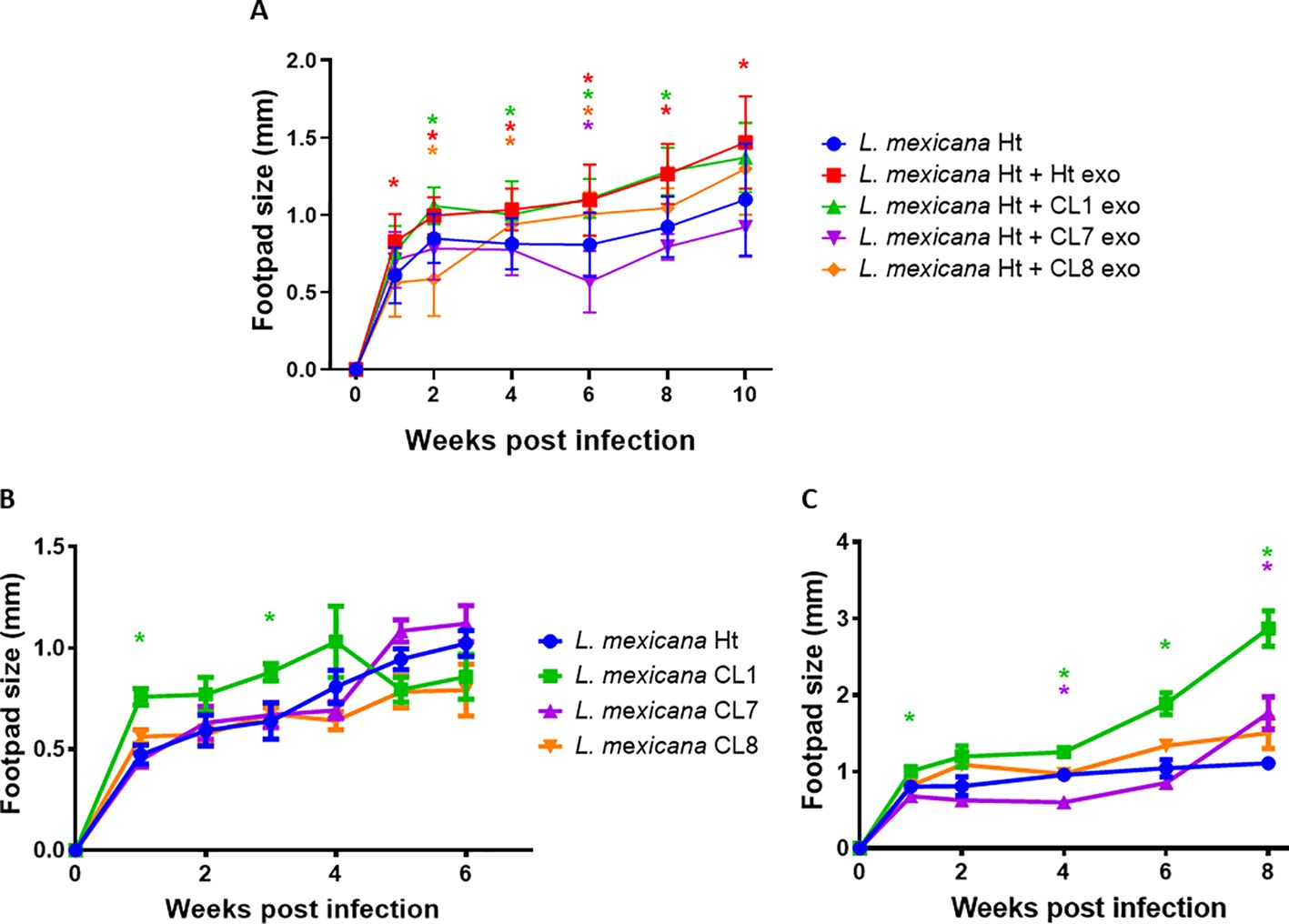
Figure 9. Graphs depicting C57BL6 (A) and BALB/C (C) mice footpad infections performed with Ht L. mexicana supplemented with CL1, 7 and 8 EVs infecting C57BL6 mice (A) or Ht, CL1, 7, and 8 L. mexicana parasite alone infecting C57BL6 mice (B) or Balb/C mice (C). Significance (P<0.05) is denoted by "*", comparing to the Ht L. mexicana infection alone, with color indicating the significant infection corresponding to the legend.
Discussion
The heterogeneity of EVs is a source of great discussion within the field of EVs, leading to great interest in isolating and comparing subpopulations of EVs secreted by a single population of cells or organisms. By isolating clonal colonies of L. mexicana, a highly heterogenous species of Leishmania, we are attempting to similarly isolate different populations of EVs from a single progenitor strain of L. mexicana. When comparing clonal L. mexicana EVs based on their physical properties, they are indistinguishable from one another. There are slight variations in mean diameter and size distribution, but such variations are common between different preparations of EVs rather than being indicative of an overall trend. The parasites themselves are also indistinguishable in terms of morphology and growth rate.
However, proteomic analysis reveals that there is significant variation in the EV protein content of each L. mexicana clone. With over 200 individual proteins identified across all groups, only 51 were shared between all groups. As evident from the STRING diagram, these are all important Leishmania proteins that are typically found in Leishmania EVs, relating to stress response, virulence, cytoskeleton, and metabolism (Nugent et al., 2004; Besteiro et al., 2007; da Silva Lira Filho et al., 2024). Surprisingly, Ht EVs had the fewest individual proteins identified, despite having comparable protein abundance to other groups. This may be due to the inherent heterogeneity of the Ht EVs, with the large amount of unique low-abundance proteins causing the equivalent of noise and preventing their detection by mass spectrometry, especially when compounded with the relatively low starting protein quantity. Such difficulties are commonly reported when attempting proteomic analysis on samples with only a few highly abundant proteins (Echan et al., 2005; Bellei et al., 2011).
On the other hand, CL3 EVs had fewer identified proteins as well as low protein abundance, which could suggest material loss during sample preparation and processing for mass spectrometry. Interestingly, despite the low overall protein abundance, CL3 EVs had the greatest abundance of clathrin heavy chain, which has been implicated in EV uptake by cells (Tian et al., 2014). However, many other such uptake mechanisms have been reported that are clathrin-independent (Svensson et al., 2013; Costa Verdera et al., 2017), and the high abundance of clathrin may even suggest CL3 EVs originate from a unique pathway compared to EVs from the other clones which contain much less clathrin, explaining the lower quantity of collected material. Regardless, due to the low amount of material, CL3 EVs were not used in further experiments.
CL1, 4, and 8 EVs had higher overall protein abundance compared to other clones, suggesting potential enrichment of protein cargo in the EVs of these clones. Of particular interest were CL1 EVs, which contained the highest amount of GP63, EF1α, and EF2, all important Leishmania virulence factors (Nandan et al., 2002; Kushawaha et al., 2011; Isnard et al., 2012). These differences are important due to the limited size of EVs, which means cargo sorted into the EVs must be selected by some mechanism and such minute differences can heavily impact EV physiological function (Kalluri and LeBleu, 2020), specifically their ability to enhance infection in the case of these Leishmania EVs. However, these differences are relatively small when considering the variance that is inherent to mass spectrometry-based proteomic analysis. Indeed, analysis using gene ontology did not reveal any significant broad functional differences between L. mexicana clonal EVs.
The most striking differences between the L. mexicana clones were apparent when testing the infectious capabilities of the EVs. Ht L. mexicana EVs were able to augment infection as expected, L. mexicana CL7 EVs completely lost their infection-enhancing properties and failed to significantly augment infection at any time point. This reduced virulence was not observed when comparing the infectious capabilities of the clonal L. mexicana alone without EV co-inoculation in both C57BL6 and Balb/c mice, with Balb/C infection resulting in greater footpad swelling as expected (Sacks and Noben-Trauth, 2002). This is consistent with what was observed in L. amazonensis, where EVs containing high amounts of GP63 did not significantly enhance infection compared to WT L. amazonensis EVs, but GP63low EVs did not significantly augment infection compared to L. amazonensis alone (da Silva Lira Filho et al., 2021). Additionally, it has been reported that exosomal GP63 modulates hepatic lipid metabolism and lowers serum cholesterol through the cleavage of DICER1, which leads to the inhibition of miR-122 (Ghosh et al., 2013). This further demonstrates that GP63-loaded EVs are specifically capable of modulating host cells. However, the difference in GP63 quantity observed in this study is much lower comparatively, so this explanation alone is not sufficient in explaining the loss of infectious capability of CL1 EVs. Of particular interest, CL7 EVs were also deficient in surface protein amastin and CL8 EVs were deficient in cytochrome C. Cytochrome C is an important oxidase that allows Leishmania to adapt to human macrophage environment and amastin is a classical Leishmania surface virulence factor, important for parasite survival in the sandfly vector as well as infection (Cardenas et al., 2015; de Paiva et al., 2015). However, as we observed, the lack of cytochrome C did not result in any reduced infectivity in either CL8 parasites or its EVs.
In conclusion, although the EVs secreted by different L. mexicana clones are physically indistinguishable from each other, proteomic analysis reveals that they each have unique protein content when considering the overall presence/absence of individual proteins as well as protein abundance. These differences may lead to varying infectious capabilities of the clonal L. mexicana EVs, as we observed when infecting C57BL6 footpads with CL1, 7, and 8 EVs, comparing to Ht L. mexicana, with CL7 EVs losing their infection-augmenting properties. Comparatively, the clonal parasites alone caused mostly consistent levels of footpad swelling. This shows that EVs have unique properties compared to the parasite that secreted them. Despite each clonal L. mexicana being derived from the same L. mexicana Ht culture, their EVs have notable differences in protein content and infectious capability. To validate this work, we would like to sequence the L. mexicana clones used in this study, especially CL1, CL7, and CL8, in order to verify the genetic homogeneity of each clone as well as identify the genetic variations that may lead to the observed differences in infectious capability. This would open the up further gene manipulation studies. It will also be prudent to perform further immunoblotting to validate the proteomic data.
Data availability statement
Original proteomic datasets are available in the publicly accessible PRIDE database. This data can be found at DOI 10.6019/PXD058429 under accession number PXD058429.
Ethics statement
All experiments with mice were carried out in pathogen-free housing and in accordance with the regulations of the Canadian Council of Animal Care, approved by the McGill University Animal Care Committee. The study was conducted in accordance with the local legislation and institutional requirements.
Author contributions
GD: Conceptualization, Data curation, Formal analysis, Investigation, Methodology, Validation, Visualization, Writing – original draft, Writing – review & editing. ND: Investigation, Methodology, Validation, Visualization, Writing – review & editing. CF-P: Conceptualization, Resources, Supervision, Validation, Writing – review & editing. MO: Conceptualization, Data curation, Formal analysis, Funding acquisition, Project administration, Resources, Supervision, Validation, Writing – review & editing.
Funding
The author(s) declare financial support was received for the research, authorship, and/or publication of this article. MO is supported by the Canadian Institutes of Health Research CIHR, grant number PJT-159765 and The Natural Sciences and Engineering Research Council of Canada NSERC. GD was supported by a MSc studentship from Fonds de Recherche du Québec en Santé FRQS and the Canada Graduate Scholarships Doctoral Award CGS-D provided by the CIHR. The funders had no role in study design, data collection and analysis, decision to publish, or preparation of the manuscript.
Acknowledgments
I would like to thank my supervisor and co-supervisor, Martin Olivier and Christopher Fernandez-Prada, for their continued guidance throughout this project. I would also like to thank the entire MO lab for the support and advice I received during experiments and data analysis. Special thanks to the Denis Faubert lab at the Institut de recherches cliniques de Montréal (IRCM) for performing the protein digestion and the liquid chromatography mass spectrometry (LC-MS) necessary for this project.
Conflict of interest
The authors declare that the research was conducted in the absence of any commercial or financial relationships that could be construed as a potential conflict of interest.
Publisher’s note
All claims expressed in this article are solely those of the authors and do not necessarily represent those of their affiliated organizations, or those of the publisher, the editors and the reviewers. Any product that may be evaluated in this article, or claim that may be made by its manufacturer, is not guaranteed or endorsed by the publisher.
Supplementary material
The Supplementary Material for this article can be found online at: https://www.frontiersin.org/articles/10.3389/fcimb.2024.1443262/full#supplementary-material
References
Atayde, V. D., Aslan, H., Townsend, S., Hassani, K., Kamhawi, S., Olivier, M. (2015). Exosome secretion by the parasitic protozoan leishmania within the sand fly midgut. Cell Rep. 13, 957–967. doi: 10.1016/j.celrep.2015.09.058
Bellei, E., Bergamini, S., Monari, E., Fantoni, L. I., Cuoghi, A., Ozben, T., et al. (2011). High-abundance proteins depletion for serum proteomic analysis: concomitant removal of non-targeted proteins. Amino Acids 40, 145–156. doi: 10.1007/s00726-010-0628-x
Berzunza-Cruz, M., Bricaire, G., Romero, S. Z., Perez-Becker, R., Saavedra-Lira, E., Perez-Montfort, R., et al. (2000). Leishmania mexicana mexicana: genetic heterogeneity of mexican isolates revealed by restriction length polymorphism analysis of kinetoplast DNA. Exp. Parasitol. 95, 277–284. doi: 10.1006/expr.2000.4541
Besteiro, S., Williams, R. A., Coombs, G. H., Mottram, J. C. (2007). Protein turnover and differentiation in Leishmania. Int. J. Parasitol. 37, 1063–1075. doi: 10.1016/j.ijpara.2007.03.008
Bordanaba-Florit, G., Royo, F., Kruglik, S. G., Falcon-Perez, J. M. (2021). Using single-vesicle technologies to unravel the heterogeneity of extracellular vesicles. Nat. Protoc. 16, 3163–3185. doi: 10.1038/s41596-021-00551-z
Cardenas, D., Carter, P. M., Nation, C. S., Pizarro, J. C., Guidry, J., Aiyar, A., et al. (2015). LACK, a RACK1 ortholog, facilitates cytochrome c oxidase subunit expression to promote Leishmania major fitness. Mol. Microbiol. 96, 95–109. doi: 10.1111/mmi.2015.96.issue-1
Colombo, M., Raposo, G., Thery, C. (2014). Biogenesis, secretion, and intercellular interactions of exosomes and other extracellular vesicles. Annu. Rev. Cell Dev. Biol. 30, 255–289. doi: 10.1146/annurev-cellbio-101512-122326
Costa Verdera, H., Gitz-Francois, J. J., Schiffelers, R. M., Vader, P. (2017). Cellular uptake of extracellular vesicles is mediated by clathrin-independent endocytosis and macropinocytosis. J. Control Release. 266, 100–108. doi: 10.1016/j.jconrel.2017.09.019
da Silva Lira Filho, A., Fajardo, E. F., Chang, K. P., Clement, P., Olivier, M. (2021). Leishmania exosomes/extracellular vesicles containing GP63 are essential for enhance cutaneous leishmaniasis development upon co-inoculation of leishmania amazonensis and its exosomes. Front. Cell Infect. Microbiol. 11, 709258. doi: 10.3389/fcimb.2021.709258
da Silva Lira Filho, A., Lafleur, A., Marcet-Palacios, M., Olivier, M. (2024). Identification of potential novel proteomic markers of Leishmania spp.-derived exosomes. Front. Cell Infect. Microbiol. 14, 1354636. doi: 10.3389/fcimb.2024.1354636
de Paiva, R. M., Grazielle-Silva, V., Cardoso, M. S., Nakagaki, B. N., Mendonca-Neto, R. P., Canavaci, A. M., et al. (2015). Amastin knockdown in leishmania Braziliensis affects parasite-macrophage interaction and results in impaired viability of intracellular amastigotes. PLoS Pathog. 11, e1005296. doi: 10.1371/journal.ppat.1005296
Dong, G., Filho, A. L., Olivier, M. (2019). Modulation of host-pathogen communication by extracellular vesicles (EVs) of the protozoan parasite leishmania. Front. Cell Infect. Microbiol. 9, 100. doi: 10.3389/fcimb.2019.00100
Douanne, N., Dong, G., Douanne, M., Olivier, M., Fernandez-Prada, C. (2020). Unravelling the proteomic signature of extracellular vesicles released by drug-resistant Leishmania infantum parasites. PLoS Negl. Trop. Dis. 14, e0008439. doi: 10.1371/journal.pntd.0008439
Echan, L. A., Tang, H. Y., Ali-Khan, N., Lee, K., Speicher, D. W. (2005). Depletion of multiple high-abundance proteins improves protein profiling capacities of human serum and plasma. Proteomics. 5, 3292–3303. doi: 10.1002/pmic.200401228
Ghosh, J., Bose, M., Roy, S., Bhattacharyya, S. N. (2013). Leishmania donovani targets Dicer1 to downregulate miR-122, lower serum cholesterol, and facilitate murine liver infection. Cell Host Microbe 13, 277–288. doi: 10.1016/j.chom.2013.02.005
Hassani, K., Antoniak, E., Jardim, A., Olivier, M. (2011). Temperature-induced protein secretion by Leishmania mexicana modulates macrophage signalling and function. PLoS One 6, e18724. doi: 10.1371/journal.pone.0018724
Isnard, A., Shio, M. T., Olivier, M. (2012). Impact of Leishmania metalloprotease GP63 on macrophage signaling. Front. Cell Infect. Microbiol. 2, 72. doi: 10.3389/fcimb.2012.00072
Kalluri, R., LeBleu, V. S. (2020). The biology, function, and biomedical applications of exosomes. Science. 367 (6578). doi: 10.1126/science.aau6977
Kushawaha, P. K., Gupta, R., Sundar, S., Sahasrabuddhe, A. A., Dube, A. (2011). Elongation factor-2, a Th1 stimulatory protein of Leishmania donovani, generates strong IFN-gamma and IL-12 response in cured Leishmania-infected patients/hamsters and protects hamsters against Leishmania challenge. J. Immunol. 187, 6417–6427. doi: 10.4049/jimmunol.1102081
Lex, A., Gehlenborg, N., Strobelt, H., Vuillemot, R., Pfister, H. (2014). UpSet: visualization of intersecting sets. IEEE Trans. Vis. Comput. Graph. 20, 1983–1992. doi: 10.1109/TVCG.2014.2346248
Mann, S., Frasca, K., Scherrer, S., Henao-Martinez, A. F., Newman, S., Ramanan, P., et al. (2021). A review of leishmaniasis: current knowledge and future directions. Curr. Trop. Med. Rep. 8, 121–132. doi: 10.1007/s40475-021-00232-7
Mi, H., Muruganujan, A., Ebert, D., Huang, X., Thomas, P. D. (2019). PANTHER version 14: more genomes, a new PANTHER GO-slim and improvements in enrichment analysis tools. Nucleic Acids Res. 47, D419–DD26. doi: 10.1093/nar/gky1038
Nandan, D., Yi, T., Lopez, M., Lai, C., Reiner, N. E. (2002). Leishmania EF-1alpha activates the Src homology 2 domain containing tyrosine phosphatase SHP-1 leading to macrophage deactivation. J. Biol. Chem. 277, 50190–50197. doi: 10.1074/jbc.M209210200
Nugent, P. G., Karsani, S. A., Wait, R., Tempero, J., Smith, D. F. (2004). Proteomic analysis of Leishmania mexicana differentiation. Mol. Biochem. Parasitol. 136, 51–62. doi: 10.1016/j.molbiopara.2004.02.009
Olivier, M., Atayde, V. D., Isnard, A., Hassani, K., Shio, M. T. (2012). Leishmania virulence factors: focus on the metalloprotease GP63. Microbes Infect. 14, 1377–1389. doi: 10.1016/j.micinf.2012.05.014
Pacheco RS, G. G., Momen, H., Morel, C. M. (1990). Population heterogeneity among clones of New World Leishmania species. Parasitology. 100, 393–398. doi: 10.1017/S0031182000078677
Ramirez, M. I., Amorim, M. G., Gadelha, C., Milic, I., Welsh, J. A., Freitas, V. M., et al. (2018). Technical challenges of working with extracellular vesicles. Nanoscale. 10, 881–906. doi: 10.1039/C7NR08360B
Sacks, D., Noben-Trauth, N. (2002). The immunology of susceptibility and resistance to Leishmania major in mice. Nat. Rev. Immunol. 2, 845–858. doi: 10.1038/nri933
Silverman, J. M., Clos, J., de'Oliveira, C. C., Shirvani, O., Fang, Y., Wang, C., et al. (2010a). An exosome-based secretion pathway is responsible for protein export from Leishmania and communication with macrophages. J. Cell Sci. 123, 842–852. doi: 10.1242/jcs.056465
Silverman, J. M., Clos, J., Horakova, E., Wang, A. Y., Wiesgigl, M., Kelly, I., et al. (2010b). Leishmania exosomes modulate innate and adaptive immune responses through effects on monocytes and dendritic cells. J. Immunol. 185, 5011–5022. doi: 10.4049/jimmunol.1000541
Svensson, K. J., Christianson, H. C., Wittrup, A., Bourseau-Guilmain, E., Lindqvist, E., Svensson, L. M., et al. (2013). Exosome uptake depends on ERK1/2-heat shock protein 27 signaling and lipid Raft-mediated endocytosis negatively regulated by caveolin-1. J. Biol. Chem. 288, 17713–17724. doi: 10.1074/jbc.M112.445403
Szklarczyk, D., Gable, A. L., Lyon, D., Junge, A., Wyder, S., Huerta-Cepas, J., et al. (2019). STRING v11: protein-protein association networks with increased coverage, supporting functional discovery in genome-wide experimental datasets. Nucleic Acids Res. 47, D607–DD13. doi: 10.1093/nar/gky1131
Thery, C., Amigorena, S., Raposo, G., Clayton, A. (2006). Isolation and characterization of exosomes from cell culture supernatants and biological fluids. Curr. Protoc. Cell Biol. 30 (1). doi: 10.1002/0471143030.2006.30.issue-1
Tian, T., Zhu, Y. L., Zhou, Y. Y., Liang, G. F., Wang, Y. Y., Hu, F. H., et al. (2014). Exosome uptake through clathrin-mediated endocytosis and macropinocytosis and mediating miR-21 delivery. J. Biol. Chem. 289, 22258–22267. doi: 10.1074/jbc.M114.588046
van Niel, G., D'Angelo, G., Raposo, G. (2018). Shedding light on the cell biology of extracellular vesicles. Nat. Rev. Mol. Cell Biol. 19, 213–228. doi: 10.1038/nrm.2017.125
Keywords: Leismania mexicana, extracellular vesicles, exosomes, clonal cultures, proteomics, infectious disease
Citation: Dong G, Douanne N, Fernandez-Prada C and Olivier M (2024) Unique Leishmania mexicana clones secrete populations of extracellular vesicles with unique protein profile and variable infectious capability. Front. Cell. Infect. Microbiol. 14:1443262. doi: 10.3389/fcimb.2024.1443262
Received: 03 June 2024; Accepted: 12 November 2024;
Published: 05 December 2024.
Edited by:
Chaoqun Yao, Ross University, United StatesReviewed by:
Bin Gong, University of Texas Medical Branch at Galveston, United StatesRajan Guha, AstraZeneca, Gaithersburg, United States
Copyright © 2024 Dong, Douanne, Fernandez-Prada and Olivier. This is an open-access article distributed under the terms of the Creative Commons Attribution License (CC BY). The use, distribution or reproduction in other forums is permitted, provided the original author(s) and the copyright owner(s) are credited and that the original publication in this journal is cited, in accordance with accepted academic practice. No use, distribution or reproduction is permitted which does not comply with these terms.
*Correspondence: Martin Olivier, TWFydGluLk9saXZpZXJALm1jZ2lsbC5jYQ==; Christopher Fernandez-Prada, Q2hyaXN0b3BoZXIuRmVybmFuZGV6LlByYWRhQHVtb250cmVhbC5jYQ==