- 1Università degli Studi del Molise Department of Medicina e Scienze della Salute “V. Tiberio”, Campobasso, Italy
- 2Unità Operativa (UO) Laboratorio Analisi, Responsible Research Hospital, Campobasso, Italy
- 3Università degli Studi del Molise Department of Agricultural, Environmental and Food Sciences, Campobasso, Italy
- 4Università degli Studi di Catania Department of Drug and Health Sciences, Catania, Italy
- 5ASReM-Azienda Sanitaria Regionale del Molise, Campobasso, Italy
Introduction: Managing burn injuries is a challenge in healthcare. Due to the alarming increase in antibiotic resistance, new prophylactic and therapeutic strategies are being sought. This study aimed to evaluate the potential of live Lactic Acid Bacteria for managing burn infections, using Galleria mellonella larvae as an alternative preclinical animal model and comparing the outcomes with a common antibiotic.
Methods: The antimicrobial activity of LAB isolated from human breast milk was assessed in vitro against Pseudomonas aeruginosa ATCC 27853. Additionally, the immunomodulatory effects of LAB were evaluated in vivo using the G. mellonella burn wound infection model.
Results and discussion: In vitro results demonstrated the antimicrobial activity of Lactic Acid Bacteria against P. aeruginosa. In vivo results show that their prophylactic treatment improves, statistically significant, larval survival and modulates the expression of immunity-related genes, Gallerimycin and Relish/NF-κB, strain-dependently. These findings lay the foundation and suggest a promising alternative for burn wound prevention and management, reducing the risk of antibiotic resistance, enhancing immune modulation, and validating the potential G. mellonella as a skin burn wound model.
1 Introduction
Burn wounds represent a critical challenge in healthcare due to their potential to compromise skin, the body's first line of defense, thereby exposing individuals to the risk of infection and fluid imbalance. The burden of thermal injury worldwide, with more than 250,000 deaths reported each year, mainly affects populations in developing countries with limited access to adequate medical care (Rybarczyk et al., 2016). Often, internal injuries are stiffness in burns associated with extensive body surface area, and mortality reaches critical levels in severely burned patients (Forbinake et al., 2020). Despite efforts to maintain sterility, burn wounds are highly susceptible to microbial colonization and subsequent infection, particularly within the first week post-injury (Ladhani et al., 2021). The wound provides an ideal environment for the proliferation of microorganisms such as Gram-negative bacteria. The standard treatment for burn wounds includes wound care management, pain control, and the use of antibiotics to prevent or treat infections. Debridement, which involves the removal of dead tissue, is a critical step in preventing infection and promoting healing (Jeschke et al., 2020). Burn wounds often requiring aggressive antibiotic therapy to reduce the risk of systemic infection and sepsis. Traditionally, aminoglycosides, such as gentamicin, have been a cornerstone of antibiotic therapy in burn patients, exhibiting efficacy against a broad spectrum of bacteria, including opportunistic pathogens like Pseudomonas. aeruginosa (Corcione et al., 2021). However, the emergence of antibiotic resistance presents significant obstacles to successfully treating burn wound infections, necessitating alternative therapeutic strategies. These include the use of antimicrobial dressings, impregnated with silver or honey, which have shown efficacy in reducing bacterial load and promoting wound (Leaper et al., 2011). Other innovative approaches include the use of negative pressure wound therapy, which applies controlled suction to the wound area, thereby enhancing tissue perfusion and reducing edema (Huang et al., 2014; Agarwal et al., 2019; Norman et al., 2022). Furthermore, Lactic Acid Bacteria (LAB), particularly species within the genus Lactobacillus, have garnered attention for their potential antimicrobial and immunomodulatory properties (Argenta et al., 2016). LABs have demonstrated the ability to inhibit the growth of pathogenic bacteria, including P. aeruginosa, while simultaneously promoting wound healing processes (Andrejko et al., 2021). LABs are a promising option for future complementary therapy in burn wound prevention and management thanks to their diverse mechanisms, including competitive exclusion, generation of antimicrobial substances, and modulation of host immune responses. However, evaluating antimicrobial agents and immunomodulators in burn wound research is inherently challenging due to the complex nature of these injuries. In vitro models lack the complexity of burn wounds, while larger mammalian models present practical limitations such as high costs, ethical concerns, and logistical constraints (Hao and Nourbakhsh, 2021). The use of bacteria in burn injury and burn wound healing has been investigated by various groups in multiple in vivo animal studies with promising results (Maitz et al., 2023). In this context, Galleria mellonella offers a valuable tool for studying burn wound infections. Firstly, it avoids the ethical issues associated with vertebrate animal models. Additionally, it is an economical model that allows for high-throughput experimentation, facilitating the study of various treatments and the rapid and efficient screening of antimicrobial compounds. Also, the G. mellonella model can differentiate between high and low pathogenicity bacterial strains, making it useful for virulence studies, for testing the effectiveness of treatments against specific pathogens and it can be used to test in vivo bacterial antagonist activity. In recent years, many studies have shown that G. mellonella possesses an innate immune system, consisting of hemocytes, with the ability to produce antimicrobial peptides (AMP) and perform phagocytosis. It acts similarly to human innate immunity and shares many characteristics with mammals, including cellular and humoral defense mechanisms. The hemolymph of G. mellonella larvae is nearly germ-free, which significantly reduces the concern about internal contamination that might interfere with in vivo infection model studies and gene expression analysis (Kavanagh and Reeves, 2004; Venditti et al., 2021). Lastly, burns created on the surface of the G. mellonella larvae can replicate many aspects of human burns, including the progression of infection (Tsai et al., 2016; Lange et al., 2018; Maslova et al., 2020, 2021, 2023). Because of these characteristics, G. mellonella is a suitable alternative model to study the effectiveness of live LABs in prevention and control of burn wound infections. In fact, using this brand-new wound model, the purpose of this study is to assess the antibacterial and immunomodulatory activities of LABs isolated from human breast milk in the context of preventing burn wound infections and to investigate the regulation and modulation process of the G. mellonella immune system. This knowledge could help design more effective clinical approaches for the prophylactic treatment of human burn injuries in future (Maslova et al., 2020, 2021, 2023).
2 Materials and methods
A schematic study design representation is shown in Supplementary Figure 1.
2.1 Chemicals and reagents
De Man, Rogosa, and Sharpe (MRS) medium was purchased from Liofilchem and prepared according to manufacturer instructions (62g/L, pH 6.2).
Muller-Hinton (MH) was purchased from Liofilchem and prepared according to manufacturer instructions (21g/L, pH 7.3).
TRIzol Reagent User Guide (Invitrogen Waltham, MA, USA).
Gentamicin topical formulation produced by MSD Italia s.r.l.
Sodium Chloride (NaCl M=58.44g/mol) was purchased from PanReac AppliChem.
cDNA reverse transcription kit (Applied Biosystems™) with RNAse inhibitor.
qRT-PCR was performed using PowerUp™ SYBR™ Green Master Mix (Applied Biosystems).
Water, sterile, and molecular biology grade (DEPC treated, nuclease, and protease free) was purchased from HiMedia.
2.2 Bacterial strains
P. aeruginosa ATCC 27853 (PA) stored at -80°C with 20% glycerol.
2.2.1 No-commercial LABs
Leuconostoc citreum DSM 34870 (L1), Limosilactobacillus fermentum DSM 34871 (L2), Limosilactobacillus fermentum DSM 34872 (L3) and Limosilactobacillus fermentum DSM 34873 (L4) stored at -80°C with 20% glycerol.
2.2.2 Commercial LABs
Lacticaseibacillus rhamnosus ATCC 53103 (LR), Lentilactobacillus kefiri DSM 32079 (LK), Lactoplantibacillus plantarum ATCC 14917 (LP) stored at -80°C with 20% glycerol.
2.3 Bacterial preparations
LAB strains were inoculated into 15ml centrifuge tubes and Petri dishes containing MRS media. The cultures were grown anaerobically with the Anerogen (Thermo ScientificDiagnostic B.V. Landsmeer The Netherlands) anaerobic gas-generating sachet for 48 hours at 37°C (Linares-Morales et al., 2022).
P. aeruginosa strain was plated for growth on MH and incubated for 24 hours at 37°C (Sharma et al., 2020).
2.4 Antimicrobial susceptibility testing
The antibiotic susceptibility of P. aeruginosa ATCC 27583 was tested by Minimum Inhibitory Concentration (MIC) method as previously described by Blandino et al. and Petronio Petronio et al. with some modifications (Blandino et al., 2016; Petronio Petronio et al., 2020). Media, inoculum preparation, antibiotic dilution, and incubation conditions were chosen according to Clinical and Laboratory Standards Institute (CLSI, 2020). Gentamicin with a dilution range of 64-0.125µg/ml was used as test antibiotic. Also, the antibiotic susceptibility of P. aeruginosa ATCC 27583 was tested by the Disk Diffusion (DD) method as reported on the CLSI M100 (32nd Edition) guidelines. Gentamicin 10μg disk was used (Sami Awayid and Qassim Mohammad, 2022; Aggarwal et al., 2024).
2.5 Antimicrobial overlay assay
The antimicrobial overlay assay was performed referring to the Hossain et al. (Hossain et al., 2022) method with some modifications. Briefly, a predefined volume of MRS was poured into each 90 mm Petri dish to prepare the basal agar layer. After agarization, a 10µl aliquot of 0.5 McFarland with a final inoculum concentration of 1 × 106 CFU (Perkin Elmer Wallac Victor 3 1420 Multilabe, OD600) of each LAB strain was spotted in the center of the plate and, after a few minutes, incubated in anaerobic condition overnight at 37°C. The day after, an appropriate volume of the 0.5 McFarland suspensions of P. aeruginosa ATCC 27583 was added to the MH soft agar to seed it with a final inoculum concentration of 1 × 106 CFU/ml. Then, the appropriate volume of soft agar was overlaid to the previously prepared base layer and after agarization, plates were re-incubated overnight at 37°C (Maricic and Dawid, 2014; Hockett and Baltrus, 2017; Riera et al., 2017). After incubation, all inhibition zones were measured by eye using a ruler to the nearest millimeter (Figure 1). The inhibition halos mean diameters were calculated from 3 replicates of 3 independent experiments.
2.6 G. mellonella larvae acquisition and preparation
The larvae were acquired as proposed by our previous work by Venditti et al. (Venditti et al., 2021). G. mellonella larvae were obtained from SA.GI.P. s.a.s. (Ravenna, Italy), kept at 15°C in darkness until use and used for experiments after 2 days of acclimatization at 35°C. The larvae chosen for the experiment weigh between 280-300mg and are in the final larval stage.
2.7 In vivo induction of burn wound on G. mellonella larvae
The induction of in vivo burn wounds was conducted as described by Maslova et al (Maslova et al., 2020, 2023). Briefly, 70% ethanol was used to disinfect the surface of G. mellonella larvae, ensuring coverage of the entire larval body. Petri dishes were uncovered in a sterile environment to facilitate ethanol evaporation post-disinfection. Swabs of the disinfected larvae were plated on CLED media to verify the disinfection procedure. The larvae were positioned on their ventral side to expose the back segment and immobilized by securing the head and thorax segments. A burn instrument, a steel nail embedded in cork with a head size of 2mm², was heated in the central flame of a Bunsen burner until reaching a red/white-hot state and then applied to the middle segment of the G. mellonella larvae's back for 4 seconds until a color variation (dark brown-black) in the cuticle was observed (Figure 2). This method ensures consistent burn wounds across specimens. Larvae displaying significant hemolymph loss or protruding fat body post-procedure were discarded from experimental setting and were promptly euthanized by exposure to temperatures of -20°C for at least 20 minutes to alleviate suffering. After all, the larvae were incubated at 35°C for 48 h (Maslova et al., 2020, 2023). Every experiment was done in triplicate and using an additional group of larvae naïve called "environmental control" (EC) verify the absence of interferences.
2.8 Inoculum preparation
P. aeruginosa ATCC 27583 inoculum was made as follow. Overnight culture grown in MH broth was pelleted and suspended in saline solution water up to an optical density of 0.1 at 600nm OD (Perkin Elmer Wallac Victor 3 1420 Multilabel) corresponding to 1.3 ± 0.2X108 CFU/ml (Alghoribi et al., 2014).
2.9 Prophylactic treatment of burn wound using live LABs
Following burn induction, a sterile 10µl-loop transferred a colony of LAB strains from the MRS agar plates to the wound sites. After a 10-minute, 10µl of P. aeruginosa inoculum was pipetted onto the treated wound. The control groups received no treatment post-burn induction unless 10µl of saline solution (PW). The larvae were incubated at 35°C for 48 h. Mortality was recorded at 0, 6, 12, 18, 24, 36, 42, and 48 hours and was detected when a complete larval stillness occurred, even with external stimulation. Every following experiment was done in triplicate and using an additional group of naïve larvae (EC).
2.10 Prophylactic treatment of burn wound using 0.1% topical gentamicin
After burn induction, gentamicin 0.1% topical formulation was applied to the central part of the wound using a sterile 10µl-loop. After that, 10µl of P. aeruginosa inoculum was pipetted onto the treated wound. GENTA control group received only gentamicin 0.1% topical formulation treatment post-burn induction. PW group received no treatment post-burn induction unless 10µl of saline solution. The larvae were incubated at 35°C for 48 h. As previously detailed, mortality was recorded at 0, 6, 12, 18, 24, 36, 42, and 48 hours, every experiment was done in triplicate.
2.11 Burn wound infection
10µl P. aeruginosa inoculum (final concentration: 1.3 ± 0.2X106 CFU) was pipetted on burn wound G. mellonella larvae as described by Maslova et al. (Maslova et al., 2023). Larvae were incubated in 10cm plates at 35°C, and the number of dead larvae was scored 1 to 4 days after infection. The larvae was considered dead when it displayed no movement in response to touch (Figure 3). Twenty four Larvae were infected with P. aeruginosa only as infection control group (PA). Experiment was done in triplicate, eight larvae were tested in each replicate.
2.12 G. mellonella survival
24 larvae were distributed into 12 groups, as shown in Table 1.
2.13 RNA extraction, cDNA amplification, and qRT-PCR gene expression
12 hours after both treatment, G. mellonella larvae were anesthetized at 4°C for 10 minutes. Subsequently, the last part of the larvae abdomen was cut off using a sterile surgical blade (Swann Morton Limited Sheffield S6 2BJ, England), and hemolymph was collected into 1.5 ml Eppendorf tubes while maintained on ice to prevent melanization (Moya-Andérico et al., 2020). Following the protocol outlined in the TRIzol Reagent User Guide (Invitrogen Waltham, MA, USA), RNA was extracted from the hemolymph of each larval group. A high-capacity cDNA reverse transcription kit (Applied Biosystems™) with RNAse inhibitor was used for cDNA amplification following the instructions outlined in the user guide. Quantitative real-time PCR (qRT-PCR) was performed using PowerUp™ SYBR™ Green Master Mix (Applied Biosystems) following the manufacturer's protocol utilizing Rotor-Gene Q (Qiagen). All the qRT-PCR reactions used specific gene primers for the antimicrobial peptide Gallerimycin (GAL) and transcription factor Relish/NF-kB (REL), as shown in Table 2. Results were normalized against the housekeeping gene Elongation factor 1-Alpha (EF1) level and shown as relative values compared with larvae naïve (EC group). Expression gene fold changes are expressed by the DeltaDeltaCT method (de Melo et al., 2013; Sarvari et al., 2020). The experiment was conducted using three biological replications for three technical replications.
2.14 Statistical analysis
Survival estimates were calculated using the Kaplan-Meier method, with significance calculated from the log-rank approximation of the chi-square test (Wang et al., 2023). One-way analysis of variance (ANOVA) using Tukey's multiple-comparison test was applied to differentiate qRT-PCR data between groups (Liang et al., 2022). Correlation coefficients were calculated using non-parametric Spearman's rank correlation method. Statistical analyses were performed using SPSS Version 26.0. Armonk, NY: IBM Corp.
3 Results
3.1 Antimicrobial susceptibility testing
For P. aeruginosa ATCC 27853, the MIC of gentamicin is 2 µg/ml, and the inhibition halo diameter with 10 µg gentamicin is 17 mm. The results are shown in Table 3.
3.2 Antimicrobial overlay assay
All LABs showed excellent antibacterial activity against PA, except for L2 (Table 4). LABs isolated from human breast milk (No-commercial LABs) inhibited the growth of PA to an equal or greater extent than commercial strains; in fact, L3 had the highest antimicrobial activity in vitro (45mm) followed by LK (43mm), LP and L4 in a tie (40mm).
3.3 Burn wound infection on G. mellonella
As previously described, 10µl P. aeruginosa inoculum was administered in G. mellonella larvae and the mortality rate after 24 hours was 87.50% (Table 5).
3.4 LABs colonization improves G. mellonella survival
Previously, the survival rate of burned larvae treated with LABs only was evaluated, and no mortality was found (data not shown). Thereafter, the protective activity of LABs against PA can be observed in Figures 4A–C. Larvae of the PA group all die at 36h after infection, whereas LABs pre-treated larvae survived up to 48h. At 20h, LR emerges as the strain with the best protective activity. At 48h after infection, L1 showed a minor protective activity, reducing mortality by 41.7%, followed by LK and LP, reducing mortality by 66.7%, and the remaining ones, L2, L3, L4, and LR, respectively, reducing mortality by 75%. Statistical analysis of L1, L2, L3, L4, LR, LP, and LK prophylactic treatment groups showed a significant reduction in mortality compared with the PA group (Table 6). These results suggest that each of the LABs demonstrated efficacy in in vivo antimicrobial activity. On the other hand, no significant differences were observed between L1, L2, L3, and L4 strains compared with LR, LP, and LK strains.
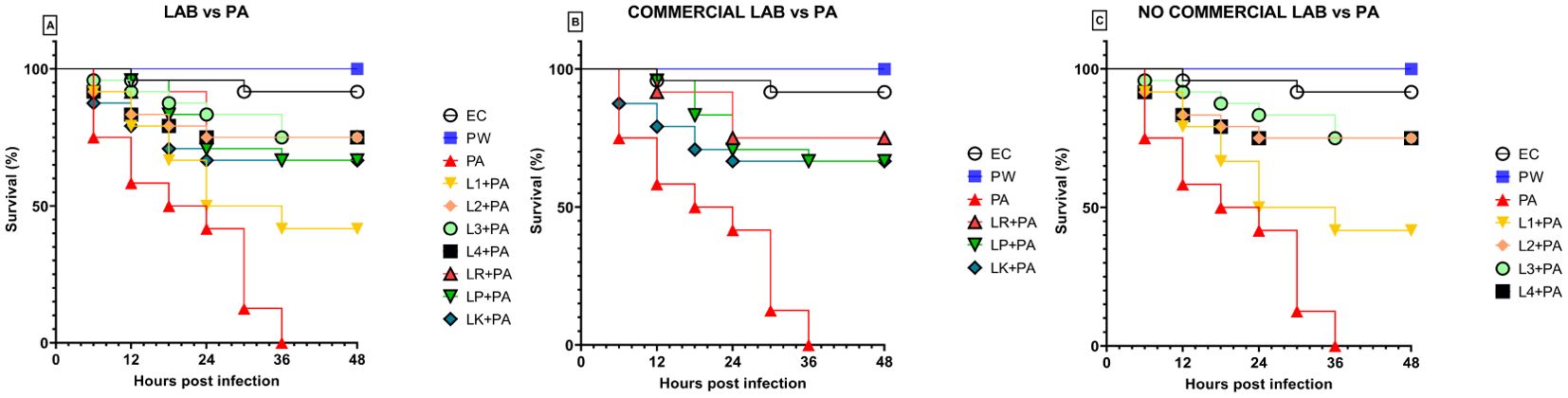
Figure 4. Survival curves of in vivo burn wounds pre-treated with all LABs (A), commercial LABs (B), and no commercial LABs (C) vs PA. EC = Naïve larvae control, PW = Burn wound larvae treated with Saline Solution, PA = Burn wound larvae infected with P. aeruginosa ATCC 27853, L1 + PA = Burn wound larvae pre-treated with L1 and infected with P. aeruginosa ATCC 27853, L2 + PA = Burn wound larvae pre-treated with L2 and infected with P. aeruginosa ATCC 27853, L3 + PA = Burn wound larvae pre-treated with L3 and infected with P. aeruginosa ATCC 27853, L4 + PA = Burn wound larvae pre-treated with L4 and infected with P. aeruginosa ATCC 27853, LR + PA = Burn wound larvae pre-treated with LR and infected with P. aeruginosa ATCC 27853, LP + PA = Burn wound larvae pre-treated with LP and infected with P. aeruginosa ATCC 27853, LK + PA = Burn wound larvae pre-treated with LK and infected with P. aeruginosa ATCC 27853.
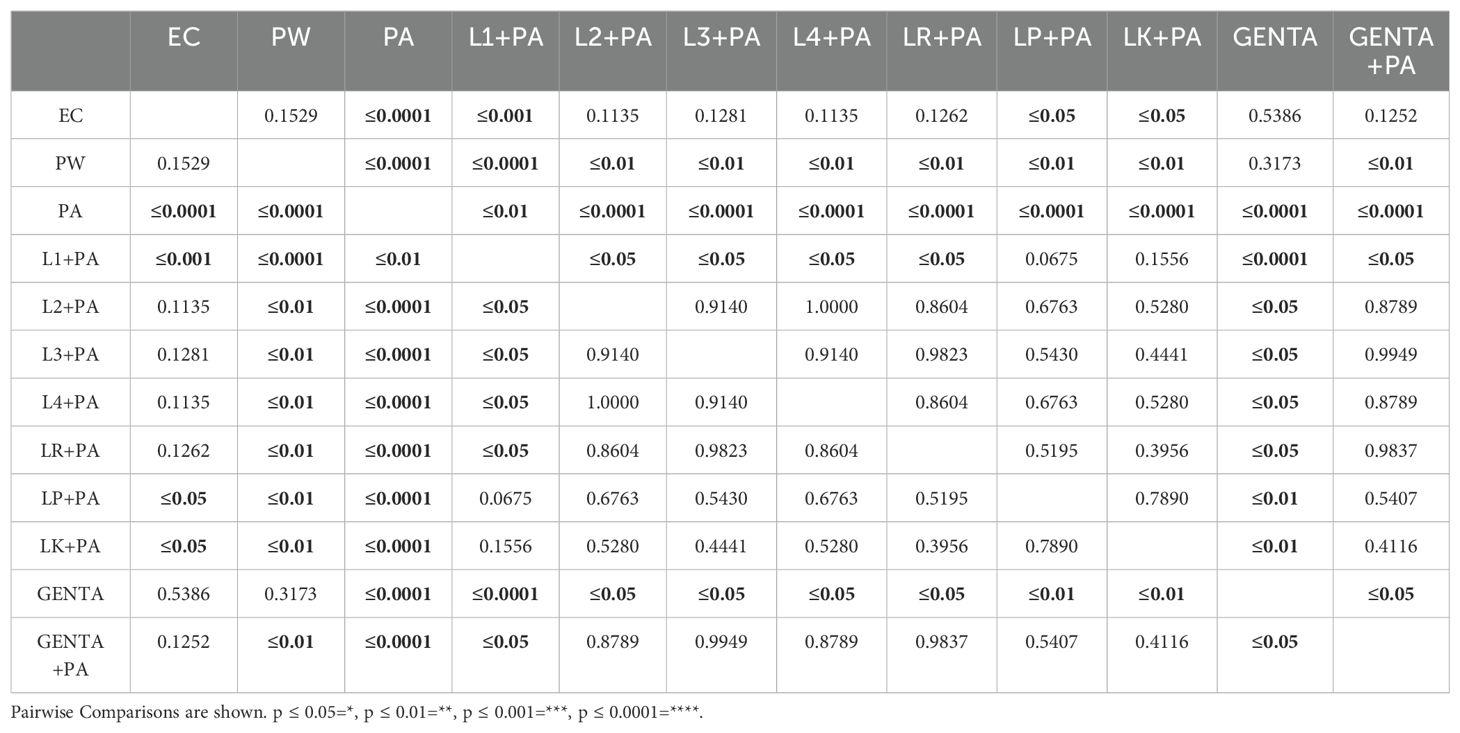
Table 6. Survival estimates were calculated using the Kaplan-Meier method, with significance from the log-rank approximation of the chi-square test.
3.5 LABs colonization matches the antibiotic activity of gentamicin topical formulation
As described above, larvae of the PA group all die at 36h after infection. At 48 hours, larvae pre-treated with the topical formulation of gentamicin, compared to the group infected with PA, showed 75% reduced mortality. As shown in Figure 5, both LABs and gentamicin prophylactic treatments induced a significant and similar reduction in infections compared with controls (p ≤ 0.001) (Figure 4).
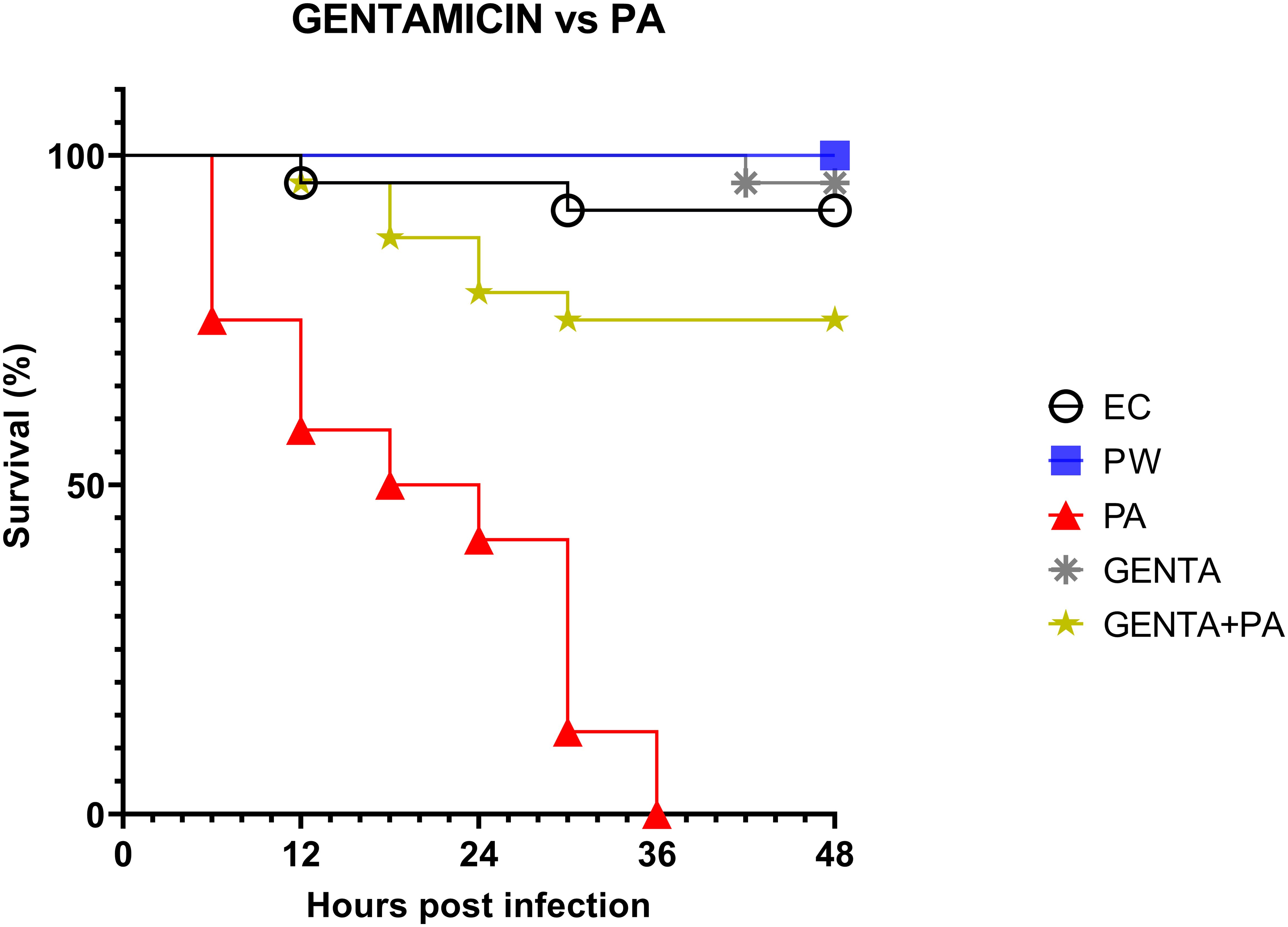
Figure 5. Survival curves of in vivo burn wound treatment with gentamicin topical formulation vs PA. EC = Naïve larvae control, PW = Burn wound larvae treated with Saline Solution, PA = Burn wound larvae infected with P. aeruginosa ATCC 27853, GENTA = Burn wound larvae treated with gentamicin topical formulation 0.1%, GENTA + PA = Burn wound larvae pre-treated with gentamicin topical formulation 0.1% and infected with P. aeruginosa ATCC 27853.
3.6 mRNA expression levels of Gallerimycin and Relish/NF-kB
The mRNA levels of Gallerimycin and Relish/NF-κB of burn wound larvae are summarized in Figures 6A, B respectively. A highly significant difference in Gallerimycin mRNA levels was observed between the PA and PW control groups (p ≤ 0.0001), indicating a substantial impact of P. aeruginosa infection on gene expression compared to the control group (Figure 6A). Likewise, between PA control group and L1+PA group a significant difference (p ≤ 0.001) was recorded as well as for all other treatments (L2+PA, L3+PA, L4+PA, LR+PA, LP+PA, LK+PA, GENTA, GENTA+PA) p ≤ 0.0001 (Figure 6A). The mRNA levels of Relish/NF-κB did not show significant differences compared to control (Figure 6B). In addition, there was a statistically significant negative correlation among PA infected, L1 and L3 treated larvae. In contrast, L2 and L4 a statistically significant positive correlation was found. While, for the other treatment groups, no statistically significant correlations have been found (Table 7).
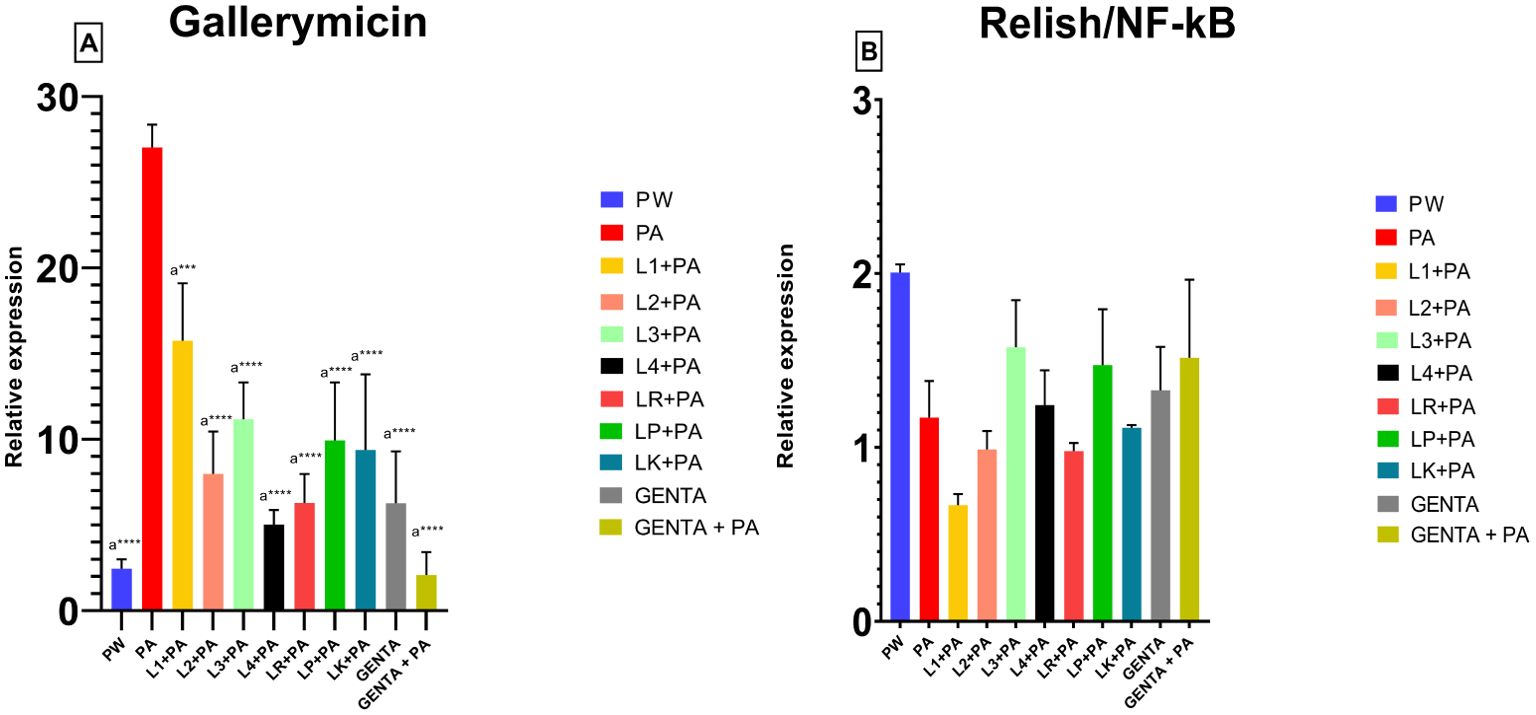
Figure 6. Gallerimycin (A) mRNA levels and Relish/NF-kB (B). PW = Burn wound larvae treated with Saline Solution, PA = Burn wound larvae infected with P. aeruginosa ATCC 27853, L1 + PA = Burn wound larvae pre-treated with L1 and infected with P. aeruginosa ATCC 27853, L2 + PA = Burn wound larvae pre-treated with L2 and infected with P. aeruginosa ATCC 27853, L3 + PA = Burn wound larvae pre-treated with L3 and infected with P. aeruginosa ATCC 27853, L4 + PA = Burn wound larvae pre-treated with L4 and infected with P. aeruginosa ATCC 27853, LR + PA = Burn wound larvae pre-treated with LR and infected with P. aeruginosa ATCC 27853, LP + PA = Burn wound larvae pre-treated with LP and infected with P. aeruginosa ATCC 27853, LK + PA = Burn wound larvae pre-treated with LK and infected with P. aeruginosa ATCC 27853, GENTA = Burn wound larvae treated with gentamicin topical formulation 0.1%, GENTA + PA = Burn wound larvae pre-treated with gentamicin topical formulation 0.1% and infected with P. aeruginosa ATCC 27853. ***=p ≤ 0.001, ****=p ≤ 0.0001, "a" indicates statistical significance toward the PA group.
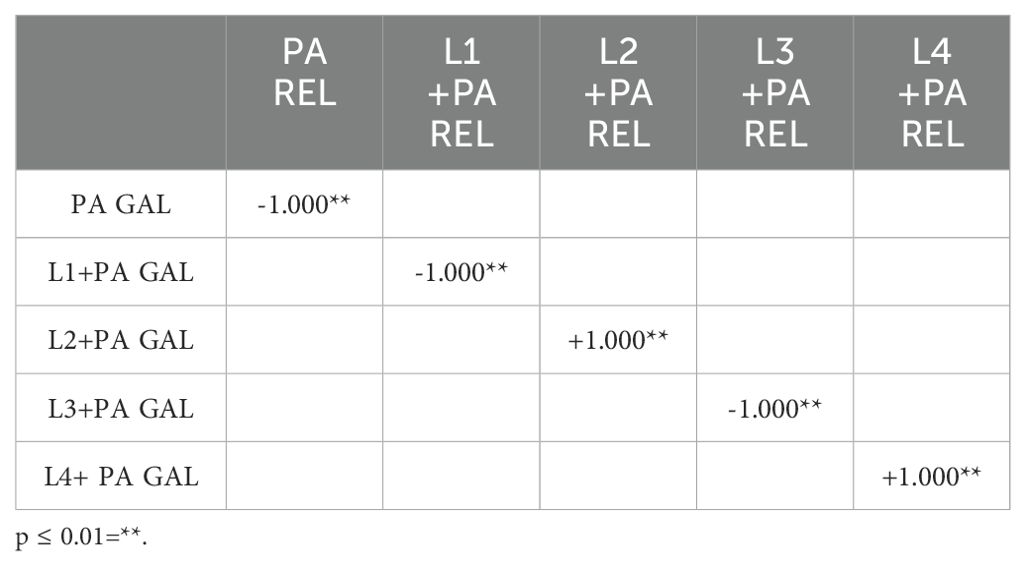
Table 7. Correlation coefficients were calculated using non-parametric Spearman's rank correlation method.
4 Discussion
Severe burns are very devastating forms of trauma that require immediate and specialized medical care. The immunosuppression state, triggered by the burn trauma, and the wound's local microenvironment are favorable for microbial colonization and proliferation. Of major concern among the bacterial etiopathological agents of infections is the opportunistic pathogen P. aeruginosa, Gram-negative, facultative anaerobic rods, non-fermentative, a-sporogenous, motile by polar flagellum (Fakhry and Aljanabi, 2024) causing severe delays in the healing of burn patients and/or leading to exitus, mainly due to multi-resistant strains (Azzopardi et al., 2014; Fournier et al., 2016; Gonzalez et al., 2016).
The work by Cutuli et al. extensively describes the key attributes of G. mellonella in microbiology, as well as its application as an in vivo model for the advancement of novel antibacterial strategies in 2019 (Cutuli et al., 2019). Moreover, Maslova et al, studying G. mellonella larvae and using them as burn model, recapitulate the hallmarks of burn trauma and infection seen in mammalian models (Maslova et al., 2020, 2023). Therefore, using this suitable model, our paper investigated the antimicrobial and immunomodulatory properties of live LABs derived from human breast milk compared to three commercial strains (L. plantarum, L. kefiri, and L. rhamnosus) in burn wound infection management (Köhler, 2015; Champion et al., 2016). In vitro antimicrobial activity performed by overlay assay unveiled the strong inhibition activity of tested LABs against P. aeruginosa ATCC 27853. The overlay assay is a fast-preliminary screening tool widely used in antimicrobial research. It is reliable, simple, inexpensive, and easy to interpret. This method makes it possible to observe and compare specific zones of inhibition that can be critical in identifying active compounds or interactions between different microorganisms (Maricic and Dawid, 2014; Hockett and Baltrus, 2017; Riera et al., 2017; Hossain et al., 2022). In our study, we used whole cells of human milk-derived LABs to closely mimic the natural conditions where live Lactobacillus interact with pathogens. The presence of whole and live cells is crucial for evaluating not just the antimicrobial compounds they produce but also the potential synergistic effects of probiotic cells in combating infections (Hernández et al., 2005; Salas-Jara et al., 2016). While the use of cell-free supernatants (CFS) can provide insights into the antimicrobial compounds secreted by the bacteria, our focus was to evaluate the comprehensive antimicrobial activity, including the direct interactions of live cells with the pathogens. Therefore, we used whole cells to capture this holistic effect. In addition, to ensure proper evaluation of antimicrobial activity during the overlay assay, the incubation period and growth conditions were set so that primary antimicrobial effects could be observed without significant influence from further cell proliferation. Notably, L. fermentum DSM 34872 (L3) exhibited remarkable inhibitory effects on P. aeruginosa growth, outperforming both the commercial strains tested and the DD test (10µg gentamicin disk); followed by L. kefiri DSM 32079 (LK), L. fermentum DSM 34873 (L4) and L. plantarum ATCC 14917 (LP) in a tie (Table 4). Our results agree with previous in vitro findings about LABs antimicrobial activity versus several bacterial pathogens, including P. aeruginosa (Azzopardi et al., 2014). LAB's ability to produce antimicrobial compounds and compete for niche colonization likely underlies their effectiveness in inhibiting bacterial growth (Varma et al., 2011). However, a direct comparison between overlay assay and DD method is not possible due to the different methodologies employed. The overlay assay involves inoculating the bacteria into a soft agar medium that is overlaid onto a pre-inoculated base agar, whereas the DD method involves applying antibiotic-impregnated disks onto a pre-inoculated agar surface. Despite these strong differences, the DD method can still provide valuable insights, especially when interpreting results obtained with gentamicin (Gaudreau et al., 2008; Hossain et al., 2022). Moreover, in vitro LABs antimicrobial activity was compared to the in vivo treated larvae survival rate. The results were consistent across all LABs tested, except for the L2 strain (Figures 4A–C). Survival rates were compared between the PW control group and the PA control group with all prophylactic conditions to determine the specific impact. The survival data indicated no mortality in the heat-treated control group (PW) (Figures 4A–C), confirming that observed deaths were due to P. aerugionosa infection rather than burn wound induction. Although L2 in vitro inhibition halo was the lowest, thus suggesting weak antimicrobial activity against P. aeruginosa. In contrast, L2 in vivo results showed the best activity along with L3 and L4, reducing larvae mortality rates by 75%. This conflicting result confirms that in vitro experimentation cannot always be replaced in vivo experiments (Lorian, 1988). Moreover, this study compared the efficacy of live LABs prophylactic treatment with gentamicin topical formulation prophylactic treatment, an aminoglycoside commonly employed antibiotic in burn wound management (Corcione et al., 2021). These findings align with prior literature, indicating that gentamicin outperforms all other antibacterial tested against P. aeruginosa, significantly reducing bacterial counts in burn-injured porcine tissue (Nuutila et al., 2020). Intriguingly, either LABs (especially L2, L3, L4, and L. rhamnosus ATCC 53103, namely LR) and gentamicin showcased the same protective effects against P. aeruginosa infection in the G. mellonella model (Figures 4A–C). Lastly, mRNA expression analysis on hemolymph revealed significant alterations in the expression levels of immune-related genes, particularly Gallerimycin, in response to P. aeruginosa infection and LABs prophylactic treatments (Figure 6A). Previous studies have demonstrated distinct immune responses in G. mellonella to different types of microorganisms. Their immune response to pathogenic bacteria like P. aeruginosa typically includes upregulation of specific AMP and increased hemocyte activity (Tsai et al., 2016). In contrast, non-pathogenic bacteria such as LAB often induce a milder immune response (Mastromarino et al., 2014). The Gallerimycin is a pivotal AMP in the innate immune response of G. mellonella. Structurally, Gallerimycin shares similarities with defensins found in other insects and even certain vertebrates, suggesting a conserved mechanism of action against pathogens across species (Tsai et al., 2016; Ménard et al., 2021). Reported findings in this paper confirm a substantial upregulation of Gallerimycin expression in larvae infected with P. aeruginosa, denoting the activation of the larval immune system in response to bacterial challenge (Figure 6A). Worth mentioning, LABs prophylactic treatment attenuated the upregulation of Gallerimycin, suggesting a modulation of the immune response towards a less inflammatory phenotype described in literature (Andrejko et al., 2021). Gallerimycin was known exclusively for fungi and not for gram positive bacteria or yeasts (Schuhmann et al., 2003) but several studies suggest that its expression is also induced by infection of Gram-negative bacteria (Bolouri Moghaddam et al., 2016; Andrejko et al., 2021). Relish/NF-κB, the second gene studied, is a critical transcription factor in the Immune deficiency (IMD) pathway of insects, analogous to the NF-κB pathway in vertebrates. The IMD pathway is an essential component of the innate immune system, responsible in the early stages of the immune response for defense against microbial infections, particularly those caused by Gram-negative bacteria, as outlined in numerous studies on insect models, including our prior research on Tenebrio molitor immunity (Sarvari et al., 2020; Petronio Petronio et al., 2022) and other on Drosophila melanogaster (Cammarata-Mouchtouris et al., 2022; Mahanta et al., 2023). qRT-PCR analysis showed mild mRNA expression changes at 12 hours of treatments. Specifically, hypo-expression was observed in all conditions except for the PW control group, L3 group, and LP group, which showed mild overexpression (Figure 6B). Spearman's correlation analysis performed beyond the Relish/NF-κB and Gallerimycin mRNA fold expression revealed a statistically significant correlation in a strains-dependent manner in no-commercial LABs (Table 7). Although this is a pioneering study correlating the expression of Relish/NF-κB and Gallerimycin in infected G. mellonella larvae, a possible explanation can be found in previous studies conducted in Drosophila. Indeed, the correlation found in human breast milk isolated LABs treated larvae, reveals a possible feedback mechanism exerted by the expression of Gallerimycin against Relish/NF-κB as already observed in Drosophila where Relish/NF-κB acts as a controller to avoid unnecessary overexpression of AMP during the acute phase of infection. Pan et al. (Pan et al., 2023) demonstrated how Relish/NF-κB can flexibly alternate its role from a positive regulator to an indirect negative regulator via directly activating miR-275 in balancing Drosophila immune responses. Furthermore, prolonged overexpression of Relish/NF-κB can reduce Drosophila lifespan (Badinloo et al., 2018) and/or cytotoxicity phenomena (Stączek et al., 2023). After all, this control mechanism is also preserved in more complex animal species, including humans. NF-κB is one of the most significant transcription factors that control inducible gene expression as cells attempt to restore homeostasis and must be subject to strict spatiotemporal control to ensure measured and context-specific cellular responses during infection (Prescott et al., 2021). All this corroborates the immunomodulatory capacity of live LABs.
In conclusion, our study demonstrated that prophylactic treatment with live LABs is effective against P. aeruginosa both in vitro and in vivo. Moreover, the activity of some strains is comparable to the prophylactic action of gentamicin. Additionally, LABs can modulate the host immune system, enhancing anti-pathogenic capabilities and reducing harmful inflammatory responses. This paper has significant innovative potential because it explores a new avenue for burn wound treatment. This approach, in addition to preventing possible opportunistic infections, could reduce reliance on traditional antibiotics, thereby helping to mitigate the global issue of antibiotic resistance. As mentioned, the mechanisms by which this occurs aren't precise yet but include several possibilities. LABs may physically occupy space in the burned tissues that would then play host to pathogenic bacteria, blocking them from a residence in the injured tissue. Since LABs have already exhibited inhibitory activity in vitro against P. aeruginosa (Shokri et al., 2018), there is more to it than mechanical action. Their presence probably induces acidification of the local tissue environment, creating conditions unfavorable for the growth of pathogens (Argenta et al., 2016). Additionally, LABs produce substances that hinder the physiological processes of P. aeruginosa, for example, fermenticin from fermentum strains (Kaur et al., 2013), plantaricin from plantarum strain (Righetto et al., 2023), and a famous example is reuterin from L. reuteri, molecules with remarkable antimicrobial activity (Asare et al., 2020). Moreover, LABs may modulate the host immune system, enhancing their anti-pathogenic capabilities and dampening detrimental inflammatory reactions (Thoda and Touraki, 2023). G. mellonella model also represents an advancement in preclinical research methodologies. As previously discussed, the immunomodulatory potential of LABs prophylactic treatments in G. mellonella model is attributed to the modulation of Gallerimycin and Relish/NF-κB expression, the strain-specific effects of LABs. Relish/NF-κB, influenced by LABs, can flexibly alternate its role from a positive regulator to an indirect negative regulator thanks to a feedback mechanism regulating expressions of AMP. Laying the foundation on this new line of research, recent studies used LABs treatment on ex-vivo human skin models (Li et al., 2023) and used L. plantarum on in vivo burn wounds, with remarkable results (Peral et al., 2009).
Despite the premises, several limitations should be acknowledged. The G. mellonella larvae, as the other in vivo alternative models as emphasized in our earlier investigation conducted by Cutuli et al. in 2021, employing the snail model Limacus flavus for in vivo assessment of mucosal irritation (Cutuli et al., 2021). Indeed, alternative models may not fully recapitulate the complexity of human burn wounds. In fact, G. mellonella does not possess the capability to fully heal burn wounds as complex organisms do. Due to their biology, larvae typically enter pupation with the burn wound still present, and the lesion does not regenerate (Maslova et al., 2020; Pereira et al., 2020). This characteristic limits the extent to which we can assess complete wound healing in this model. As such, while the G. mellonella model is valuable for studying the effects of antimicrobial treatments and general health impacts, it has inherent limitations in evaluating full tissue regeneration and wound closure (Dai et al., 2011; Kazek et al., 2019; Maslova et al., 2020; Pereira et al., 2020; Serrano et al., 2023). However, future studies should validate their efficacy in more clinical experiments, for example, comparing results in validated in vivo models, such as murine models, to affirm the real translational potential (Cutuli et al., 2019). Continued research is needed to understand how LABs influence both pathogens and hosts. Additional clinical trials exploring their application in burn and other wound scenarios will help clarify the expanding role of prophylactic live LABs treatment and their immunomodulatory action. This will enhance our understanding of their potential to prevent infections and promote healing, providing a viable alternative to traditional antibiotics.
Data availability statement
The datasets presented in this study can be found in online repositories. “Figshare” data repository at https://DOI.org/10.6084/m9.figshare.25594491.
Ethics statement
The manuscript presents research on animals that do not require ethical approval for their study.
Author contributions
AG: Conceptualization, Writing – original draft, Writing – review & editing. NV: Conceptualization, Formal analysis, Writing – original draft. MC: Writing – original draft. NB: Conceptualization, Writing – original draft. GSa: Formal analysis, Writing – review & editing. IM: Investigation, Writing – review & editing. LP: Formal analysis, Writing – review & editing. MF: Software, Writing – review & editing. FV: Data curation, Writing – review & editing. DN: Writing – review & editing. FS: Writing – review & editing. SD: Writing – review & editing. GSc: Writing – review & editing. GP: Project administration, Supervision, Writing – review & editing. RM: Funding acquisition, Project administration, Writing – review & editing.
Funding
The author(s) declare financial support was received for the research, authorship, and/or publication of this article. The author(s) declare that financial support was received for publication cost by "NATIVAL s.r.l.'', spin-off company of Università degli Studi del Molise.
Acknowledgments
No-commercial LABs, namely Leuconostoc citreum DSM 34870 (L1), Limosilactobacillus fermentum DSM 34871 (L2), Limosilactobacillus fermentum DSM 34872 (L3) and Limosilactobacillus fermentum DSM 34873 (L4) were donated from the company “NATIVAL s.r.l.”.
Conflict of interest
Authors NV, MC, IM, LP, FV, SD, GSc, GP and RM were NATIVAL s.r.l. founders at the time of the study.
The remaining authors declare that the research was conducted in the absence of any commercial or financial relationships that could be construed as a potential conflict of interest.
Publisher’s note
All claims expressed in this article are solely those of the authors and do not necessarily represent those of their affiliated organizations, or those of the publisher, the editors and the reviewers. Any product that may be evaluated in this article, or claim that may be made by its manufacturer, is not guaranteed or endorsed by the publisher.
Supplementary material
The Supplementary Material for this article can be found online at: https://www.frontiersin.org/articles/10.3389/fcimb.2024.1428525/full#supplementary-material
References
Agarwal, P., Kukrele, R., Sharma, D. (2019). Vacuum assisted closure (VAC)/negative pressure wound therapy (NPWT) for difficult wounds: A review. J. Clin. Orthop Trauma 10, 845–848. doi: 10.1016/j.jcot.2019.06.015
Aggarwal, P., Saxena, S., Nagi, N. (2024). Possible impact of revisions in disc diffusion breakpoints for aminoglycosides and piperacillin/tazobactam in the 33rd edition of CLSI M100 document on clinical reporting and use in Indian settings with low susceptibility. Indian J. Med. Microbiol. 49, 100602. doi: 10.1016/j.ijmmb.2024.100602
Alghoribi, M. F., Gibreel, T. M., Dodgson, A. R., Beatson, S. A., Upton, M. (2014). Galleria mellonella Infection Model Demonstrates High Lethality of ST69 and ST127 Uropathogenic E. coli. PloS One 9, e101547. doi: 10.1371/journal.pone.0101547
Andrejko, M., Mak, P., Siemińska-Kuczer, A., Iwański, B., Wojda, I., Suder, P., et al. (2021). A comparison of the production of antimicrobial peptides and proteins by Galleria mellonella larvae in response to infection with two Pseudomonas aeruginosa strains differing in the profile of secreted proteases. J. Insect Physiol. 131, 104239. doi: 10.1016/j.jinsphys.2021.104239
Argenta, A., Satish, L., Gallo, P., Liu, F., Kathju, S. (2016). Local application of probiotic bacteria prophylaxes against sepsis and death resulting from burn wound infection. PloS One 11, e0165294. doi: 10.1371/journal.pone.0165294
Asare, P. T., Zurfluh, K., Greppi, A., Lynch, D., Schwab, C., Stephan, R., et al. (2020). Reuterin demonstrates potent antimicrobial activity against a broad panel of human and poultry meat campylobacter spp. Isolates. Microorganisms 8, 78. doi: 10.3390/microorganisms8010078
Azzopardi, E. A., Azzopardi, E., Camilleri, L., Villapalos, J., Boyce, D. E., Dziewulski, P., et al. (2014). Gram negative wound infection in hospitalised adult burn patients-systematic review and metanalysis-. PloS One 9, e95042. doi: 10.1371/journal.pone.0095042
Badinloo, M., Nguyen, E., Suh, W., Alzahrani, F., Castellanos, J., Klichko, V. I., et al. (2018). Over-expression of antimicrobial peptides contributes to aging through cytotoxic effects in Drosophila tissues. Arch. Insect Biochem. Physiol. 98, e21464. doi: 10.1002/arch.21464
Blandino, G., Fazio, D., Petronio, G. P., Inturri, R., Tempera, G., Furneri, P. M. (2016). Labeling quality and molecular characterization studies of products containing Lactobacillus spp. strains. Int. J. Immunopathol. Pharmacol. 29, 121–128. doi: 10.1177/0394632015600534
Bolouri Moghaddam, M. R., Tonk, M., Schreiber, C., Salzig, D., Czermak, P., Vilcinskas, A., et al. (2016). The potential of the Galleria mellonella innate immune system is maximized by the co-presentation of diverse antimicrobial peptides. Biol. Chem. 397, 939–945. doi: 10.1515/hsz-2016-0157
Cammarata-Mouchtouris, A., Acker, A., Goto, A., Chen, D., Matt, N., Leclerc, V. (2022). Dynamic regulation of NF-κB response in innate immunity: the case of the IMD pathway in drosophila. Biomedicines 10, 2304. doi: 10.3390/biomedicines10092304
Champion, O. L., Wagley, S., Titball, R. W. (2016). Galleria mellonella as a model host for microbiological and toxin research. Virulence 7, 840–845. doi: 10.1080/21505594.2016.1203486
CLSI. (2020). Performance Standards for Antimicrobial Susceptibility Testing (Wayne, PA, USA: Clinical and Laboratory Standards Institute). Available online at: https://www.google.com/search?q=CLSI.+Performance+Standards+for+Antimicrobial+Susceptibility+Testing%3B+Clinical+and+Laboratory+Standards+Institute%3A+Wayne%2C+PA%2C+USA%2C+2020.&oq=CLSI.+Performance+Standards+for+Antimicrobial+Susceptibility+Testing%3B+Clinical+and+Laboratory+Standards+Institute%3A+Wayne%2C+PA%2C+USA%2C+2020.&gs_lcrp=EgZjaHJvbWUqBggAEEUYOzIGCAAQRRg70gEHMzU3ajBqOagCALACAA&sourceid=chrome&ie=UTF-8 (Accessed March 6, 2024).
Corcione, S., De Nicolò, A., Lupia, T., Segala, F. V., Pensa, A., Corgiat Loia, R., et al. (2021). Observed concentrations of amikacin and gentamycin in the setting of burn patients with gram-negative bacterial infections: Preliminary data from a prospective study. Therapies 76, 409–414. doi: 10.1016/j.therap.2020.10.003
Cutuli, M. A., Guarnieri, A., Pietrangelo, L., Magnifico, I., Venditti, N., Recchia, L., et al. (2021). Potential Mucosal Irritation Discrimination of Surface Disinfectants Employed against SARS-CoV-2 by Limacus flavus Slug Mucosal Irritation Assay. Biomedicines 9, 424. doi: 10.3390/biomedicines9040424
Cutuli, M. A., Petronio Petronio, G., Vergalito, F., Magnifico, I., Pietrangelo, L., Venditti, N., et al. (2019). Galleria mellonella as a consolidated in vivo model hosts: New developments in antibacterial strategies and novel drug testing. Virulence 10, 527–541. doi: 10.1080/21505594.2019.1621649
Dai, T., Kharkwal, G. B., Tanaka, M., Huang, Y.-Y., Bil de Arce, V. J., Hamblin, M. R. (2011). Animal models of external traumatic wound infections. Virulence 2, 296–315. doi: 10.4161/viru.2.4.16840
de Melo, N. R., Abdrahman, A., Greig, C., Mukherjee, K., Thornton, C., Ratcliffe, N. A., et al. (2013). Myriocin significantly increases the mortality of a non-mammalian model host during candida pathogenesis. PloS One 8, e78905. doi: 10.1371/journal.pone.0078905
Dubovskiy, I. M., Miranda, M. M. A., Yaroslavtseva, O. N., Greig, C., Kryukov, V. Y., Ekaterina, V., et al. (2013). Can insects develop resistance to insect pathogenic fungi? PloS one 8(4), e60248.
Fakhry, A. K., Aljanabi, A. O. (2024). The effect of pseudomonas infection with burns patients. J. Curr. Med. Res. Opin. 7, 2135–2157. doi: 10.52845/CMRO/2024/7-2-11
Forbinake, N. A., Ohandza, C. S., Fai, K. N., Agbor, V. N., Asonglefac, B. K., Aroke, D., et al. (2020). Mortality analysis of burns in a developing country: a CAMEROONIAN experience. BMC Public Health 20, 1269. doi: 10.1186/s12889-020-09372-3
Fournier, A., Voirol, P., Krähenbühl, M., Bonnemain, C.-L., Fournier, C., Pantet, O., et al. (2016). Antibiotic consumption to detect epidemics of Pseudomonas aeruginosa in a burn centre: A paradigm shift in the epidemiological surveillance of Pseudomonas aeruginosa nosocomial infections. Burns 42, 564–570. doi: 10.1016/j.burns.2015.10.030
Gaudreau, C., Girouard, Y., Gilbert, H., Gagnon, J., Bekal, S. (2008). Comparison of Disk Diffusion and Agar Dilution Methods for Erythromycin, Ciprofloxacin, and Tetracycline Susceptibility Testing of Campylobacter coli and for Tetracycline Susceptibility Testing of Campylobacter jejuni subsp. jejuni. Antimicrob. Agents Chemother. 52, 4475–4477. doi: 10.1128/AAC.00767-08
Gonzalez, M. R., Fleuchot, B., Lauciello, L., Jafari, P., Applegate, L. A., Raffoul, W., et al. (2016). Effect of human burn wound exudate on pseudomonas aeruginosa virulence. mSphere 1, e00111–e00115. doi: 10.1128/mSphere.00111-15
Hao, D., Nourbakhsh, M. (2021). Recent advances in experimental burn models. Biol. (Basel) 10, 526. doi: 10.3390/biology10060526
Hernández, D., Cardell, E., Zárate, V. (2005). Antimicrobial activity of lactic acid bacteria isolated from Tenerife cheese: initial characterization of plantaricin TF711, a bacteriocin-like substance produced by Lactobacillus plantarum TF711. J. Appl. Microbiol. 99, 77–84. doi: 10.1111/j.1365-2672.2005.02576.x
Hockett, K. L., Baltrus, D. A. (2017). Use of the soft-agar overlay technique to screen for bacterially produced inhibitory compounds. J. Vis. Exp., 55064. doi: 10.3791/55064
Hossain, M. L., Hammer, K., Lim, L. Y., Hettiarachchi, D., Locher, C. (2022). Optimisation of an agar overlay assay for the assessment of the antimicrobial activity of topically applied semi-solid antiseptic products including honey-based formulations. J. Microbiol. Methods 202, 106596. doi: 10.1016/j.mimet.2022.106596
Huang, C., Leavitt, T., Bayer, L. R., Orgill, D. P. (2014). Effect of negative pressure wound therapy on wound healing. Curr. Problems Surg. 51, 301–331. doi: 10.1067/j.cpsurg.2014.04.001
Jeschke, M. G., van Baar, M. E., Choudhry, M. A., Chung, K. K., Gibran, N. S., Logsetty, S. (2020). Burn injury. Nat. Rev. Dis. Primers 6, 1–25. doi: 10.1038/s41572-020-0145-5
Kaur, B., Balgir, P. P., Mittu, B., Kumar, B., Garg, N. (2013). Biomedical applications of fermenticin HV6b isolated from lactobacillus fermentum HV6b MTCC10770. BioMed. Res. Int. 2013, 168438. doi: 10.1155/2013/168438
Kavanagh, K., Reeves, E. P. (2004). Exploiting the potential of insects for in vivo pathogenicity testing of microbial pathogens. FEMS Microbiol. Rev. 28, 101–112. doi: 10.1016/j.femsre.2003.09.002
Kazek, M., Kaczmarek, A., Wrońska, A. K., Boguś, M. I. (2019). Diet influences the bacterial and free fatty acid profiles of the cuticle of Galleria mellonella larvae. PloS One 14, e0211697. doi: 10.1371/journal.pone.0211697
Köhler, G. (2015). Probiotics research in Galleria mellonella. Virulence 6, 3–5. doi: 10.1080/21505594.2014.998967
Ladhani, H. A., Yowler, C. J., Claridge, J. A. (2021). Burn wound colonization, infection, and sepsis. Surg. Infect. 22, 44–48. doi: 10.1089/sur.2020.346
Lange, A., Beier, S., Huson, D. H., Parusel, R., Iglauer, F., Frick, J.-S. (2018). Genome sequence of galleria mellonella (Greater wax moth). Genome Announc. 6, e01220–e01217. doi: 10.1128/genomeA.01220-17
Leaper, D., Assadian, O., Hubner, N.-O., McBain, A., Barbolt, T., Rothenburger, S., et al. (2011). Antimicrobial sutures and prevention of surgical site infection: assessment of the safety of the antiseptic triclosan. Int. Wound J. 8, 556–566. doi: 10.1111/j.1742-481X.2011.00841.x
Li, Z., Zhang, S., Zuber, F., Altenried, S., Jaklenec, A., Langer, R., et al. (2023). Topical application of Lactobacilli successfully eradicates Pseudomonas aeruginosa biofilms and promotes wound healing in chronic wounds. Microbes Infect. 25, 105176. doi: 10.1016/j.micinf.2023.105176
Liang, Z., Li, H., Qu, M., Liu, Y., Wang, Y., Wang, H., et al. (2022). Intranasal bovine β-defensin-5 enhances antituberculosis immunity in a mouse model by a novel protein-based respiratory mucosal vaccine. Virulence 13, 949–962. doi: 10.1080/21505594.2022.2080342
Linares-Morales, J. R., Salmerón-Ochoa, I., Rivera-Chavira, B. E., Gutiérrez-Méndez, N., Pérez-Vega, S. B., Nevárez-Moorillón, G. V. (2022). Influence of culture media formulated with agroindustrial wastes on the antimicrobial activity of lactic acid bacteria. J. Microbiol. Biotechnol. 32, 64–71. doi: 10.4014/jmb.2107.07030
Lorian, V. (1988). Differences between in vitro and in vivo studies. Antimicrob. Agents Chemother. 32, 1600–1601. doi: 10.1128/AAC.32.10.1600
Mahanta, D. K., Bhoi, T. K., Komal, J., Samal, I., Nikhil, R. M., Paschapur, A. U., et al. (2023). Insect-pathogen crosstalk and the cellular-molecular mechanisms of insect immunity: uncovering the underlying signaling pathways and immune regulatory function of non-coding RNAs. Front. Immunol. 14. doi: 10.3389/fimmu.2023.1169152
Maitz, J., Merlino, J., Rizzo, S., McKew, G., Maitz, P. (2023). Burn wound infections microbiome and novel approaches using therapeutic microorganisms in burn wound infection control. Adv. Drug Deliv. Rev. 196, 114769. doi: 10.1016/j.addr.2023.114769
Maricic, N., Dawid, S. (2014). Using the overlay assay to qualitatively measure bacterial production of and sensitivity to pneumococcal bacteriocins. J. Vis. Exp., 51876. doi: 10.3791/51876
Maslova, E., Eisaiankhongi, L., Sjöberg, F., McCarthy, R. R. (2021). Burns and biofilms: priority pathogens and in vivo models. NPJ Biofilms Microbiomes 7, 1–9. doi: 10.1038/s41522-021-00243-2
Maslova, E., Osman, S., McCarthy, R. R. (2023). Using the Galleria mellonella burn wound and infection model to identify and characterize potential wound probiotics. Microbiol. (Reading) 169, 1350. doi: 10.1099/mic.0.001350
Maslova, E., Shi, Y., Sjöberg, F., Azevedo, H. S., Wareham, D. W., McCarthy, R. R. (2020). An invertebrate burn wound model that recapitulates the hallmarks of burn trauma and infection seen in mammalian models. Front. Microbiol. 11. doi: 10.3389/fmicb.2020.00998
Mastromarino, P., Capobianco, D., Campagna, G., Laforgia, N., Drimaco, P., Dileone, A., et al. (2014). Correlation between lactoferrin and beneficial microbiota in breast milk and infant’s feces. Biometals 27, 1077–1086. doi: 10.1007/s10534-014-9762-3
Ménard, G., Rouillon, A., Cattoir, V., Donnio, P.-Y. (2021). Galleria mellonella as a suitable model of bacterial infection: past, present and future. Front. Cell. Infect. Microbiol. 11. doi: 10.3389/fcimb.2021.782733
Moya-Andérico, L., Admella, J., Fernandes, R., Torrents, E. (2020). Monitoring Gene Expression during a Galleria mellonella Bacterial Infection. Microorganisms 8, 1798. doi: 10.3390/microorganisms8111798
Norman, G., Shi, C., Goh, E. L., Murphy, E. M., Reid, A., Chiverton, L., et al. (2022). Negative pressure wound therapy for surgical wounds healing by primary closure. Cochrane Database Syst. Rev. 2022, CD009261. doi: 10.1002/14651858.CD009261.pub7
Nuutila, K., Grolman, J., Yang, L., Broomhead, M., Lipsitz, S., Onderdonk, A., et al. (2020). Immediate treatment of burn wounds with high concentrations of topical antibiotics in an alginate hydrogel using a platform wound device. Adv. Wound Care (New Rochelle) 9, 48–60. doi: 10.1089/wound.2019.1018
Pan, W., Yao, X., Lin, L., Liu, X., Jin, P., Ma, F. (2023). The Relish/miR-275/Dredd mediated negative feedback loop is crucial to restoring immune homeostasis of Drosophila Imd pathway. Insect Biochem. Mol. Biol. 162, 104013. doi: 10.1016/j.ibmb.2023.104013
Peral, M. C., Huaman Martinez, M. A., Valdez, J. C. (2009). Bacteriotherapy with Lactobacillus plantarum in burns. Int. Wound J. 6, 73–81. doi: 10.1111/j.1742-481X.2008.00577.x
Pereira, M. F., Rossi, C. C., da Silva, G. C., Rosa, J. N., Bazzolli, D. M. S. (2020). Galleria mellonella as an infection model: an in-depth look at why it works and practical considerations for successful application. Pathog. Dis. 78, ftaa056. doi: 10.1093/femspd/ftaa056
Petronio Petronio, G., Cutuli, M. A., Magnifico, I., Venditti, N., Pietrangelo, L., Vergalito, F., et al. (2020). In vitro and in vivo biological activity of berberine chloride against uropathogenic E. coli strains using galleria mellonella as a host model. Molecules 25, 5010. doi: 10.3390/molecules25215010
Petronio Petronio, G., Pietrangelo, L., Cutuli, M. A., Magnifico, I., Venditti, N., Guarnieri, A., et al. (2022). Emerging evidence on tenebrio molitor immunity: A focus on gene expression involved in microbial infection for host-pathogen interaction studies. Microorganisms 10, 1983. doi: 10.3390/microorganisms10101983
Prescott, J. A., Mitchell, J. P., Cook, S. J. (2021). Inhibitory feedback control of NF-κB signalling in health and disease. Biochem. J. 478, 2619–2664. doi: 10.1042/BCJ20210139
Riera, N., Handique, U., Zhang, Y., Dewdney, M. M., Wang, N. (2017). Characterization of antimicrobial-producing beneficial bacteria isolated from huanglongbing escape citrus trees. Front. Microbiol. 8. doi: 10.3389/fmicb.2017.02415
Righetto, G. M., Lopes, J. L., de, S., Bispo, P. J. M., André, C., Souza, J. M., et al. (2023). Antimicrobial activity of an fmoc-plantaricin 149 derivative peptide against multidrug-resistant bacteria. Antibiotics (Basel) 12, 391. doi: 10.3390/antibiotics12020391
Rybarczyk, M. M., Schafer, J. M., Elm, C. M., Sarvepalli, S., Vaswani, P. A., Balhara, K. S., et al. (2016). Prevention of burn injuries in low- and middle-income countries: A systematic review. Burns 42, 1183–1192. doi: 10.1016/j.burns.2016.04.014
Salas-Jara, M. J., Ilabaca, A., Vega, M., García, A. (2016). Biofilm forming lactobacillus: new challenges for the development of probiotics. Microorganisms 4, 35. doi: 10.3390/microorganisms4030035
Sami Awayid, H., Qassim Mohammad, S. (2022). Prevalence and antibiotic resistance pattern of methicillin-resistant staphylococcus aureus isolated from Iraqi hospitals. Arch. Razi Inst 77, 1147–1156. doi: 10.22092/ARI.2022.357391.2031
Sarvari, M., Mikani, A., Mehrabadi, M. (2020). The innate immune gene Relish and Caudal jointly contribute to the gut immune homeostasis by regulating antimicrobial peptides in Galleria mellonella. Dev. Comp. Immunol. 110, 103732. doi: 10.1016/j.dci.2020.103732
Schuhmann, B., Seitz, V., Vilcinskas, A., Podsiadlowski, L. (2003). Cloning and expression of gallerimycin, an antifungal peptide expressed in immune response of greater wax moth larvae, Galleria mellonella. Arch. Insect Biochem. Physiol. 53, 125–133. doi: 10.1002/arch.10091
Serrano, I., Verdial, C., Tavares, L., Oliveira, M. (2023). The virtuous galleria mellonella model for scientific experimentation. Antibiotics 12, 505. doi: 10.3390/antibiotics12030505
Sharma, P., Elofsson, M., Roy, S. (2020). Attenuation of Pseudomonas aeruginosa infection by INP0341, a salicylidene acylhydrazide, in a murine model of keratitis. Virulence 11, 795–804. doi: 10.1080/21505594.2020.1776979
Shokri, D., Khorasgani, M. R., Mohkam, M., Fatemi, S. M., Ghasemi, Y., Taheri-Kafrani, A. (2018). The inhibition effect of lactobacilli against growth and biofilm formation of pseudomonas aeruginosa. Probiotics Antimicrob. Proteins 10, 34–42. doi: 10.1007/s12602-017-9267-9
Stączek, S., Cytryńska, M., Zdybicka-Barabas, A. (2023). Unraveling the role of antimicrobial peptides in insects. Int. J. Mol. Sci. 24, 5753. doi: 10.3390/ijms24065753
Thoda, C., Touraki, M. (2023). Immunomodulatory properties of probiotics and their derived bioactive compounds. Appl. Sci. 13, 4726. doi: 10.3390/app13084726
Tsai, C. J.-Y., Loh, J. M. S., Proft, T. (2016). Galleria mellonella infection models for the study of bacterial diseases and for antimicrobial drug testing. Virulence 7, 214–229. doi: 10.1080/21505594.2015.1135289
Varma, P., Nisha, N., Dinesh, K. R., Kumar, A. V., Biswas, R. (2011). Anti-infective properties of Lactobacillus fermentum against Staphylococcus aureus and Pseudomonas aeruginosa. J. Mol. Microbiol. Biotechnol. 20, 137–143. doi: 10.1159/000328512
Venditti, N., Vergalito, F., Magnifico, I., Cutuli, M. A., Pietrangelo, L., Cozzolino, A., et al. (2021). The Lepidoptera Galleria mellonella “in vivo” model: A preliminary pilot study on oral administration of Lactobacillus plantarum (now Lactiplantibacillus plantarum). New Microbiol. 44, 42–50.
Keywords: host-pathogen interaction, antimicrobial peptides, Pseudomonas aeruginosa, Galleria mellonella, burn wound infection model, lactic acid bacteria, immunomodulatory activity, burn infection prevention
Citation: Guarnieri A, Venditti N, Cutuli MA, Brancazio N, Salvatore G, Magnifico I, Pietrangelo L, Falcone M, Vergalito F, Nicolosi D, Scarsella F, Davinelli S, Scapagnini G, Petronio Petronio G and Di Marco R (2024) Human breast milk isolated lactic acid bacteria: antimicrobial and immunomodulatory activity on the Galleria mellonella burn wound model. Front. Cell. Infect. Microbiol. 14:1428525. doi: 10.3389/fcimb.2024.1428525
Received: 06 May 2024; Accepted: 16 August 2024;
Published: 06 September 2024.
Edited by:
Kingsley Yin, Rowan University School of Osteopathic Medicine, United StatesReviewed by:
Rajagopal Kammara, Central Food Technological Research Institute (CSIR), IndiaEmoke Pall, University of Agricultural Sciences and Veterinary Medicine of Cluj-Napoca, Romania
Mikaeel Young, Baylor University, United States
Copyright © 2024 Guarnieri, Venditti, Cutuli, Brancazio, Salvatore, Magnifico, Pietrangelo, Falcone, Vergalito, Nicolosi, Scarsella, Davinelli, Scapagnini, Petronio Petronio and Di Marco. This is an open-access article distributed under the terms of the Creative Commons Attribution License (CC BY). The use, distribution or reproduction in other forums is permitted, provided the original author(s) and the copyright owner(s) are credited and that the original publication in this journal is cited, in accordance with accepted academic practice. No use, distribution or reproduction is permitted which does not comply with these terms.
*Correspondence: Giulio Petronio Petronio, Z2l1bGlvLnBldHJvbmlvcGV0cm9uaW9AdW5pbW9sLml0
†ORCID: Antonio Guarnieri, orcid.org/0000-0003-3949-4143
Noemi Venditti, orcid.org/0000-0003-4952-1889
Marco Alfio Cutuli, orcid.org/0000-0003-1753-2399
Natasha Brancazio, orcid.org/0009-0001-2203-3327
Giovanna Salvatore, orcid.org/0000-0001-5427-8911
Irene Magnifico, orcid.org/0000-0003-0691-3524
Laura Pietrangelo, orcid.org/0000-0001-7636-5581
Marilina Falcone, orcid.org/0009-0009-7277-1377
Franca Vergalito, orcid.org/0000-0003-4274-414X
Daria Nicolosi, orcid.org/0000-0003-4822-0363
Franco Scarsella, orcid.org/0009-0000-9320-2178
Sergio Davinelli, orcid.org/0000-0003-2578-7199
Giovanni Scapagnini, orcid.org/0000-0003-1592-5586
Giulio Petronio Petronio, orcid.org/0000-0002-5098-7785
Roberto Di Marco, orcid.org/0000-0001-5075-4597