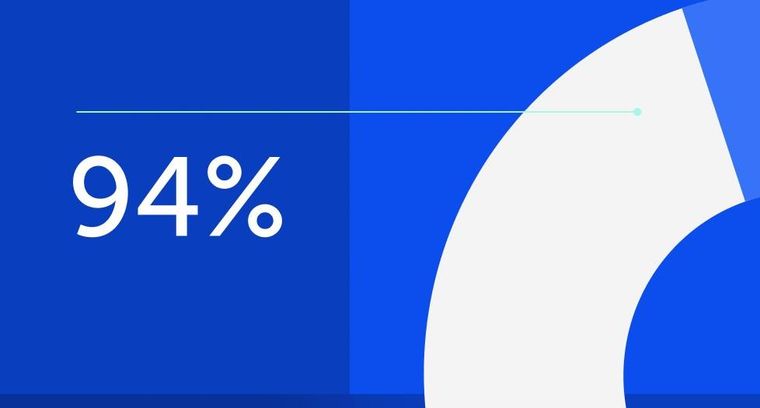
94% of researchers rate our articles as excellent or good
Learn more about the work of our research integrity team to safeguard the quality of each article we publish.
Find out more
ORIGINAL RESEARCH article
Front. Cell. Infect. Microbiol., 31 July 2024
Sec. Bacteria and Host
Volume 14 - 2024 | https://doi.org/10.3389/fcimb.2024.1425367
This article is part of the Research TopicBacteria-Host Interactions: From Infection to CarcinogenesisView all 11 articles
Helicobacter pylori (H. pylori) infection is the primary risk factor for the progress of gastric diseases. The persistent stomach colonization of H. pylori is closely associated with the development of gastritis and malignancies. Although the involvement of progranulin (PGRN) in various cancer types has been well-documented, its functional role and underlying mechanisms in gastric cancer (GC) associated with H. pylori infection remain largely unknown. This report demonstrated that PGRN was up-regulated in GC and associated with poor prognosis, as determined through local and public database analysis. Additionally, H. pylori induced the up-regulation of PGRN in gastric epithelial cells both in vitro and in vivo. Functional studies have shown that PGRN promoted the intracellular colonization of H. pylori. Mechanistically, H. pylori infection induced autophagy, while PGRN inhibited autophagy to promote the intracellular colonization of H. pylori. Furthermore, PGRN suppressed H. pylori-induced autophagy by down-regulating decorin (DCN) through the mTOR pathway. In general, PGRN inhibited autophagy to facilitate intracellular colonization of H. pylori via the PGRN/mTOR/DCN axis. This study provides new insights into the molecular mechanisms underlying the progression of gastric diseases, suggesting PGRN as a potential therapeutic target and prognostic predictor for these disorders.
Helicobacter pylori (H. pylori) is a Gram-negative microaerobic bacterium that colonizes the gastric mucosa. It initiates a chronic inflammatory response, which progresses through a multi-step gastric tumorigenesis cascade known as Correa’s cascade (Thrift et al., 2023). Upon infection, H. pylori establishes long-term colonization on the gastric mucosal surface and subsequently invades large cytoplasmic vacuoles, where it continues to survive and alter the molecular composition of the vacuole (Amieva et al., 2002). H. pylori internalization in gastric epithelial cells exhibit greater resistance to immune response and antibiotic treatment and played a major role in tumor progression (Sit et al., 2020). Therefore, despite acting as an extracellular pathogen, a comprehensive understanding of the intracellular colonization mechanisms of H. pylori is crucial for preventing and treating persistent infections.
Autophagy is an intracellular degradation process that cells use to degrade and recycle cellular components. The process involves the formation of special structures called autophagosomes, which engulf damaged organelles, proteins, other cellular components and invading pathogens. These autophagosomes then fuse with lysosomes, which contain enzymes that break down the contents of the autophagosome for recycling. Autophagy plays a crucial role in maintaining cellular homeostasis, eliminating damaged components (Miller and Thorburn, 2021; Debnath et al., 2023). Pathogenic microorganisms have evolved multiple strategies to regulate or impede autophagy, leading to sustained intracellular survival (Kim et al., 2019; Chidambaram et al., 2022; Zhou et al., 2023). Autophagy has been found to have a complex role in regulating the survival of H. pylori, although the specific mechanisms involved are not yet well understood.
Progranulin (PGRN), known as GEP, GP88, or PC cell-derived multifunctional growth factor, consists of 593 amino acid residues (Anakwe and Gerton, 1990). It is involved in various physiological processes, including cell development, cell cycle progression, vascular and tissue repair, as well as the growth of bone and cartilage (Chen Q. et al., 2022). Numerous studies have shown the abnormal expression of PGRN in various cancers, including gastric cancer (GC), where its overexpression is closely associated with tumor proliferation, aggressiveness and adverse prognostic outcomes (Chen S. et al., 2022; Zhao et al., 2020; Do et al., 2021; Purrahman et al., 2022). Our preceding investigation revealed that H. pylori infection upregulated PGRN expressions in gastric epithelial cells and activated related signaling pathways, thereby enhancing the proliferation and migration of gastric cancer cells (Wang et al., 2011). Therefore, we aim to further investigate the mechanism through which PGRN exerts its oncogenic role. It has been documented that PGRN inhibits autophagy, thereby reducing MHC class I (MHC I) expression, limiting CD8+ T cell infiltration, and promoting immune evasion in pancreatic ductal adenocarcinoma (Cheung et al., 2022). However, the interplay between PGRN and autophagy within gastric mucosal epithelial cells has not been investigated, and it remains unclear whether the upregulation of PGRN triggered by H. pylori infection influences autophagy, potentially facilitating the colonization of H. pylori in these cells.
To further elucidate the mechanism by which PGRN modulates autophagy and contributes to H. pylori colonization, we employed gene chip analysis and identified that decorin (DCN), as the primary target protein of PGRN, indicating its role in regulating H. pylori intracellular colonization. DCN, a small leucine-rich proteoglycan, is crucial in mediating a variety of cellular functions, including proliferation, differentiation, and inflammatory responses, by interacting with multiple growth factors and receptors (Schneider et al., 2021). Moreover, DCN functions as a tumor suppressor in GC, with its expression levels significantly associated with angiogenesis and patient prognosis, suggesting its potential as a therapeutic target for GC (Basak et al., 2021). Recent research has demonstrated that DCN can modulate the tumor microenvironment, thus inhibiting tumor progression by inducing autophagy and apoptosis (Wang et al., 2020; Liu et al., 2022). However, the precise role of DCN in PGRN-mediated autophagy warrants further investigation.
In this study, we elucidated the association between H. pylori intracellular colonization and autophagy, analyzing the impact of PGRN on the internalization of H. pylori and its mechanistic role in inhibiting autophagy via the PGRN/mTOR/DCN pathway.
Human immortalized gastric epithelial cell line GES−1 and human GC cell lines BGC-823 were cultured in RPMI−1640 medium (Gibco, USA), supplemented with 10% newborn bovine serum (Gibco, USA) and maintained in a humidified atmosphere containing 5% CO2 at 37°C. The autophagy inhibitor 3-MA (Selleck, USA) and mTOR inhibitor Everolimus (Selleck, USA) were dissolved in dimethyl sulfoxide (DMSO, China) for use in experiments.
As previously described, the wild-type H. pylori strain 26695 and SS1 were maintained in our laboratory. Briefly, the H. pylori strains were cultured in Brucella broth containing 5% FBS at 37°C under microaerobic conditions. Gastric cells were cocultured with H. pylori 26695 as a concentration of different multiplicity of infection (MOI) for designated durations.
Atrophic gastric specimens were collected from 43 patients undergoing gastroscopic examination at the Affiliated Hospital of Shandong Second Medical University. These samples included H. pylori-positive chronic gastritis (n=24) and H. pylori-negative controls (n=19). 16S rRNA test and 13C-urea breath test were used to demonstrate H. pylori infection status. Patients were regarded as being H. pylori-positive if two tests yielded positive results. None of the patients had taken nonsteroidal anti-inflammatory drugs, antibiotics or proton pump inhibitors in the four weeks prior to the study. Additionally, 35 samples of paraneoplastic tissues and 50 GC tissue specimens were collected. The GC tissue specimens included H. pylori-negative samples (n=11), H. pylori-positive samples (n=32), and 7 samples that were not tested with 13C-urea breath test. Informed consent was obtained from each patient before participation. Data on age, gender, and pertinent clinical history were collected for all subjects in accordance with the approval from the Ethics Committee at Shandong Second Medical University (2022YX045).
Specific pathogen-free (SPF) C56BL/6 mice (female, four weeks old) were used for H. pylori SS1 infection in vivo and purchased from Beijing Huafukang Bio-technology Co (SCXK(Beijing)2019–0008). All mice were randomly divided into two groups: uninfected (n=6) and H. pylori-infected (n=6) based on “complete randomization” rules. Mice were inoculated via oral gavage with 2.2×108 colony forming units of H. pylori strain SS1 every other day for one month. Gastric tissues were collected 24 weeks later for rapid urease testing to confirm successful colonization of H. pylori, followed by additional analyses. All procedures and animal experiments were approved by the Animal Care and Use Committee of Shandong Second Medical University (2021SDL555).
The gene expression levels of PGRN in GC and the clinical information of GC patients were derived from the Gene Expression Profiling Interactive Analysis (GEPIA, http://gepia.cancer-pku.cn/) and The Cancer Genome Atlas Program (TCGA, https://www.cancer.gov/ccg/research/genome-sequencing/tcga) database. Analysis of PGRN malignant expression in GC microenvironment by CTD database (https://www.ctdbase.org/) and TISCH database (http://tisch.comp-genomics.org/home/). Based on the expression of PGRN in patients, the expression levels were classified as high or low using the median expression level as the standard, survival analysis for GC patients was performed using the Kaplan–Meier Plotter (https://kmplot.com/analysis/). Gene set enrichment analysis (GSEA, https://www.gsea-msigdb.org/gsea/index.jsp) algorithm was employed to identify pathways significantly enriched between PGRN low and high tumor cells.
Gastric tissues were fixed in 4% paraformaldehyde, paraffin-embedded, sectioned, deparaffinized with xylene, and rehydrated in ethanol. Following standard protocols, immunohistochemistry was conducted. Color development was achieved using DAB chromogenic solution (ZSGB Biotech), with subsequent hematoxylin staining of nuclei. Observations were made with an orthogonal fluorescence microscope (Olympus, Japan). The AOD is displayed in the bar chart as the measurement metric, which were assessed by ImageJ and checked by the individual pathologist.
The plasmid overexpressing PGRN and its corresponding negative control vector (pcDNA3.1) were successfully constructed and preserved in the laboratory. The PGRN and DCN siRNAs were purchased from Genepharma along with control siRNA (siNC). The lipofectamine 2000 (Invitrogen, USA) facilitated the transfection of plasmids and siRNA into GC cells. All experimental procedures are performed according to the manufacturer’s instructions.
Trizol reagent (Invitrogen) was used to extract the total RNA from the GC cells or gastric tissues according to the manufacturer’s protocol. RNA was reverse-transcribed into cDNA with a ReverTra Ace qPCR RT Kit (Toyobo, Japan). The mRNA expression levels of PGRN, DCN and H. pylori 16S rRNA were determined by using SYBR Green kit (AG, China) and the QuantStudio™ 1 Plus System (Applied Biosystems, USA) according to the protocol of manufacturer. Calculation of target mRNA levels was based on the CT method and normalization to human β-actin expression. The primer sequences are as follows:
PGRN, forward-5’-GGACAGTACTGAAGACTCTG-3’, reverse-5’-GGATGGCAGCTTGTAATGTG-3’;
DCN, forward-5’-GACAACAACAAGCTTACCAGAG-3’, reverse-5’-TGAAAAGACTCACACCCGAATA-3’;
β-actin, forward-5’-AGTTGCGTTACACCCTTTCTTG-3’, reverse-5’-CACCTTCACCGTTCCAGTTTT-3’;
16S rRNA, forward-5’-TGAGTACAAGACCCGGGAAC, reverse-5’-CAGTTCGGATTGTAGGCTGC-3’.
Cell and tissue proteins were lysed using RIPA buffer containing PMSF protease inhibitor (Solarbio, China). The total protein concentration was quantified using a NanoDrop Lite Spectrophotometer (Thermo Scientific, USA). Proteins were then resolved by SDS-PAGE using either 10% or 12% gels and subsequently transferred onto PVDF membranes. The membranes were blocked with 5% nonfat milk at room temperature for one hour before overnight incubation with specific primary antibodies at 4°C. Following primary antibody binding, the membranes were washed and incubated with HRP-conjugated secondary antibodies. HRP-linked anti-mouse IgG (7076) and anti-rabbit IgG (7074) antibodies were from Cell Signal Technology. The protein bands were visualized using an enhanced chemiluminescence (ECL) detection system (EMD Millipore, USA). The bar graph was the intensities of the corresponding bands, which were measured using ImageJ software and normalized to β-actin, dividing the target protein band intensity by the internal control protein band intensity. PGRN (sc-377036), β-actin (sc-47778) antibodies were from Santa Cruz Biotechnology. LC3B(ab192890), DCN (ab277636) antibodies were from Abcam, mTOR (A2445) and phospho-mTOR (AP0115) antibodies were from Abconal Biotechnology.
Cells were collected, fixed in 2% paraformaldehyde, 0.1% glutaraldehyde and in 0.1 mol/L sodium cacodylate for 2 h, subsequently post-fixed in 1% osmium tetroxide (OsO4) for 1.5 h, and then stained with 3% aqueous uranyl acetate for 1 h. Following an additional wash, cells were dehydrated through a graded series of and embedded in Epon-Araldite resin (Canemco, Canada). Ultrathin sections (0.05 μm) were prepared using an ultramicrotome, counterstained with 0.3% lead citrate, and examined on HT7700 (Hitachi, Japan) electron microscopy.
Cells were infected with RFP-LC3B-expressing lentivirus and selected using 2 µg/ml puromycin. Subsequently, they were co-transfected with either PGRN plasmids or siRNAs, followed by infection with H. pylori at MOI 100:1 for 12 h. The formation of LC3B autophagic puncta was then monitored through sequential scanning with confocal laser scanning microscopy (CLSM) (Leica, Germany).
The GPA was performed as previous studies to assess the invasion of gastric cells by H. pylori. H. pylori was introduced to gastric cells seeded in 24-well plates with antibiotic-free RPMI 1640 medium at an MOI of 100:1 for various durations. Unattached bacteria were removed by washing with 1 mL of warm PBS per well, followed by gentamicin (100 μg/ml, G1272, Sigma) treatment for 1 h to eliminate extracellular bacteria. After incubation, cells were washed and lysed with 0.5% saponin (47036, Sigma) in PBS at 37°C for 15 min. The lysates were then serially diluted from 101 to 103 into Brucella broth solid medium to culture viable intracellular H. pylori and the colonies were counted after an incubation period of 3–5 days.
The experimental data were statistically processed using SPSS 24.0 statistical software. Experimental data of three independent repeats were shown as mean ± standard deviation (SD). Paired t-test was used to compare the means between two groups; one-way ANOVA and multi way ANOVA was used for multiple groups. Survival analysis was performed using Kaplan-Meier. Figures were created in GraphPad Prism 8.0. P<0.01 and P<0.05 were considered statistically significant.
PGRN plays a significant and pivotal role in the modulation and progression of tumorous growths (Dong et al., 2023). We firstly elucidated the relationship between PGRN and GC. Our findings revealed a marked upregulation of PGRN in GC specimens compared with normal gastric tissues based on TCGA database (Figures 1A, B). Furthermore, PGRN levels were significantly higher in malignant cells than in other cellular constituents of the GC microenvironment (Figure 1C). Notably, PGRN was highest in inflammation phenotype among the various malignant phenotypes studied (Figure 1D). Building on previous studies that linked increased PGRN with poor prognosis in certain cancers, Kaplan-Meier Plotter survival analysis also showed that higher PGRN expression is associated with poorer overall survival in GC patients (Figure 1E). To evaluate the association between PGRN expression and clinicopathological parameters, we analysed PGRN mRNA expression in TCGA database. The results showed that up-regulation of PGRN corresponded with advanced tumor/node/metastasis (TNM) stage and correlated with the increased tumor size and/or the extent of invasion into adjacent tissues (Supplementary Table 1). We further used clinical gastric tissues to prove the correlation between PGRN and GC, demonstrating higher expression of PGRN in GC compared to normal gastric tissues (Figure 1F). Collectively, these findings indicate that PGRN is up-regulated in GC and is indicative of poor prognosis.
Figure 1 PGRN is up-regulated in GC and associated with poor prognosis. (A) Analysis of mRNA expression level of PGRN in GC tissues and non-tumor tissues according to TCGA database, with T representing GC tissue and N representing normal gastric mucosal epithelial tissue. (B) PGRN expression levels in GC and adjacent normal tissues across TCGA. The lines between the samples are one-to-one paired GC and para-cancerous tissue. (C) TISCH database analysis of PGRN expression in the microenvironment of GC (STAD-GSE134520). The number means the expression levels of PGRN in different cell types. (D) CTD database analysis of PGRN expression in malignant phenotype. (E) Kaplan-Meier analysis of overall survival (OS) in GC patients based on PGRN expression level. (F) Immunohistochemistry staining of PGRN in human adjacent normal and GC tissues. Scale bars, 20 μm. (*P<0.05, **P<0.01, ***P<0.001).
H. pylori infection is one of the major causes of chronic gastritis and GC. We further explore the association between H. pylori infection and PGRN. GSEA confirmed a significant association between PGRN mRNA expression and H. pylori infection (Figure 2A). To elucidate the clinical significance of PGRN in gastritis, we analyzed gastric tissues from patients at varying stages of gastritis and found a progressive increase in PGRN expression, ranging from normal gastric mucosa through superficial gastritis to atrophic gastritis (Figure 2B). Furthermore, comparative analysis revealed that PGRN mRNA expression in H. pylori-positive chronic gastritis tissues was higher than that in H. pylori-negative counterparts (Figure 2C). In vitro experiments demonstrated that H. pylori infection promoted PGRN expression in gastric epithelial cells (Figure 2D). This up-regulation was corroborated in vivo both in H. pylori-negative, H. pylori-positive GC tissues and a mouse model infected with H. pylori (Figures 2E–G). Therefore, it is evident that H. pylori triggers the up-regulation of PGRN in gastric epithelial cells.
Figure 2 H. pylori induces the up-regulation of PGRN in gastric epithelial cells. (A) Enrichment plots of gene expression signatures for H. pylori infection according to PGRN mRNA expression in a GSEA analysis of TCGA. (B) Immunohistochemistry staining of PGRN in human normal, superficial gastritis and atrophic gastritis tissues. Scale bars, 20 μm. (C) qRT-PCR analysis of PGRN mRNA expression level in H. pylori-negative (n=19) and H. pylori-positive (n=24) chronic gastritis tissue samples. (D) Western blot of PGRN in in BGC-823 and GES-1 cells infected with H. pylori (MOI = 100) for 0, 6, 12 and 24 h. (E) Immunohistochemistry staining of PGRN in H. pylori-negative (n=11) and H. pylori-positive (n=32) GC tissues. Scale bars, 20 μm. (F) Immunohistochemistry staining of PGRN in containing H. pylori-negative and H. pylori- positive gastric tissues of mice. Scale bars, 50 μm (20X), 20 μm (40X). (G) Western blot of PGRN in H. pylori-negative and H. pylori-positive gastric tissues of mice. (*P<0.05, **P<0.01, ***P<0.001).
Although H. pylori was previously considered an extracellular bacterium, increasing evidence has shown that it can survive and even multiply inside gastric epithelial cells (Dubois and Borén, 2007). Therefore, our study aimed to investigate the impact of PGRN on the intracellular colonization of H. pylori. Utilizing H. pylori 16S rRNA and colony forming units assay followed by gentamicin treatment, we demonstrated the colonization of H. pylori within gastric epithelial cells (Figures 3A, B). Furthermore, overexpression of PGRN promoted the intracellular colonization of H. pylori into gastric epithelial cells, whereas knockdown of PGRN got the opposite effect (Figures 3C–E). These results indicate that PGRN promotes the intracellular colonization of H. pylori.
Figure 3 PGRN promotes the intracellular colonization of H. pylori. (A) qRT-PCR analysis of 16S rRNA in BGC-823 and GES-1 cells infected with H. pylori for 6 h, 12 h (n=3). (B) CFU analysis in BGC-823 and GES-1 cells infected with H. pylori (MOI = 100) for 3, 6, 12 and 24 h, then followed with gentamicin protection assay. (C) qRT-PCR analysis of PGRN mRNA expression level in BGC-823 and GES-1 cells transfected with PGRN siRNA or WT PGRN (n=3). (D) CFU analysis in BGC-823 cells transfected with PGRN siRNA or WT PGRN following H. pylori infection for indicated times, then followed with gentamicin protection assay (n=3). (E) qRT-PCR analysis of 16S rRNA levels in BGC-823 and GES-1 cells transfected with PGRN siRNA or WT PGRN following H. pylori infection for 6 h, 12 h (n=3). (*P<0.05, **P<0.01, ***P<0.001).
Autophagy is involved in multiple biological processes regulated by H. pylori infection (Yang et al., 2022). To ascertain the effect of H. pylori on autophagy within gastric epithelial cells, we investigated the expression of LC3BI/II and the formation of RFP-LC3B puncta, a reliable autophagy marker, following H. pylori infection. Our findings showed that H. pylori infection promoted LC3B expression and RFP-LC3B puncta formation (Supplementary Figures 1A, B). These results indicate that H. pylori infection induces autophagy in gastric epithelial cells.
In order to investigate the underlying mechanism of PGRN in facilitating intracellular colonization of H. pylori, we employed gene microarray high pathway screening to examine the functions of PGRN involved in the regulation, revealing a close association between PGRN and the autophagic process in gastric epithelial cells (Supplementary Figure 1C). Meanwhile, GSEA highlighted the involvement of PGRN in regulating lysosome-associated pathways and cellular endocytosis (Supplementary Figures 1D, E), indicating a potential mechanism through which PGRN may influence H. pylori-induced autophagy. Furthermore, we found that down-regulation of PGRN significantly increased the expression of LC3B, while overexpression of PGRN had the opposite effects (Figure 4A). Using TEM, we also observed a significant increase in autophagosome formation in gastric epithelial cells following PGRN interference (Figure 4B). CLSM analysis further confirmed the difference in LC3B autophagy puncta formation corresponding with PGRN expression (Figure 4C). More importantly, the inhibition of 16S rRNA of H. pylori due to PGRN knockdown could be rescued in the cells pretreated with 3-MA (Figure 4D). Taken together, we confirm that PGRN promotes H. pylori colonization in gastric epithelial cells by inhibiting cellular autophagy.
Figure 4 PGRN inhibits autophagy to promote intracellular colonization of H. pylori. (A) Western blot of LC3B in BGC-823 cells transfected with PGRN siRNA or WT PGRN following H. pylori infection for 12 h. (B) TEM images of autophagosomes in BGC-823 cells transfected with PGRN siRNA. Scale bars, 2 μm. (C) CLSM images of LC3B autophagy puncta in BGC-823 cells with stably expressing RFP-LC3B transfected with PGRN siRNA or WT PGRN following H. pylori infection. Scale bars, 10 μm. (D) qRT-PCR analysis of 16S rRNA levels in BGC-823 and GES-1 cells transfected with PGRN siRNA or WT PGRN following H. pylori infection for 12 h (MOI=100:1), with or without a pretreatment of the autophagy inhibitor 3-MA (10 nM, 2 h) (n=3). (*P<0.05, **P<0.01, ***P<0.001).
To investigate the role of PGRN in regulating autophagy, a gene microarray was conducted to predict the interaction proteins of PGRN, and several genes were identified as a potential PGRN-associated protein (Figure 5A; Supplementary Table 2). Subsequent validation experiments confirmed that PGRN could negatively regulate DCN expression (Figure 5B). To confirm the effects of DCN on autophagy in gastric epithelial cells, we detected LC3B expression after down-regulation of DCN. Results showed that DCN knockdown inhibited autophagy induced by H. pylori infection (Figures 5C, D). Additionally, down-regulation of DCN rescued the level of LC3B, which was increased by PGRN knockdown (Figure 5E). Subsequently, the effect of DCN on intracellular bacterial colonization was investigated, showing that down-regulation of DCN enhanced the intracellular colonization of H. pylori (Figure 5F). Furthermore, co-transfection with si-PGRN and si-DCN attenuated the reduction of intracellular H. pylori colonization initially induced by PGRN knockdown (Figure 5G). These findings indicate that PGRN inhibits H. pylori-induced autophagy via down-regulation of DCN.
Figure 5 PGRN suppresses H. pylori-induced autophagy via down-regulation of DCN. (A) Gene microarray analysis of PGRN downstream target genes shown as volcano plots. (B) Western blot of DCN in BGC-823 cells transfected with WT PGRN and PGRN siRNA. (C) Western blot of DCN in BGC-823 cells transfected with DCN siRNA. (D) Western blot of LC3B in BGC-823 cells transfected with DCN siRNA following H. pylori infection for 12 h. (E) Western blot of LC3B in BGC-823 cells transfected with PGRN or/and DCN siRNA. (F) qRT-PCR analysis of 16S rRNA levels in BGC-823 cells transfected with DCN siRNA following H. pylori infection for 12 h (n=3). (G) qRT-PCR analysis of 16S rRNA levels in BGC-823 cells transfected with DCN siRNA, PGRN siRNA following H. pylori infection for 6, 12 h (n=3). (*P<0.05, **P<0.01, ***P<0.001, ns, not significant).
The biogenesis of autophagic vesicles is intricately linked to the regulation by upstream pathways, including the mechanistic target of the rapamycin (mTOR) pathway (Pei et al., 2023). GSEA analysis revealed the involvement of PGRN in modulating the mTOR pathway (Figure 6A), suggesting that PGRN may regulate autophagy by influencing DCN expression through the mTOR signaling pathway. Upon the siRNA-mediated down-regulation of PGRN, an increase in phosphorylated mTOR (p-mTOR) levels was observed, with a corresponding alteration following PGRN overexpression (Figure 6B). Furthermore, up-regulation of DCN by si-PGRN was rescued via inhibiting mTOR (Figure 6C). Taken together, we confirmed that PGRN down-regulated DCN by inhibiting the mTOR pathway. And these findings highlight the crucial role of the mTOR pathway in mediating the effects of PGRN on DCN expression, autophagy and intracellular colonization.
Figure 6 PGRN down-regulates DCN by inhibiting the mTOR pathway. (A) GSEA analyzed the involvement of PGRN in the mTOR pathway. (B) Western blot of mTOR signaling in BGC-823 cells transfected with PGRN siRNA or WT PGRN. (C) Western blot of DCN in BGC-823 cells transfected with PGRN siRNA or WT PGRN, then treated with Everolious (10 nM) for 2 h. (*P<0.05).
H. pylori, a significant risk factor closely associated with gastric carcinogenesis, has become a focal point of current research due to the urgent need to improve diagnostic and therapeutic strategies (Malfertheiner et al., 2023). Once individuals acquire H. pylori infection, the pathogen can persists in the gastric mucosa for a lifetime if left untreated. Although H. pylori is considered an extracellular pathogen, it also has the ability to invade and colonize host cells, thereby evading clearance by the immune system and causing long-term infection. Zhang discovered that H. pylori infection can reduce the sensitivity of Toll-like receptors 6 (TLR6) and down-regulate the expression of inflammatory factors, leading to persistent infection (Zhang et al., 2024). Our study also found that H. pylori is capable of intracellular invasion, colonization, and survival, with this ability increasing over time, as shown by intracellular 16S rRNA detection and colony formation after gentamicin treatment. However, the mechanisms underlying H. pylori-induced intracellular colonization remain unclear.
Autophagy is a cellular “cleanup” process that cells use to degrade and recycle unnecessary or dysfunctional cellular components. It plays a crucial role in maintaining cellular homeostasis, removing damaged organelles, clearing protein aggregates, and combating invading pathogens like bacteria, parasites, and viruses. However, pathogens can evade host cell elimination through various mechanisms, resulting in persistent bacterial infections. Studies has shown that the heparin-binding hemagglutinin (HBHA) of Mycobacterium tuberculosis inhibited autophagy in macrophages via Toll-like receptor 4, thereby promoting intracellular survival (Zheng et al., 2024). Similarly, Uropathogenic Escherichia coli PldA suppressed pre-autophagosomal structures, decreasing lysosomal cytotoxicity and escaping host immunity (Li et al., 2024). Furthermore, H. pylori also developed highly evolved mechanisms to escape autophagic degradation, manipulate autophagic pathways, and reconstruct autophagosomal compartments for survival in gastric cells (Capurro et al., 2020). Therefore, a key mechanism for H. pylori to evade host immune responses and maintain infection involves inhibiting autophagy and facilitating intracellular colonization in gastric epithelial cells. In our study, we discovered that H. pylori infection promoted the formation of LC3B autophagy puncta and up-regulated the expression of the autophagy-associated protein LC3B, while inhibiting autophagy using 3-MA resulted in increased intracellular bacterial loading and survival. However, the mechanism by which H. pylori regulates autophagy are complex and requires further study.
PGRN is a growth factor that plays a crucial role in various biological processes, including inflammation, wound healing, neurodegeneration and cancer development and progression. It has been reported that PGRN modulates PD-1 expression in tumor-associated macrophages (TAMs), promotes CD8+ T cell rejection, and induces immune escape from breast cancer (Fang et al., 2021). Our previous research indicated that H. pylori can upregulate the expression of PGRN in gastric mucosal epithelial cells through the p38 MAPK and MEK1/2 signaling pathways. The elevated expression of PGRN can subsequently modulate G2/M stage and CDK4 to promote cell cycle progression, leading to the proliferation and migration of gastric cancer cells (Wang et al., 2011; Yang et al., 2017; Ren et al., 2022). While the intricate mechanisms by which PGRN regulate cell cycle and promote proliferation requires further study. Additionally, several studies have demonstrated that PGRN possesses pro-lysosomal functions, such as regulating protease activity, promoting lysosomal acidification, and facilitating protein trafficking (Tanaka et al., 2017; Zhou et al., 2017a, Zhou et al., 2017b). Therefore, it is worth investigating how H. pylori-induced upregulation of PGRN expression promotes lysosomal function in gastric mucosal epithelial cells. In this study, we present evidence that H. pylori-mediated PGRN induction, both in vitro and in vivo, suppresses autophagy, thus promoting the intracellular colonization and survival of H. pylori in gastric epithelial cells. Therefore, our findings may provide a novel mechanism that high expression of PGRN can inhibit autophagy in gastric mucosal cells, suppress pro-lysosomal functions, leading to the failure in eliminating intracellular H. pylori, resulting in persistent infection and proliferation of H. pylori in the host cells.
To elucidate the molecular mechanisms underlying PGRN function, we employed high-throughput gene chip screening and bioinformatic analyses, which identified DCN as a probable functional target. Our findings demonstrated that DCN expression is up-regulated in PGRN-knockdown gastric epithelial cells. As a matrix-derived proteoglycan, DCN functions as a partial agonist for a variety of biological activities, directly interacting with a wide range of receptor tyrosine kinases (RTKs) and activating autophagy (Neill et al., 2017). Our data indicated that H. pylori infection triggered autophagy in gastric epithelial cells, while downregulation of DCN expression curtailed autophagy thus facilitating intracellular H. pylori colonization. Meanwhile, PGRN-mediated cellular autophagy and intracellular colonization were rescued by DCN. The autophagy inhibited by DCN downregulation may result from reduced interactions with PTKs, which will be further investigated in subsequent studies. Additionally, PGRN is significantly enriched in the mTOR pathway. The mTOR complex plays a critical role not only in gene transcription regulation and tumor metabolism but also in the regulation of autophagy and apoptosis. Our study shows that PGRN can inhibit mTOR phosphorylation to down-regulate DCN expression. Chen et al. indicated that Nobiletin can inhibit the protective effect of autophagy in GC cells through the PI3K/Akt/mTOR pathway (Chen et al., 2024). Additionally, Wang et al. proposed that Vitamin D3 promotes autophagy in GC cells by mediating AMPK/mTOR pathway (Wang et al., 2024). Therefore, the upregulated PGRN by H. pylori may inhibit mTOR phosphorylation through the regulation of PI3K/AKT and AMPK. Additional experiments using the mTOR inhibitor Everolimus confirmed that PGRN impeded H. pylori-induced autophagy in gastric epithelial cells by modulating DCN expression through the mTOR pathway.
In conclusion, H. pylori, previously regarded as an extracellular bacterium, can colonize and even multiply inside gastric epithelial cells. Our study revealed that H. pylori infection induces upregulation of PGRN, which in turn facilitates the intracellular colonization of H. pylori by attenuating cellular autophagy. This suppression occurs via the downregulation of DCN, mediated by the inhibition of the mTOR pathway (Figure 7). Our findings provide a novel mechanism by which PGRN enhances H. pylori colonization and promotes proliferation of gastric epithelial cells. Targeting PGRN in H. pylori infection and GC may offer new opportunities for treatment strategies in the future.
The datasets presented in this study can be found in online repositories. The name of the repository and accession number can be found below: https://www.ncbi.nlm.nih.gov/geo/, GSE266076.
The studies involving humans were approved by the Ethics Committee at Shandong Second Medical University (2022YX045). The studies were conducted in accordance with the local legislation and institutional requirements. The participants provided their written informed consent to participate in this study. The animal study was approved by the Animal Care and Use Committee of Shandong Second Medical University (2021SDL555). The study was conducted in accordance with the local legislation and institutional requirements. Written informed consent was obtained from the individual(s) for the publication of any potentially identifiable images or data included in this article.
LL: Writing – original draft, Project administration, Methodology, Investigation, Software. MX: Writing – original draft, Methodology, Investigation, Software, Project administration. JZ: Writing – original draft, Investigation, Project administration, Software, Methodology. ZR: Writing – original draft, Investigation, Methodology, Project administration, Software. WS: Writing – original draft, Funding acquisition, Methodology. XD: Writing – original draft, Methodology. XF: Writing – original draft, Methodology. PL: Writing – original draft, Writing – review & editing, Funding acquisition, Project administration, Validation, Formal analysis, Supervision, Software, Investigation, Methodology, Visualization, Resources. HW: Writing – original draft, Writing – review & editing, Supervision, Project administration, Validation, Methodology, Investigation, Formal analysis, Funding acquisition, Visualization, Resources, Software.
The author(s) declare financial support was received for the research, authorship, and/or publication of this article. This work was supported by grants from the Natural Science Foundation of Shandong Province (ZR2022MH172, ZR2022QH165), Development Programme for Young Innovation Teams in Shandong Higher Education Institutions (2023KJ254) and Weifang Science and Technology Development Programme (2023YX042).
We would like to give our sincere gratitude to the reviewers for their constructive comments, and members of our research laboratories for reading and guidance.
The authors declare that the research was conducted in the absence of any commercial or financial relationships that could be construed as a potential conflict of interest.
All claims expressed in this article are solely those of the authors and do not necessarily represent those of their affiliated organizations, or those of the publisher, the editors and the reviewers. Any product that may be evaluated in this article, or claim that may be made by its manufacturer, is not guaranteed or endorsed by the publisher.
The Supplementary Material for this article can be found online at: https://www.frontiersin.org/articles/10.3389/fcimb.2024.1425367/full#supplementary-material
Amieva, M. R., Salama, N. R., Tompkins, L. S., Falkow, S. (2002). Helicobacter pylori enter and survive within multivesicular vacuoles of epithelial cells. Cell Microbiol. 4, 677–690. doi: 10.1046/j.1462-5822.2002.00222.x
Anakwe, O. O., Gerton, G. L. (1990). Acrosome biogenesis begins during meiosis: evidence from the synthesis and distribution of an acrosomal glycoprotein, acrogranin, during Guinea pig spermatogenesis. Biol. Reprod. 42, 317–328. doi: 10.1095/biolreprod42.2.317
Basak, D., Jamal, Z., Ghosh, A., Mondal, P. K., Dey Talukdar, P., Ghosh, S., et al. (2021). Reciprocal interplay between asporin and decorin: Implications in gastric cancer prognosis. PloS One 16, e0255915. doi: 10.1371/journal.pone.0255915
Capurro, M. I., Prashar, A., Jones, N. L. (2020). MCOLN1/TRPML1 inhibition - a novel strategy used by Helicobacter pylori to escape autophagic killing and antibiotic eradication therapy in vivo. Autophagy 16, 169–170. doi: 10.1080/15548627.2019.1677322
Chen, S., Bie, M., Wang, X., Fan, M., Chen, B., Shi, Q., et al. (2022). PGRN exacerbates the progression of non-small cell lung cancer via PI3K/AKT/Bcl-2 antiapoptotic signaling. Genes Dis. 9, 1650–1661. doi: 10.1016/j.gendis.2021.05.005
Chen, M., Li, H., Zheng, S., Shen, J., Chen, Y., Li, Y., et al. (2024). Nobiletin targets SREBP1/ACLY to induce autophagy-dependent cell death of gastric cancer cells through PI3K/Akt/mTOR signaling pathway. Phytomedicine 128, 155360. doi: 10.1016/j.phymed.2024.155360
Chen, Q., Wu, Z., Xie, L. (2022). Progranulin is essential for bone homeostasis and immunology. Ann. N Y Acad. Sci. 1518, 58–68. doi: 10.1111/nyas.14905
Cheung, P. F., Yang, J., Fang, R., Borgers, A., Krengel, K., Stoffel, A., et al. (2022). Progranulin mediates immune evasion of pancreatic ductal adenocarcinoma through regulation of MHCI expression. Nat. Commun. 13, 156. doi: 10.1038/s41467-021-27088-9
Chidambaram, S. B., Essa, M. M., Rathipriya, A. G., Bishir, M., Ray, B., Mahalakshmi, A. M., et al. (2022). Gut dysbiosis, defective autophagy and altered immune responses in neurodegenerative diseases: Tales of a vicious cycle. Pharmacol. Ther. 231, 107988. doi: 10.1016/j.pharmthera.2021.107988
Debnath, J., Gammoh, N., Ryan, K. M. (2023). Autophagy and autophagy-related pathways in cancer. Nat. Rev. Mol. Cell Biol. 24, 560–575. doi: 10.1038/s41580-023-00585-z
Do, I. G., Jung, K. U., Koo, D. H., Lee, Y. G., Oh, S., Kim, K., et al. (2021). Clinicopathological characteristics and outcomes of gastrointestinal stromal tumors with high progranulin expression. PloS On. 16, e0245153. doi: 10.1371/journal.pone.0245153
Dong, Y., Tan, H., Wang, L., Liu, Z. (2023). Progranulin promoted the proliferation, metastasis, and suppressed apoptosis via JAK2-STAT3/4 signaling pathway in papillary thyroid carcinoma. Cancer Cell In. 23, 191. doi: 10.1186/s12935-023-03033-2
Dubois, A., Borén, T. (2007). Helicobacter pylori is invasive and it may be a facultative intracellular organism. Cell Microbiol. 9, 1108–1116. doi: 10.1111/j.1462-5822.2007.00921.x
Fang, W., Zhou, T., Shi, H., Yao, M., Zhang, D., Qian, H., et al. (2021). Progranulin induces immune escape in breast cancer via up-regulating PD-L1 expression on tumor-associated macrophages (TAMs) and promoting CD8(+) T cell exclusion. J. Exp. Clin. Cancer Res. 40, 4. doi: 10.1186/s13046-020-01786-6
Kim, T. S., Jin, Y. B., Kim, Y. S., Kim, S., Kim, J. K., Lee, H. M., et al. (2019). SIRT3 promotes antimycobacterial defenses by coordinating mitochondrial and autophagic functions. Autophagy 15, 1356–1375. doi: 10.1080/15548627.2019.1582743
Li, X., Jiang, L., Zhang, S., Zhou, J., Liu, L., Jin, C., et al. (2024). Uropathogenic Escherichia coli subverts host autophagic defenses by stalling pre-autophagosomal structures to escape lysosome exocytosis. J. Infect. Dis. 8, jiae063. doi: 10.1093/infdis/jiae063
Liu, J., Zhu, S., Zeng, L., Li, J., Klionsky, D. J., Kroemer, G., et al. (2022). DCN released from ferroptotic cells ignites AGER-dependent immune responses. Autophagy 18, 2036–2049. doi: 10.1080/15548627.2021.2008692
Malfertheiner, P., Camargo, M. C., El-Omar, E., Liou, J. M., Peek, R., Schulz, C., et al. (2023). Helicobacter pylori infection. Nat. Rev. Dis. Primers. 9, 19. doi: 10.1038/s41572-023-00431-8
Miller, D. R., Thorburn, A. (2021). Autophagy and organelle homeostasis in cancer. Dev. Cell. 56, 906–918. doi: 10.1016/j.devcel.2021.02.010
Neill, T., Sharpe, C., Owens, R. T., Iozzo, R. V. (2017). Decorin-evoked paternally expressed gene 3 (PEG3) is an upstream regulator of the transcription factor EB (TFEB) in endothelial cell autophagy. J. Biol. Chem. 292, 16211–16220. doi: 10.1074/jbc.M116.769950
Pei, F., Ma, L., Jing, J., Feng, J., Yuan, Y., Guo, T., et al. (2023). Sensory nerve niche regulates mesenchymal stem cell homeostasis via FGF/mTOR/autophagy axis. Nat. Commun. 14, 344. doi: 10.1038/s41467-023-35977-4
Purrahman, D., Mahmoudian-Sani, M. R., Saki, N., Wojdasiewicz, P., Kurkowska-Jastrzębska, I., Poniatowski Ł, A. (2022). Involvement of progranulin (PGRN) in the pathogenesis and prognosis of breast cancer. Cytokine 151, 155803. doi: 10.1016/j.cyto.2022.155803
Ren, Z., Li, J., Du, X., Shi, W., Guan, F., Wang, X., et al. (2022). Helicobacter pylori-induced progranulin promotes the progression of the gastric epithelial cell cycle by regulating CDK4. J. Microbiol. Biotechnol. 28, 844–854. doi: 10.4014/jmb.2203.03053
Schneider, M., Dillinger, A. E., Ohlmann, A., Iozzo, R. V., Fuchshofer, R. (2021). Decorin-an antagonist of TGF-β in astrocytes of the optic nerve. Int. J. Mol. Sci. 22, 7660. doi: 10.3390/ijms22147660
Sit, W. Y., Chen, Y. A., Chen, Y. L., Lai, C. H., Wang, W. C. (2020). Cellular evasion strategies of Helicobacter pylori in regulating its intracellular fate. Semin. Cell Dev. Biol. 101, 59–67. doi: 10.1016/j.semcdb.2020.01.007
Tanaka, Y., Suzuki, G., Matsuwaki, T., Hosokawa, M., Serrano, G., Beach, T. G., et al. (2017). Progranulin regulates lysosomal function and biogenesis through acidification of lysosomes. Hum. Mol. Genet. 1, 969–988. doi: 10.1093/hmg/ddx011
Thrift, A. P., Wenker, T. N., El-Serag, H. B. (2023). Global burden of gastric cancer: epidemiological trends, risk factors, screening and prevention. Nat. Rev. Clin. Oncol. 20, 338–349. doi: 10.1038/s41571-023-00747-0
Wang, Y., He, Q., Rong, K., Zhu, M., Zhao, X., Zheng, P., et al. (2024). Vitamin D3 promotes gastric cancer cell autophagy by mediating p53/AMPK/mTOR signaling. Front. Pharmacol. 14. doi: 10.3389/fphar.2023.1338260
Wang, H., Sun, Y., Liu, S., Yu, H., Li, W., Zeng, J., et al. (2011). Upregulation of progranulin by Helicobacter pylori in human gastric epithelial cells via p38MAPK and MEK1/2 signaling pathway: role in epithelial cell proliferation and migration. FEMS Immunol. Med. Microbiol. 63, 82–92. doi: 10.1111/j.1574-695X.2011.00833.x
Wang, Y., Zhang, H., Zhang, Y., Li, X., Hu, X., Wang, X. (2020). Decorin promotes apoptosis and autophagy via suppressing c-Met in HTR-8 trophoblasts. Reproduction 159, 669–677. doi: 10.1530/REP-19-0458
Yang, D., Li, R., Wang, H., Wang, J., Han, L., Pan, L., et al. (2017). Clinical implications of progranulin in gastric cancer and its regulation via a positive feedback loop involving AKT and ERK signaling pathways. Mol. Med. Rep. 16, 9685–9691. doi: 10.3892/mmr.2017.7796
Yang, Y., Shu, X., Xie, C. (2022). An overview of autophagy in Helicobacter pylori infection and related gastric cancer. Front. Cell Infect. Microbiol. 12. doi: 10.3389/fcimb.2022.847716
Zhang, X., He, Y., Zhang, X., Fu, B., Song, Z., Wang, L., et al. (2024). Sustained exposure to Helicobacter pylori induces immune tolerance by desensitizing TLR6. Gastric Cancer. 27, 324–342. doi: 10.1007/s10120-023-01461-7
Zhao, Z., Li, E., Luo, L., Zhao, S., Liu, L., Wang, J., et al. (2020). A PSCA/PGRN-NF-κB-integrin-α4 axis promotes prostate cancer cell adhesion to bone marrow endothelium and enhances metastatic potential. Mol. Cancer Res. 18, 501–513. doi: 10.1158/1541-7786.MCR-19-0278
Zheng, Q., Li, Z., Zhou, Y., Li, Y., Gong, M., Sun, H., et al. (2024). Heparin-binding hemagglutinin of Mycobacterium tuberculosis inhibits autophagy via TLR4 and drives M2 polarization in macrophages. J. Infect. Dis. 24, jiae030. doi: 10.1093/infdis/jiae030
Zhou, X., Paushter, D. H., Feng, T., Pardon, C. M., Mendoza, C. S., Hu, F. (2017a). Regulation of cathepsin D activity by the FTLD protein progranulin. Acta Neuropathol. 134, 151–153. doi: 10.1007/s00401-017-1719-5
Zhou, X., Sun, L., Bracko, O., Choi, J. W., Jia, Y., Nana, A. L., et al. (2017b). Impaired prosaposin lysosomal trafficking in frontotemporal lobar degeneration due to progranulin mutations. Nat. Commun. 25, 15277. doi: 10.1038/ncomms15277
Keywords: Helicobacter pylori, autophagy, PGRN, intracellular colonization, DCN
Citation: Liu L, Xiang M, Zhou J, Ren Z, Shi W, Du X, Fu X, Li P and Wang H (2024) Progranulin inhibits autophagy to facilitate intracellular colonization of Helicobacter pylori through the PGRN/mTOR/DCN axis in gastric epithelial cells. Front. Cell. Infect. Microbiol. 14:1425367. doi: 10.3389/fcimb.2024.1425367
Received: 29 April 2024; Accepted: 11 July 2024;
Published: 31 July 2024.
Edited by:
Maurizio Sanguinetti, Catholic University of the Sacred Heart, ItalyReviewed by:
Shintaro Nakajima, California Institute of Technology, United StatesCopyright © 2024 Liu, Xiang, Zhou, Ren, Shi, Du, Fu, Li and Wang. This is an open-access article distributed under the terms of the Creative Commons Attribution License (CC BY). The use, distribution or reproduction in other forums is permitted, provided the original author(s) and the copyright owner(s) are credited and that the original publication in this journal is cited, in accordance with accepted academic practice. No use, distribution or reproduction is permitted which does not comply with these terms.
*Correspondence: Panpan Li, bGlwYW5wYW5Ac2RzbXUuZWR1LmNu; Hongyan Wang, d2FuZ2h5QHNkc211LmVkdS5jbg==
†These authors have contributed equally to this work and share first authorship
Disclaimer: All claims expressed in this article are solely those of the authors and do not necessarily represent those of their affiliated organizations, or those of the publisher, the editors and the reviewers. Any product that may be evaluated in this article or claim that may be made by its manufacturer is not guaranteed or endorsed by the publisher.
Research integrity at Frontiers
Learn more about the work of our research integrity team to safeguard the quality of each article we publish.