- 1SALUVET, Animal Health Department, Complutense University of Madrid, Madrid, Spain
- 2Institute for Parasitology, Vetsuisse Faculty, University of Bern, Bern, Switzerland
As for many other organisms, CRISPR-Cas9 mediated genetic modification has gained increasing importance for the identification of vaccine candidates and drug targets in Neospora caninum, an apicomplexan parasite causing abortion in cattle and neuromuscular disease in dogs. A widely used approach for generating knock-out (KO) strains devoid of virulence factors is the integration of a drug selectable marker such as mutated dihydrofolate reductase-thymidylate synthase (mdhfr-ts) into the target gene, thus preventing the synthesis of respective protein and mediating resistance to pyrimethamine. However, CRISPR-Cas9 mutagenesis is not free of off-target effects, which can lead to integration of multiple mdhfr-ts copies into other sites of the genome. To determine the number of integrated mdhfr-ts in N. caninum, a duplex quantitative TaqMan PCR was developed. For this purpose, primers were designed that amplifies a 106 bp fragment from wild-type (WT) parasites corresponding to the single copy wtdhfrs-ts gene, as well as the mutated mdhfrs-ts present in KO parasites that confers resistance and were used simultaneously with primers amplifying the diagnostic NC5 gene. Thus, the dhfr-ts to NC5 ratio should be approximately 1 in WT parasites, while in KO parasites with a single integrated mdhrf-ts gene this ratio is doubled, and in case of multiple integration events even higher. This approach was applied to the Neospora KO strains NcΔGRA7 and NcΔROP40. For NcΔGRA7, the number of tachyzoites determined by dhfr-ts quantification was twice the number of tachyzoites determined by NC5 quantification, thus indicating that only one mdhfr-ts copy was integrated. The results obtained with the NcΔROP40 strain, however, showed that the number of dhfr-ts copies per genome was substantially higher, indicating that at least three copies of the selectable mdhfr-ts marker were integrated into the genomic DNA during gene editing by CRISPR-Cas9. This duplex TaqMan-qPCR provides a reliable and easy-to-use tool for assessing CRISPR-Cas9 mediated mutagenesis in WT N. caninum strains.
1 Introduction
Neospora caninum is an obligate intracellular apicomplexan cyst-forming parasite and is closely related to Toxoplasma gondii (Dubey and Schares, 2011; Dubey et al., 2017). It is a significant veterinary pathogen and the etiological agent of bovine neosporosis, which is one of the main causes of cattle abortion worldwide (Lindsay and Dubey, 2020; Hecker et al., 2023), resulting in significant economic losses (Reichel et al., 2013). Currently, there are no vaccines or drugs available to treat the disease, and the knowledge of the molecular mechanisms involved in the virulence of parasite is limited.
In this respect, functional genomics can aid in the reliable characterization of potential parasite virulence factors, as well as in the identification of vaccine candidates and drug targets. Validation of such factors is usually carried out by the generation of knockouts (KOs) that are deficient in their expression. In this context, the development of genetic manipulation methods has been crucial in advancing our understanding of the biology of apicomplexan parasites (Suarez et al., 2017).
In 2018, the CRISPR-Cas9 technology was successfully applied to N. caninum, using the same constructs previously developed for T. gondii (Arranz-Solís et al., 2018). Subsequently, an increased number of mutant parasites deficient in certain genes have been developed by disrupting the target gene sequence by incorporation of a selection marker. The most frequently used selection marker is the modified dihydrofolate reductase-thymidylate synthase (mdhfr-ts) (Nishikawa et al., 2018; Zhao et al., 2020; Dong et al., 2021; Wang et al., 2021; Yang et al., 2021), which provides resistance to pyrimethamine, allowing the selection of mutant parasites that grow in the presence of pyrimethamine.
Generation of KO mutants is followed by verification of target gene editing (Zhang et al., 2015). However, it is also important to consider off-target effects (OTEs), such as multiple insertions of the mdhfr-ts marker and OTEs are often overlooked. While the CRISPR-Cas9 system is highly effective, achieving absolute specificity remains a challenge. Despite efforts to enhance precision, excision may occur at various locations within the genome due to the presence of PAM sequences (Di Cristina and Carruthers, 2018), DNA/RNA bulges (Lin et al., 2014), electroporation conditions (Alkan et al., 2018), as well as through unspecific targeting by the gRNAs themselves (Jinek et al., 2012).
Recently, in order to identify OTEs resulting from multiple insertion of the selection marker in T. gondii KO strains generated by CRISPR-Cas9, a duplex-TaqMan qPCR-based approach has been developed (Hänggeli et al., 2022). N. caninum shares a high degree of homology and synteny with T. gondii (Reid et al., 2012). Thus, the purpose of this work was to develop and validate a duplex TaqMan-qPCR for N. caninum to assess mdhfr-ts copy numbers inserted into the genome of CRISPR-Cas9-generated N. caninum mutants.
2 Materials and methods
2.1 Parasite culture and generation of KO strains
The parasite strains used in this study were the N. caninum Nc-Spain7 isolate (wild-type strain, WT) and the KO strains NcΔGRA7 and NcΔROP40, which are defective in the NcGRA7 and NcROP40 genes, respectively. These KO parasites were previously generated using CRISPR-Cas9 technology, by deleting the target gene with two gRNAs directed to the 5’ and 3’ end of the gene and replacing it with the pLoxP-mCherry-DHFR plasmid (Addgene Plasmid #70147; P-mDHFR) (Arranz-Solís et al., 2018; Rico-San Román et al., 2022). The P-mDHFR plasmid contains the modified Toxoplasma DHFR-TS pyrimethamine-resistant allele marker (mdhfr-ts), which allows the selection of mutant parasites by adding pyrimethamine in cell culture medium (10 µM). Tachyzoites were routinely in vitro maintained by continuous passage in a monolayer culture of the MARC-145 cell line, as previously described (Regidor-Cerrillo et al., 2011; Rico-San Román et al., 2023).
Finally, the mutant parasites were analyzed by PCR to confirm deletion of the targeted gene and its replacement by the mdhfr-ts (Arranz-Solís et al., 2018; Rico-San Román et al., 2022). Additionally, Western blotting (WB) and Immunofluorescence assay (IFA) were used to confirm the lack of protein expression in KO tachyzoites compared to WT parasites, as described (Rico-San Román et al., 2022).
2.2 Design of primers and probe
To determine the copy numbers of the inserted mdhfr-ts selectable marker in the genome of KO clones, we designed a TaqMan-qPCR taking advantage of the fact that WT tachyzoites have a single copy of dhfr-ts in their genome (wtdhfr-ts). The copy number of the dhfr-ts fragment in a given DNA quantity of KO parasites with a single site-specific integration should be twice the number of dhfr-ts copies recorded in the same DNA quantity from WT parasites.
The dhfr-ts DNA sequences coding for T. gondii RH and N. caninum were retrieved from ToxoDB database (TGRH88_062920 and NCLIV_065390). Sequences were aligned, and different primers were designed in exon 7: a forward primer (5’-ATCTGGGACAAGAATGTGAC-3’), a reverse primer (5’-CGAAGTGTCTCCACTGGA-3’) and a TaqMan probe (Cy5-CGAGAGGTCGGAGACATCGGC-BHQ). Nucleotide sequences for primers and probe were designed in 100% conserved between T. gondii dhfr-ts, N. canium dhfr-ts, plasmid P972 (Hänggeli et al., 2022) and P-mDHFR. Plasmid P972 was previously used by Hänggeli et al. for amplification of the selectable marker mdhfr-ts and generation of sag1-defective T. gondii KO mutants (T. gondii RH Δsag1).
2.3 Validation of the designed primers and probe by TaqMan q-PCR
Specific dhfr-ts forward and reverse primers were designed to amplify a 106 bp fragment and were tested by conventional PCR and agarose gel using DNA from WT-T. gondii RH, T. gondii RH Δsag1 C18, WT-N. caninum, plasmid P972 and plasmid P-mDHFR. The PCR was performed in 25 µl final volume containing 12.5 µl of GoTaq® G2 Master Mix (Promega, Madison, WI, USA), 0.5 µl of each primer (forward and reverse, 50 µM), 10.5 µl of distilled water and 1 µl of template DNA. Conditions were as follows: an initial denaturation step at 98°C for 3 min, followed by 25 cycles of denaturation at 95°C for 5 min, annealing at 58°C for 30 sec, and elongation at 72°C for 20 sec. The final cycle was followed by an extension step at 72°C for 2 min.
Subsequently, dhfr-ts primers were validated by qPCR. DNA controls of WT-T. gondii RH and T. gondii RH Δsag1 C18 were used (Hänggeli et al., 2022). The C18 clone was selected as a certified control for single insertion of the complete mdhfr-ts selectable marker. PCR amplification was performed using a Bio-Rad CFX 96 qPCR instrument (Bio-Rad, Hercules, CA, USA) in a total reaction mixture of 10 μL containing 1x SensiFast master mix (Bioline, Meridian Bioscience, Cincinnati, OH, USA), 0.5 μM of reverse and forward primers, 0.1 μM of DHFRQ-P probe, 0.3 mM dUTP, one unit of heat-labile Uracil DNA Glycosylase (UDG) (Longo et al., 1990) and 5 ng DNA template. The following thermal profile was applied: an initial incubation of 10 min at 42°C, followed by a denaturation step of 5 min at 95°C and 50 cycles of two-step amplification (10 sec at 95°C and 20 sec at 62°C). Samples were tested in triplicates and a negative control with double-distilled water was included for each experiment. For quantification, a standard curve based on the use of a 10-fold serial dilution of DNA from WT-T. gondii RH was used, with tachyzoite numbers ranging from 105 to 101 per 3 μL.
2.4 Duplex TaqMan-qPCR targeting dhfr-ts and NC5
Once the TaqMan-qPCR was validated, the number of tachyzoites corresponding to a given DNA quantity and the copy number of the dhfr-ts DNA fragment in the same given quantity were assessed simultaneously. Quantification of tachyzoites was achieved by preparation of a N. caninum standard curve, using 10-fold serial dilutions with parasite concentrations ranging from 8.5 x 104 to 8.5 ng/μL and amplification of a 338 bp region of the N. caninum NC5 gene (Collantes-Fernández et al., 2002). Amplifications were carried-out in total volume of 10 μL containing 1 x SensiFast master mix (Bioline, Meridian Bioscience, Cincinnati, OH, USA), 0.5 μM of each primer set (i: NC5-F 5’-CCCAGTGCGTCCAATCCTGTAAC-3’ and NC5-R 5’-CTCGCCAGTCAACCTACGTCTTCT-3’; ii: dhfr-F/R), 0.1 μM of each probe (DHFRQ-P Cy5 and NCQ-PFAM-TGGTAGCGGTGAGAGGTGGGATACGTG-BHQ), 0.3 mM dUTP, and one unit of UDG. From each sample, 4.25 ng of DNA were used in the reaction mix. All reactions were run in triplicates and amplifications were carried-out under the same thermal profile used for the single TaqMan-qPCR. The cycle threshold values (CT) were plotted as mean of triplicates against the standard curve values to determine the number of tachyzoites. Parasite concentrations were determined after the calculation of the linear regression equation (y = ax + b), where y = CT; a = curve slope (slope); x = parasite number; and b = where the curve intersects y-axis (y intercept). Then, the whole experiment test with duplex TaqMan qPCR was repeated with 8.5 ng of DNA for validating results.
3 Results
3.1 Validation of designed primers for the duplex TaqMan q-PCR
Assessment of the specificity of primers to amplify a 106 bp fragment of wtdhfr-ts in N. caninum and T. gondii RH wild type strains, and mdhfr-ts in plasmids P972 and P-mDHFR, resulted in the amplification of one band with expected size in all samples. Subsequently, the primers were tested to quantify numbers of dhfr-ts copies in the genome of WT-T. gondii RH parasites and the T. gondii RH Δsag1 C18 mutant parasites. As show in Figure 1, the number of tachyzoites estimated in 5 ng of DNA extracted from T. gondii RH Δsag1 C18 was twice that estimated in 5 ng of DNA extracted from T. gondii RH. This indicates that T. gondii RH Δsag1 C18 tachyzoites harbor two copies of the dhfr-ts gene -one is the wtdhfr-ts, and the other is the integrated mdhfr-ts conferring pyrimethamine resistance-, thus revealing a single insertion of the mdhfr-ts selectable marker in their genome. These results confirm previous findings and validate the designed primers.
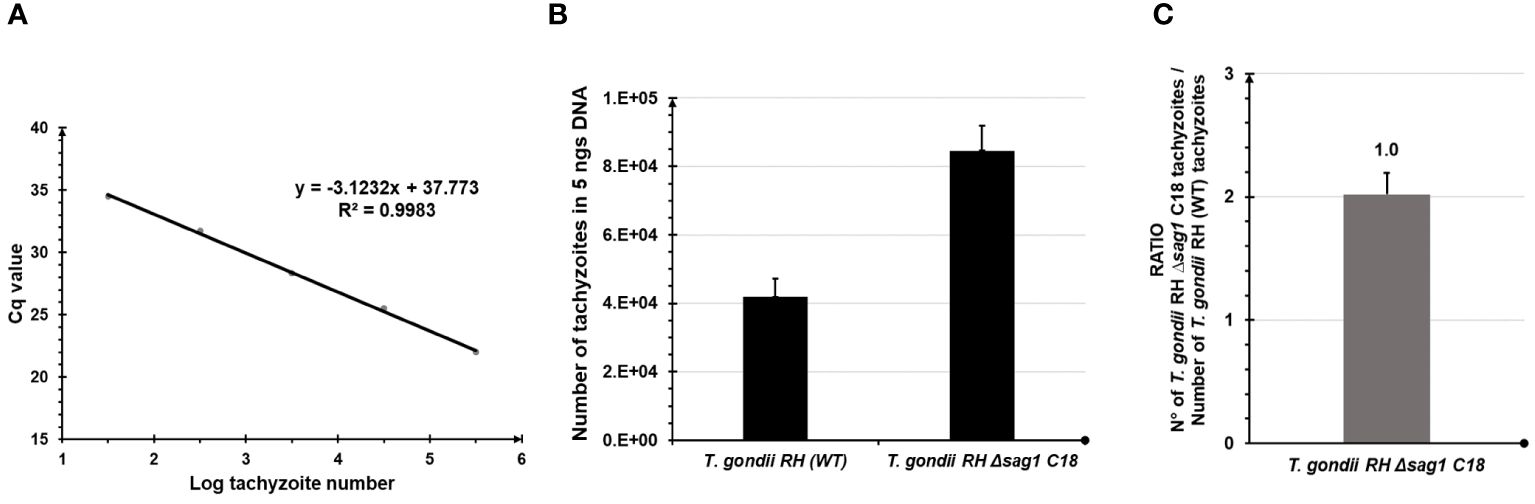
Figure 1 Validation of designed primers for determining the copy number of integrated mdhfr-ts marker using Toxoplasma gondii samples and a single TaqMan-qPCR. (A) WT-T.gondii RH standard curve. (B) Number of tachyzoites in 5 ng DNA determined using the specific dhfr-ts forward and reverse primers designed for Neospora caninum knock-out samples and the WT-T.gondii RH standard curve. Error bars indicate standard deviations of triplicates for each sample. (C) Number of inserted mdhfr-ts in T.gondii RH Δsag1 C18 calculated using the ratio of the number of T.gondii RH Δsag1 C18 tachyzoites and the number of WT-T.gondii RH tachyzoites determined by dhfr-ts amplification. Error bars indicate standard deviations of triplicates for each sample.
Data and detailed calculations are shown in Supplementary Table 1.
3.2 Duplex TaqMan qPCR
The two N. caninum KO strains (NcΔGRA7 and NcΔROP40) were tested in the duplex TaqMan qPCR by applying the same principle described by Hänggeli et al., 2022. For N. caninum WT parasites, the number of tachyzoites determined by the amplification of dhfr is equal to the number of tachyzoites determined by amplification of NC5, resulting in a ratio of 1. In case of a KO with single insertion of the mdhfr-ts, the number of tachyzoites given by the dhfr qPCR is the double of the number of tachyzoites determined by NC5, resulting in a ratio of 2. In case of multiple insertion, the ratio will be > 2.
As shown in Figure 2, the standard curves performed for the two target genes, NC5 and dhfr, had similar slopes and efficiencies (dhfr-slope = -3.34 and R2 = 0.9963 and NC5-slope = -3.47 and R2 = 0.9996).
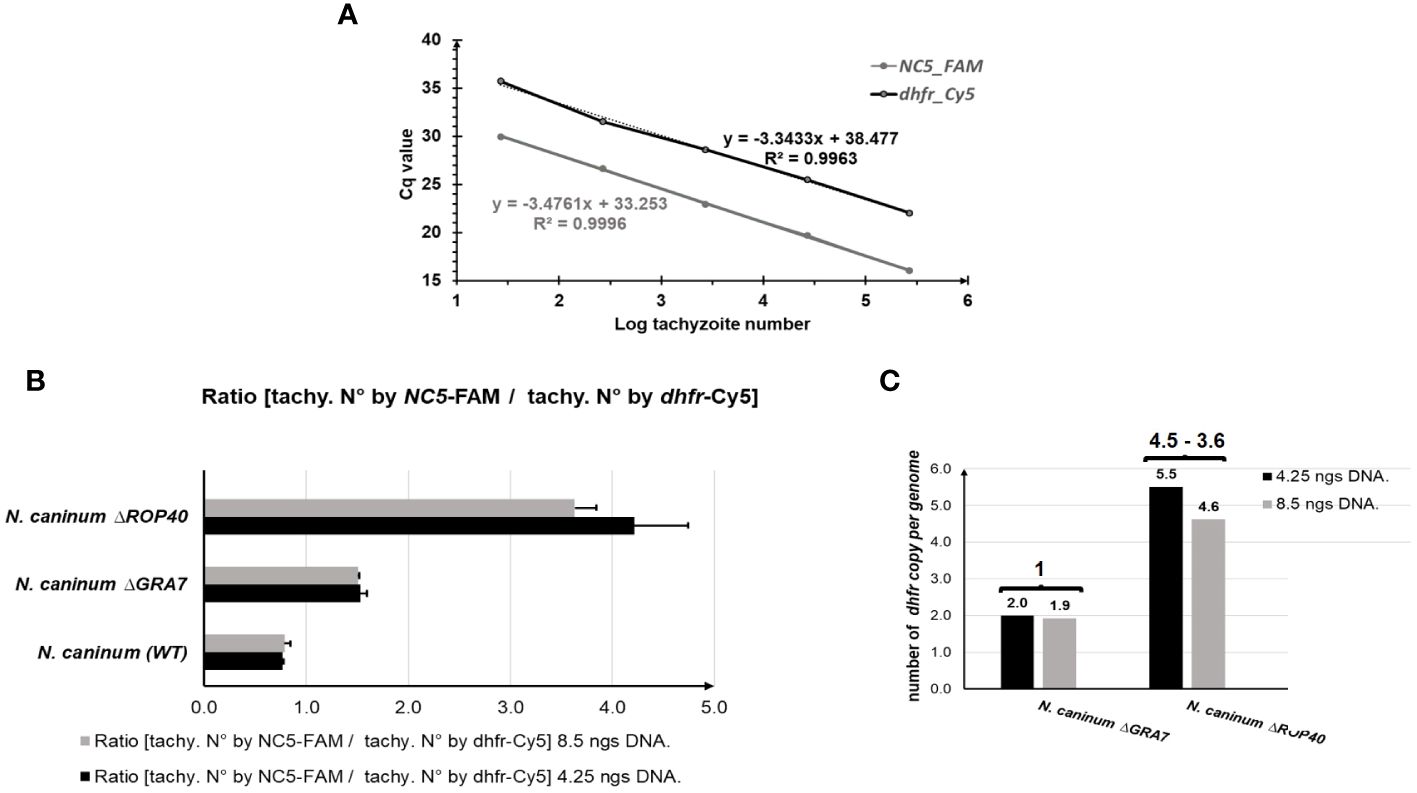
Figure 2 TaqMan-qPCR for determining the copy number of integrated mdhfr-ts selectable marker. (A) Neospora caninum (WT) standard curve amplified with dhfr (black line) and NC5 (grey line) primers. (B) Numer of dhfr copy per genome given by the ratio of the number of tachyzoites determined by using the NC5 primer and the number of tachyzoites determined by dhfr amplification, using 2 different DNA concentrations: 8.5 ng DNA (grey bar) and 4.25 ng DNA (black bar). Error bars indicate standard deviations of triplicates for each sample. (C) Number of inserted mdhfr-ts marker in the N. caninum mutants knock-out (KO). Bars indicate the ratios calculated in (B) and numbers above brackets indicate the calculated number of mdhfr-ts copies inserted in each KO. This number is defined by substracting the dhfr-ts copy number of the WT, 1, from the ratio calculated for each KO. The result of 1 in NcΔGRA7 indicates a single integration event of mdhfr-ts. However, the result of 4.5–3.6 in NcΔROP40 indicates multiple integration events of mdhfr-ts.
Also, the mean number of N. caninum WT tachyzoites determined by NC5 amplification was similar to the mean number of tachyzoites determined by dhfr-ts amplification for the tested DNA quantities (4.25 ng and 8.5 ng), resulting thus in a ratio of 0.8 (Figure 2B). For the KO parasites NcΔGRA7, the number of tachyzoites determined by dhfr-ts quantification was twice the number of tachyzoites determined by NC5 quantification, thus resulting in a ratio of 1.5 for both quantities (Figure 2B). This ratio is the double of WT ratio (0.8), indicating a single insertion of the mdhfr-ts selectable marker (Figure 2C). However, in the NcΔROP40 strain, for both DNA quantities analyzed, the number of dhfr-ts copies per genome was higher than 2 (5.5 and 4.6), indicating that at least three copies of the selectable mdhfr-ts marker were integrated into the genomic DNA during gene editing by CRISPR-Cas9 (Figures 2B, C).
Detailed results and calculation for the duplex TaqMan qPCR are included in Supplementary Table 2.
4 Discussion
It is imperative to examine N. caninum KO mutants for OTEs due to the insertion of multiple dhfr-ts copies, especially when employing a gene replacement strategy with two gRNAs. In the CRISPR-Cas9 system, gRNA(s) sometimes bind other than target loci (Pacesa et al., 2022). Off-target sites represent a significant challenge, particularly when the targeted gene belongs to a gene family such as ROP40, a member of rhoptry family protein (Camejo et al., 2014). Therefore, tools are needed to identify whether off-target insertion of the selection marker occurs in the genome.
Previously, Hänggeli et al. designed a single and a duplex TaqMan-qPCR to determine the copy number of the integrated mdhfr-ts selection marker during site-specific mutagenesis in T. gondii by CRISPR-Cas9 (Hänggeli et al., 2022). However, this qPCR could not be directly used in N. caninum for the following reasons: i) when aligning T. gondii wt dhfr-ts and N. caninum wt dhfr-ts sequences, it became evident that several single nucleotide polymorphisms occurred in the forward/reverse primers and in the probe, which would affect the accuracy of the qPCR; ii) it is not possible to use the T. gondii diagnostic PCR based on the 529 bp repeat element for the quantification of N. caninum in the duplex qPCR. Thus, the Neospora-specific diagnostic qPCR based on NC5 had to be used, and conditions had to be adapted.
Although the single qPCR can be applied on its own, it is recommended to perform multiplexing with the NC5-qPCR. The determination of dhfr-ts copy numbers in the KOs samples using the single dhfr-qPCR is completely dependent on the WT DNA sample. Therefore, it is important to extract DNA from the same number of parasites for all samples. However, there is still a risk that the amount of DNA from host cells may not be consistent in all DNA preparation, even if the tachyzoites were column purified before counting and DNA purification. In the duplex qPCR, a ratio is calculated for each sample independently of the WT, as explained in Hänggeli et al., 2022. For this reason, the single PCR was used to validate the primers and probe using certified T. gondii WT and KO DNA controls for single insertion.
In this work, the duplex qPCR for detecting the mdhfr-ts selection marker was successfully adapted to be used in N. caninum. The results showed that the NcΔGRA7 strain harbors a single mdhfr-ts insertion in the genome, located at the site of the deleted gene of interest, as found in a previous study (Rico-San Román et al., 2022). However, multiple insertions of the mdhfr-ts cassette were detected in the NcΔROP40 strain. Upon generation of the NcΔROP40 strain, the fragment corresponding to the ROP40 gene was found to be correctly disrupted by a single mdhfr-ts insertion (Rico-San Román et al., 2022). However, whole genome sequencing was not performed, thus introduction of other mdhfr-ts copies in the genome was not detected. Nevertheless, complementation of NcΔROP40 resulted in restoration of virulence (Rico-San Román et al., 2022), thus the additional mdhfr-ts copies inserted elsewhere in the genome did not produce phenotypic changes.
Multiple insertions of the selection cassette out of the targeted region are not problematic for the KO phenotype if the multiple integration occurs in non-coding DNA regions, especially if the complemented KO allows recovery of the WT phenotype. However, multiple insertion of the selection cassette, particularly mdhfr-ts, could be a concern if it leads to significantly high levels of the active mDHFR-TS enzyme, which plays a crucial role in pyrimidine synthesis (Rosowsky et al., 2003; Hopper et al., 2019). This issue is still largely unknown, in particular the impact of multiple insertion of mdhfr-ts on parasite growth and gene expression pattern. This aspect may contribute to the observed differences in the behavior of GRA7-deficient parasites generated by different groups using CRISPR-Cas9 and the selection marker mdhfr-ts (Nishikawa et al., 2018; Wang et al., 2021; Abdou et al., 2022; Rico-San Román et al., 2022). It is therefore important to develop tools that allow to determine the number of mdhfr-ts copies inserted in mutant parasites, such as the duplex qPCR presented herein. Its use in the generation and selection protocols of mutants and the identification of those with a single insertion of the selection marker, will help to achieve greater homogeneity and reliability of the results between groups.
Data availability statement
The original contributions presented in the study are included in the article/Supplementary Material. Further inquiries can be directed to the corresponding author/s.
Author contributions
LR: Formal analysis, Investigation, Visualization, Writing – original draft. KH: Methodology, Writing – review & editing. AH: Resources, Writing – review & editing. PH: Funding acquisition, Writing – review & editing. EC-F: Funding acquisition, Writing – review & editing. L-MO-O: Funding acquisition, Resources, Writing – review & editing. GB: Conceptualization, Formal analysis, Methodology, Supervision, Visualization, Writing – original draft.
Funding
The author(s) declare financial support was received for the research, authorship, and/or publication of this article. This work was supported by the Spanish Ministry of Science, Innovation and Universities (PID2019-104713RB-C21 and PID2022-139673OB-I00) and the Swiss National Science Foundation (310030_214897). LR and KH were financially supported by a grant from the Spanish Ministry of Science, Innovation and Universities (BES-2017-079810) and the Uniscentia Foundation, respectively.
Conflict of interest
The authors declare that the research was conducted in the absence of any commercial or financial relationships that could be construed as a potential conflict of interest.
The author(s) declared that they were an editorial board member of Frontiers, at the time of submission. This had no impact on the peer review process and the final decision.
Publisher’s note
All claims expressed in this article are solely those of the authors and do not necessarily represent those of their affiliated organizations, or those of the publisher, the editors and the reviewers. Any product that may be evaluated in this article, or claim that may be made by its manufacturer, is not guaranteed or endorsed by the publisher.
Supplementary material
The Supplementary Material for this article can be found online at: https://www.frontiersin.org/articles/10.3389/fcimb.2024.1419209/full#supplementary-material
References
Abdou, A. M., Ikeda, R., Watanabe, K., Furuoka, H., Nishikawa, Y. (2022). Role of dense granule antigen 7 in vertical transmission of Neospora caninum in C57BL/6 mice infected during early pregnancy. Parasitol. Int. 89, 102576. doi: 10.1016/j.parint.2022.102576
Alkan, F., Wenzel, A., Anthon, C., Havgaard, J. H., Gorodkin, J. (2018). CRISPR-Cas9 off-targeting assessment with nucleic acid duplex energy parameters. Genome Biol. 19, 177. doi: 10.1186/s13059-018-1534-x
Arranz-Solís, D., Regidor-Cerrillo, J., Lourido, S., Ortega-Mora, L. M., Saeij, J. P. J. (2018). Toxoplasma CRISPR/Cas9 constructs are functional for gene disruption in. Neospora caninum. Int. J. Parasitol. 48, 597–600. doi: 10.1016/j.ijpara.2018.03.002
Camejo, A., Gold, D. A., Lu, D., McFetridge, K., Julien, L., Yang, N., et al. (2014). Identification of three novel Toxoplasma gondii rhoptry proteins. Int. J. Parasitol. 44, 147–160. doi: 10.1016/j.ijpara.2013.08.002
Collantes-Fernández, E., Zaballos, Á., Álvarez-García, G., Ortega-Mora, L. M. (2002). Quantitative detection of Neospora caninum in bovine aborted fetuses and experimentally infected mice by real-time PCR. J. Clin. Microbiol. 40, 1194–1198. doi: 10.1128/JCM.40.4.1194-1198.2002
Di Cristina, M., Carruthers, V. B. (2018). New and emerging uses of CRISPR/Cas9 to genetically manipulate apicomplexan parasites. Parasitology 145, 1119–1126. doi: 10.1017/S003118201800001X
Dong, J., Zhang, N., Zhao, P., Li, J., Cao, L., Wang, X., et al. (2021). Disruption of dense granular protein 2 (GRA2) decreases the virulence of Neospora caninum. Front. Vet. Sci. 8. doi: 10.3389/fvets.2021.634612
Dubey, J. P., Hemphill, A., Calero-Bernal, R., Schares, G. (2017). Neosporosis in Animals (Boca Ratón, FL, USA: CRC Press). doi: 10.1201/9781315152561
Dubey, J. P., Schares, G. (2011). Neosporosis in animals-The last five years. Vet. Parasitol. 180, 90–108. doi: 10.1016/j.vetpar.2011.05.031
Hänggeli, K. P. A., Hemphill, A., Müller, N., Schimanski, B., Olias, P., Müller, J., et al. (2022). Single- and duplex TaqMan-quantitative PCR for determining the copy numbers of integrated selection markers during site-specific mutagenesis in Toxoplasma gondii by CRISPR-Cas9. PloS One 17, e0271011. doi: 10.1371/journal.pone.0271011
Hecker, Y. P., González-Ortega, S., Cano, S., Ortega-Mora, L. M., Horcajo, P. (2023). Bovine infectious abortion: a systematic review and meta-analysis. Front. Vet. Sci. 10, 1249410. doi: 10.3389/fvets.2023.1249410
Hopper, A. T., Brockman, A., Wise, A., Gould, J., Barks, J., Radke, J. B., et al. (2019). Discovery of selective Toxoplasma gondii dihydrofolate reductase inhibitors for the treatment of toxoplasmosis. J. Med. Chem. 62, 1562–1576. doi: 10.1021/acs.jmedchem.8b01754
Jinek, M., Chylinski, K., Fonfara, I., Hauer, M., Doudna, J. A., Charpentier, E. (2012). A programmable dual-RNA-guided DNA endonuclease in adaptive bacterial immunity. Science 337, 816–821. doi: 10.1126/science.1225829
Lin, Y., Cradick, T. J., Brown, M. T., Deshmukh, H., Ranjan, P., Sarode, N., et al. (2014). CRISPR/Cas9 systems have off-target activity with insertions or deletions between target DNA and guide RNA sequences. Nucleic Acids Res. 42, 7473–7485. doi: 10.1093/nar/gku402
Lindsay, D. S., Dubey, J. P. (2020). Neosporosis, toxoplasmosis, and sarcocystosis in ruminants: an update. Veterinary Clinics North America - Food Anim. Pract. 36, 205–222. doi: 10.1016/j.cvfa.2019.11.004
Longo, M. C., Berninger, M. S., Hartley, J. L. (1990). Use of uracil DNA glycosylase to control carry-over contamination in polymerase chain reactions. Gene 93, 125–128. doi: 10.1016/0378-1119(90)90145-H
Nishikawa, Y., Shimoda, N., Fereig, R. M., Moritaka, T., Umeda, K., Nishimura, M., et al. (2018). Neospora caninum dense granule protein 7 regulates the pathogenesis of neosporosis by modulating host immune response. Appl. Environ. Microbiol. 84, 1–23. doi: 10.1128/AEM.01350-18
Pacesa, M., Lin, C.-H., Cléry, A., Saha, A., Arantes, P. R., Bargsten, K., et al. (2022). Structural basis for Cas9 off-target activity. Cell 185, 4067–4081.e21. doi: 10.1016/j.cell.2022.09.026
Regidor-Cerrillo, J., Gámez-Bautista, M., Sodupe, I., Aduriz, G., Álvarez-García, G., Del Pozo, I., et al. (2011). In vitro invasion efficiency and intracellular proliferation rate comprise virulence-related phenotypic traits of Neospora caninum. Vet. Res. 42 (1), 41. doi: 10.1186/1297-9716-42-41
Reichel, M. P., Ayanegui-Alcérreca, A. M., Gondim, L. F. P., Ellis, J. T. (2013). What is the global economic impact of Neospora caninum in cattle - The billion dollar question. Int. J. Parasitol. 43, 133–142. doi: 10.1016/j.ijpara.2012.10.022
Reid, A. J., Vermont, S. J., Cotton, J. A., Harris, D., Hill-Cawthorne, G. A., Könen-Waisman, S., et al. (2012). Comparative genomics of the apicomplexan parasites Toxoplasma gondii and Neospora caninum: Coccidia differing in host range and transmission strategy. PloS Pathog. 8 (3), e1002567. doi: 10.1371/journal.ppat.1002567
Rico-San Román, L., Amieva, R., Horcajo, P., García-Sánchez, M., Pastor-Fernández, I., Ortega-Mora, L. M., et al. (2023). Characterization of Neospora caninum virulence factors NcGRA7 and NcROP40 in bovine target cells. Vet. Parasitol. 320, 109973. doi: 10.1016/j.vetpar.2023.109973
Rico-San Román, L., Amieva, R., Regidor-Cerrillo, J., García-Sánchez, M., Collantes-Fernández, E., Pastor-Fernández, I., et al. (2022). NcGRA7 and ncROP40 play a role in the virulence of Neospora caninum in a pregnant mouse model. Pathogens 11, 998. doi: 10.3390/pathogens11090998
Rosowsky, A., Chen, H., Fu, H., Queener, S. F. (2003). Synthesis of new 2,4-Diaminopyrido[2,3-d]pyrimidine and 2,4-Diaminopyrrolo[2,3-d]pyrimidine inhibitors of Pneumocystis carinii, Toxoplasma gondii, and Mycobacterium avium dihydrofolate reductase. Bioorg Med. Chem. 11, 59–67. doi: 10.1016/S0968-0896(02)00325-5
Suarez, C. E., Bishop, R. P., Alzan, H. F., Poole, W. A., Cooke, B. M. (2017). Advances in the application of genetic manipulation methods to apicomplexan parasites. Int. J. Parasitol. 47, 701–710. doi: 10.1016/j.ijpara.2017.08.002
Wang, F., Wang, X., Song, X., Ma, L., Yang, J., Liu, Q., et al. (2021). Function of Neospora caninum dense granule protein 7 in innate immunity in mice. Parasitol. Res. 120, 197–207. doi: 10.1007/s00436-020-06961-4
Yang, C., Wang, C., Liu, J., Liu, Q. (2021). Biotinylation of the Neospora caninum parasitophorous vacuole reveals novel dense granule proteins. Parasit Vectors 14, 1–12. doi: 10.1186/s13071-021-05023-7
Zhang, X.-H., Tee, L. Y., Wang, X.-G., Huang, Q.-S., Yang, S.-H. (2015). Off-target effects in CRISPR/cas9-mediated genome engineering. Mol. Ther. Nucleic Acids 4, e264. doi: 10.1038/mtna.2015.37
Keywords: duplex TaqMan-qPCR, single TaqMan-qPCR, DHFR-TS, mdhfr-ts, CRISPR-Cas9, off target effects, Neospora caninum, Toxoplasma gondii
Citation: Rico-San Román L, Hänggeli KPA, Hemphill A, Horcajo P, Collantes-Fernández E, Ortega-Mora LM and Boubaker G (2024) TaqMan-quantitative PCR assays applied in Neospora caninum knock-outs generated through CRISPR-Cas9 allow to determine the copy numbers of integrated dihydrofolate reductase-thymidylate synthase drug selectable markers. Front. Cell. Infect. Microbiol. 14:1419209. doi: 10.3389/fcimb.2024.1419209
Received: 17 April 2024; Accepted: 07 June 2024;
Published: 21 June 2024.
Edited by:
Debanjan Mukhopadhyay, Presidency University, IndiaReviewed by:
Musa Hassan, University of Edinburgh, United KingdomJulia Romano, Johns Hopkins University, United States
Copyright © 2024 Rico-San Román, Hänggeli, Hemphill, Horcajo, Collantes-Fernández, Ortega-Mora and Boubaker. This is an open-access article distributed under the terms of the Creative Commons Attribution License (CC BY). The use, distribution or reproduction in other forums is permitted, provided the original author(s) and the copyright owner(s) are credited and that the original publication in this journal is cited, in accordance with accepted academic practice. No use, distribution or reproduction is permitted which does not comply with these terms.
*Correspondence: Laura Rico-San Román, laura.rico@universidadeuropea.es; Ghalia Boubaker, ghalia.boubaker@unibe.ch