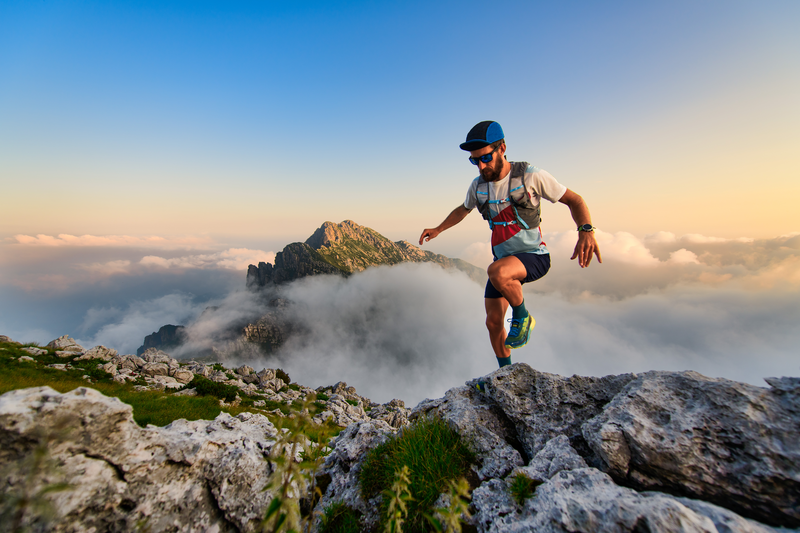
94% of researchers rate our articles as excellent or good
Learn more about the work of our research integrity team to safeguard the quality of each article we publish.
Find out more
REVIEW article
Front. Cell. Infect. Microbiol. , 25 September 2024
Sec. Intestinal Microbiome
Volume 14 - 2024 | https://doi.org/10.3389/fcimb.2024.1416739
This article is part of the Research Topic Interaction of Microbiota and Metabolomic Disorders View all 9 articles
Osteoporosis (OP) is characterized by decreased bone mineral density (BMD) and increased fracture risk, poses a significant global health burden. Recent research has shed light on the bidirectional relationship between gut microbiota (GM) and bone health, presenting a novel avenue for understanding OP pathogenesis and developing targeted therapeutic interventions. This review provides a comprehensive overview of the GM-bone axis, exploring the impact of GM on OP development and management. We elucidate established risk factors and pathogenesis of OP, delve into the diversity and functional changes of GM in OP. Furthermore, we examine experimental evidence and clinical observations linking alterations in GM composition or function with variations in BMD and fracture risk. Mechanistic insights into microbial mediators of bone health, such as microbial metabolites and products, are discussed. Therapeutic implications, including GM-targeted interventions and dietary strategies, are also explored. Finally, we identify future research directions and challenges in translating these findings into clinical practice.
Osteoporosis (OP), a common skeletal disorder characterized by low bone mineral density (BMD) and microarchitectural deterioration of bone tissue, poses a significant public health concern globally (Pouresmaeili et al., 2018). It is associated with an increased risk of osteoporotic fractures (OPF, or fragility fractures, low-trauma fractures), resulting in substantial morbidity, mortality, and healthcare costs. The pathogenesis of OP involves an imbalance between bone resorption and formation processes, leading to compromised bone strength and increased susceptibility to fractures, particularly in the spine, hip, and wrist (Sozen et al., 2017). Factors contributing to OP include aging, hormonal changes (especially estrogen deficiency in postmenopausal women), genetic predisposition, nutritional deficiencies (e.g., calcium, vitamin D), sedentary lifestyle, and certain medications (e.g., glucocorticoids/GCS) (Bhattarai et al., 2020; Cheng et al., 2022a; Erdelyi et al., 2023; Smit et al., 2024). Other risk factors such as excessive alcohol consumption and tobacco use can also exacerbate the development of OP (Yang et al., 2021; Khiyali et al., 2024).
The gut microbiota (GM) refers to the complex community of microorganisms, including bacteria, viruses, fungi, and protozoa, that reside in the gastrointestinal tract. These microorganisms play crucial roles in multiple functions, including digestion, metabolism, and immune regulation, and are integral to host physiology and health (Thursby and Juge, 2017; Hou et al., 2022). The composition of GM is influenced by various factors, including diet, host genetics, age, medications, and environmental exposures. Dysbiosis is a term used to describe an imbalance in the GM. This imbalance can result from factors such as antibiotic use, poor diet, or illness and is associated with a range of health issues, including gastrointestinal disorders, metabolic conditions, and immune dysregulation. Alterations in GM composition and function, has been implicated in the pathogenesis of various diseases, including metabolic disorders like obesity and type 2 diabetes (Moreno-Indias et al., 2014), inflammatory conditions such as rheumatoid arthritis and inflammatory bowel disease (Li and Wang, 2021; Shan et al., 2022), gastrointestinal diseases like irritable bowel syndrome (Shaikh et al., 2023), and even neurological disorders like Parkinson’s disease and depression (Dinan and Cryan, 2017).
Recent research has highlighted the bidirectional interaction between GM and bone health, emphasizing the importance of deciphering the GM-bone axis in OP pathogenesis and management (Lyu et al., 2023). The GM influences bone metabolism through mechanisms such as nutrient absorption, immune modulation, and production of microbial metabolites. Understanding these interactions may reveal novel therapeutic targets for OP prevention and treatment. Moreover, dietary interventions and probiotics targeting GM composition hold promise for optimizing bone health and reducing the risk of OP or OPF. Exploring the GM-bone axis represents a paradigm shift in OP research, with implications for personalized approaches to disease management and improved clinical outcomes.
Bone remodeling is a continuous physiological process responsible for maintaining skeletal integrity and adapting to mechanical stresses. This dynamic equilibrium between bone resorption and formation is tightly regulated by various cellular and molecular mechanisms, involving osteoclasts, osteoblasts, osteocytes, and bone matrix proteins (Bolamperti et al., 2022; Rowe et al., 2024). Osteoclasts, multinucleated cells originating from hematopoietic progenitors in the bone marrow, are specialized for bone resorption. They adhere to the bone surface and create an acidic microenvironment that facilitates the dissolution of the mineralized bone matrix through the secretion of hydrochloric acid and proteolytic enzymes such as cathepsin K, which results in the release of calcium and phosphate ions into the bloodstream (Bar-Shavit, 2007). Osteoblasts, on the other hand, are derived from mesenchymal stem cells (MSCs) and are responsible for the synthesis and deposition of new bone matrix, which subsequently undergoes mineralization to form mature bone tissue. This matrix is primarily composed of type I collagen and other non-collagenous proteins that provide the framework for mineral deposition (Florencio-Silva et al., 2015). Once osteoblasts become entrapped in the bone matrix, they differentiate into osteocytes, which play a crucial role in mechanotransduction and the regulation of both osteoclast and osteoblast activity through signaling pathways.
The equilibrium between bone resorption and formation is tightly regulated by a complex interplay of systemic and local factors. Systemic factors include hormones such as estrogen, which inhibits bone resorption by inducing osteoclast apoptosis and reducing the production of pro-resorptive cytokines; parathyroid hormone (PTH), which in low intermittent doses stimulates bone formation but in chronic elevation can increase bone resorption; and calcitonin, which directly inhibits osteoclast activity. Local factors involve cytokines like interleukin-6 (IL-6) and tumor necrosis factor-α (TNF-α), which promote osteoclast differentiation and activity, and growth factors such as insulin-like growth factor-1 (IGF-1) and transforming growth factor-β (TGF-β), which enhance osteoblast proliferation and function (Feng and McDonald, 2011; Singh et al., 2012). Disruption of this balance, characterized by increased bone resorption or decreased bone formation, results in bone loss and increased fracture risk, as observed in OP.
Mechanical loading also plays a pivotal role in bone remodeling by stimulating osteocytes to produce signaling molecules that regulate osteoblast and osteoclast activity (Robling and Turner, 2009; Du et al., 2020). Adequate mechanical loading is essential to maintain bone density and structure; conversely, reduced mechanical loading, as seen in sedentary lifestyles or prolonged bed rest, leads to bone loss.
Understanding the cellular and molecular mechanisms underlying bone remodeling provides insights into the pathogenesis of OP and highlights potential therapeutic targets for preventing and treating this condition. Efforts to modulate the activity of osteoclasts and osteoblasts, either through pharmacological agents or lifestyle interventions, are critical in maintaining bone health and reducing fracture risk in individuals with OP.
As summarize in Figure 1, established risk factors for OP include aging, hormonal changes (especially estrogen deficiency in postmenopausal women), genetic predisposition, nutritional deficiencies (such as calcium and vitamin D), sedentary lifestyle, certain medications, excessive alcohol consumption, and tobacco use. These factors collectively contribute to the deterioration of BMD and increase the susceptibility to fractures and other skeletal complications.
Aging is a major risk factor for OP, as bone mass typically peaks in early adulthood and gradually declines with age. Older adults are at increased risk of bone loss due to decreased osteoblast activity, hormonal changes, and cumulative exposure to other risk factors over time (Pignolo et al., 2021).
Women are at higher risk of OP compared to men, primarily due to the decline in estrogen levels associated with menopause. Estrogen plays a crucial role in maintaining BMD by suppressing osteoclast activity and promoting osteoblast function. Consequently, postmenopausal women experience accelerated bone loss and are more susceptible to OPF (Almeida et al., 2017; Charde et al., 2023).
Hormonal imbalances, including estrogen deficiency in women and androgen deficiency in men, contribute to OP development. Other endocrine disorders, such as hyperparathyroidism, hyperthyroidism, and adrenal insufficiency, can disrupt bone metabolism and increase fracture risk (Cheng et al., 2022a).
Genetic factors play a significant role in determining BMD and fracture risk. Family history of OP or OPF, as well as genetic polymorphisms associated with bone turnover, mineralization, and skeletal structure, contribute to individual susceptibility to OP (Ralston, 2002; Makitie et al., 2019).
Inadequate intake of calcium, vitamin D, and other essential nutrients essential for bone health increases the risk of OP. Calcium is required for bone mineralization, while vitamin D facilitates calcium absorption and bone metabolism regulation (Khazai et al., 2008).
Sedentary lifestyle, smoking, excessive alcohol consumption, and low body mass index (BMI) are associated with increased OP risk. Physical inactivity and tobacco use adversely affect bone metabolism, while excessive alcohol intake interferes with calcium absorption and hormone levels (Padilla Colon et al., 2018; Niemela et al., 2022).
Certain medications, such as GCS, anticonvulsants, proton pump inhibitors (PPIs), and aromatase inhibitors (AIs), are associated with bone loss and increased fracture risk (Panday et al., 2014; Wang et al., 2023b). These medications may affect bone remodeling dynamics, calcium absorption, or hormone levels, leading to OP development.
The GM is a complex ecosystem consisting of bacteria, archaea, fungi, viruses, and other microorganisms. Bacteria are the most abundant and extensively studied components of the GM, with thousands of different species identified to date. The composition of the GM varies significantly among individuals, influenced by factors such as age, diet, genetics, medications, and environmental exposures (Matijasic et al., 2020; Salazar et al., 2023).
Recent advancements in high-throughput sequencing technologies have enabled comprehensive characterization of GM composition at the taxonomic and functional levels (Wei et al., 2021a; Kwa et al., 2023). Key bacterial phyla inhabiting the human gut include Firmicutes, Bacteroidetes, Actinobacteria, Proteobacteria, Fusobacteria, and Verrucomicrobia (Rinninella et al., 2019). Within these phyla, numerous genera and species exhibit considerable diversity, contributing to the overall complexity of GM.
GM contribute to the digestion and fermentation of dietary components, including complex carbohydrates, fiber, and resistant starches, producing metabolites such as short-chain fatty acids (SCFAs), amino acids, vitamins, and other bioactive compounds (Portincasa et al., 2022). SCFAs, particularly acetate, propionate, and butyrate, serve as energy sources for colonocytes, modulate immune responses, and influence host metabolism (Nogal et al., 2021).
GM play a vital role in shaping host immune responses, maintaining immune homeostasis, and protecting against pathogens. Commensal bacteria interact with intestinal epithelial cells and immune cells, such as dendritic cells (DCs), macrophages, and T lymphocytes, influencing the development and function of the mucosal immune system (Zheng et al., 2020; Mazziotta et al., 2023). Dysbiosis, characterized by alterations in GM composition, has been associated with immune dysregulation and increased susceptibility to inflammatory and autoimmune diseases.
GM contribute to the maintenance of gut barrier integrity by promoting epithelial cell proliferation, enhancing mucin production, and modulating tight junction protein expression (Gierynska et al., 2022). By reinforcing the gut barrier, GM help prevent the translocation of harmful pathogens and microbial antigens from the intestinal lumen into systemic circulation, thereby reducing the risk of systemic inflammation and infection.
GM influence host metabolism through various mechanisms, including energy harvest, regulation of lipid metabolism, bile acid metabolism, and modulation of glucose homeostasis (Ghazalpour et al., 2016; Martin et al., 2019). Alterations in GM composition and function have been implicated in the pathogenesis of metabolic disorders, such as obesity, insulin resistance, and type 2 diabetes mellitus (T2DM) (Crudele et al., 2023).
GM has emerged as a key regulator of bone metabolism, exerting both direct and indirect effects on bone health. Understanding the bidirectional interaction between GM and bone is essential for elucidating the mechanisms underlying OP pathogenesis and developing novel therapeutic strategies (Wang et al., 2022f; Lyu et al., 2023).
Evidences from preclinical and clinical studies indicate that GM composition influences bone metabolism and contributes to variations in BMD and bone strength. Experimental studies in germ-free (GF) animal models, devoid of GM, have demonstrated altered bone phenotypes characterized by decreased BMD, impaired bone microarchitecture, and compromised bone strength compared to conventionally raised animals. Reconstitution of GF animals with specific microbes or microbial metabolites has been shown to partially restore BMD and integrity, highlighting the role of GM in regulating bone metabolism. Furthermore, alterations in GM composition, induced through dietary interventions, probiotics, antibiotics, or fecal microbiota transplantation (FMT), have been associated with changes in bone turnover markers (BTMs), calcium absorption, and skeletal phenotypes in animal models. Clinical and animal studies have also reported associations between GM dysbiosis and OP prevalence, low BMD, and fracture risk in humans (refer to Tables 1, 2 below). Collectively, these findings support the notion that GM play a significant role in modulating bone metabolism and influencing skeletal health.
Table 2. Summary of clinical studies examining GM alterations or interventions among patients with low BMD.
The mechanisms of the GM-bone axis involve multiple pathways. Firstly, GM contribute to nutrient metabolism and absorption, particularly calcium and vitamin D, essential for bone mineralization and remodeling (Wang et al., 2022a). Secondly, GM interact with the host immune system, influencing local and systemic immune responses, with dysbiosis potentially leading to chronic inflammation and bone loss (D’Amelio and Sassi, 2018). Thirdly, GM produce various metabolites such as SCFAs and bile acids, which act as signaling molecules affecting host metabolism and immune function, thereby influencing bone remodeling processes (Yoon et al., 2023). Additionally, GM influence hormonal regulation pathways involved in bone metabolism, including estrogen, vitamin D, and PTH signaling, with dysbiosis potentially disrupting hormone levels and receptor activation, leading to imbalances in bone homeostasis and increase fracture risk (Iwobi and Sparks, 2023). Lastly, GM produce microbial-derived factors like lipopolysaccharides (LPS) and extracellular vesicles, which interact with host cells and modulate inflammatory and signaling pathways relevant to bone remodeling (Chen et al., 2023b).
Animal studies have provided valuable insights into the relationship between GM and bone health. GF animals, devoid of gut microbiota, exhibit altered bone phenotypes characterized by reduced BMD and compromised bone microarchitecture compared to conventionally raised animals. Experimental manipulation of GM composition through dietary interventions, probiotics, antibiotics, or FMT has further elucidated the impact of GM on bone metabolism. Reconstitution of GF animals with specific microbial strains or microbial metabolites has been shown to attenuate bone loss and improve skeletal phenotypes, highlighting the therapeutic potential of modulating GM for bone health. Mechanistic studies in animal models have revealed potential pathways through which GM influence bone metabolism, including nutrient absorption, immune modulation, and production of microbial metabolites. SCFAs, bile acids, and secondary bile acids derived from gut microbial fermentation have been implicated as key mediators of the GM-bone axis, affecting osteoclast and osteoblast activity, bone remodeling dynamics, and skeletal homeostasis. Table 1 provides a summary of recent animal studies investigating or linking the effects of GM on bone metabolism.
Clinical studies in human populations have provided further evidence supporting the association between GM dysbiosis and OP prevalence, BMD changes, and fracture risk. Analysis of GM composition in osteoporotic individuals has revealed alterations in GM diversity and abundance compared to healthy controls. Epidemiological studies have identified associations between dietary patterns, GM composition, and bone health outcomes. High-fiber diets rich in fruits, vegetables, and whole grains, which promote a diverse GM profile, have been associated with higher BMD and reduced fracture risk. Conversely, diets high in saturated fats, refined sugars, and processed foods, which disrupt GM composition, may contribute to bone loss and OP development. Table 2 summarizes clinical studies examining GM alterations or interventions among patients with low BMD.
Overall, both experimental evidence from animal studies and clinical observations in human populations support the notion of a bidirectional interaction between GM and bone health. Further research is needed to elucidate the underlying mechanisms and determine the clinical utility of GM-targeted interventions for OP prevention and management.
GM-targeted interventions aim to modulate GM composition and activity to promote bone health and mitigate OP risk. Several approaches have been proposed and investigated in preclinical and clinical studies.
Probiotics are live microorganisms that confer health benefits when administered in adequate amounts (Nagpal et al., 2012). Certain probiotic strains, such as Lactobacillus and Bifidobacterium species, have been shown to positively influence bone metabolism and improve skeletal phenotypes in animal models (Schepper et al., 2017). Clinical trials investigating the efficacy of probiotic supplementation in improving BMD and reducing fracture risk in humans are ongoing.
Prebiotics are non-digestible dietary fibers that selectively promote the growth and activity of beneficial gut bacteria (Davani-Davari et al., 2019). By fermenting prebiotic fibers, GM produce SCFAs and other metabolites with potential bone-protective effects. Dietary supplementation with prebiotics, such as inulin, oligofructose, and resistant starch, may enhance GM diversity and function, thereby improving bone health.
Synbiotics are combinations of probiotics and prebiotics designed to synergistically promote GM balance and function (Roy and Dhaneshwar, 2023). By providing both beneficial microbes and substrates for their growth, synbiotics aim to optimize GM composition and activity. Clinical trials investigating the effects of synbiotic supplementation on bone health outcomes are underway.
FMT is a medical procedure in which fecal matter from a healthy donor is transplanted into the gastrointestinal tract of a recipient. This procedure aims to restore a balanced GM in individuals suffering from conditions linked to dysbiosis, such as Clostridioides difficile infection and certain inflammatory bowel diseases (IBDs). While primarily used to treat gastrointestinal disorders, FMT may also impact systemic health outcomes, including bone metabolism (Biazzo and Deidda, 2022; Zhang et al., 2022c; Zheng et al., 2022).
Overall, targeting GM composition and activity through these interventions holds promise for optimizing bone metabolism, preserving BMD, and reducing fracture risk in individuals at risk of OP. However, further research is needed to elucidate the optimal dosing, duration, and efficacy of GM-targeted interventions for OP prevention and management.
Adequate intake of calcium and vitamin D is essential for bone mineralization and remodeling. Calcium-rich foods, such as dairy products, leafy greens, and fortified foods, should be consumed as part of a balanced diet. Vitamin D sources include fatty fish, fortified foods, and sunlight exposure. Supplementation may be necessary for individuals with inadequate dietary intake or limited sun exposure.
Protein is essential for bone formation and maintenance, as it provides amino acids necessary for collagen synthesis and bone matrix deposition (Devignes et al., 2022; Selvaraj et al., 2024). Consuming adequate protein from sources such as lean meats, poultry, fish, eggs, legumes, and dairy products supports bone health. However, excessive protein intake, particularly from animal sources, may have adverse effects on bone health and should be moderated.
Consuming a nutrient-rich diet rich in fruits, vegetables, whole grains, and lean proteins provides essential vitamins, minerals, and antioxidants necessary for bone health. Phytochemicals found in plant-based foods may have beneficial effects on bone metabolism and reduce inflammation associated with OP.
High sodium intake and excessive caffeine consumption have been associated with calcium excretion and bone loss (Heaney, 2002; Park et al., 2014; Reuter et al., 2021). Limiting sodium intake and moderating caffeine consumption from sources such as coffee, tea, and soda may help preserve BMD and reduce OP risk.
Excessive alcohol consumption has been linked to decreased BMD and increased fracture risk. Moderating alcohol intake and avoiding binge drinking are recommended to support bone health.
Engaging in weight-bearing and resistance exercises, such as walking, jogging, strength training, and yoga, helps stimulate bone formation and improve BMD. Regular physical activity is essential for maintaining bone strength and reducing OP risk.
By adopting a balanced diet rich in essential nutrients, limiting harmful dietary factors, and engaging in weight-bearing exercise, individuals can support bone health and mitigate the risk of OP. Dietary approaches and nutritional interventions complement GM-targeted interventions in promoting overall skeletal health and reducing fracture risk in susceptible populations.
As research on the GM-bone axis continues to evolve, several areas for future investigation and challenges in translating research findings into clinical practice warrant attention. Here, we discuss potential avenues for future research and the obstacles faced in applying research findings to clinical management.
In future research, elucidating mechanistic insights into the interaction between the GM and bone health is crucial. Investigating specific microbial species, metabolites, and signaling pathways can enhance understanding of the GM-bone axis, paving the way for novel therapeutic targets for OP. Additionally, longitudinal studies tracking changes in GM over time and their associations with bone outcomes are essential for establishing causal relationships and understanding temporal dynamics. Well-designed clinical trials are needed to evaluate the efficacy, safety, and long-term effects of GM-targeted interventions, such as probiotics and prebiotics, in improving bone health outcomes. Personalized medicine approaches, incorporating individual variability in GM composition and lifestyle factors, hold promise for tailoring OP prevention and treatment strategies to specific patient populations. Exploring the gut-brain-bone axis represents a promising area of research for uncovering novel pathways regulating bone metabolism and remodeling.
Translating research findings into clinical practice faces several challenges. Standardization of GM analysis methods and data reporting guidelines is necessary to ensure reproducibility and reliability of research in clinical settings. The heterogeneity of OP phenotypes, influenced by genetics, lifestyle factors, and comorbidities, complicates the identification of consistent biomarkers and therapeutic targets. Regulatory approval of GM-targeted therapies requires robust clinical evidence and careful consideration of safety concerns. One of the significant challenges in implementing probiotic and prebiotic therapies is ensuring patient adherence. Factors such as taste preferences, dietary habits, and the need for consistent long-term use can affect compliance. Improving adherence requires patient education on the benefits of these interventions, developing more palatable and convenient formulations, and integrating these therapies into daily routines in a manner that is easy to follow. Additionally, while short-term studies have demonstrated the benefits of probiotics, prebiotics, and FMT, long-term safety remains a concern. Potential risks include alterations in GM composition that could lead to negative health outcomes, interactions with existing medications, and the possibility of infections, especially with FMT. Therefore, more extensive long-term studies are needed to fully understand the safety profiles of these interventions. Furthermore, the regulatory environment for GM-based therapies is still developing. Probiotics and prebiotics are often classified as dietary supplements, which are subject to less stringent regulatory scrutiny compared to pharmaceuticals. FMT, due to its complexity and potential risks, involves more rigorous regulations. Navigating these regulatory requirements and obtaining necessary approvals can be significant hurdles. Collaboration between researchers, healthcare providers, and regulatory bodies is essential to establish clear guidelines and protocols for the safe and effective use of these therapies. Addressing these challenges and advancing research in the field of GM and OP will pave the way for personalized, evidence-based approaches to bone health management and fracture prevention in diverse patient populations. Collaborative efforts among researchers, clinicians, industry partners, and regulatory agencies are essential for realizing the potential of GM-targeted interventions in improving skeletal health and reducing the burden of OP on a global scale.
The burgeoning field of research on the GM-bone axis has shed light on the intricate interplay between gut microbial communities and skeletal health. Through a comprehensive review of experimental evidence and clinical observations, this review has elucidated the multifaceted impact of GM on OP pathogenesis and management. In conclusion, unraveling the impact of GM on OP pathogenesis offers promising opportunities for personalized, evidence-based strategies to optimize bone health and reduce fracture risk. Collaborative endeavors across disciplines are essential for advancing our understanding of the GM-bone axis and translating scientific discoveries into tangible clinical benefits for individuals at risk of OP.
Moving forward, future research efforts should focus on elucidating mechanistic insights into the GM-bone axis, conducting longitudinal studies to establish causal relationships, and evaluating the efficacy of GM-targeted interventions in clinical trials. Challenges such as standardizing GM analysis, addressing heterogeneity in OP phenotypes, and translating research findings into clinical practice must be overcome to realize the potential of GM-based approaches in OP management.
LH: Investigation, Writing – original draft. YY: Conceptualization, Funding acquisition, Project administration, Writing – review & editing. GH: Writing – review & editing. HL: Funding acquisition, Project administration, Supervision, Writing – review & editing.
The author(s) declare financial support was received for the research, authorship, and/or publication of this article. This study was supported by the Natural Science Foundation of Shaanxi Province (No. 2018JM7049); the Project of Xi’an Science and Technology (No. 22YXYJ0041).
The authors declare that the research was conducted in the absence of any commercial or financial relationships that could be construed as a potential conflict of interest.
All claims expressed in this article are solely those of the authors and do not necessarily represent those of their affiliated organizations, or those of the publisher, the editors and the reviewers. Any product that may be evaluated in this article, or claim that may be made by its manufacturer, is not guaranteed or endorsed by the publisher.
Ai, T., Shang, L., Li, B., Li, J., Qin, R. (2024). Konjac oligosaccharides alleviated ovariectomy-induced bone loss through gut microbiota modulation and treg/th17 regulation. J. Agric. Food Chem. 72, 7969–7979. doi: 10.1021/acs.jafc.4c00281
Almeida, M., Laurent, M. R., Dubois, V., Claessens, F., O’Brien, C. A., Bouillon, R., et al. (2017). Estrogens and androgens in skeletal physiology and pathophysiology. Physiol. Rev. 97, 135–187. doi: 10.1152/physrev.00033.2015
Bar-Shavit, Z. (2007). The osteoclast: a multinucleated, hematopoietic-origin, bone-resorbing osteoimmune cell. J. Cell Biochem. 102, 1130–1139. doi: 10.1002/jcb.21553
Bhattarai, H. K., Shrestha, S., Rokka, K., Shakya, R. (2020). Vitamin D, calcium, parathyroid hormone, and sex steroids in bone health and effects of aging. J. Osteoporos. 2020, 9324505. doi: 10.1155/2020/9324505
Biazzo, M., Deidda, G. (2022). Fecal microbiota transplantation as new therapeutic avenue for human diseases. J. Clin. Med. 11, 4119. doi: 10.3390/jcm11144119
Bolamperti, S., Villa, I., Rubinacci, A. (2022). Bone remodeling: an operational process ensuring survival and bone mechanical competence. Bone Res. 10, 48. doi: 10.1038/s41413-022-00219-8
Britton, R. A., Irwin, R., Quach, D., Schaefer, L., Zhang, J., Lee, T., et al. (2014). Probiotic L. reuteri treatment prevents bone loss in a menopausal ovariectomized mouse model. J. Cell Physiol. 229, 1822–1830. doi: 10.1002/jcp.24636
Cai, X., Li, Z., Yao, Y., Zheng, Y., Zhang, M., Ye, Y. (2024). Glycolithocholic acid increases the frequency of circulating Tregs through constitutive androstane receptor to alleviate postmenopausal osteoporosis. Biochem. Pharmacol. 219, 115951. doi: 10.1016/j.bcp.2023.115951
Charde, S. H., Joshi, A., Raut, J. (2023). A comprehensive review on postmenopausal osteoporosis in women. Cureus 15, e48582. doi: 10.7759/cureus.48582
Chargo, N. J., Kang, H. J., Das, S., Jin, Y., Rockwell, C., Cho, J. Y., et al. (2024). Korean red ginseng extract prevents bone loss in an oral model of glucocorticoid induced osteoporosis in mice. Front. Pharmacol. 15. doi: 10.3389/fphar.2024.1268134
Chen, C., Dong, B., Wang, Y., Zhang, Q., Wang, B., Feng, S., et al. (2020a). The role of Bacillus acidophilus in osteoporosis and its roles in proliferation and differentiation. J. Clin. Lab. Anal. 34, e23471. doi: 10.1002/jcla.23471
Chen, C., Lei, H., Zhao, Y., Hou, Y., Zheng, H., Zhang, C., et al. (2023a). A novel small molecule effectively ameliorates estrogen deficiency-induced osteoporosis by targeting the gut-bone signaling axis. Eur. J. Pharmacol. 954, 175868. doi: 10.1016/j.ejphar.2023.175868
Chen, S., Lei, Q., Zou, X., Ma, D. (2023b). The role and mechanisms of gram-negative bacterial outer membrane vesicles in inflammatory diseases. Front. Immunol. 14. doi: 10.3389/fimmu.2023.1157813
Chen, Z., Lv, M., Liang, J., Yang, K., Li, F., Zhou, Z., et al. (2023d). Neuropeptide Y-mediated gut microbiota alterations aggravate postmenopausal osteoporosis. Adv. Sci. 10, e2303015. doi: 10.1002/advs.202303015
Chen, Y., Yang, C., Dai, Q., Tan, J., Dou, C., Luo, F. (2023c). Gold-nanosphere mitigates osteoporosis through regulating TMAO metabolism in a gut microbiota-dependent manner. J. Nanobiotechnol. 21, 125. doi: 10.1186/s12951-023-01872-9
Chen, X., Zhang, Z., Hu, Y., Cui, J., Zhi, X., Li, X., et al. (2020b). Lactulose suppresses osteoclastogenesis and ameliorates estrogen deficiency-induced bone loss in mice. Aging Dis. 11, 629–641. doi: 10.14336/AD.2019.0613
Cheng, C. H., Chen, L. R., Chen, K. H. (2022a). Osteoporosis due to hormone imbalance: an overview of the effects of estrogen deficiency and glucocorticoid overuse on bone turnover. Int. J. Mol. Sci. 23, 1376. doi: 10.3390/ijms23031376
Cheng, M., Tan, B., Wu, X., Liao, F., Wang, F., Huang, Z. (2021). Gut microbiota is involved in alcohol-induced osteoporosis in young and old rats through immune regulation. Front. Cell Infect. Microbiol. 11. doi: 10.3389/fcimb.2021.636231
Cheng, J., Zhong, W. L., Zhao, J. W., Zhai, J. H., Chen, C., Chao, A. J., et al. (2022b). Alterations in the composition of the gut microbiota affect absorption of cholecalciferol in severe osteoporosis. J. Bone Min. Metab. 40, 478–486. doi: 10.1007/s00774-021-01303-5
Chevalier, C., Kieser, S., Colakoglu, M., Hadadi, N., Brun, J., Rigo, D., et al. (2020). Warmth prevents bone loss through the gut microbiota. Cell Metab. 32, 575–590 e7. doi: 10.1016/j.cmet.2020.08.012
Crudele, L., Gadaleta, R. M., Cariello, M., Moschetta, A. (2023). Gut microbiota in the pathogenesis and therapeutic approaches of diabetes. EBioMedicine 97, 104821. doi: 10.1016/j.ebiom.2023.104821
D’Amelio, P., Sassi, F. (2018). Gut microbiota, immune system, and bone. Calcif. Tissue Int. 102, 415–425. doi: 10.1007/s00223-017-0331-y
Davani-Davari, D., Negahdaripour, M., Karimzadeh, I., Seifan, M., Mohkam, M., Masoumi, S. J., et al. (2019). Prebiotics: definition, types, sources, mechanisms, and clinical applications. Foods 8, 92. doi: 10.3390/foods8030092
Devignes, C. S., Carmeliet, G., Stegen, S. (2022). Amino acid metabolism in skeletal cells. Bone Rep. 17, 101620. doi: 10.1016/j.bonr.2022.101620
Di, D. S., Li, C., Dai, Y., Wei, M. H., Wang, S. S., Song, W. J., et al. (2021). Integrative analysis of LGR5/6 gene variants, gut microbiota composition and osteoporosis risk in elderly population. Front. Microbiol. 12. doi: 10.3389/fmicb.2021.765008
Dinan, T. G., Cryan, J. F. (2017). Gut feelings on parkinson’s and depression. Cerebrum 2017. Available at: https://www.ncbi.nlm.nih.gov/pubmed/28698775.
Ding, P., Tan, Q., Wei, Z., Chen, Q., Wang, C., Qi, L., et al. (2022). Correction: Toll-like receptor 9 deficiency induces osteoclastic bone loss via gut microbiota-associated systemic chronic inflammation. Bone Res. 10, 47. doi: 10.1038/s41413-022-00221-0
Dou, J., Liang, Z., Liu, J., Liu, N., Hu, X., Tao, S., et al. (2024). Quinoa alleviates osteoporosis in ovariectomized rats by regulating gut microbiota imbalance. J. Sci. Food Agric. 104, 5052–5063. doi: 10.1002/jsfa.13339
Du, J., Yang, J., He, Z., Cui, J., Yang, Y., Xu, M., et al. (2020). Osteoblast and osteoclast activity affect bone remodeling upon regulation by mechanical loading-induced leukemia inhibitory factor expression in osteocytes. Front. Mol. Biosci. 7. doi: 10.3389/fmolb.2020.585056
Erdelyi, A., Palfi, E., Tuu, L., Nas, K., Szucs, Z., Torok, M., et al. (2023). The importance of nutrition in menopause and perimenopause-A review. Nutrients 16, 27. doi: 10.3390/nu16010027
Feng, X., McDonald, J. M. (2011). Disorders of bone remodeling. Annu. Rev. Pathol. 6, 121–145. doi: 10.1146/annurev-pathol-011110-130203
Florencio-Silva, R., Sasso, G. R., Sasso-Cerri, E., Simoes, M. J., Cerri, P. S. (2015). Biology of bone tissue: structure, function, and factors that influence bone cells. BioMed. Res. Int. 2015, 421746. doi: 10.1155/2015/421746
Ghazalpour, A., Cespedes, I., Bennett, B. J., Allayee, H. (2016). Expanding role of gut microbiota in lipid metabolism. Curr. Opin. Lipidol. 27, 141–147. doi: 10.1097/MOL.0000000000000278
Gierynska, M., Szulc-Dabrowska, L., Struzik, J., Mielcarska, M. B., Gregorczyk-Zboroch, K. P. (2022). Integrity of the intestinal barrier: the involvement of epithelial cells and microbiota-A mutual relationship. Anim. 12, 145. doi: 10.3390/ani12020145
Guan, Z., Xuanqi, Z., Zhu, J., Yuan, W., Jia, J., Zhang, C., et al. (2023). Estrogen deficiency induces bone loss through the gut microbiota. Pharmacol. Res. 196, 106930. doi: 10.1016/j.phrs.2023.106930
Guo, M., Liu, H., Yu, Y., Zhu, X., Xie, H., Wei, C., et al. (2023). Lactobacillus rhamnosus GG ameliorates osteoporosis in ovariectomized rats by regulating the Th17/Treg balance and gut microbiota structure. Gut Microbes 15, 2190304. doi: 10.1080/19490976.2023.2190304
Guo, X., Zhong, K., Zhang, J., Hui, L., Zou, L., Xue, H., et al. (2022a). Gut microbiota can affect bone quality by regulating serum estrogen levels. Am. J. Transl. Res. 14, 6043–6055. Available at: https://www.ncbi.nlm.nih.gov/pubmed/36247294.
Guo, X., Zhong, K., Zou, L., Xue, H., Zheng, S., Guo, J., et al. (2022b). Effect of Lactobacillus casei fermented milk on fracture healing in osteoporotic mice. Front. Endocrinol. 13. doi: 10.3389/fendo.2022.1041647
Guss, J. D., Horsfield, M. W., Fontenele, F. F., Sandoval, T. N., Luna, M., Apoorva, F., et al. (2017). Alterations to the gut microbiome impair bone strength and tissue material properties. J. Bone Min. Res. 32, 1343–1353. doi: 10.1002/jbmr.3114
Han, X., Fu, Y., Wang, K., Li, S., Jiang, C., Wang, S., et al. (2023). Epigallocatechin gallate alleviates osteoporosis by regulating the gut microbiota and serum metabolites in rats. Food Funct. 14, 10564–10580. doi: 10.1039/d3fo03233g
Hao, H., Liu, Q., Zheng, T., Li, J., Zhang, T., Yao, Y., et al. (2024). Oral milk-derived extracellular vesicles inhibit osteoclastogenesis and ameliorate bone loss in ovariectomized mice by improving gut microbiota. J. Agric. Food Chem. 72, 4726–4736. doi: 10.1021/acs.jafc.3c07095
He, W., Xie, Z., Thogersen, R., Rasmussen, M. K., Zachariassen, L. F., Jorgensen, N. R., et al. (2022). Effects of calcium source, inulin, and lactose on gut-bone associations in an ovarierectomized rat model. Mol. Nutr. Food Res. 66, e2100883. doi: 10.1002/mnfr.202100883
Heaney, R. P. (2002). Effects of caffeine on bone and the calcium economy. Food Chem. Toxicol. 40, 1263–1270. doi: 10.1016/s0278-6915(02)00094-7
Hong, S., Cha, K. H., Kwon, D. Y., Son, Y. J., Kim, S. M., Choi, J. H., et al. (2021). Agastache rugosa ethanol extract suppresses bone loss via induction of osteoblast differentiation with alteration of gut microbiota. Phytomedicine 84, 153517. doi: 10.1016/j.phymed.2021.153517
Hong, S., Cha, K. H., Park, J. H., Jung, D. S., Choi, J. H., Yoo, G., et al. (2022). Cinnamic acid suppresses bone loss via induction of osteoblast differentiation with alteration of gut microbiota. J. Nutr. Biochem. 101, 108900. doi: 10.1016/j.jnutbio.2021.108900
Hou, K., Wu, Z. X., Chen, X. Y., Wang, J. Q., Zhang, D., Xiao, C., et al. (2022). Microbiota in health and diseases. Signal Transduct Target Ther. 7, 135. doi: 10.1038/s41392-022-00974-4
Hu, X. H., Yang, X. Y., Lian, J., Chen, Y., Zheng, C. Y., Tao, S. Y., et al. (2023). Moringa oleifera leaf attenuate osteoporosis in ovariectomized rats by modulating gut microbiota composition and MAPK signaling pathway. BioMed. Pharmacother. 161, 114434. doi: 10.1016/j.biopha.2023.114434
Huang, D., Wang, J., Zeng, Y., Li, Q., Wang, Y. (2023). Identifying microbial signatures for patients with postmenopausal osteoporosis using gut microbiota analyses and feature selection approaches. Front. Microbiol. 14. doi: 10.3389/fmicb.2023.1113174
Iwobi, N., Sparks, N. R. (2023). Endocrine disruptor-induced bone damage due to hormone dysregulation: A review. Int. J. Mol. Sci. 24, 8263. doi: 10.3390/ijms24098263
Jhong, J. H., Tsai, W. H., Yang, L. C., Chou, C. H., Lee, T. Y., Yeh, Y. T., et al. (2022). Heat-killed lacticaseibacillus paracasei GMNL-653 exerts antiosteoporotic effects by restoring the gut microbiota dysbiosis in ovariectomized mice. Front. Nutr. 9. doi: 10.3389/fnut.2022.804210
Jia, L., Tu, Y., Jia, X., Du, Q., Zheng, X., Yuan, Q., et al (2021). Probiotics ameliorate alveolar bone loss by regulating gut microbiota. Cell Prolif. 4 (7), e13075. doi: 10.1111/cpr.13075
Jia, X., Jia, L., Mo, L., Yuan, S., Zheng, X., He, J., et al. (2019). Berberine ameliorates periodontal bone loss by regulating gut microbiota. J. Dent. Res. 98, 107–116. doi: 10.1177/0022034518797275
Jiang, X., Qi, X., Xie, C. (2023). Lactobacillus plantarum LP45 inhibits the RANKL/OPG signaling pathway and prevents glucocorticoid-induced osteoporosis. Food Nutr. Res. 67, 9064. doi: 10.29219/fnr.v67.9064
Khazai, N., Judd, S. E., Tangpricha, V. (2008). Calcium and vitamin D: skeletal and extraskeletal health. Curr. Rheumatol. Rep. 10, 110–117. doi: 10.1007/s11926-008-0020-y
Khiyali, Z., Rashedi, V., Tavacol, Z., Dehghan, A., Bijani, M. (2024). Smoking, alcohol consumption, drug abuse, and osteoporosis among older adults: a cross-sectional study on PERSIAN cohort study in Fasa. BMC Geriatr. 24, 80. doi: 10.1186/s12877-024-04678-y
Kim, D. E., Kim, J. K., Han, S. K., Jang, S. E., Han, M. J., Kim, D. H. (2019). Lactobacillus plantarum NK3 and Bifidobacterium longum NK49 Alleviate Bacterial Vaginosis and Osteoporosis in Mice by Suppressing NF-kappaB-Linked TNF-alpha Expression. J. Med. Food 22, 1022–1031. doi: 10.1089/jmf.2019.4419
Kosaka, S., Nadatani, Y., Higashimori, A., Otani, K., Fujimoto, K., Nagata, Y., et al. (2021). Ovariectomy-induced dysbiosis may have a minor effect on bone in mice. Microorganisms 9, 2563. doi: 10.3390/microorganisms9122563
Kwa, W. T., Sundarajoo, S., Toh, K. Y., Lee, J. (2023). Application of emerging technologies for gut microbiome research. Singapore Med. J. 64, 45–52. doi: 10.4103/Singaporemedj.SMJ-2021-432
Lan, H., Liu, W. H., Zheng, H., Feng, H., Zhao, W., Hung, W. L., et al. (2022). Bifidobacterium lactis BL-99 protects mice with osteoporosis caused by colitis via gut inflammation and gut microbiota regulation. Food Funct. 13, 1482–1494. doi: 10.1039/d1fo02218k
Lawenius, L., Cowardin, C., Grahnemo, L., Scheffler, J. M., Horkeby, K., Engdahl, C., et al. (2023). Transplantation of gut microbiota from old mice into young healthy mice reduces lean mass but not bone mass. Gut Microbes 15, 2236755. doi: 10.1080/19490976.2023.2236755
Lee, C. C., Liao, Y. C., Lee, M. C., Lin, K. J., Hsu, H. Y., Chiou, S. Y., et al. (2021). Lactobacillus plantarum TWK10 attenuates aging-associated muscle weakness, bone loss, and cognitive impairment by modulating the gut microbiome in mice. Front. Nutr. 8. doi: 10.3389/fnut.2021.708096
Li, J. Y., Chassaing, B., Tyagi, A. M., Vaccaro, C., Luo, T., Adams, J., et al. (2016). Sex steroid deficiency-associated bone loss is microbiota dependent and prevented by probiotics. J. Clin. Invest. 126, 2049–2063. doi: 10.1172/JCI86062
Li, L., Chen, B., Zhu, R., Li, R., Tian, Y., Liu, C., et al. (2019). Fructus Ligustri Lucidi preserves bone quality through the regulation of gut microbiota diversity, oxidative stress, TMAO and Sirt6 levels in aging mice. Aging (Albany NY) 11, 9348–9368. doi: 10.18632/aging.102376
Li, S., Han, X., Liu, N., Chang, J., Liu, G., Hu, S. (2023). Lactobacillus plantarum attenuates glucocorticoid-induced osteoporosis by altering the composition of rat gut microbiota and serum metabolic profile. Front. Immunol. 14. doi: 10.3389/fimmu.2023.1285442
Li, B., Liu, M., Wang, Y., Gong, S., Yao, W., Li, W., et al. (2020a). Puerarin improves the bone micro-environment to inhibit OVX-induced osteoporosis via modulating SCFAs released by the gut microbiota and repairing intestinal mucosal integrity. BioMed. Pharmacother. 132, 110923. doi: 10.1016/j.biopha.2020.110923
Li, M., Wang, F. (2021). Role of intestinal microbiota on gut homeostasis and rheumatoid arthritis. J. Immunol. Res. 2021, 8167283. doi: 10.1155/2021/8167283
Li, J., Yang, M., Lu, C., Han, J., Tang, S., Zhou, J., et al. (2020b). Tuna bone powder alleviates glucocorticoid-induced osteoporosis via coregulation of the NF-kappaB and wnt/beta-catenin signaling pathways and modulation of gut microbiota composition and metabolism. Mol. Nutr. Food Res. 64, e1900861. doi: 10.1002/mnfr.201900861
Li, Y. J., Zhang, Y. W., Cao, M. M., Zhang, R. L., Wu, M. T., Rui, Y. F., et al. (2024). The supplementation of Rothia as a potential preventive approach for bone loss in mice with ovariectomy-induced osteoporosis. Food Sci. Nutr. 12, 340–353. doi: 10.1002/fsn3.3747
Lin, H., Liu, T., Li, X., Gao, X., Wu, T., Li, P. (2020). The role of gut microbiota metabolite trimethylamine N-oxide in functional impairment of bone marrow mesenchymal stem cells in osteoporosis disease. Ann. Transl. Med. 8, 1009. doi: 10.21037/atm-20-5307
Lin, X., Xiao, H. M., Liu, H. M., Lv, W. Q., Greenbaum, J., Gong, R., et al. (2023). Gut microbiota impacts bone via Bacteroides vulgatus-valeric acid-related pathways. Nat. Commun. 14, 6853. doi: 10.1038/s41467-023-42005-y
Liu, C., Chen, J., Wang, Z., Li, Y., Zhang, Y., Li, G. (2024). Nodakenin ameliorates ovariectomy-induced bone loss by regulating gut microbiota. Molecules 29, 1240. doi: 10.3390/molecules29061240
Liu, H., Gu, R., Li, W., Zhou, W., Cong, Z., Xue, J., et al. (2019). Lactobacillus rhamnosus GG attenuates tenofovir disoproxil fumarate-induced bone loss in male mice via gut-microbiota-dependent anti-inflammation. Ther. Adv. Chronic Dis. 10, 2040622319860653. doi: 10.1177/2040622319860653
Liu, H., Gu, R., Zhu, Y., Lian, X., Wang, S., Liu, X., et al. (2020a). D-mannose attenuates bone loss in mice via Treg cell proliferation and gut microbiota-dependent anti-inflammatory effects. Ther. Adv. Chronic Dis. 11, 2040622320912661. doi: 10.1177/2040622320912661
Liu, Y., Guo, Y. L., Meng, S., Gao, H., Sui, L. J., Jin, S., et al. (2020c). Gut microbiota-dependent Trimethylamine N-Oxide are related with hip fracture in postmenopausal women: a matched case-control study. Aging (Albany NY) 12, 10633–10641. doi: 10.18632/aging.103283
Liu, J., Liu, J., Liu, L., Zhang, G., Zhou, A., Peng, X. (2020b). The gut microbiota alteration and the key bacteria in Astragalus polysaccharides (APS)-improved osteoporosis. Food Res. Int. 138, 109811. doi: 10.1016/j.foodres.2020.109811
Liu, Z., Xu, X., Shen, Y., Hao, Y., Cui, W., Li, W., et al. (2022). Altered gut microbiota and metabolites profile are associated with reduced bone metabolism in ethanol-induced osteoporosis. Cell Prolif. 55, e13245. doi: 10.1111/cpr.13245
Lu, L., Tang, M., Li, J., Xie, Y., Li, Y., Xie, J., et al. (2021a). Gut microbiota and serum metabolic signatures of high-fat-induced bone loss in mice. Front. Cell Infect. Microbiol. 11. doi: 10.3389/fcimb.2021.788576
Lu, Y., Yang, J., Dong, C., Fu, Y., Liu, H. (2021b). Gut microbiome-mediated changes in bone metabolism upon infrared light exposure in rats. J. Photochem. Photobiol. B 217, 112156. doi: 10.1016/j.jphotobiol.2021.112156
Lyu, Z., Hu, Y., Guo, Y., Liu, D. (2023). Modulation of bone remodeling by the gut microbiota: a new therapy for osteoporosis. Bone Res. 11, 31. doi: 10.1038/s41413-023-00264-x
Ma, S., Qin, J., Hao, Y., Fu, L. (2020a). Association of gut microbiota composition and function with an aged rat model of senile osteoporosis using 16S rRNA and metagenomic sequencing analysis. Aging (Albany NY) 12, 10795–10808. doi: 10.18632/aging.103293
Ma, S., Qin, J., Hao, Y., Shi, Y., Fu, L. (2020b). Structural and functional changes of gut microbiota in ovariectomized rats and their correlations with altered bone mass. Aging (Albany NY) 12, 10736–10753. doi: 10.18632/aging.103290
Ma, S., Wang, N., Zhang, P., Wu, W., Fu, L. (2021). Fecal microbiota transplantation mitigates bone loss by improving gut microbiome composition and gut barrier function in aged rats. PeerJ 9, e12293. doi: 10.7717/peerj.12293
Makitie, R. E., Costantini, A., Kampe, A., Alm, J. J., Makitie, O. (2019). New insights into monogenic causes of osteoporosis. Front. Endocrinol. 10. doi: 10.3389/fendo.2019.00070
Martin, A. M., Sun, E. W., Rogers, G. B., Keating, D. J. (2019). The influence of the gut microbiome on host metabolism through the regulation of gut hormone release. Front. Physiol. 10. doi: 10.3389/fphys.2019.00428
Matijasic, M., Mestrovic, T., Paljetak, H. C., Peric, M., Baresic, A., Verbanac, D. (2020). Gut microbiota beyond bacteria-mycobiome, virome, archaeome, and eukaryotic parasites in IBD. Int. J. Mol. Sci. 21, 2668. doi: 10.3390/ijms21082668
Mazziotta, C., Tognon, M., Martini, F., Torreggiani, E., Rotondo, J. C. (2023). Probiotics mechanism of action on immune cells and beneficial effects on human health. Cells 12, 184. doi: 10.3390/cells12010184
McCabe, L. R., Irwin, R., Tekalur, A., Evans, C., Schepper, J. D., Parameswaran, N., et al. (2019). Corrigendum to “Exercise prevents high fat diet-induced bone loss, marrow adiposity and dysbiosis in male mice” [Bone, volume 118, January 2019, pages 20-31. Bone 127, 677–678. doi: 10.1016/j.bone.2019.07.033
Mei, F., Meng, K., Gu, Z., Yun, Y., Zhang, W., Zhang, C., et al. (2021). Arecanut (Areca catechu L.) Seed Polyphenol-Ameliorated Osteoporosis by Altering Gut Microbiome via LYZ and the Immune System in Estrogen-Deficient Rats. J. Agric. Food Chem. 69, 246–258. doi: 10.1021/acs.jafc.0c06671
Moreno-Indias, I., Cardona, F., Tinahones, F. J., Queipo-Ortuno, M. I. (2014). Impact of the gut microbiota on the development of obesity and type 2 diabetes mellitus. Front. Microbiol. 5. doi: 10.3389/fmicb.2014.00190
Nagpal, R., Kumar, A., Kumar, M., Behare, P. V., Jain, S., Yadav, H. (2012). Probiotics, their health benefits and applications for developing healthier foods: a review. FEMS Microbiol. Lett. 334, 1–15. doi: 10.1111/j.1574-6968.2012.02593.x
Niemela, O., Bloigu, A., Bloigu, R., Halkola, A. S., Niemela, M., Aalto, M., et al. (2022). Impact of physical activity on the characteristics and metabolic consequences of alcohol consumption: A cross-sectional population-based study. Int. J. Env. Res. Public Heal. 19, 15048. doi: 10.3390/ijerph192215048
Niu, Y. B., Zhang, Y. H., Sun, Y., Song, X. Z., Li, Z. H., Xie, M., et al. (2023). Asperosaponin VI protects against bone loss due to hindlimb unloading in skeletally growing mice through regulating microbial dysbiosis altering the 5-HT pathway. Calcif. Tissue Int. 112, 389–402. doi: 10.1007/s00223-022-01057-w
Nogal, A., Valdes, A. M., Menni, C. (2021). The role of short-chain fatty acids in the interplay between gut microbiota and diet in cardio-metabolic health. Gut Microbes 13, 1–24. doi: 10.1080/19490976.2021.1897212
Okoro, P. C., Orwoll, E. S., Huttenhower, C., Morgan, X., Kuntz, T. M., McIver, L. J., et al. (2023). A two-cohort study on the association between the gut microbiota and bone density, microarchitecture, and strength. Front. Endocrinol. 14. doi: 10.3389/fendo.2023.1237727
Padilla Colon, C. J., Molina-Vicenty, I. L., Frontera-Rodriguez, M., Garcia-Ferre, A., Rivera, B. P., Cintron-Velez, G., et al. (2018). Muscle and Bone Mass Loss in the Elderly Population: Advances in diagnosis and treatment. J. BioMed. 3, 40–49. doi: 10.7150/jbm.23390
Pan, W., Du, J., An, L., Xu, G., Yuan, G., Sheng, Y., et al. (2023). Sika deer velvet antler protein extract modulater bone metabolism and the structure of gut microbiota in ovariectomized mice. Food Sci. Nutr. 11, 3309–3319. doi: 10.1002/fsn3.3316
Panday, K., Gona, A., Humphrey, M. B. (2014). Medication-induced osteoporosis: screening and treatment strategies. Ther. Adv. Musculoskelet Dis. 6, 185–202. doi: 10.1177/1759720X14546350
Park, S. M., Jee, J., Joung, J. Y., Cho, Y. Y., Sohn, S. Y., Jin, S. M., et al. (2014). High dietary sodium intake assessed by 24-hour urine specimen increase urinary calcium excretion and bone resorption marker. J. Bone Metab. 21, 189–194. doi: 10.11005/jbm.2014.21.3.189
Parvaneh, K., Ebrahimi, M., Sabran, M. R., Karimi, G., Hwei, A. N., Abdul-Majeed, S., et al. (2015). Probiotics (Bifidobacterium longum) increase bone mass density and upregulate sparc and bmp-2 genes in rats with bone loss resulting from ovariectomy. BioMed. Res. Int. 2015, 897639. doi: 10.1155/2015/897639
Peng, R., Song, C., Gou, S., Liu, H., Kang, H., Dong, Y., et al. (2024). Gut Clostridium sporogenes-derived indole propionic acid suppresses osteoclast formation by activating pregnane X receptor. Pharmacol. Res. 202, 107121. doi: 10.1016/j.phrs.2024.107121
Pignolo, R. J., Law, S. F., Chandra, A. (2021). Bone aging, cellular senescence, and osteoporosis. JBMR Plus 5, e10488. doi: 10.1002/jbm4.10488
Portincasa, P., Bonfrate, L., Vacca, M., De Angelis, M., Farella, I., Lanza, E., et al. (2022). Gut microbiota and short chain fatty acids: implications in glucose homeostasis. Int. J. Mol. Sci. 23, 1105. doi: 10.3390/ijms23031105
Pouresmaeili, F., Kamalidehghan, B., Kamarehei, M., Goh, Y. M. (2018). A comprehensive overview on osteoporosis and its risk factors. Ther. Clin. Risk Manag. 14, 2029–2049. doi: 10.2147/TCRM.S138000
Qi, W., Ma, T., Ji, Y., Jia, H., Sun, Q., Zhang, D. (2024). Correction: Cordymin alleviates osteoporosis induced by hindlimb unloading via regulating the gut - microelements -bone axis –for non-clinical studies. BMC Musculoskelet Disord. 25, 88. doi: 10.1186/s12891-023-07154-7
Qiao, X., Zhang, K., Li, X., Lv, Z., Wei, W., Zhou, R., et al. (2022). Gut microbiota and fecal metabolic signatures in rat models of disuse-induced osteoporosis. Front. Cell Infect. Microbiol. 12. doi: 10.3389/fcimb.2022.1018897
Quach, D., Collins, F., Parameswaran, N., McCabe, L., Britton, R. A. (2018). Microbiota reconstitution does not cause bone loss in germ-free mice. mSphere 3, e00545-17. doi: 10.1128/mSphereDirect.00545-17
Ralston, S. H. (2002). Genetic control of susceptibility to osteoporosis. J. Clin. Endocrinol. Metab. 87, 2460–2466. doi: 10.1210/jcem.87.6.8621
Reuter, S. E., Schultz, H. B., Ward, M. B., Grant, C. L., Paech, G. M., Banks, S., et al. (2021). The effect of high-dose, short-term caffeine intake on the renal clearance of calcium, sodium and creatinine in healthy adults. Br. J. Clin. Pharmacol. 87, 4461–4466. doi: 10.1111/bcp.14856
Rinninella, E., Raoul, P., Cintoni, M., Franceschi, F., Miggiano, G. A. D., Gasbarrini, A., et al. (2019). What is the healthy gut microbiota composition? A changing ecosystem across age, environment, diet, and diseases. Microorganisms 7, 14. doi: 10.3390/microorganisms7010014
Robling, A. G., Turner, C. H. (2009). Mechanical signaling for bone modeling and remodeling. Crit. Rev. Eukaryot. Gene Expr. 19, 319–338. doi: 10.1615/critreveukargeneexpr.v19.i4.50
Rosello-Anon, A., Chiappe, C., Valverde-Vazquez, M. R., Sanguesa-Nebot, M. J., Gomez-Cabrera, M. C., Perez-Martinez, G., et al. (2023). Pilot study to determine the association between gut microbiota and fragility hip fracture. Rev. Esp. Cir. Ortop. Traumatol. 67, 279–289. doi: 10.1016/j.recot.2023.01.002
Rowe, P., Koller, A., Sharma, S. (2024). “Physiology, bone remodeling,” in StatPearls [Internet]. (Treasure Island (FL): StatPearls Publishing). Available at: https://www.ncbi.nlm.nih.gov/pubmed/29763038.
Roy, S., Dhaneshwar, S. (2023). Role of prebiotics, probiotics, and synbiotics in management of inflammatory bowel disease: Current perspectives. World J. Gastroenterol. 29, 2078–2100. doi: 10.3748/wjg.v29.i14.2078
Salazar, J., Duran, P., Diaz, M. P., Chacin, M., Santeliz, R., Mengual, E., et al. (2023). Exploring the relationship between the gut microbiota and ageing: A possible age modulator. Int. J. Env. Res. Public Heal. 20, 5845. doi: 10.3390/ijerph20105845
Schepper, J. D., Collins, F., Rios-Arce, N. D., Kang, H. J., Schaefer, L., Gardinier, J. D., et al. (2020). Involvement of the gut microbiota and barrier function in glucocorticoid-induced osteoporosis. J. Bone Min. Res. 35, 801–820. doi: 10.1002/jbmr.3947
Schepper, J. D., Irwin, R., Kang, J., Dagenais, K., Lemon, T., Shinouskis, A., et al. (2017). Probiotics in gut-bone signaling. Adv. Exp. Med. Biol. 1033, 225–247. doi: 10.1007/978-3-319-66653-2_11
Selvaraj, V., Sekaran, S., Dhanasekaran, A., Warrier, S. (2024). Type 1 collagen: Synthesis, structure and key functions in bone mineralization. Differentiation 136, 100757. doi: 10.1016/j.diff.2024.100757
Shaikh, S. D., Sun, N., Canakis, A., Park, W. Y., Weber, H. C. (2023). Irritable bowel syndrome and the gut microbiome: A comprehensive review. J. Clin. Med. 12, 2558. doi: 10.3390/jcm12072558
Shan, Y., Lee, M., Chang, E. B. (2022). The gut microbiome and inflammatory bowel diseases. Annu. Rev. Med. 73, 455–468. doi: 10.1146/annurev-med-042320-021020
Shen, Q., Zhang, C., Qin, X., Zhang, H., Zhang, Z., Richel, A. (2021). Modulation of gut microbiota by chondroitin sulfate calcium complex during alleviation of osteoporosis in ovariectomized rats. Carbohydr. Polym. 266, 118099. doi: 10.1016/j.carbpol.2021.118099
Singh, A., Mehdi, A. A., Srivastava, R. N., Verma, N. S. (2012). Immunoregulation of bone remodelling. Int. J. Crit. Illn. Inj. Sci. 2, 75–81. doi: 10.4103/2229-5151.97271
Sjogren, K., Engdahl, C., Henning, P., Lerner, U. H., Tremaroli, V., Lagerquist, M. K., et al. (2012). The gut microbiota regulates bone mass in mice. J. Bone Min. Res. 27, 1357–1367. doi: 10.1002/jbmr.1588
Smit, A. E., Meijer, O. C., Winter, E. M. (2024). The multi-faceted nature of age-associated osteoporosis. Bone Rep. 20, 101750. doi: 10.1016/j.bonr.2024.101750
Song, C., Ma, Y., Wang, Y., Li, P., Chen, Y., Liu, H., et al. (2023). Diosgenin reduces bone loss through the regulation of gut microbiota in ovariectomized rats. Gene 869, 147383. doi: 10.1016/j.gene.2023.147383
Sozen, T., Ozisik, L., Basaran, N. C. (2017). An overview and management of osteoporosis. Eur. J. Rheumatol. 4, 46–56. doi: 10.5152/eurjrheum.2016.048
Sun, P., Zhang, C., Huang, Y., Yang, J., Zhou, F., Zeng, J., et al. (2022). Jiangu granule ameliorated OVX rats bone loss by modulating gut microbiota-SCFAs-Treg/Th17 axis. BioMed. Pharmacother. 150, 112975. doi: 10.1016/j.biopha.2022.112975
Tanabe, K., Nakamura, S., Moriyama-Hashiguchi, M., Kitajima, M., Ejima, H., Imori, C., et al. (2019). Dietary fructooligosaccharide and glucomannan alter gut microbiota and improve bone metabolism in senescence-accelerated mouse. J. Agric. Food Chem. 67, 867–874. doi: 10.1021/acs.jafc.8b05164
Tavakoli, S., Xiao, L. (2019). Depletion of intestinal microbiome partially rescues bone loss in sickle cell disease male mice. Sci. Rep. 9, 8659. doi: 10.1038/s41598-019-45270-4
Thursby, E., Juge, N. (2017). Introduction to the human gut microbiota. Biochem. J. 474, 1823–1836. doi: 10.1042/BCJ20160510
Tousen, Y., Matsumoto, Y., Nagahata, Y., Kobayashi, I., Inoue, M., Ishimi, Y. (2019). Resistant starch attenuates bone loss in ovariectomised mice by regulating the intestinal microbiota and bone-marrow inflammation. Nutrients 11, 297. doi: 10.3390/nu11020297
Tu, M. Y., Han, K. Y., Chang, G. R., Lai, G. D., Chang, K. Y., Chen, C. F., et al. (2020). Kefir peptides prevent estrogen deficiency-induced bone loss and modulate the structure of the gut microbiota in ovariectomized mice. Nutrients 12, 3432. doi: 10.3390/nu12113432
Ul-Haq, A., Seo, H., Jo, S., Park, H., Kim, S., Lee, Y., et al. (2022). Characterization of fecal microbiomes of osteoporotic patients in korea. Pol. J. Microbiol. 71, 601–613. doi: 10.33073/pjm-2022-045
Wan, X., Eguchi, A., Fujita, Y., Ma, L., Wang, X., Yang, Y., et al. (2022). Effects of (R)-ketamine on reduced bone mineral density in ovariectomized mice: A role of gut microbiota. Neuropharmacology 213, 109139. doi: 10.1016/j.neuropharm.2022.109139
Wang, L. T., Chen, L. R., Chen, K. H. (2023b). Hormone-related and drug-induced osteoporosis: A cellular and molecular overview. Int. J. Mol. Sci. 24, 5814. doi: 10.3390/ijms24065814
Wang, Z., Chen, K., Wu, C., Chen, J., Pan, H., Liu, Y., et al. (2021). An emerging role of Prevotella histicola on estrogen deficiency-induced bone loss through the gut microbiota-bone axis in postmenopausal women and in ovariectomized mice. Am. J. Clin. Nutr. 114, 1304–1313. doi: 10.1093/ajcn/nqab194
Wang, Y., Gao, X., Lv, J., Zeng, Y., Li, Q., Wang, L., et al. (2022f). Gut microbiome signature are correlated with bone mineral density alterations in the Chinese elders. Front. Cell Infect. Microbiol. 12. doi: 10.3389/fcimb.2022.827575
Wang, H., Liu, J., Wu, Z., Zhao, Y., Cao, M., Shi, B., et al. (2023a). Gut microbiota signatures and fecal metabolites in postmenopausal women with osteoporosis. Gut Pathog. 15, 33. doi: 10.1186/s13099-023-00553-0
Wang, N., Ma, S., Fu, L. (2022b). Gut microbiota dysbiosis as one cause of osteoporosis by impairing intestinal barrier function. Calcif. Tissue Int. 110, 225–235. doi: 10.1007/s00223-021-00911-7
Wang, N., Ma, S., Fu, L. (2022c). Gut microbiota feature of senile osteoporosis by shallow shotgun sequencing using aged rats model. Genes (Basel) 13, 619. doi: 10.3390/genes13040619
Wang, N., Meng, F., Ma, S., Fu, L. (2022d). Species-level gut microbiota analysis in ovariectomized osteoporotic rats by Shallow shotgun sequencing. Gene 817, 146205. doi: 10.1016/j.gene.2022.146205
Wang, J., Wang, Y., Gao, W., Wang, B., Zhao, H., Zeng, Y., et al. (2017). Diversity analysis of gut microbiota in osteoporosis and osteopenia patients. PeerJ 5, e3450. doi: 10.7717/peerj.3450
Wang, S., Wang, S., Wang, X., Xu, Y., Zhang, X., Han, Y., et al. (2022e). Effects of icariin on modulating gut microbiota and regulating metabolite alterations to prevent bone loss in ovariectomized rat model. Front. Endocrinol. 13. doi: 10.3389/fendo.2022.874849
Wang, J., Wu, S., Zhang, Y., Yang, J., Hu, Z. (2022a). Gut microbiota and calcium balance. Front. Microbiol. 13. doi: 10.3389/fmicb.2022.1033933
Wei, L. Q., Cheong, I. H., Yang, G. H., Li, X. G., Kozlakidis, Z., Ding, L., et al. (2021a). The application of high-throughput technologies for the study of microbiome and cancer. Front. Genet. 12. doi: 10.3389/fgene.2021.699793
Wei, M., Li, C., Dai, Y., Zhou, H., Cui, Y., Zeng, Y., et al. (2021b). High-throughput absolute quantification sequencing revealed osteoporosis-related gut microbiota alterations in Han Chinese elderly. Front. Cell Infect. Microbiol. 11. doi: 10.3389/fcimb.2021.630372
Wei, Q., Zhou, Y., Hu, Z., Shi, Y., Ning, Q., Ren, K., et al. (2024). Function-oriented mechanism discovery of coumarins from Psoralea corylifolia L. in the treatment of ovariectomy-induced osteoporosis based on multi-omics analysis. J. Ethnopharmacol. 329, 118130. doi: 10.1016/j.jep.2024.118130
Wen, K., Tao, L., Tao, Z., Meng, Y., Zhou, S., Chen, J., et al. (2020). Fecal and serum metabolomic signatures and microbial community profiling of postmenopausal osteoporosis mice model. Front. Cell Infect. Microbiol. 10. doi: 10.3389/fcimb.2020.535310
Wu, M., Chen, C., Lei, H., Cao, Z., Zhang, C., Du, R., et al. (2023). Dietary isoquercetin ameliorates bone loss via restoration of the gut microbiota and lipopolysaccharide-triggered inflammatory status in ovariectomy mice. J. Agric. Food Chem. 71, 15981–15990. doi: 10.1021/acs.jafc.3c00205
Xiao, H. H., Yu, X., Yang, C., Chan, C. O., Lu, L., Cao, S., et al. (2021). Prenylated isoflavonoids-rich extract of erythrinae cortex exerted bone protective effects by modulating gut microbial compositions and metabolites in ovariectomized rats. Nutrients 13, 2943. doi: 10.3390/nu13092943
Xie, H., Hua, Z., Guo, M., Lin, S., Zhou, Y., Weng, Z., et al. (2022). Gut microbiota and metabonomics used to explore the mechanism of Qing’e Pills in alleviating osteoporosis. Pharm. Biol. 60, 785–800. doi: 10.1080/13880209.2022.2056208
Xu, Z., Xie, Z., Sun, J., Huang, S., Chen, Y., Li, C., et al. (2020). Gut microbiome reveals specific dysbiosis in primary osteoporosis. Front. Cell Infect. Microbiol. 10. doi: 10.3389/fcimb.2020.00160
Xue, C., Pan, W., Lu, X., Guo, J., Xu, G., Sheng, Y., et al. (2021). Effects of compound deer bone extract on osteoporosis model mice and intestinal microflora. J. Food Biochem. 45, e13740. doi: 10.1111/jfbc.13740
Yang, X., Chang, T., Yuan, Q., Wei, W., Wang, P., Song, X., et al. (2022). Changes in the composition of gut and vaginal microbiota in patients with postmenopausal osteoporosis. Front. Immunol. 13. doi: 10.3389/fimmu.2022.930244
Yang, C. Y., Cheng-Yen Lai, J., Huang, W. L., Hsu, C. L., Chen, S. J. (2021). Effects of sex, tobacco smoking, and alcohol consumption osteoporosis development: Evidence from Taiwan biobank participants. Tob. Induc. Dis. 19, 52. doi: 10.18332/tid/136419
Yin, Q., Gu, J., Ren, P., Guan, Z., Wang, Y., Bai, R., et al. (2024). Microbiome dysbiosis by antibiotics protects cartilage degradation in OAOP mice. J. Endocrinol. 261, e230330. doi: 10.1530/JOE-23-0330
Yoon, J. H., Do, J. S., Velankanni, P., Lee, C. G., Kwon, H. K. (2023). Gut microbial metabolites on host immune responses in health and disease. Immune Netw. 23, e6. doi: 10.4110/in.2023.23.e6
Yu, J., Hang, Y., Sun, W., Wang, G., Xiong, Z., Ai, L., et al. (2022). Anti-osteoporotic effect of lactobacillus brevis AR281 in an ovariectomized mouse model mediated by inhibition of osteoclast differentiation. Biol. 11, 359. doi: 10.3390/biology11030359
Yuan, Y., Gan, C., Wang, M., Zou, J., Wang, Z., Li, S., et al. (2024). Association of serum trimethylamine N-oxide levels and bone mineral density in type 2 diabetes mellitus. Endocrine. 84, 958–968. doi: 10.1007/s12020-024-03699-2
Yuan, S., Shen, J. (2021). Bacteroides vulgatus diminishes colonic microbiota dysbiosis ameliorating lumbar bone loss in ovariectomized mice. Bone 142, 115710. doi: 10.1016/j.bone.2020.115710
Yuan, Y., Yang, J., Zhuge, A., Li, L., Ni, S. (2022). Gut microbiota modulates osteoclast glutathione synthesis and mitochondrial biogenesis in mice subjected to ovariectomy. Cell Prolif. 55, e13194. doi: 10.1111/cpr.13194
Zeng, H. Q., Li, G., Zhou, K. X., Li, A. D., Liu, W., Zhang, Y. (2024). Causal link between gut microbiota and osteoporosis analyzed via Mendelian randomization. Eur. Rev. Med. Pharmacol. Sci. 28, 542–555. doi: 10.26355/eurrev_202401_35052
Zhang, Y. W., Cao, M. M., Li, Y. J., Lu, P. P., Dai, G. C., Zhang, M., et al. (2022b). Fecal microbiota transplantation ameliorates bone loss in mice with ovariectomy-induced osteoporosis via modulating gut microbiota and metabolic function. J. Orthop. Transl. 37, 46–60. doi: 10.1016/j.jot.2022.08.003
Zhang, Y. W., Cao, M. M., Li, Y. J., Sheng, R. W., Zhang, R. L., Wu, M. T., et al. (2023c). The preventive effects of probiotic prevotella histicola on the bone loss of mice with ovariectomy-mediated osteoporosis. Microorganisms 11, 950. doi: 10.3390/microorganisms11040950
Zhang, Y. W., Cao, M. M., Li, Y. J., Zhang, R. L., Wu, M. T., Yu, Q., et al. (2022c). Fecal microbiota transplantation as a promising treatment option for osteoporosis. J. Bone Min. Metab. 40, 874–889. doi: 10.1007/s00774-022-01375-x
Zhang, J., Liang, X., Tian, X., Zhao, M., Mu, Y., Yi, H., et al. (2024). Bifidobacterium improves oestrogen-deficiency-induced osteoporosis in mice by modulating intestinal immunity. Food Funct. 15, 1840–1851. doi: 10.1039/d3fo05212e
Zhang, Z., Lin, T., Meng, Y., Hu, M., Shu, L., Jiang, H., et al. (2021). FOS/GOS attenuates high-fat diet induced bone loss via reversing microbiota dysbiosis, high intestinal permeability and systemic inflammation in mice. Metabolism 119, 154767. doi: 10.1016/j.metabol.2021.154767
Zhang, F., Xu, J., Hu, Y., Fang, J., Yang, M., Huang, K., et al. (2023a). Diallyl trisulfide ameliorates bone loss and alters specific gut microbiota and serum metabolites in natural aging mice. Food Funct. 14, 7642–7653. doi: 10.1039/d3fo01840g
Zhang, R. K., Yan, K., Chen, H. F., Zhang, Y., Li, G. J., Chen, X. G., et al. (2023b). Anti-osteoporotic drugs affect the pathogenesis of gut microbiota and its metabolites: a clinical study. Front. Cell Infect. Microbiol. 13. doi: 10.3389/fcimb.2023.1091083
Zhang, J., Zhang, Q., Liu, H., Liu, X., Yu, Y., Han, D., et al. (2022a). Soy-whey dual-protein alleviates osteoporosis of ovariectomized rats via regulating bone fat metabolism through gut-liver-bone axis. Nutrition 103–104, 111723. doi: 10.1016/j.nut.2022.111723
Zhao, X., Ai, J., Mao, H., Gao, X. (2019). Effects of Eclipta prostrata on gut microbiota of SAMP6 mice with osteoporosis. J. Med. Microbiol. 68, 402–416. doi: 10.1099/jmm.0.000936
Zhao, X., Wang, Y., Nie, Z., Han, L., Zhong, X., Yan, X., et al. (2020). Eucommia ulmoides leaf extract alters gut microbiota composition, enhances short-chain fatty acids production, and ameliorates osteoporosis in the senescence-accelerated mouse P6 (SAMP6) model. Food Sci. Nutr. 8, 4897–4906. doi: 10.1002/fsn3.1779
Zheng, L., Ji, Y. Y., Wen, X. L., Duan, S. L. (2022). Fecal microbiota transplantation in the metabolic diseases: Current status and perspectives. World J. Gastroenterol. 28, 2546–2560. doi: 10.3748/wjg.v28.i23.2546
Zheng, D., Liwinski, T., Elinav, E. (2020). Interaction between microbiota and immunity in health and disease. Cell Res. 30, 492–506. doi: 10.1038/s41422-020-0332-7
Zhou, J., Cheng, J., Liu, L., Luo, J., Peng, X. (2023). Lactobacillus acidophilus (LA) fermenting astragalus polysaccharides (APS) improves calcium absorption and osteoporosis by altering gut microbiota. Foods 12, 275. doi: 10.3390/foods12020275
Keywords: osteoporosis, gut microbiota, risk factors, short-chain fatty acids, fecal microbiota transplantation, therapeutic interventions
Citation: Hao L, Yan Y, Huang G and Li H (2024) From gut to bone: deciphering the impact of gut microbiota on osteoporosis pathogenesis and management. Front. Cell. Infect. Microbiol. 14:1416739. doi: 10.3389/fcimb.2024.1416739
Received: 13 April 2024; Accepted: 06 September 2024;
Published: 25 September 2024.
Edited by:
Jorge A. M. Pereira, Universidade da Madeira, PortugalReviewed by:
Yangyang Wang, Northwestern Polytechnical University, ChinaCopyright © 2024 Hao, Yan, Huang and Li. This is an open-access article distributed under the terms of the Creative Commons Attribution License (CC BY). The use, distribution or reproduction in other forums is permitted, provided the original author(s) and the copyright owner(s) are credited and that the original publication in this journal is cited, in accordance with accepted academic practice. No use, distribution or reproduction is permitted which does not comply with these terms.
*Correspondence: Hui Li, bGlodWkzMjdAMTYzLmNvbQ==
Disclaimer: All claims expressed in this article are solely those of the authors and do not necessarily represent those of their affiliated organizations, or those of the publisher, the editors and the reviewers. Any product that may be evaluated in this article or claim that may be made by its manufacturer is not guaranteed or endorsed by the publisher.
Research integrity at Frontiers
Learn more about the work of our research integrity team to safeguard the quality of each article we publish.