- 1Departamento de Biofísica, Universidade Federal do Rio Grande do Sul, RS, Porto Alegre, Brazil
- 2Institut National de la Recherche Scientifique (INRS)-Centre Armand-Frappier Santé Biotechnologie, Laval, QC, Canada
In Escherichia coli, the disaccharide trehalose can be metabolized as a carbon source or be accumulated as an osmoprotectant under osmotic stress. In hypertonic environments, E. coli accumulates trehalose in the cell by synthesis from glucose mediated by the cytosolic enzymes OtsA and OtsB. Trehalose in the periplasm can be hydrolyzed into glucose by the periplasmic trehalase TreA. We have previously shown that a treA mutant of extraintestinal E. coli strain BEN2908 displayed increased resistance to osmotic stress by 0.6 M urea, and reduced production of type 1 fimbriae, reduced invasion of avian fibroblasts, and decreased bladder colonization in a murine model of urinary tract infection. Since loss of TreA likely results in higher periplasmic trehalose concentrations, we wondered if deletion of otsA and otsB genes, which would lead to decreased internal trehalose concentrations, would reduce resistance to stress by 0.6 M urea and promote type 1 fimbriae production. The BEN2908ΔotsBA mutant was sensitive to osmotic stress by urea, but displayed an even more pronounced reduction in production of type 1 fimbriae, with the consequent reduction in adhesion/invasion of avian fibroblasts and reduced bladder colonization in the murine urinary tract. The BEN2908ΔtreAotsBA mutant also showed a reduction in production of type 1 fimbriae, but in contrast to the ΔotsBA mutant, resisted better than the wild type in the presence of urea. We hypothesize that, in BEN2908, resistance to stress by urea would depend on the levels of periplasmic trehalose, but type 1 fimbriae production would be influenced by the levels of cytosolic trehalose.
1 Introduction
Trehalose is an α(1➔1)-dimer of glucose and is present in organisms of all Domains of life, being absent only in vertebrates (Germer et al., 1998; Joseph et al., 2010; Argüelles, 2014). In all organisms that have been studied, trehalose plays a dual role: as an energy source and as a protector against various forms of stress, such as high osmolarity, dehydration, heat, cold and oxidative stress (Elbein et al., 2003; Ruhal et al., 2013). Accordingly, Escherichia coli can metabolize trehalose as a carbon source, or can accumulate it under osmotic stress or low temperature (Boos et al., 1990; Kandror et al., 2002). Under isotonic conditions, E. coli K-12 internalizes trehalose through a phosphotransferase system (EIICBTre, encoded by treB) (Rimmele and Boos, 1994), and trehalose-6-phosphate is then hydrolyzed by cytosolic trehalose-6-phosphate hydrolase (encoded by treC) into two glucose molecules, which are used in glycolysis (Rimmele and Boos, 1994). Under hypertonic conditions, however, trehalose is synthesized internally from glucose-6-phosphate and UDP-glucose in two reactions, catalyzed by the osmoregulated-trehalose synthesis (Ots) enzymes trehalose-6-phosphate synthase (encoded by otsA) and trehalose-6-phosphate phosphatase (encoded by otsB) (Giaever et al., 1988). Any trehalose sent to the periplasm is degraded into two glucose molecules by the periplasmic trehalase (encoded by treA), whose activity is also increased under osmotic stress (Boos et al., 1987). The glucose, in turn, is transported back to the cytoplasm by the glucose phosphotransferase system (EIICBGlu) to be a substrate for cytoplasmic trehalose synthesis (Strom and Kaasen, 1993; Li et al., 2012).
In recent years, a relationship between trehalose metabolism and virulence has been demonstrated in a few bacterial pathogens. Collins et al. (2018) reported that Closteroides difficile ribotypes RT027 and RT078 emerged as major epidemic ribotypes shortly after trehalose had been introduced as an additive in industrialized food. The hypothesis raised was that these C. difficile ribotypes emerged as a result of their ability to metabolize low concentrations of trehalose, conferring a competitive advantage over the intestinal microbiota. In Ralstonia solanacearum, biosynthesis of trehalose via the OtsA and OtsB enzymes was proposed to play a fundamental role in successful plant colonization and infection, thus being one of the virulence/fitness mechanisms that contributes to tomato wilt disease (MacIntyre et al., 2020). In Burkholderia pseudomallei, loss of treA increased tolerance to thermal stress but reduced biofilm production and bacterial survival in macrophages (Vanaporn et al., 2017).
In a previous report (Pavanelo et al., 2018), we determined that trehalose metabolism influences virulence of an extraintesinal pathogenic Escherichia coli (ExPEC) strain of avian origin BEN2908 (previously known as MT78). In a screening of BEN2908’s transposon (STM) mutants, we reported that a treA null mutant was reduced in production of type 1 fimbriae, demonstrated decreased adherence and invasion of avian fibroblasts and decreased bladder colonization in the murine model of urinary tract infection (UTI) (Pavanelo et al., 2018). BEN2908ΔtreA also showed increased resistance to osmotic stress caused by urea (but not by NaCl), presumably caused by increased levels of trehalose in the periplasm due to the lack of its periplasmic trehalase (Pavanelo et al., 2018).
In this work, we investigated whether reduced levels of type 1 fimbriae in BEN2908ΔtreA could potentially be due to higher levels of periplasmic trehalose that could have accumulated in the absence of the periplasmic trehalase, TreA. To answer this, we generated a mutant lacking the otsA and otsB genes required for trehalose synthesis (ΔotsBA) as well as a mutant lacking otsA and otsB and the treA gene required for hydrolysis of periplasmic trehalose (ΔtreAotsBA), and analyzed their phenotypes for bacterial growth in the presence of NaCl and urea, production of type 1 fimbriae determined by yeast agglutination, and adhesion and invasion of CEC-32 avian fibroblasts. The trehalose synthesis mutant was also tested in the murine UTI model.
2 Results
2.1 Loss of trehalose synthesis decreased growth of BEN2908 on 0.6 M urea
In E. coli intracellular production of trehalose as an osmoprotectant has been characterized mainly in response to external osmotic strength caused by NaCl. However, the ΔtreA mutant of BEN2908, unable to hydrolyze trehalose in the periplasm, did not show increased osmoprotection against NaCl, but did so against urea (Pavanelo et al., 2018). To verify how the BEN2908 ΔotsBA and ΔtreAotsBA mutants, unable to synthesize trehalose, respond to stress caused by these two molecules, mutants and complemented strains were grown on LB agar containing NaCl or urea, and were compared to growth of the WT and ΔtreA mutant. In the presence of 0.3 M and 0.6 M NaCl or 0.3 M urea (average concentration of urea in human urine) all strains displayed similar growth (Figures 1A–C). However, in the presence of 0.6 M urea, where the growth of the WT was severely impaired and the ΔtreA mutant grew as well as in the absence of urea (as previously reported (Pavanelo et al., 2018), the ΔotsBA was unable to grow (Figure 1D). Trans-complementation of the ΔotsBA mutant restored growth to WT levels on medium containing 0.6 M urea. Growth of the ΔtreAotsBA mutant, lacking all the osmoregulated enzymes of trehalose metabolism, also grew similarly to the WT in the presence of 0.6 M urea.
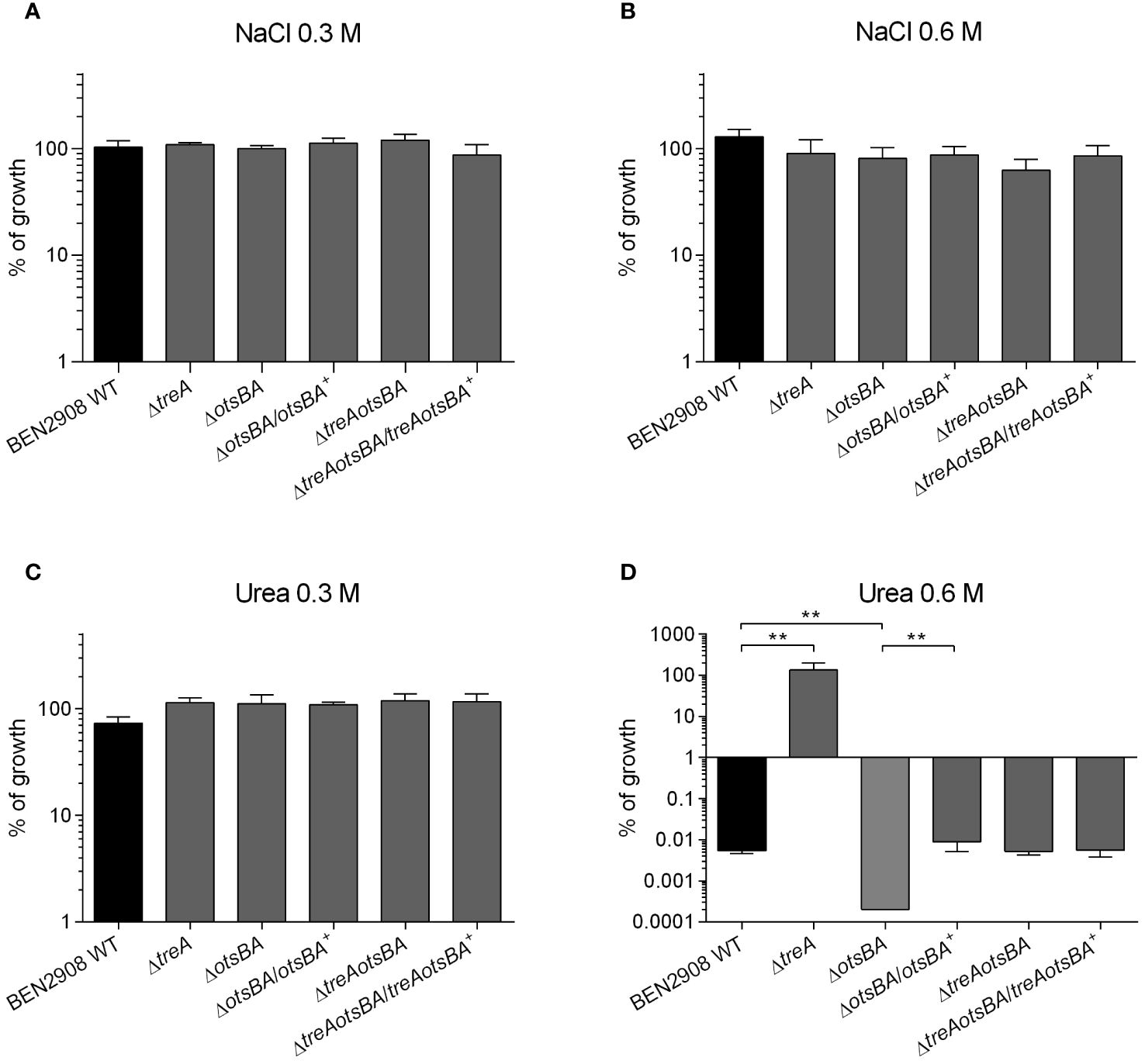
Figure 1 Growth of BEN2908 WT and mutants on LB agar under conditions of osmotic stress. Strains were grown under shaking in LB medium until mid-log phase (OD600 = 0.6) and plated on LB agar (without added NaCl; taken as 100% growth) and on LB agar with 0.3 M of NaCl (A), 0.6 M of NaCl (B), 0.3 M of urea (C), and 0.6 M of urea (D). The ΔotsBA mutant showed no growth on plates with 0.6 M urea (0 CFU), % growth were given a % growth value of 0.0002%. The values are the average growth relative to CFUs on LB agar (without NaCl) ± SEM of three and four experiments (biological replicates) performed in duplicates (technical replicates) for NaCl and urea, respectively. **, P < 0.01 (Mann-Whitney test).
2.2 OtsA, OtsB, and TreA are not required for growth with trehalose as the only carbon source
At low osmolarity, import and catabolism of trehalose by the TreB/TreC pathway are induced in the presence of trehalose in the growth medium, whereas under osmotic stress E. coli accumulates trehalose internally through the osmoregulated OtsA/OtsB/TreA pathway (Strom and Kaasen, 1993). Since the common molecule between these pathways is trehalose-6-phosphate (also an inducer of the trehalose import system) (Klein et al., 1991), we wondered if the absence of the OtsA/OtsB/TreA pathway is required for growth when trehalose is the sole carbon source.
We have previously not observed growth of the ΔtreA mutant in M9 minimal medium with trehalose as the carbon source at 24 h (Pavanelo et al., 2018). Now we tested growth of the ΔtreA, ΔotsBA and ΔtreAotsBA mutants on M9-agar supplemented with 0.6% glycerol or 10 mM trehalose as the only carbon source. All mutants grew as well as the WT with either glycerol or trehalose as the sole carbon source (Figure 2), although at 24 h colonies were too small and were only visible after 48 h of incubation. It is thus unlikely that the absence of the OtsA/OtsB/TreA pathway impairs trehalose assimilation by the TreB/TreC pathway. The slow growth may explain why we did not previously observe growth of the ΔtreA mutant in M9 minimal medium with trehalose as the sole carbon source after 24 h (Pavanelo et al., 2018).
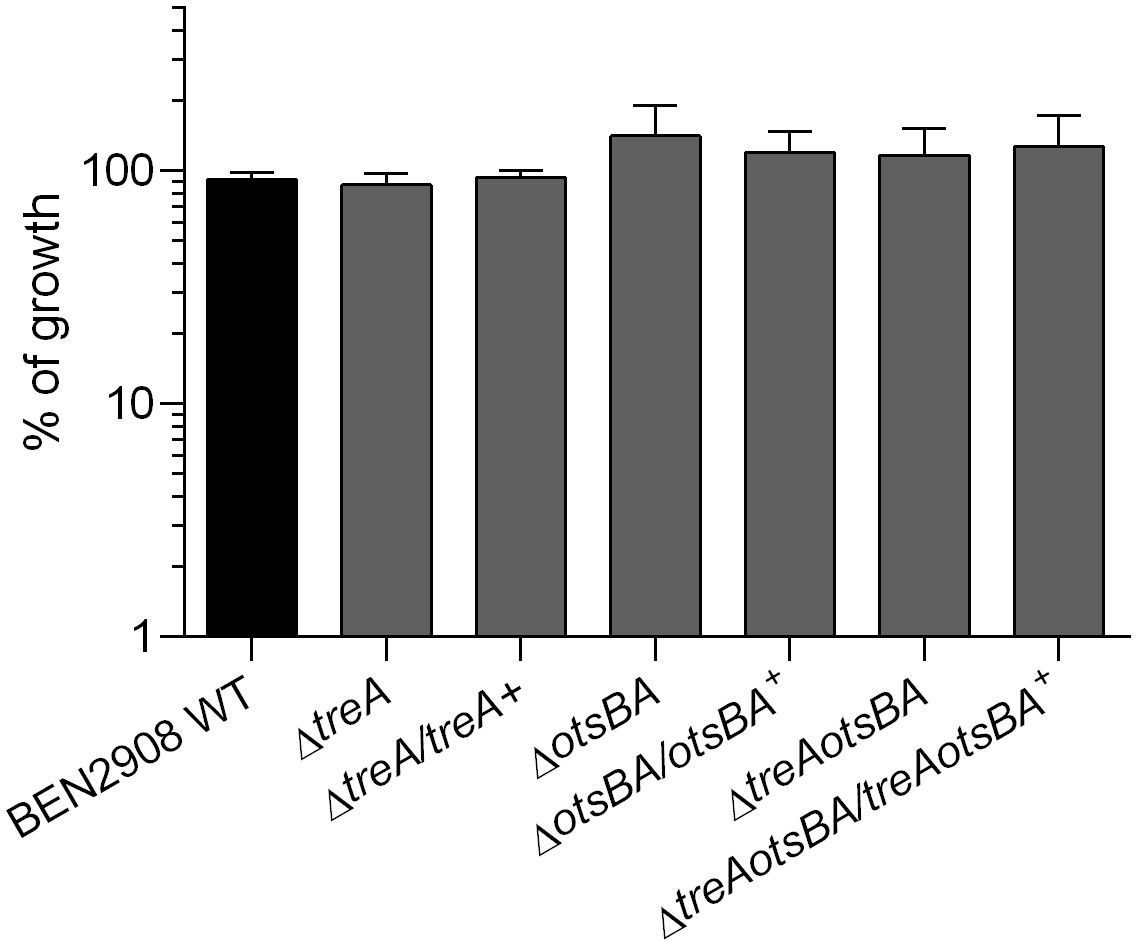
Figure 2 Growth of BEN2908 WT and mutants on M9 agar supplemented with 10 mM trehalose as the sole carbon source. Strains were grown in LB medium until mid-log phase (OD600 = 0.6) and plated on M9 agar with glycerol 0.6% (taken as 100% growth) or with trehalose 10 mM as the sole carbon source. The values are the average growth relative to M9 agar with glycerol 0.6% ± SEM of three experiments performed in duplicates.
2.3 Loss of treA or otsBA reduced yeast agglutination titer
Because the observed decrease in type 1 fimbriae production in BEN2908ΔtreA may result from increased levels of periplasmic trehalose (as suggested by greater resistance to stress by urea in this mutant) (Pavanelo et al., 2018), we wondered whether suppression of trehalose synthesis would have the opposite effect, i.e., would possibly increase type 1 fimbriae production by strain BEN2908. We performed yeast agglutination assays with the WT, and the ΔtreA, ΔotsBA and ΔtreAotsBA mutants, as well as their respective complemented strains. Figure 3A shows that, while the ΔtreA mutant had a two-fold reduction in the production of type 1 fimbriae, the ΔotsBA and ΔtreAotsBA mutants had a four-fold reduction when compared to the WT parent strain. Further, trans-complementation of ΔotsBA mutant restored yeast agglutination titers to WT levels, or higher for complementation of the ΔtreAotsBA mutant. These results suggest that changes in osmoregulation due to altered trehalose metabolism either through loss of trehalose de novo synthesis or lack of degradation in the periplasm may contribute to a decrease in type 1 fimbriae production.
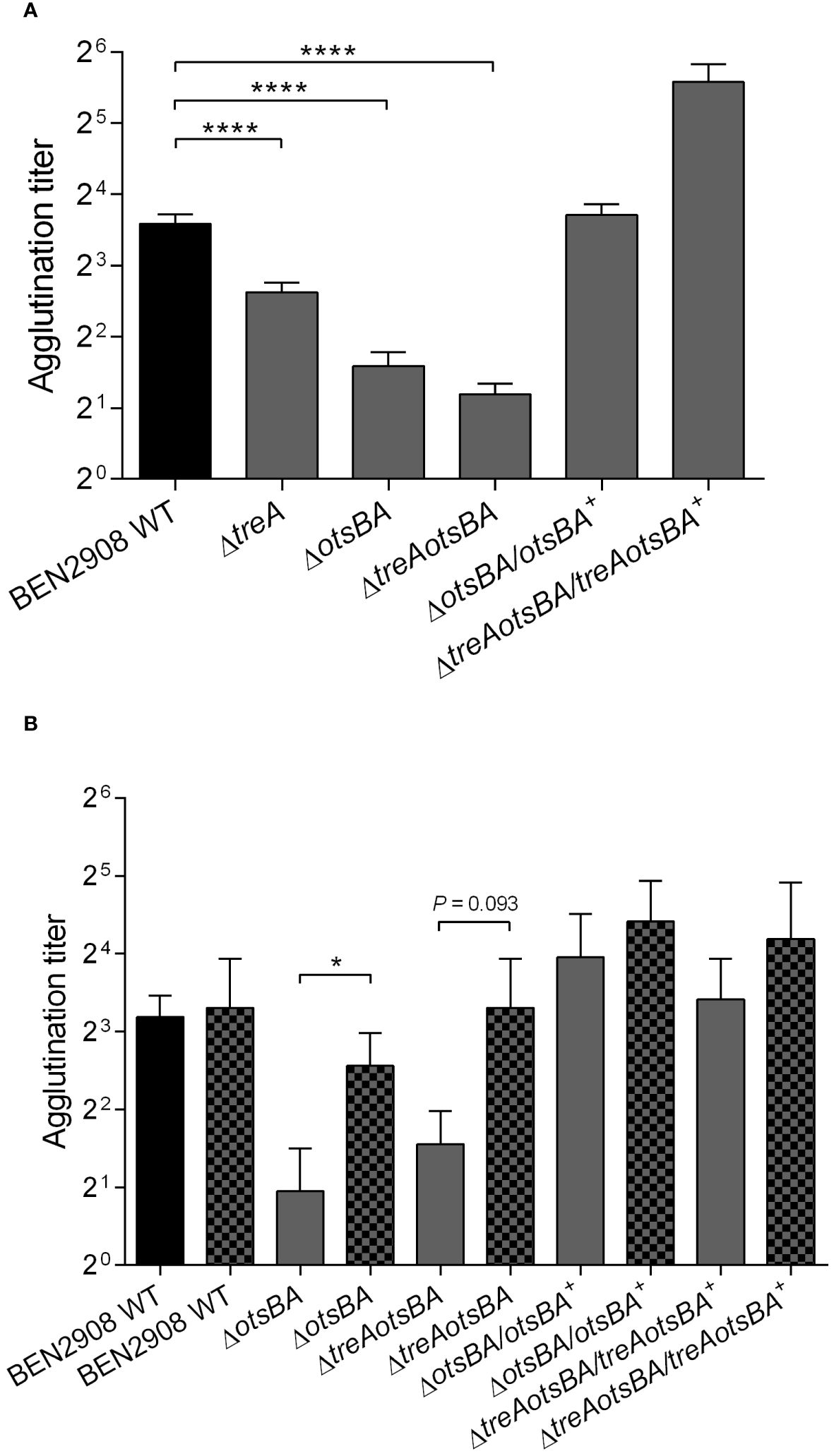
Figure 3 Yeast agglutination of BEN2908 WT and mutants was measured after mid-log growth (OD600nm= 0.6) with shaking in LB broth (A) and in LB broth without NaCl (B) in the absence (left bar of each pair) and presence of 0.3 M urea (right, patterned bar of each pair),. The values are the average ± SEM of eleven and three experiments done in duplicates for A and B, respectively. ****P < 0.0001 (Mann-Whitney test) in (A); * P < 0.05 (Unpaired t test) in (B).
2.4 Growth with 0.3 M urea increased type 1 fimbriae production by the ΔotsBA mutant
The absence of the enzymes for de novo synthesis of trehalose (otsA and otsB) rendered strain BEN2908 more sensitive to 0.6 M urea (Figure 1D), indicating that synthesis of trehalose provided osmoprotection against urea, in line with our previous observations (Pavanelo et al., 2018). On the other hand, 0.6 M urea upregulated expression of fim genes in the UPEC strain CFT073 (Withman et al., 2013). Since optimal production of type 1 fimbriae required the osmoregulated synthetic enzymes of trehalose (Figure 3A), we wondered if urea could trigger type 1 fimbriae production through increased synthesis of trehalose by OtsA and OtsB.
To verify this hypothesis, strains were grown in 0.3 M urea and tested for production of type 1 fimbriae by yeast agglutination (Figure 3B). The otsAB mutant displayed a significantly higher agglutination titer when grown in the presence of 0.3 M urea, indicating that urea-mediated upregulation of type 1 fimbriae production is independent of metabolism of trehalose.
2.5 Swimming motility of WT and mutants
A decrease in type 1 fimbriae expression in E. coli may also result in an increase in flagella-mediated motility (Lane et al., 2007; Spurbeck and Mobley, 2013). To determine if this occurs in BEN2908, we tested the swimming motility of WT and mutant strains (Figure 4). Despite the absence of statistical significance, there was an observed increase in motility for the otsAB mutant, to levels similar to the fim null mutant DM34. The complemented strain rescued the WT phenotype.
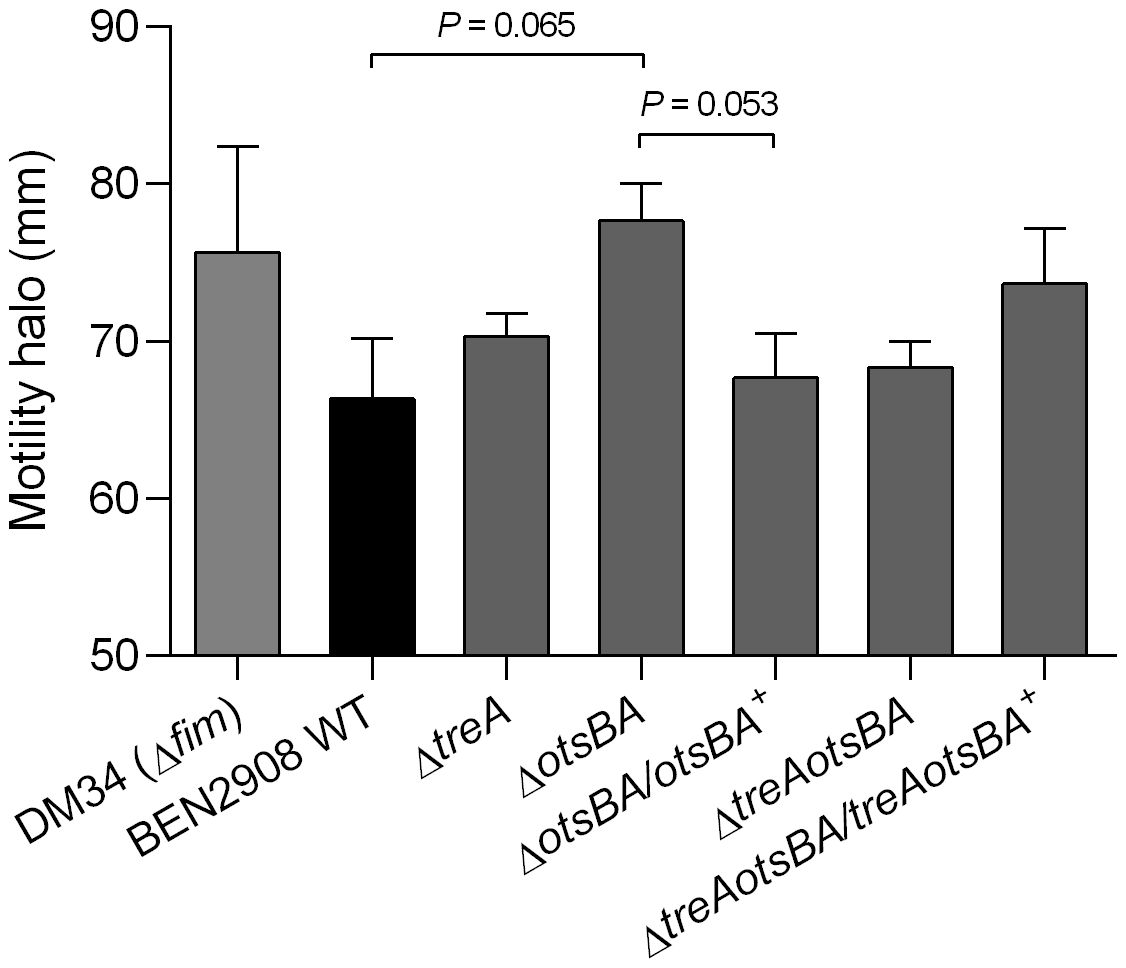
Figure 4 Diameters of swimming motility of BEN2908, mutants and complemented mutants. DM34 strain, a fim null mutant of BEN2908, was used as a positive control. The swimming motility was verified on soft agar (1% tryptone, 0.5% NaCl, 0.3% agar). Strains were stabbed onto the surface of soft agar and incubated at 37° C for 16 hours. The values represent the average ± SEM of three experiments done in duplicates. Significant differences in motility were analyzed using unpaired t-test.
2.6 Adherence to avian fibroblasts was decreased for ΔotsBA and ΔtreAotsBA mutants
E. coli type 1 fimbriae contribute to virulence and play a crucial role in bacterial adhesion (Kaper et al., 2004; Mobley et al., 2013). Since adhesion to and invasion of CEC-32 fibroblasts are dependent on type 1 fimbriae production, we wondered if BEN2908ΔotsBA and BEN2908ΔtreAotsBA mutants, that produce less type 1 fimbriae than WT, would be impaired in their adherent and invasive capacities. While 27% of the initial inoculum of the WT strain adhered to avian fibroblasts after 1 hour of interaction, the ΔotsBA and ΔtreAotsBA mutants adhered significantly less (P < 0.05) with respectively only 14% and 13% adherence of the initial inoculum (Figure 5A). There was also a reduction in the invasion levels of the mutants compared to WT, although this was not statistically significant (Figure 5B).
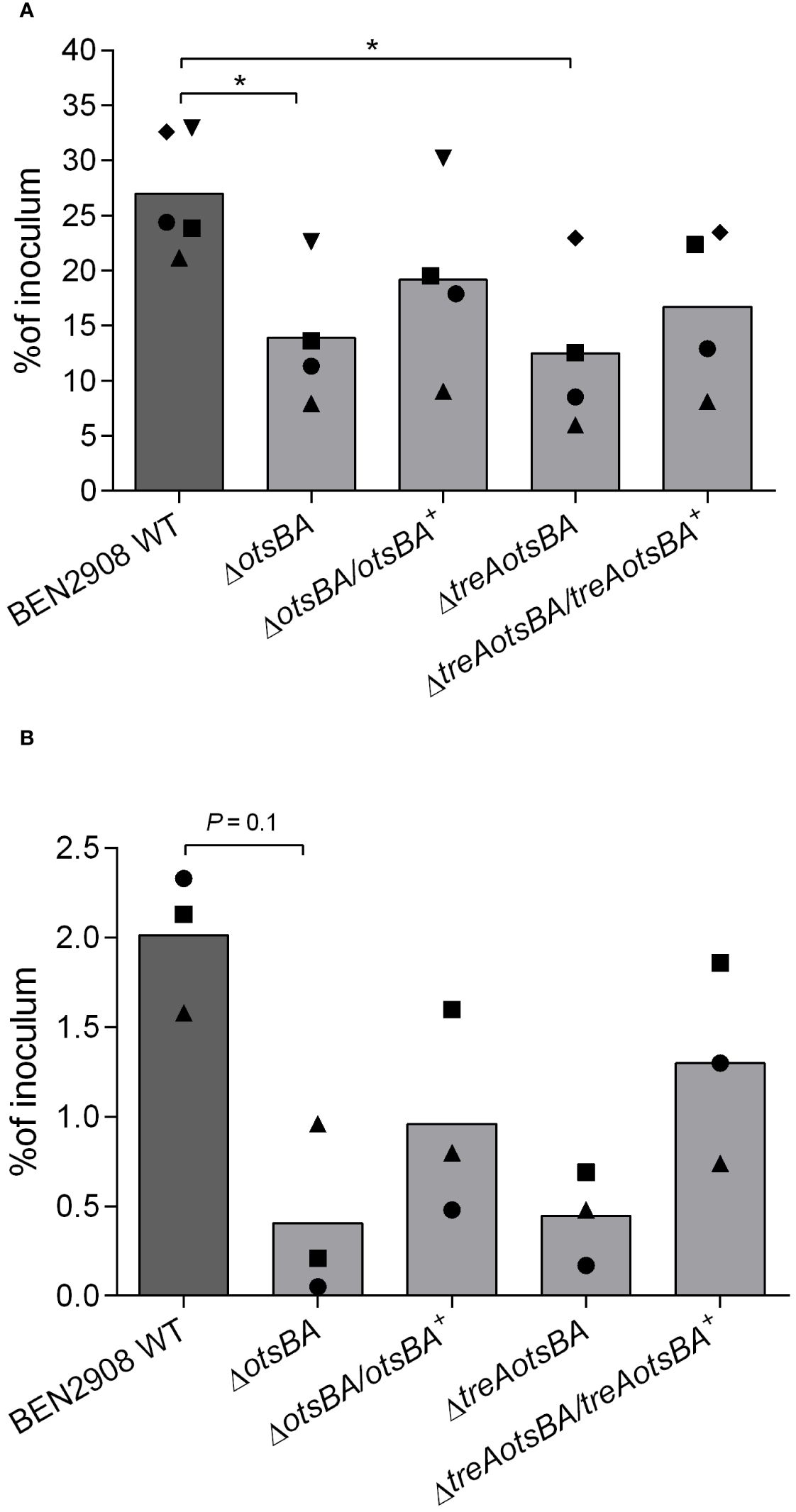
Figure 5 Adhesion to and invasion of CEC-32 avian fibroblasts. Cells were infected at an MOI of 20 CFU/cell. Bars represent the median of recovered CFU compared to that of inoculum 1 h after infection (adhesion) (A) and 4 h after infection, the first hour without gentamicin, followed by washes and reincubation in the presence of gentamicin at 50 µg/mL for a further 3 h (invasion) (B). Data are from four and three experiments done in triplicates, respectively. Same symbols represent data from the same experiment. *P < 0.05 (Mann-Whitney test).
2.7 The ΔotsBA mutant was less able to colonize the bladder during murine urinary tract infection
Type 1 fimbriae are important for adherence and colonization of E. coli in the urinary tract. BEN2908 WT strain is able to cause urinary tract infection in a murine model, and BEN2908ΔtreA presents a reduced ability to colonize the murine bladder (Pavanelo et al., 2018). We therefore tested whether the decrease in type 1 fimbriae production observed in vitro would also reduce bladder or kidney colonization in a murine model of urinary tract infection. Indeed, the otsBA mutant colonized the murine bladder 10-fold less than the WT (P < 0.05), whereas the complemented mutant colonized the bladder at levels similar to the WT (Figure 6). There was no significant difference in colonization of the kidney between the mutant and the WT strain.
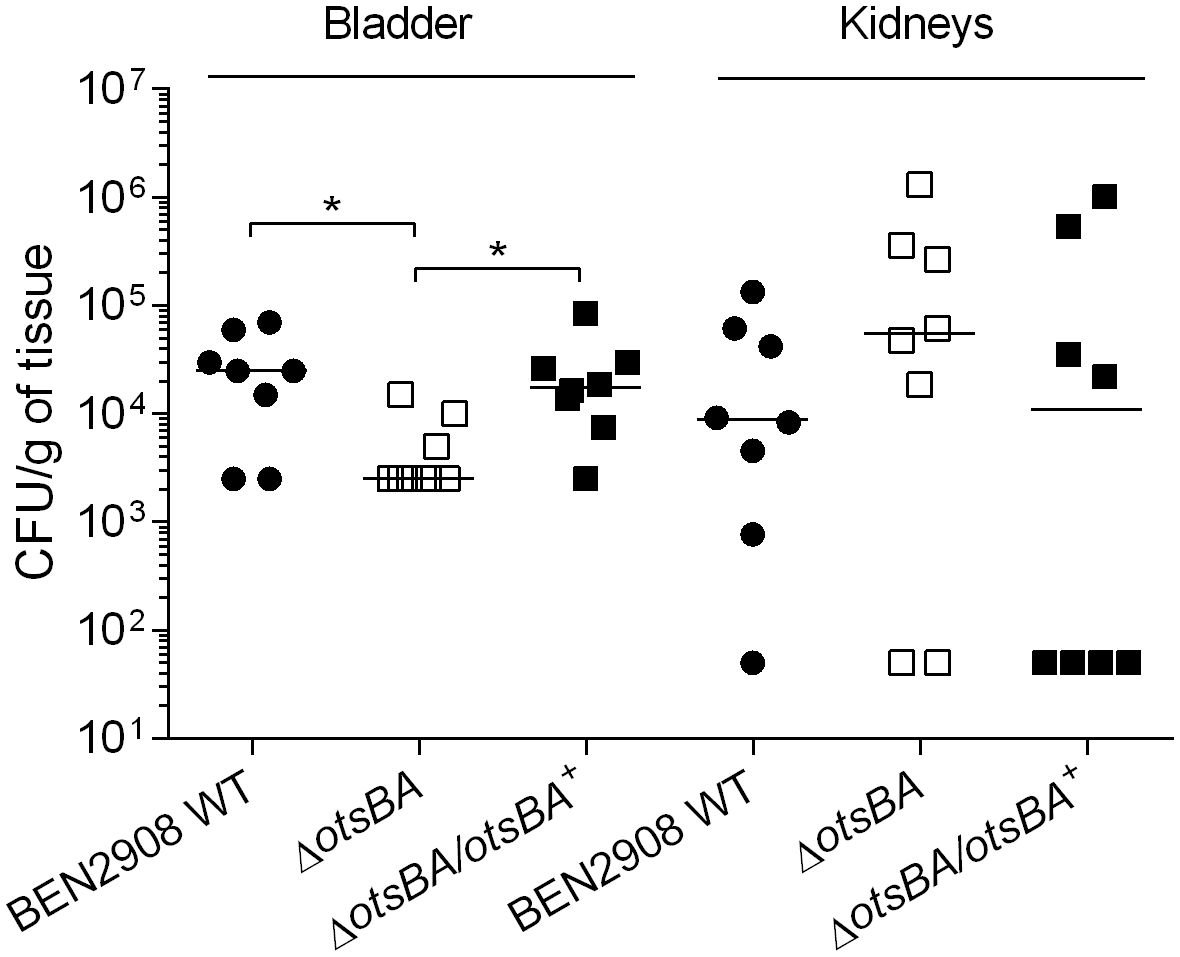
Figure 6 Loss of trehalose synthesis reduces bladder colonization during urinary tract infection in mice. CBJ/A mice were infected transurethrally, the animals were euthanized, and bladder and kidneys were collected 48 h post-infection. For bladders, each data point represents bacterial numbers recovered from the bladder of an individual mouse. For kidneys, each data point represents the mean of both right and left kidneys from the same mouse. Horizontal bars indicate the medians. *P < 0.05 (Mann-Whitney test).
3 Discussion
To resist osmotic stress, bacteria produce osmolytes to counteract the high external osmolarity and avoid fluid loss, thus maintaining the turgor by reducing water activity (Ruhal et al., 2013). In E. coli, the osmoprotectant trehalose has been shown to accumulate in cells in response to osmotic stress by monovalent cations (Strom and Kaasen, 1993). The increased levels of intracellular trehalose in response to NaCl are a consequence of increased activities of the cytoplasmic synthetic enzymes OtsA and OtsB (Boos et al., 1987; Giaever et al., 1988) and of the periplasmic trehalase, TreA (Boos et al., 1990). A 2-fold increase in expression of otsA and otsB in the presence of NaCl (but not in urea) has been observed in uropathogenic E. coli strain CFT073 (Withman et al., 2013). E. coli mutants unable to synthesize trehalose have impaired growth under osmotic stress caused by NaCl (Giaever et al., 1988). Contrary to what has been observed for E. coli K-12, deletion of the osmoregulated enzymes of trehalose metabolism had no effect on growth of avian pathogenic E. coli strain BEN2908 when osmolarity was increased with NaCl (Figure 1A, B).
We have previously shown that deletion of treA in strain BEN2908 resulted, on one hand, in increased resistance to osmotic stress caused by the permeant osmolyte urea, and, on the other hand, in decreased production of type 1 fimbriae. This strain consequently was less able to adhere to and invade avian fibroblasts and had decreased bladder colonization in the murine urinary tract (Pavanelo et al., 2018). As the ΔtreA mutant most likely accumulates trehalose in the periplasm, we wondered whether decreasing the endogenous levels of trehalose by deleting the cytosolic enzymes of trehalose synthesis would potentially cause reduced osmotic resistance and enhanced type 1 fimbriae production. In contrast to some other bacterial species such as Pseudomonas or Ralstonia (Freeman et al., 2010; Djonović et al., 2013; MacIntyre et al., 2020), synthesis of trehalose in E. coli is completely dependent on OtsA and OtsB enzymes, and deletion of otsAB abrogates trehalose synthesis (Giaever et al., 1988).
We first tested the resistance of WT and null mutants to osmotic stress induced by urea. While the ΔtreA mutant grew better than the WT parent in minimal medium in the presence of 0.6 M urea, in agreement with our previous results (Pavanelo et al., 2018), ΔotsBA, a mutant that is unable to synthesize trehalose, grew less than the WT under the same conditions (Figure 1D). The growth defect of the ΔotsBA mutant in urea was alleviated by additional loss of treA encoding the periplasmic trehalase TreA (ΔtreAotsBA, Figure 1D). This result suggests that loss of all osmoregulated enzymes involved in trehalose metabolism likely results in increased production of other osmoprotectants, such as betaines, in response to osmotic stress caused by urea (Strom and Kaasen, 1993; Randall et al., 1996).
In E. coli, periplasmic trehalose can have two distinct fates. Under hypertonic conditions, trehalose is hydrolyzed by TreA, and the resulting glucose molecules can be internalized and serve as the precursors for cytoplasmic trehalose synthesis (by OtsA and OtsB). Under isotonic conditions, trehalose is transported to the cytoplasm via a trehalose-specific PTS system (TreB); trehalose-6-phosphate can then be hydrolyzed by the cytosolic trehalose-6-phosphate hydrolase (TreC) to glucose and glucose-6-P, which can be substrates in the glycolysis pathway. BEN2908ΔotsBA, ΔtreAotsBA and ΔtreA mutants were able to grow on minimal medium with trehalose as the only carbon source (Figure 2), indicating that the genes responsible for metabolism of trehalose in high osmotic conditions were not required for utilization of trehalose as a carbon source when E. coli is grown under low osmotic conditions, as reported previously (Boos et al., 1990).
The treA mutant of BEN2908 produced less type 1 fimbriae, which likely resulted in reduced invasion of avian fibroblasts and reduced bladder colonization of the murine urinary tract (Pavanelo et al., 2018). In urinary tract infections, bladder colonization by uropathogenic E. coli (UPEC) depends on the binding of type 1 fimbriae to host cell uroplakin, which can promote bacterial internalization (Mulvey et al., 1998). Without type 1 fimbriae, E. coli is less able to establish urinary tract colonization. Notably, fimA (encoding the major subunit of type 1 fimbriae) is the fourth most expressed protein in UPEC strain CTF073 following infection in mice, only behind three proteins involved in protein synthesis machinery (Snyder et al., 2004). Moreover, when strain CFT073 was grown in the presence of 0.6 M urea (but not when supplemented with 0.3 M NaCl), expression of fim genes was upregulated ~4-fold (Withman et al., 2013). Neonatal meningitis-causing E. coli is thought to require type 1 fimbriae to adhere to human brain microendothelial cells and may promote traversal of the blood-brain barrier (Kim, 2008). Type 1 fimbriae may also mediate adhesion of avian pathogenic E. coli to avian cells and tissues, and several attenuated APEC mutants were also reduced in their levels of production of type 1 fimbriae (Cortes et al., 2008; De Pace et al., 2010; Verma et al., 2015). Since a decrease in production of type 1 fimbriae in BEN2908ΔtreA could be caused by accumulation of periplasmic trehalose, we investigated whether loss of endogenous trehalose synthesis in the ΔotsBA and ΔtreAotsBA mutants would alter levels of type 1 fimbriae production. Yeast agglutination assays revealed that both of these mutants actually produced even less type 1 fimbriae than the treA mutant (Figure 3A). The effects of urea-mediated osmotic stress were not as adverse on the ΔtreA mutant which showed greater resistance compared to WT, whereas the trehalose synthesis mutant ΔotsBA was more sensitive to urea-mediated stress. However, all trehalose mutants had decreased levels of production of type 1 fimbriae. Our hypothesis to explain this apparent paradox is that, in BEN2908, resistance to stress by urea would depend on the levels of periplasmic trehalose, but type 1 fimbriae production would rather be influenced by the levels of cytosolic trehalose. We hypothesize that the inability of BEN2908ΔtreA to hydrolyze periplasmic trehalose would decrease the quantity of glucose entering the cytoplasm (through EIICBGlu), therefore lowering the rate of trehalose synthesis by OtsA-OtsB. The decrease in trehalose synthesis would not be as pronounced in ΔtreA mutant compared to the ΔotsBA and ΔtreAotsBA mutants, as reflected in the yeast agglutination assays. Li et al. (2012) previously reported that TreA activity was related to activities of OtsA-OtsB rather than directly to osmotic stress. If this interpretation proves correct, basal levels of cytosolic trehalose would be required for optimal production of type 1 fimbriae in ExPEC BEN2908; the mechanism of this regulation, however, remains to be elucidated. A diagram illustrating our hypothesis for the observed phenotypes of ΔtreA and ΔotsBA mutants is shown in Figure 7.
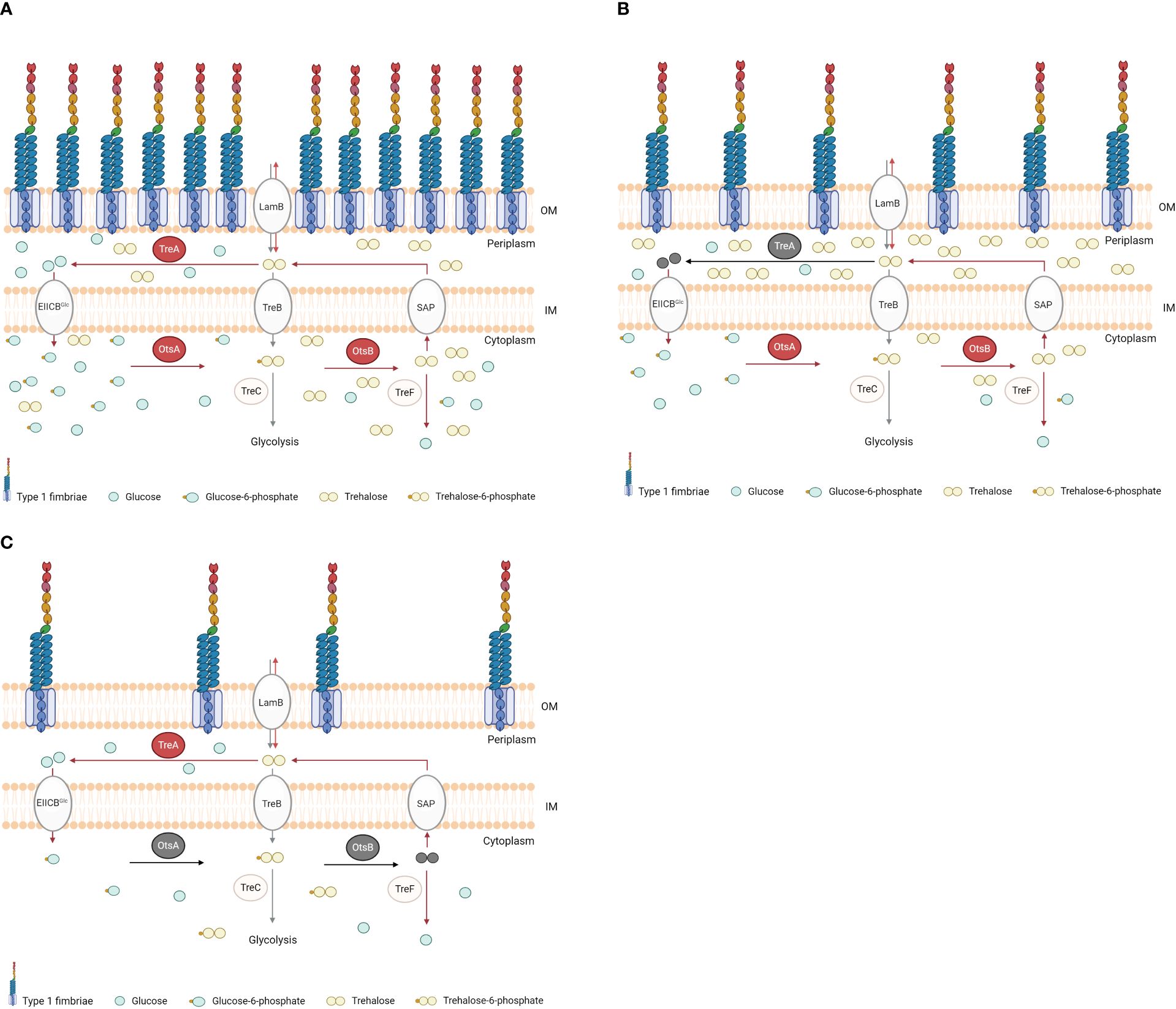
Figure 7 Diagram illustrating our hypothesis for the periplasmic and cytosolic concentrations of trehalose in BEN2908 wild type and in ΔtreA and ΔotsBA mutants. The respective phenotypes of type 1 fimbriae are shown. In WT (A), the osmoregulated-trehalose synthesis (Ots) enzymes trehalose-6-phosphate synthase (OtsA) and trehalose-6-phosphate phosphatase (OtsB), and the periplasmic trehalase TreA, whose activities are increased under osmotic stress (as indicated by arrows in red), keep basal concentrations of trehalose in cytosol and periplasm. In the ΔtreA mutant (B, deletion indicated in grey), absence of trehalose hydrolysis results in increased concentrations of periplasmic trehalose, thus protecting against extracellular urea, while lowering the quantity of glucose available to enter the cytosol (through EIICBGlu) and serve as substrate for OtsA-OtsB to synthesize cytosolic, endogenous trehalose. As consequence, levels of cytosolic trehalose are reduced in the ΔtreA mutant. In the ΔotsBA mutant (C, deletions indicated in grey), absence of trehalose synthesis in the cytosol lowers trehalose concentrations both in cytosol and periplasm, rendering the mutant more susceptible than WT to urea. Any trehalose in the periplasm would be of exogenous source. The defect in type 1 fimbriae production observed in the ΔtreA and ΔotsBA mutants could be explained by lower levels of cytosolic trehalose, through an as yet unknown mechanism. OM, outer membrane; IM, inner membrane; LamB, sugar transporter; EIIAGlc, glucose phosphotransferase system; TreB, trehalose-specific PTS system; SAP, stretch-activated protein; TreC, trehalose-6-phosphate hydrolase; TreF, cytoplasmic trehalase.
Human urine has a high osmolality (average 500–600 mOsm/kg) and consists mainly of urea and some inorganic ions (mainly NaCl; (Ipe and Ulett, 2016)). Along with the osmoprotectants glycine-betaine and proline, trehalose is produced by E. coli to resist osmotic stress (Ruhal et al., 2013). In E. coli, trehalose as an osmoprotectant has been mainly investigated in response to stress by NaCl, whose effects on trehalose internal concentrations are a consequence of increased activities of OtsA and OtsB (Giaever et al., 1988) and increased expression of otsA and otsB (Withman et al., 2013). On the other hand, even though trehalose levels in bacterial cells growing in the presence of urea were not determined, increased expression of otsA and otsB was not detected in these conditions (Withman et al., 2013). Needless to say, however, the activity of an enzyme is subjected to regulation by other mechanisms (e.g., substrate concentrations, allosteric regulation) than only expression. Yet, according to our data, increased levels of periplasmic trehalose protected BEN2908 against urea (Figure 1; (Pavanelo et al., 2018).
Type 1 fimbriae expression is enhanced when UPEC is grown in urine (Snyder et al., 2004), and the component that would cause this enhancement is likely to be urea (Withman et al., 2013). As with E. coli, Klebsiella pneumoniae also has increased production of type 1 fimbriae when grown with 0.6 M urea (Lin et al., 2022). We also investigated whether trehalose synthesis by OtsA and OtsB were the mechanism by which urea stimulates type 1 fimbriae expression. However, in the ΔotsBA mutant which cannot produce endogenous trehalose, type 1 fimbriae production was also increased in the presence of 0.3 M urea (the average urea concentration in human urine) (Figure 3B). Thus, the mechanism by which urea acts on type 1 fimbriae production seems to be independent of trehalose levels in the bacterial cell.
Since production of type 1 fimbriae is energetically costly, it needs to be tightly regulated in E. coli (Blomfield and van der Woude, 2007). There are several mechanisms that control type 1 fimbriae, such as global regulators (H-NS, LRP, FNR) (Corcoran and Dorman, 2009; Barbieri et al., 2017), ibeA and ibeT genes and the phosphate (Pho) regulon (pst) (Cortes et al., 2008; Crépin et al., 2012b). More recently, several studies have shown that sugar metabolism plays a role in virulence of many gram-negative bacteria, such as Ralstonia solanecearum and Burkholderia pseudomallei (Vanaporn et al., 2017; MacIntyre et al., 2020). Based on that, it would not be surprising that some metabolites or osmolytes act as regulators of type 1 fimbriae. Here, we have shown that the removal of the genes responsible for metabolism of trehalose under high osmolality in BEN2908 resulted in decreased type 1 fimbriae production, as observed in reduced yeast agglutination, reduced capacity of adhesion to avian fibroblasts in vitro and reduced capacity of bladder colonization in a murine UTI model (Figures 3, 6, 7).
In summary, we generated BEN2908ΔotsBA, BEN2908ΔtreAotsBA and the respective complemented mutants to better understand the relationship between trehalose metabolism and virulence of ExPEC. We have also shown that the loss of trehalose synthesis further impaired bladder colonization in a murine urinary tract infection model. Our results suggest that strain BEN2908 requires the osmoregulated enzymes of trehalose metabolism to optimally produce type 1 fimbriae, which is a key ExPEC virulence factor.
4 Materials and methods
4.1 Bacterial strains, plasmids, and growth conditions
All strains and plasmids are listed in Table 1. Strains and mutants were grown in Luria Bertani (LB) medium, LB with different amounts of NaCl or urea, or in M9 minimal medium containing 10 mM trehalose or 0.6% glycerol as the only carbon source. The following antibiotics were added when necessary: gentamicin 15 µg/µL, chloramphenicol 30 µg/µL, kanamycin 50 µg/µL or ampicillin 100 µg/µL. Before electroporation with pGpTn7-Gm plasmid, diaminopimelic acid (DAP)-negative MG617 strain was grown in the presence of DAP 50 µg/µL. Bacterial strains were stored in LB broth containing 20% glycerol at -80°C.
4.2 Construction of specific mutants and complemented strains
All mutants were generated by the lambda-Red recombinase technique (Datsenko and Wanner, 2000). Genes were disrupted by inserting a resistance gene cassette, using pDK3 or pKD4 plasmids as templates for chloramphenicol and kanamycin resistance, respectively. To eliminate any potential polar effects, the cassettes, flanked by FRT sites, were eliminated using the Flp resolvase expressed from plasmid pCP20 (Crépin et al., 2012a).
Chromosomal complementation was achieved by insertion of genes at the attTn7 site on the bacterial chromosome as previously described (Crépin et al., 2012a). Briefly, the otsBA operon and its promoter region from E. coli BEN2908 were cloned into pGpTn7-Gm in E. coli strain DH5α pir, generating pGpTn7-Gm-otsBA. The treA gene and its promoter were then amplified from genomic DNA of BEN2908 and cloned into the pGpTn7-Gm-otsBA plasmid in E. coli strain DH5α pir, generating pGpTn7-Gm-treAotsBA. The ΔotsBA and ΔtreAotsBA strains were electroporated with plasmid pSTNSK and grown at 30 °C. The generated ΔotsBA + pSTNSK and ΔtreAotsBA + pSTNSK strains were mated with donor strain MGN-617 pGpTn7Gm+treAotsAB, generating the complemented strains ΔotsBA/treAotsBA+ and ΔtreAotsBA/treAotsBA+. The complementations were achieved through single-copy integration in trans at the attTn7 site, upstream the glmS gene. Since the ΔotsBA/treAotsBA+ strain contained two copies of treA, the treA gene and Gm resistance cassette at the attTn7 site were replaced with a Km cassette, generating the complemented ΔotsBA/otsBA+ strain (Datsenko and Wanner, 2000). All primers used are listed in Table 2.
4.3 Yeast agglutination assay
The strains were inoculated from an overnight pre-inoculum in LB medium and grown in LB broth at 37° C under shaking (240 rpm) until mid-log phase (OD600 = 0.6). A suspension of 2 x 109 bacterial cells was serially diluted 1:2 in phosphate buffered saline (PBS) in a microtiter plate. A 1.5% suspension of commercially available yeast was added to each well. After 30 minutes incubation on ice, agglutination was visually monitored and the agglutination titer was determined as the most diluted well wherein agglutination was observed. In order to verify the influence of urea on production of type 1 fimbriae, yeast agglutination assays were performed as above, except that strains were grown in LB broth without NaCl in the absence (control) or presence of 0.3 M urea.
4.4 Growth in conditions of osmotic stress
Strains were tested for the capacity to grow under conditions of osmotic stress caused by NaCl or urea. Strains were inoculated 1:100 from an overnight pre-inoculum in LB broth and grown until mid-log phase (OD600 = 0.6) under shaking (240 rpm) at 37° C. They were serially diluted and plated on LB agar without NaCl or on LB agar plates with 0.3 M NaCl, 0.6 M NaCl, 0.3 M urea or 0.6 M urea. After incubation at 37 °C for 24 h, colonies were counted and growth under each condition was compared to growth on LB agar without NaCl.
4.5 Growth on minimal medium with trehalose as the only carbon source
To verify if the mutants retained their ability to use trehalose as a sole carbon source, BEN2908 and mutants were grown in M9 medium plates (60 g/L Na2HPO4, 30 g/L KH2PO4, 5 g/L NaCl, 10 g/L NH4Cl; 0.5 mL of MgSO4.7H2O 1 M, 0.5 mL of CaCl2 0.1 M and 1 mL of thiamine 0.5 M; 1.5% agar) with 0.6% glycerol or 10 mM trehalose as the only carbon source. Strains were inoculated 1:100 from an overnight preinoculum in LB broth and grown until mid-log phase (OD600 = 0.6) under shaking at 37 °C. All of them were serially diluted and plated on M9 agar with 0.6% glycerol or 10 mM trehalose. After incubation at 37 °C for 48 h, colonies were counted and growth on trehalose was compared to growth on glycerol.
4.6 Swimming motility assay
Swimming motility was done as previously described (Verma et al., 2015). An overnight culture of each strain was diluted 1:100 in LB broth and grown at 37° C under shaking until mid-log phase (OD600 = 0.6). Cultures of each strain were stabbed into the surface of LB 0.3% (soft) agar using an inoculating needle and care was taken not to touch the bottom of the plate during inoculation to ensure only swimming motility was assessed. The plates were incubated face up at 37° C for 16 hours. Three independent experiments were performed for each strain.
4.7 Cell adhesion and invasion assays
Bacteria-eukaryotic cell association assays were performed with CEC-32 avian fibroblasts as described previously (Pavanelo et al., 2018). Briefly, 5 x 104 cells/well were distributed in 96-well plates in DMEM high glucose with 10% fetal bovine serum. On the following day, cultures were washed once with PBS to remove dead cells and returned to the culture medium. Bacterial strains were inoculated from an overnight pre-inoculum in LB medium and grown at 37° C under shaking (240 rpm) until mid-log phase (OD600 = 0.6). Cells were infected at a multiplicity of infection (MOI) of 20 bacteria per cell. After 1 h of incubation at 37°C and 5% of CO2, the medium was removed, and the cells were washed 3 times with PBS. For the adhesion assays, cells were then lysed with Triton X-100 1% (v/v) in PBS for 5 min. Lysates were serially diluted and plated on LB agar for CFU counting. For the invasion assays, cells were further incubated in culture medium supplemented with 50 µg/ml gentamicin for 3 h, after which they were lysed, and lysates diluted and plated.
4.8 Experimental urinary tract infection
Experimental urinary tract infections were performed as previously described (Hagberg et al., 1983). Five-week-old-female CBA/J mice were infected with 20 µL (2 x 109 CFU) using a catheter to insert the bacterial suspension through the urethra. At 48 hours post-infection, mice were euthanized and the bladder and both kidneys were collected, homogenized in PBS, serially diluted and plated onto MacConkey-agar; plates were incubated at 37° C for 16 hours for colony counting.
4.9 Statistical analysis
The Mann-Whitney test was used to compare the samples by pairs, and Kruskal Wallis was used to compare groups, since distribution of results was nonparametric. Tests were performed using GraphPad Prism version 6.00 for Windows, GraphPad Software, La Jolla California USA, www.graphpad.com.
4.10 Animal ethics statement
The protocol for mice urinary tract infection was approved by the animal ethics evaluation committee – Comité Institutionel de Protection des Animaux (CIPA No. 1608–02) of the INRS-Centre Armand-Frappier.
Data availability statement
The original contributions presented in the study are included in the article/supplementary material. Further inquiries can be directed to the corresponding author.
Ethics statement
The animal study was approved by Comité Institutionel de Protection des Animaux (CIPA No. 1608–02) of the INRS-Centre Armand-Frappier. The study was conducted in accordance with the local legislation and institutional requirements.
Author contributions
VK: Data curation, Formal analysis, Investigation, Methodology, Writing – original draft, Writing – review & editing. DP: Conceptualization, Formal analysis, Methodology, Supervision, Writing – review & editing, Writing – original draft. SH: Data curation, Investigation, Methodology, Writing – review & editing. SD: Investigation, Methodology, Writing – review & editing. PP: Investigation, Methodology, Writing – review & editing. SI-J: Investigation, Methodology, Writing – review & editing. CD: Conceptualization, Formal analysis, Funding acquisition, Project administration, Supervision, Writing – review & editing. FH: Conceptualization, Formal analysis, Funding acquisition, Investigation, Methodology, Project administration, Supervision, Writing – review & editing, Writing – original draft.
Funding
The author(s) declare financial support was received for the research, authorship, and/or publication of this article. This work was supported by CAPES, Ministry of Education, (PVE A093/2013, 23038.009613/2013-11) and CNPq (Projeto Universal 423.902/2016-4), Brazil (FH), and Natural Science and Engineering Research Council of Canada (NSERC) (https://www.nserc-crsng.gc.ca) (Discovery Program, project 2019-06642) and CRIPA-Programme des Regroupements stratégiques du Fonds de recherche du Québec - Nature et technologies (FRQNT) (https://www.cripa.center/home-1) 2020-RS4-265142 (CD). VK was the recipient of a CAPES Ph.D. studentship (DS 88882.439720/2018-01 and PDSE-PPGMAA 88881.188644/2018-01) and travel grant from FRQNT-CRIPA.
Acknowledgments
We warmly thank Dr. Catherine Schouler (INRAe, Nouzilly, France) for the gift of strain DM34.
Conflict of interest
The authors declare that the research was conducted in the absence of any commercial or financial relationships that could be construed as a potential conflict of interest.
The author(s) declared that they were an editorial board member of Frontiers, at the time of submission. This had no impact on the peer review process and the final decision.
Publisher’s note
All claims expressed in this article are solely those of the authors and do not necessarily represent those of their affiliated organizations, or those of the publisher, the editors and the reviewers. Any product that may be evaluated in this article, or claim that may be made by its manufacturer, is not guaranteed or endorsed by the publisher.
References
Argüelles, J. C. (2014). Why can’t vertebrates synthesize trehalose? J. Mol. Evol. 79, 111–116. doi: 10.1007/s00239-014-9645-9
Barbieri, N. L., Vande Vorde, J. A., Baker, A. R., Horn, F., Li, G., Logue, C. M., et al. (2017). FNR regulates the expression of important virulence factors contributing to the pathogenicity of Avian Pathogenic Escherichia coli. Front. Cell. Infect. Microbiol. 7. doi: 10.3389/fcimb.2017.00265
Blomfield, I., van der Woude, M. (2007). Regulation of fimbrial expression. EcoSal Plus 2, 1–30. doi: 10.1128/ecosal.2.4.2.2
Boos, W., Ehmann, U., Bremer, E., Middendorf, A., Postma, P. (1987). Trehalase of Escherichia coli: Mapping and cloning of its structural gene and identification of the enzyme as a periplasmic protein induced under high osmolarity growth conditions. J. Biol. Chem. 262, 13212–13218. doi: 10.1016/S0021-9258(18)45189-7
Boos, W., Ehmann, U., Forkl, H., Klein, W., Rimmele, M., Postma, P. (1990). Trehalose transport and metabolism in Escherichia coli. J. Bacteriol. 172, 3450–3461. doi: 10.1128/jb.172.6.3450-3461.1990
Collins, J., Robinson, C., Danhof, H., Knetsch, C. W., Van Leeuwen, H. C., Lawley, T. D., et al. (2018). Dietary trehalose enhances virulence of epidemic Clostridium difficile. Nature 553, 291–294. doi: 10.1038/nature25178
Corcoran, C. P., Dorman, C. J. (2009). DNA relaxation-dependent phase biasing of the fim genetic switch in Escherichia coli depends on the interplay of H-NS, IHF and LRP. Mol. Microbiol. 74, 1071–1082. doi: 10.1111/j.1365-2958.2009.06919.x
Cortes, M. A. M., Gibon, J., Chanteloup, N. K., Moulin-Schouleur, M., Gilot, P., Germon, P. (2008). Inactivation of ibeA and ibeT results in decreased expression of type 1 fimbriae in Extraintestinal pathogenic Escherichia coli strain BEN2908. Infect. Immun. 76, 4129–4136. doi: 10.1128/IAI.00334-08
Crépin, S., Harel, S., Dozois, C. M. (2012a). Chromosomal complementation using Tn7 transposon vectors in enterobacteriaceae. Appl. Environ. Microbiol. 78, 6001–6008. doi: 10.1128/AEM.00986-12
Crépin, S., Houle, S., Charbonneau, M.-E., Mourez, M., Harel, J., Dozois, C. M. (2012b). Decreased expression of type 1 fimbriae by a pst mutant of uropathogenic Escherichia coli reduces urinary tract infection. Infect. Immun. 80, 2802–2815. doi: 10.1128/IAI.00162-12
Datsenko, K. A., Wanner, B. L. (2000). One-step inactivation of chromosomal genes in Escherichia coli K-12 using PCR products. PNAS 97, 6640–6645. doi: 10.1073/pnas.120163297
De Pace, F., Nakazato, G., Pacheco, A., De Paiva, J. B., Sperandio, V., Da Silveira, W. D. (2010). The type VI secretion system plays a role in type 1 fimbria expression and pathogenesis of an avian pathogenic Escherichia coli strain. Infect. Immun. 78, 4990–4998. doi: 10.1128/IAI.00531-10
Dho, M., Lafont, J. P. (1982). Escherichia coli colonization of the trachea in poultry: comparison of virulent and avirulent strains in gnotoxenic chickens. Avian Dis. 26, 787–797. doi: 10.2307/1589865
Djonović, S., Urbach, J. M., Drenkard, E., Bush, J., Feinbaum, R., Ausubel, J. L., et al. (2013). Trehalose biosynthesis promotes Pseudomonas aeruginosa pathogenicity in plants. PloS Pathog. 9, 1–15. doi: 10.1371/journal.ppat.1003217
Dozois, C. M., Dho-Moulin, M., Brée, A., Fairbrother, J. M., Desautels, C., Curtiss, III, R. (2000). Relationship between the tsh autotransporter and pathogenicity analysis of the tsh genetic region. Infect. Immun. 68, 4145–4154. doi: 10.1128/IAI.68.7.4145-4154.2000
Elbein, A. D., Pan, Y. T., Pastuszak, I., Carroll, D. (2003). New insights on trehalose: a multifunctional molecule. Glycobiology 13, 17–27. doi: 10.1093/glycob/cwg047
Freeman, B. C., Chen, C., Beattie, G. A. (2010). Identification of the trehalose biosynthetic loci of Pseudomonas syringae and their contribution to fitness in the phyllosphere. Environ. Microbiol. 12, 1486–1497. doi: 10.1111/j.1462-2920.2010.02171.x
Germer, J., Muffler, A., Aronis, R. (1998). Trehalose is not relevant for in vivo activity of sigma S-containing RNA polymerase in Escherichia coli. J. Bacteriol. 180, 1603–1606. doi: 10.1128/JB.180.6.1603-1606.1998
Giaever, H. M., Styrvold, O. B., Kaasen, I., Strøm, A. R. (1988). Biochemical and genetic characterization of osmoregulatory trehalose synthesis in Escherichia coli. J. Bacteriol. 170, 2841–2849. doi: 10.1128/jb.170.6.2841-2849.1988
Hagberg, L., Engberg, I., Freter, R., Lam, J., Olling, S., Svanborg Edén, C. (1983). Ascending, unobstructed urinary tract infection in mice caused by pyelonephritogenic Escherichia coli of human origin. Infect. Immun. 40, 273–283. doi: 10.1128/iai.40.1.273-283.1983
Ipe, D. S., Ulett, G. C. (2016). Evaluation of the in vitro growth of urinary tract infection-causing gram-negative and gram-positive bacteria in a proposed synthetic human urine (SHU) medium. J. Microbiol. Methods 127, 164–171. doi: 10.1016/j.mimet.2016.06.013
Joseph, T. C., Rajan, L. A., Thampuran, N., James, R. (2010). Functional characterization of trehalose biosynthesis genes from E. coli: An osmolyte involved in stress tolerance. Mol. Biotechnol. 46, 20–25. doi: 10.1007/s12033-010-9259-4
Kandror, O., DeLeon, A., Goldberg, A. L. (2002). Trehalose synthesis is induced upon exposure of Escherichia coli to cold and is essential for viability at low temperatures. PNAS 99, 9727–9732. doi: 10.1073/pnas.142314099
Kaper, J. B., Nataro, J. P., Mobley, H. L. T. (2004). Pathogenic Escherichia coli. Nat. Rev. Microbiol. 2, 123–140. doi: 10.1038/nrmicro818
Kim, K. S. (2008). Mechanisms of microbial traversal of the blood-brain barrier. Nat. Rev. Microbiol. 6, 625–634. doi: 10.1038/nrmicro1952
Klein, W., Ehmann, U., Boos, W. (1991). The repression of trehalose transport and metabolism in Escherichia coli by high osmolarity is mediated by trehalose-6-phosphate phosphatase. Res. Microbiol. 142, 359–371. doi: 10.1016/0923-2508(91)90105-J
Lane, M. C., Simms, A. N., Mobley, H. L. T. (2007). Complex interplay between type 1 fimbrial expression and flagellum-mediated motility of uropathogenic Escherichia coli. J. Bacteriol. 189, 5523–5533. doi: 10.1128/JB.00434-07
Li, H., Su, H., Kim, S. B., Chang, Y. K., Hong, S. K., Seo, Y. G., et al. (2012). Enhanced production of trehalose in Escherichia coli by homologous expression of otsBA in the presence of the trehalase inhibitor, validamycin A, at high osmolarity. J. Biosci. Bioeng. 113, 224–232. doi: 10.1016/j.jbiosc.2011.09.018
Lin, W. F., Hu, R. Y., Chang, H. Y., Lin, F. Y., Kuo, C. H., Su, L. H., et al. (2022). The role of urease in the acid stress response and fimbriae expression in Klebsiella pneumoniae CG43. J. Microbiol. Immunol. Infect. 55, 620–633. doi: 10.1016/j.jmii.2022.02.002
MacIntyre, A. M., Barth, J. X., Pellitteri Hahn, M. C., Scarlett, C. O., Genin, S., Allen, C. (2020). Trehalose synthesis contributes to osmotic stress tolerance and virulence of the bacterial wilt pathogen Ralstonia solanacearum. Mol. Plant-Microbe Interact. 33, 462–473. doi: 10.1094/MPMI-08-19-0218-R
Marc, D., Arné, P., Brée, A., Dho-Moulin, M. (1998). Colonization ability and pathogenic properties of a fim- mutant of an avian strain of Escherichia coli. Res. Microbiol. 149, 473–485. doi: 10.1016/S0923-2508(98)80002-8
Mobley, H. L. T., Donnenberg, ,. M. S., Hagan, E. C. (2013). Uropathogenic Escherichia coli. EcoSal Plus 9, 275–304. doi: 10.1016/B978-0-12-397048-0.00009-7
Mulvey, M. A., Boado, Y. S. L., Wilson, C. L., Roth, R., Parks, W. C., Heuser, J., et al. (1998). Induction and evasion of host defenses by type I-piliated Uropathogenic Escherichia coli. Sci. (80-.). 282, 1494–1497. doi: 10.1126/science.282.5393.1494
Pavanelo, D. B., Houle, S., Matter, L. B., Dozois, C. M., Horn., F. (2018). The periplasmic trehalase affects type 1 fimbria production and virulence of Extraintestinal Pathogenic Escherichia coli Strain MT78. Infect. Immun. 86, 1–12. doi: 10.1128/IAI.00241-18
Pósfai, G., Koob, M. D., Kirkpatrick, H. A., Blattner, F. R. (1997). Versatile insertion plasmids for targeted genome manipulations in bacteria: Isolation, deletion, and rescue of the pathogenicity island LEE of the Escherichia coli O157:H7 genome. J. Bacteriol. 179, 4426–4428. doi: 10.1128/jb.179.13.4426-4428.1997
Randall, K., Lever, M., Peddie, B. A., Chambers, S. T. (1996). Natural and synthetic betaines counter the effects of high NaC1 and urea concentrations. Biochim. Biophys. Acta 65, 189–194. doi: 10.1016/S0304-4165(96)00057-8
Rimmele, M., Boos, W. (1994). Trehalose-6-phosphate hydrolase of Escherichia coli. J. Bacteriol. 176, 5654–5664. doi: 10.1128/jb.176.18.5654-5664.1994
Ruhal, R., Kataria, R., Choudhury, B. (2013). Trends in bacterial trehalose metabolism and significant nodes of metabolic pathway in the direction of trehalose accumulation. Microb. Biotechnol. 6, 493–502. doi: 10.1111/1751-7915.12029
Snyder, J. A., Haugen, B. J., Buckles, E. L., Lockatell, C. V., Johnson, D. E., Donnenberg, M. S., et al. (2004). Transcriptome of uropathogenic Escherichia coli during urinary tract infection. Infect. Immun. 72, 6373–6381. doi: 10.1128/IAI.72.11.6373-6381.2004
Spurbeck, R. R., Mobley, H. L. T. (2013). “Uropathogenic Escherichia coli”, in Escherichica coli. Pathotypes and Principles of Pathogenesis, ed. M.S. Donnenberg (Elsevier ISBN 978-0-12-397048-0), 499–521.
Strom, A. R., Kaasen, I. (1993). Trehalose metabolism in Escherichia coli: stress protection and stress regulation of gene expression. Mol. Microbiol. 8, 205–210. doi: 10.1111/j.1365-2958.1993.tb01564.x
Vanaporn, M., Sarkar-Tyson, M., Kovacs-Simon, A., Ireland, P. M., Pumirat, P., Korbsrisate, S., et al. (2017). Trehalase plays a role in macrophage colonization and virulence of Burkholderia pseudomallei in insect and mammalian hosts. Virulence 8, 30–40. doi: 10.1080/21505594.2016.1199316
Verma, R., Rojas, T. C. G., Maluta, R. P., Leite, J. L., da Silva, L. P. M., Nakazato, G., et al. (2015). Fimbria-encoding gene yadC has a pleiotropic effect on several biological characteristics and plays a role in avian pathogenic Escherichia coli pathogenicity. Infect. Immun. 84, 187–193. doi: 10.1128/IAI.01138-15
Keywords: ExPEC, extraintestinal E. coli, BEN2908, trehalose metabolism, type 1 fimbriae
Citation: Klemberg VS, Pavanelo DB, Houle S, Dhakal S, Pokharel P, Iahnig-Jacques S, Dozois CM and Horn F (2024) The osmoregulated metabolism of trehalose contributes to production of type 1 fimbriae and bladder colonization by extraintestinal Escherichia coli strain BEN2908. Front. Cell. Infect. Microbiol. 14:1414188. doi: 10.3389/fcimb.2024.1414188
Received: 08 April 2024; Accepted: 10 June 2024;
Published: 24 June 2024.
Edited by:
Sébastien Bontemps-Gallo, Univ. Lille, FranceReviewed by:
Brandon Robin, Institut Pasteur de Lille, FranceJean-Marie Lacroix, Université de Lille, France
Jianjun Dai, Nanjing Agricultural University, China
Copyright © 2024 Klemberg, Pavanelo, Houle, Dhakal, Pokharel, Iahnig-Jacques, Dozois and Horn. This is an open-access article distributed under the terms of the Creative Commons Attribution License (CC BY). The use, distribution or reproduction in other forums is permitted, provided the original author(s) and the copyright owner(s) are credited and that the original publication in this journal is cited, in accordance with accepted academic practice. No use, distribution or reproduction is permitted which does not comply with these terms.
*Correspondence: Fabiana Horn, ZmFiaWFuYS5ob3JuQHVmcmdzLmJy
†Present address: Daniel Brisotto Pavanelo, Laboratório Central do Rio Grande do Sul, Centro Estadual de Vigilância em Saúde, Secretaria da Saúde, RS, Brazil