- 1Department of Clinical Laboratory, The Affiliated Suzhou Hospital of Nanjing Medical University, Suzhou Municipal Hospital, Gusu School, Nanjing Medical University, Suzhou, Jiangsu, China
- 2Zhejiang University School of Medicine, Hangzhou, Zhejiang, China
As a complicated and heterogeneous condition, breast cancer (BC) has posed a tremendous public health challenge across the world. Recent studies have uncovered the crucial effect of human microbiota on various perspectives of health and disease, which include cancer. The oral-gut microbiome axis, particularly, have been implicated in the occurrence and development of colorectal cancer through their intricate interactions with host immune system and modulation of systemic inflammation. However, the research concerning the impact of oral-gut microbiome axis on BC remains scarce. This study focused on comprehensively reviewing and summarizing the latest ideas about the potential bidirectional relation of the gut with oral microbiota in BC, emphasizing their potential impact on tumorigenesis, treatment response, and overall patient outcomes. This review can reveal the prospect of tumor microecology and propose a novel viewpoint that the oral-gut microbiome axis can be a breakthrough point in future BC studies.
1 Introduction
Breast cancer (BC), the complicated and heterogeneous disorder, influences millions of women worldwide. It occupies 1/4 of female caners and exhibits the highest morbidity among female cancers, especially in transitioning countries (Arnold et al., 2022; Heer et al., 2020). Consistent with GLOBOCAN 2022 analysis of cancer patients in either developed countries or developing countries, major concerned cancers including BC, may increase eventually (Fatima et al., 2023; Wang et al., 2019, Wang et al., 2024; Liu et al., 2024). The World Health Organization (WHO) has initiated the Global BC Initiative to enhance awareness about women’s health issues and improve public health interventions (Anderson et al., 2021). Numerous studies have been performed to understand underlying mechanisms, risk factors, diagnostic techniques, and treatment options for BC (Koual et al., 2020; Zhang et al., 2023c). A large and growing body of literature has concentrated on the drug resistance, pharmacological anti-tumor efficiency, as well as clinicopathological and prognostic significances of BC, especially the triple-negative breast cancer (TNBC) (Geng et al., 2023; Zhang et al., 2023a; Kwon et al., 2023; Chen et al., 2023). Actually, early BC screening and prevention are vital for selecting treatment strategies for BC.
Recently, an increasing number of studies are conducted on different microbial species in the human body, their biological characteristics, and their correlations with various diseases (Wu et al., 2022b; Xiang et al., 2023). This growth can be attributed to the rapid advancements in microbiology and bioinformatics, as well as the improvements in microbial profiling technologies. In addition, it is becoming evident that every microbial habitat possesses unique microbial populations with different impacts on physiological homeostasis (Liu et al., 2022; Sun et al., 2023). Research has consistently indicated the association between dysbiosis in the microbiota and tumor initiation. Specific microbial species are correlated with a higher or lower risk of various cancers. For instance, primary gastric lymphoma carries a heightened susceptibility to gastric cancer or precancerous lesions, with the risk potentially related to Helicobacter pylori infection (Feng et al., 2023; Zhang et al., 2023e), while certain strains of Escherichia coli have been related to colon cancer (Blanco-Miguez et al., 2023; Wirbel et al., 2019). Emerging evidence indicates that breast microbiota is related to BC. Dysbiosis in the mammary gland and axillary region is associated with a higher BC risk. Specific bacterial species, including Staphylococcus and Streptococcus, have been found to be more abundant among BC patients than normal controls (Urbaniak et al., 2016; Kost et al., 2023). These microbes may promote chronic inflammation, DNA damage, and immune system dysregulation, therefore facilitating tumorigenesis. More investigations are warranted to completely explore the mechanisms and clinical implications of microbiota in different cancer.
The concept of multiple organ microbiota axes has entered the public awareness, including the ‘skin-gut’ microbiome axis, the ‘microbiota-gut-brain’ axis, the ‘lung−gut’ microbial axis, and the ‘oral-gut-liver’ axis (Russo et al., 2023a; Molina-Mateo et al., 2023; Nuzum et al., 2023; Wei et al., 2023; Niu et al., 2023). Recent research indicates that the construction of the microbiota-metabolic axis, such as the “gut microbiota-metabolites-serum lipid index” axis, contributes to identifying alternative strategies to improve high-fat diet (HFD)-induced hyperlipidemia (Zeng et al., 2023a). Based on these studies, it can be inferred that each microorganism exhibits different microbial population patterns in different habitats. The interplay and interactions between physical and biological communities among different organs influence the genesis and progression of various disorders.
Gut and oral microbiomes are two main microbial ecosystems in human body, which are vital for microbiome−related disorders. The most common bacteria in the oral cavity are members of Firmicutes, Proteobacteria, and Actinobacteria. However, compared to the gut microbiota, which undergoes more significant fluctuations, the oral microbiota ecosystem exhibits a relatively stable nature. The gut microbiota is a diverse and intricate microbial ecosystem that primarily comprises bacteria, viruses, fungi, and archaea. They work in coordination with the human body to provide a range of health benefits, such as enhancing the intestinal barrier, supporting the immune system, and providing energy (Schwabe and Jobin, 2013). The oral-gut microbiome axis indicates the dynamic relationship of microbial communities within oral cavity and the gut, and it is of great importance for human health and disease. Recent studies have emphasized the influence of oral-gut microbiome axis on cancer development and progression (Liu et al., 2022; Russo et al., 2023b; Elghannam et al., 2023; Jeong et al., 2023). The oral-gut microbiome axis can impact cancer through multiple mechanisms. Microbes can directly interact with host cells, affecting immune responses, inflammation, and DNA damage, which are key factors in cancer development. Evidence from mechanistic studies reveals that the microbiota can interact with host immune cells and epithelia by releasing a wide array of metabolites, proteins, and macromolecules, further affecting tumor cell behavior, angiogenesis, and treatment response. The oral-gut microbiome axis also regulates systemic metabolism, endocrine signaling, and drug metabolism, therefore influencing cancer progression and treatment outcomes (Pushalkar et al., 2018; Routy et al., 2018; Li et al., 2022; Qu et al., 2023; Wong and Yu, 2023).
Currently, the oral-gut microbiome axis exhibits a closer correlation with intestinal disorders due to the intricate relationship between microbial communities and gut health. The oral-gut microbiome axis emerges as the significant research topic in colorectal cancer (Liu et al., 2022; Lam et al., 2022). However, studies on the correlation of microbial communities of oral-gut microbiome axis with another major female cancer, BC, have certain limitations. This study provides the comprehensive review on the research related to remicrobial communities in the oral-gut microbiome axis in relation to BC. It is vital to understand the impact of oral-gut microbiome axis on BC, which holds implications for clinical practice. Oral-gut microbiome may be a source of biomarkers for BC risk assessment, early detection, and monitoring of treatment response.
2 The oral-gut microbiome axis
2.1 The oral and gut
The oral cavity and the gut are integral components in human body, serving vital functions. Anatomically, the human digestive system comprises the digestive tract and accessory digestive organs, including the liver and pancreas. The digestive tract spans from oral cavity to the gut, specifically the anus, making oral cavity and gut the anatomically neighboring regions that are interconnected through the gastrointestinal tract (Schmidt et al., 2019; Liu et al., 2022). Oral cavity is the primary site in the digestive tract, encompasses structures such as teeth, gums, and the tongue, and it fulfills the critical roles of mechanically breaking down food by chewing and initiating the digestive process. Furthermore, it hosts a complex microbial community that influences oral health and is important for systemic health (Liu et al., 2022; Eltay and Van Dyke, 2023). Especially, it provides various binding sites that microorganisms can adhere to and colonize. The gut, specifically the intestines, is primarily responsible for nutrient digestion and absorption (Belkaid and Hand, 2014). In the gut resides, there are various microorganisms called the gut microbiota. This intricate microbial community is important for maintaining overall health. It actively participates in digestion, synthesizes essential vitamins, modulates immune system, and contributes to defense against harmful pathogens (Tilg et al., 2020; Zeng et al., 2023b). Therefore, there is a potential correlation of oral microbes with the initiation and progression of gastrointestinal diseases (Liu et al., 2022). In fact, the oral and gut microbiomes exert a vital impact on keeping homeostasis in human body. The presence of oral-gut barrier ensures a clear separation in microbial distribution between oral cavity and gastrointestinal tract. Many studies have reported that certain bacteria, when colonizing specific sites and causing dysbiosis of oral and gut microbiota, may trigger the genesis and progression of various tumors, while also exerting a series of impacts on the surrounding microenvironment (Table 1).
2.2 Bidirectional microbial translocation between oral and gut microbiota
Recently, numerous studies have revealed the presence of microbial translocation between oral and gut microbiota. In general, intestinal microbial colonization in the oral cavity is uncommon. However, specific conditions such as poor hygiene or immunological alterations may facilitate microorganisms translocation from gastrointestinal tract to oral cavity (Liu et al., 2022). Bifidobacterium, a bacterial genus, is known to be the predominant microorganism present in the intestinal tract of newborns. However, recent investigations have identified gut-dwelling Bifidobacterium in oral fluid in neonates (Makino, 2018). In individuals with inflammatory bowel disease (IBD), gut microbes have the capacity of impacting oral flora composition in a direct or indirect manner by modulating the host’s immune function (Liu et al., 2022). In addition to in vivo transmission, gastrointestinal microorganisms such as Helicobacter pylori, Vibrio cholerae or enteric viruses like Hepatitis A virus and Hepatitis E virus, can be transmitted through fecal-oral axis by directly contacting or indirectly contacting with polluted fluids and food (De Graaf et al., 2017; Bui et al., 2016; Chapman et al., 2023). Recent research has revealed that Klebsiella pneumoniae has the ability to disseminate to sterile sites in one host or transmit to another host via the fecal-oral route (Hudson et al., 2022).
Under specific circumstances, oral microbes have the potential to overcome physicochemical barriers existing between oral cavity and the gut, allowing for their potential translocation into the gut (Park et al., 2021). The changes observed in gut microbiome are caused by a decrease in stomach acidity, which enables a higher survival rate for bacteria ingested along with food and oral mucus. Therefore, the species present in the oral microbiome exhibit increased abundance in the gut microbiome of individuals who use proton pump inhibitors (PPIs) (Imhann et al., 2016). As suggested by Koji Atarashi, certain Klebsiella bacterial strains present in the salivary microbiome can colonize in the gut (Atarashi et al., 2017). Another research has shown that the prevalence of oral bacterial transition such as Porphyromonas, Fusobacterium, and Pseudoramibacter to gut increases among the elderly relative to adults, hoping that oral health care among the elderly can influence the gut microbiota composition (Iwauchi et al., 2019). In patients diagnosed with inflammatory bowel disease (IBD), Haemophilus and Veillonella abundances are significantly enriched within gut mucosa. These particular microbial species are recognized as oral commensal microbes (Gevers et al., 2014).
In tumor-related diseases, several studies have reported that significant dysbiosis is observed in the colonic environment of tumor-bearing colons, indicating a considerable degree of microbial imbalance. Gemella, Peptostreptococcus, Parvimonas, and other oral-origin microbes form a robust symbiotic network that characterizes the metacommunity associated with colorectal cancer (CRC). Through probabilistic partitioning of relative abundance profiles, the CRC-associated metacommunity is primarily dominated by members of oral microbiome (Nakatsu et al., 2015). According to the above results, under circumstances of disrupted mucosal homeostasis, the normal human oral microbiota possesses the ability of invading and colonizing gut mucosa, potentially transforming into an opportunistic pathogen (Park et al., 2021). In an observational study, Streptococcus salivarius and Streptococcus parasanguinis had significantly increased total relative abundances in the intestinal tract of elderly participants or those with dental plaque accumulation. This study provides evidence for oral bacterial translocation into the gut (Kageyama et al., 2023).
Among the gut-colonized oral bacteria, Hongze Zhang et al. reported that Fusobacterium abundance significantly increased, while Prevotella and Ruminococcus abundances obviously reduced among CRC samples (Zhang et al., 2023b). Oral strains of Fusobacterium nucleatum could be usually detected in CRC tissues, indicating the possible oral origin of F. nucleatum in cancers. In addition, the F. nucleatum level is related to the development, metastasis, and resistance to chemotherapy in colorectal cancer, potentially through the induction of autophagy pathways (Boyanova et al., 2023; Fujiwara et al., 2020; Sun et al., 2020). Certain oral anaerobes, such as Fusobacterium necrophorum and Fusobacterium nucleatum, are components of normal pharyngeal microbiota. Their potential translocation to other gastrointestinal tissues has been related to carcinogenesis (Boyanova et al., 2023). In conclusion, there is a significant intrinsic connection between oral and gut microbiota. Bidirectional microbial translocation between the oral and gut can mutually reshape microbial ecosystems of both environments, finally modulating the physiopathological processes of gastrointestinal diseases, including cancer. Investigating the association of oral-gut microbiota with various diseases may be vital for disease prediction and future therapeutic approaches.
3 Oral and gut microbes in breast cancer
Human commensal microbiota is vital for both health and disease. The microbial composition within various organs of human body reflects the differences in host genetics and lifestyle. The dynamic equilibrium between microbiota and host makes local and systemic effects on the body, and microbial dysbiosis may increase a variety of health risks. Many bacteria can encode genes for estrogen-metabolizing enzymes, therefore influencing the regulation of serum estrogen contents. On the contrary, estrogen-like compounds can enhance the growth and development of some bacteria. Therefore, the interaction of the microbiota with endogenous hormones, and estrogen-like compounds, may make synergistic effects in providing protection against diseases but also potentially increasing the hormone-related disorder risk. Recently, microbiota in BC patients has been found to be different from normal females, implying that some bacteria are related to the occurrence of BC and diverse cancer treatment responses. The gastrointestinal tract harbors the highest abundance of commensal microbiota, while oral microbiota, at the entry point of human digestive tract, consists of nearly 700 different microbial species, second only to the gut microbiota in diversity. Changes in the abundance of these two most abundant microbial habitats have also been correlated with BC (Table 2).
3.1 Oral microbes in breast cancer
Female BC is actually the prevalent metastatic cancer and leading factor inducing cancer-associated mortality in females globally (Fatima et al., 2023). Based on the geographic variation and distribution of the disease, Arnold et al. have made a prediction that by the year 2040, the incidence of newly diagnosed BC cases will be elevated by over 40%, causing approximately 3 million patients every year, especially in transitioning countries. To address this growing burden, global initiatives and public health interventions that target the entire cancer control spectrum, including early diagnosis, primary prevention, screening, and treatment, are imperative (Arnold et al., 2022). Despite the wide attention and relatively comprehensive treatment options in clinical practice, BC still poses significant challenges in terms of understanding its pathogenesis and achieving precise prevention measures. Besides, 20% of BC cases belong to the TNBC category, characterized by a heightened propensity for recurrence, unfavorable prognosis, significant metastatic potential, and reduced survival rate (Lu et al., 2023).
The most common risk factors for BC include aging, family history, lifestyle factors, and endogenous/exogenous estrogen exposure (Niccolai et al., 2023; Sung et al., 2021). The human microbiota exerts significant influence on the occurrence and progression of BC, which may be the potential factor in early diagnosis. The complex microbial community residing in various body sites, including the breast tissue, makes a vital impact on keeping tissue homeostasis and immune regulation (Kim et al., 2021; Vitorino et al., 2022). Dysbiosis of microbial community is associated with inflammatory responses, genetic mutations, and changes in hormone metabolism that can promote carcinogenesis in the breast tissue (Niccolai et al., 2023; Saud Hussein et al., 2021). It was found that Bacteria isolated from breast tissue of Caucasian women exhibited the highest Proteobacteria and Firmicutes phyla relative abundances, alongside the presence of common oral bacteria including Fusobacteria and Streptococcus genera (Hao et al., 2019; Urbaniak et al., 2014). The oral microbiota is shown to have a potential effect on BC occurrence and development. Proteobacteria were found to be the predominant phylum within breast tissue. However, in the oral cavity, members of this phylum constitute a smaller portion of the overall bacterial community (Wade, 2013; Saud Hussein et al., 2021).
Significantly, Fusobacterium nucleatum (F. nucleatum) has been an anaerobic oral commensal that can be detected in BC tissue. Based on AXIS tool analysis, oral Fusobacterium nucleatum was positively related to BC. The high oral Fusobacterium nucleatum level, often associated with gingivitis/periodontitis, was found to increase the BC risk (Gaba et al., 2022). Based on Van der Merwe et al., F. nucleatum enhanced BC development via TLR4/MyD88 pathway activation, showing immunomodulation (Fan et al., 2023; Van Der Merwe et al., 2021). In recent studies, BC specimens exhibit the presence of Fusobacterium nucleatum chromosomal DNA, which is positively correlated with high levels of Gal-GalNAc and BC progresses. Fusobacterium nucleatum depends on its Fap2 to bind to BC specimens, which can be inhibited by GalNAc. Intravenous injection of Fusobacterium nucleatum expressing Fap2 can specifically colonize mouse mammary tumors. Injection of Fusobacterium nucleatum can hinder anti-tumor T lymphocyte accumulation and infiltration and enhance tumor development and migration, which is reversed through antibiotic application. Therefore, the study results suggest that colonization of Fusobacterium nucleatum in BC can accelerate tumor development and migration (Parhi et al., 2020). Targeting Fusobacterium nucleatum or its Fap2 during BC treatment may hold promise for therapeutic interventions. In another investigation about whether oral microbiome is related to BC and benign breast disease in Ghana, Porphyromonas and Fusobacterium have decreased relative abundances in BC patients relative to controls. Alpha-diversity and presence/relative abundance of specific genera in oral microbiome was closely related in BC patients (Wu et al., 2022c). This study suggests a potential correlation between the abundance of different bacterial taxa in cancer cases and geographical regions. In the Women’s Health Initiative Observational Study, a comprehensive and extensively studied prospective cohort of postmenopausal women, a history of diagnosed periodontal disease was associated with a higher susceptibility to primary, invasive BC. These findings are consistent with the notion that chronic inflammation may contribute to BC risk, and they indicate that oral microbiome may be involved in BC occurrence and prevention (Freudenheim et al., 2016).
Recent studies on BC patients receiving chemotherapy reveal dysbiosis of oral microbiota based on saliva data. Following chemotherapy, Neisseria, an aerobic genus found in the human oral cavity, has reduced abundance, while potentially pathogenic taxa including Escherichia/Shigella have significantly increased abundances. Our findings indicate that certain oral bacterial taxa are related to side effects of cancer treatment. Therefore, during chemotherapy, the oral microbial status during cancer treatment is of great importance (Klymiuk et al., 2022). However, there have been conflicting opinions concerning the possible association of periodontal disease with BC. Bernhard et al. suggested that there is no direct association between the subgingival microbiota including Actinomyces gerencseriae and BC by clinical and microbiological assessment in BC patients, and an indirect pathway should be addressed in further studies (Silva-Boghossian et al., 2018).
In another hospital-based study, it was observed that women diagnosed with periodontitis had a two to threefold increased likelihood of developing BC compared to women without periodontitis, and these findings strongly suggest that periodontitis is significantly related to BC (Sfreddo et al., 2017). In a recent prospective cohort study investigating the potential relation of periodontitis with invasive BC, no definitive association of periodontal disease with the overall risk of developing BC was found (Jia et al., 2020). Some scholars argue that there is no obvious evidence supporting the association of periodontitis with BC, the divergent outcomes across studies may be caused by variations in research design, assessment of periodontal health, confounder adjustment, duration of follow-up, and sample size (Issrani et al., 2021).
In conclusion, while the relation of oral microbiota with BC remains controversial, there is significant potential in exploring certain oral microbial species as risk factors for BC development and biomarkers. Investigating the intricate connection between oral microbiota and BC holds profound research value and promising prospects.
3.2 Gut microbes in breast cancer
The gut microbiota comprises a variety of microorganisms, including bacteria, yeast, and viruses. The major gut microbial phyla include Firmicutes, Bacteroidetes, Actinobacteria, Proteobacteria, Fusobacteria, and Verrucomicrobia. In the Firmicutes phylum, there are over 200 diverse genera, such as Lactobacillus, Bacillus, Clostridium, Enterococcus, and Ruminicoccus. Bacteroidetes mainly include Bacteroides and Prevotella. The Actinobacteria phylum has a relatively lower abundance, which is primarily represented by Bifidobacterium genus (Thursby and Juge, 2017; Rinninella et al., 2019). Gut microbiomes participate in the pathogenesis, produce inflammatory cytokines, and break the intestinal barrier integrity, promoting BC (Xia et al., 2023).
3.2.1 Gut microbiota dysbiosis in breast cancer
As reported by Rosean et al., gut microbiota dysbiosis directly influences BC dissemination. Prior to tumorigenesis, a disruption of the gut microflora, can sufficiently promote collagen accumulation in tissue and tumor microenvironments, further resulting in systemic alterations corresponding to the more invasive HR+ BC (Buchta Rosean et al., 2019). In a clinical analysis on invasive cancer of non-specific type (HR+ and HER2-), results from newly diagnosed BC patients indicated that Shannon index, employed as a measure for diversity, significantly decreased in BC group relative to the control group. The study emphasizes that microbial diversity decreases, the Firmicutes relative abundance increases, and Bacteroidetes abundance reduces among early BC patients in comparison with normal controls. A trend to the reduced Odoribacter sp, Butyricimonas sp, and Coprococcus sp abundances could also be observed (Bobin-Dubigeon et al., 2021; Jaye et al., 2021). Based on this preliminary study, some variations may be observed in intestinal bacterial composition in BC patients compared with healthy individuals. Consistent with this conclusion, Haque et al. found the reduced gut microbiota diversity among BC patients, accompanied by the elevated Clostridiales abundance in relative to normal controls (Haque et al., 2022).
Recent studies have shown that Bacillus, Staphylococcus, Enterobacteriaceae, Comamondaceae, and Bacteroidetes exhibited significantly elevated relative abundances among BC patients (Saud Hussein et al., 2021; Urbaniak et al., 2016). A recent cross-sectional study first suggested that Bifidobacterium abundance in gut microbiota was positively related to omega-3 polyunsaturated fatty acids (PUFAs) in blood of BC survivors (Horigome et al., 2019). A retrospective analysis demonstrated that Bacteroides exhibit frequent and significant enrichment into the intestinal tract in BC patients (Wang et al., 2022). In another clinical study involving BC and Benign Breast Lesions (BBLs), BC patients exhibited increased levels of Porphyromonas and Peptoniphilus compared to healthy individuals, while the benign breast lesion group exhibited higher abundance of Escherichia and Lactobacillus in comparison (Ma et al., 2022). It is of note that specific gut microbiota abundance is related to difficult clinicopathological factors, including ER, PR, Ki-67, Her2, and hormone receptor+ or triple-negative levels (Nandi et al., 2023; Haque et al., 2022).
In investigations specifically addressing select groups of BC patients, Postmenopausal BC patients were shown to exhibit increased levels of Escherichia coli, Enterococcus gallinarum, Actinomyces, Shewanella putrefaciens, and Erwinia amylovora, while showing reduced levels of Eubacterium eligens and Lactoisacus. The intestinal metagenomes of postmenopausal BC patients exhibited the increased levels of genes related to lipopolysaccharide biosynthesis, a vital factor triggering systemic inflammation, which may potentially contribute to the promotion of neoplastic transformation (Barrea et al., 2023; Zhu et al., 2018). It was reported that two SCFA (short-chain fatty acids)-producing bacteria had substantially decreased abundances in premenopausal BC group, implying that Pediococcus and Desulfovibrio might be the diagnostic biomarkers for premenopausal BC (He et al., 2021). Some vivo studies showed that tumor-bearing mice exhibited compromised colonic barrier integrity, including decreased Lactobacillus abundance, and increased Bacteroides abundance in the orthotopic and syngeneic mammary tumor model. Various bacteria separated from spleens in these mice were determined to have originated from intestines (Loman et al., 2022). Intestinal bacteria including Lachnospiraceae, Lactobacillus and Alistipes were significantly changed in the xenograft nude mice challenged with BC cells (Zhang et al., 2021). Noteworthily, fecal microbiome in BC patients also displayed significant changes. According to Ma et al., Faecalibacterium had decreased abundance among BC patients, which was negatively associated with phosphorocholine. It indicates that the combination of detecting flora bacteria like Faecalibacterium and flora metabolites including phosphorolcholine, is beneficial for BC screening (Mikó et al., 2018; Ruo et al., 2021; Ma et al., 2020).
Collectively, these studies provide compelling evidence supporting that BC is related to obvious changes of gut microbiota. In addition, gut microbiome significantly affects the host overall health and physiology, establishing itself as a critical frontier in understanding BC pathogenesis.
3.2.2 Metabolites and mechanisms associated with gut microbiota dysbiosis in BC
Gut microbiota includes diverse commensal microorganisms, including trillions of bacteria, viruses, and fungi. These microbial populations coexist in symbiosis with host; besides, their associated metabolites significantly influence human health. The gut microbiota makes an important effect on regulating metabolic, immune, and endocrine functions (Portincasa et al., 2022). Actually, current evidence strongly supports that metabolic disorders are crucial for microbial dysbiosis, therefore indirectly influencing the initiation and progression of neoplasms. Some of the key mechanisms include indirect processes like metabolite generation, including short-chain fatty acids (SCFAs). The glucuronidase activity of estroboloma bacteria, related to estrogen metabolism, also exerts a vital effect on this progression (Cazzaniga et al., 2022). Among the bacterial metabolites, short-chain fatty acids (SCFAs) such as acetate (C2), propionate (C3), and butyrate (C4), have the highest abundances in human body and colon, serving as prominent anions in the colon (Portincasa et al., 2022).SCFAs, secondary bile acids, and other metabolites are related to BC development, which may also be vital for modulating the response to chemotherapy (Plaza-Diaz and Álvarez-Mercado, 2023). SCFAs are a vital group of metabolites generated by bacterial species Eubacterium rectale, Clostridium leptum, and Faecalibacterium prausitzii, and by lactate-utilizing species Anaerostipes and Eubacterium hallii, directly influence can be exerted on different immune cells in the intestinal environment. Obviously, a substantial reduction in colonic SCFA levels has been found in premenopausal BC patients, indicating that SCFAs are vital for underlying pathological mechanisms of premenopausal BC (Zhang et al., 2022; Hill et al., 2014; Helmink et al., 2019). By being engaged with FFAR3, SCFAs impede the activation of MAPK signaling in BC cells, suppressing Hippo/YAP pathway, up-regulating E-cadherin, and prompting a transition of these malignant cells towards a non-invasive phenotype (Thirunavukkarasan et al., 2017; Yang et al., 2023). In the mouse model following BC cell injection, Zhang et al. reported that butyrate-producing genera, Lachnospiraceae and Ruminococcaceae, had decreased levels, which are known to promote the production of butyric acid and exert a protective role against colon cancer and triple-negative BC (TNBC) (Zhang et al., 2020a). Butyrate plays a crucial role as a nutrient source for colonocytes lining mammalian colon, and it is the histone deacetylase (HDAC) inhibitor. Sodium butyrate can stimulate apoptosis, induce ultrastructural changes, and enhance anti-tumor activity.
Besides, along with other HDAC inhibitors, Sodium butyrate has exhibited therapeutic effects on TNBC, particularly when used in combination with other anticancer agents (Zhang et al., 2021; Lee et al., 2016; Salimi et al., 2017; Wang et al., 2016; Sharma and Tollefsbol, 2022). Thus, the observed reduction in butyrate-producing genera, specifically Lachnospiraceae and Ruminococcaceae, in tumor-bearing mice supports the hypothesis that intestinal dysbiosis plays a role in enhancing BC. The following studies demonstrated that the gut microbiota-produced compound cadaverine exerts tumor-suppressive effects on BC by reversing epithelial-mesenchymal transition (EMT) and diminishing cancer cell stemness, motility, and metastatic characteristics (Kovács et al., 2019; Vergara et al., 2019). In addition, an in-vitro study findings revealed that the metabolite deoxycholic acid (DCA), specifically associated with Clostridium, enhances HER2-positive BC cell growth. It also stimulates G0/G1 phase cells transition into the S phase, potentially by activating peptide-O-fucosyltransferase and neuroactive ligand-receptor interaction pathway (Wang et al., 2022). Based on intestinal microbiota analysis, Vitamin D receptor (VDR) deficiency results in the shift of bacterial profile from normal to tumorigenesis risk in BC (Zhang et al., 2023d).
Sex hormone levels were closely related to some identified or suspected risk factors related to BC, and disruptions in microbiota/estrobolome may lead to increased levels of circulating estrogens and the corresponding metabolites, increasing the susceptibility to BC (Endogenous et al., 2011). The metabolism of estrogens occurs primarily in the liver, where they undergo conjugation and are subsequently excreted into the gastrointestinal tract through the bile. In the gut, these conjugated estrogens are deconjugated by bacterial β-glucuronidase enzymes produced by various bacteria, including members of the Clostridia and Ruminococcaceae families, the Clostridium leptum cluster, the coccoides cluster, and the Escherichia/Shigella bacterial group. After being deconjugated, the estrogens are then re-absorbed in their free form through the enterohepatic circulation, ultimately reaching the breast (Fernández et al., 2018; Rea et al., 2018). According to some relevant studies, the most common phyla in breast cancer tumors are Proteobacteria, Actinobacteria, and Firmicutes. Additionally, Methylobacterium radiotolerans are relatively abundant, and breast cancer patients exhibit significantly higher relative abundances of Bacillus, Staphylococcus, Enterobacteriaceae, Comamondaceae, and Bacteroidetes in their tumor tissues (Fernández et al., 2018; Xuan et al., 2014) (Figure 1). In the gut, microbes decompose indigestible dietary polyphenols into estrogen-like metabolites with biological activities which are generated through oxidation and reduction, and the induced estrogen-inducible growth factor production, such as estromedin by these bacteria may be potentially used for carcinogenesis (Alvarez-Mercado et al., 2023; Dabek et al., 2008; Toumazi et al., 2021). Certain commensal organisms possess the ability, through sulfatase activity, to convert inactive steroids into active estrogen. Stimulating estrogen receptors in the breast can promote cells that enter G0 and G1 phases, triggering BC cell growth. Activating mitochondrial estrogen receptors can promote mitochondrial oxidation, which leads to elevated production of free radicals, thereby promoting BC occurrence (Ruo et al., 2021). The potential therapeutic strategy of recovering gut microbiota balance and decreasing systemic estrogen content is recognized to lower the overall risk and advancement of BC. More efforts must prioritize the examination of gut microbial metabolites and the potential association with an increased BC risk.
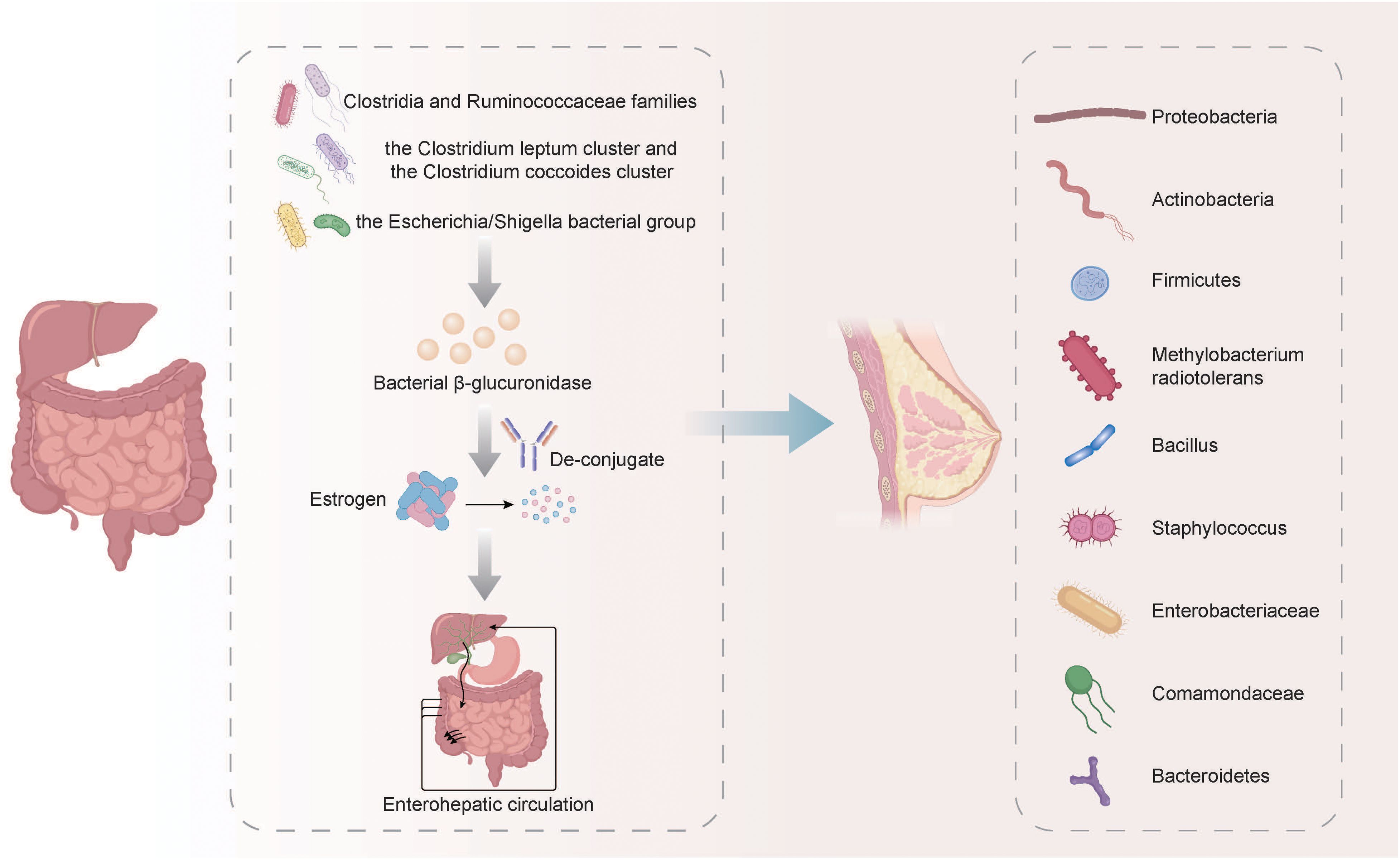
Figure 1. The metabolism of estrogens and its relationship with the microbiota in BC. The metabolism of estrogens occurs primarily in the liver, where they undergo conjugation and are subsequently excreted into the gastrointestinal tract through the bile. In the gut, these conjugated estrogens are deconjugated by bacterial β-glucuronidase enzymes produced by various bacteria, including members of the Clostridia and Ruminococcaceae families, the Clostridium leptum cluster, the coccoides cluster, and the Escherichia/Shigella bacterial group. After being deconjugated, the estrogens are then re-absorbed in their free form through the enterohepatic circulation, ultimately reaching the breast. The breast cancer tumors exhibit relatively high abundance of Proteobacteria, Actinobacteria, Firmicutes, Methylobacterium radiotolerans, Bacillus, Staphylococcus, Enterobacteriaceae, Comamondaceae and Bacteroidetes.
Obviously, dysbiosis of gut microbiota and disturbances in their metabolites can directly or indirectly impact cancer genesis and progression. Many microorganisms and the metabolites are related to signaling pathways linked to BC genesis and progression, as well as changes of surrounding microenvironment. Understanding these signaling pathways can shed novel lights on clinical interventions for the treatment of BC (Figure 2). Microbial composition in BC females differs from normal controls, in terms of bacterial species, their relative abundance, and functional attributes like metabolism and ability to induce DNA damage (Plaza-Díaz et al., 2019). Ana Isabel et al. proposed that double-strand breaks which pose the greatest threat among various DNA damage types resulting from genotoxins, ionizing radiation, reactive oxygen species (ROS), are probably related to BC development. Breast cancer is usually caused by epithelial cells and human exposure to carcinogens through diet and the environment encompasses contact with many fat-soluble genotoxins like polycyclic aromatic hydrocarbons (PAHs), nitro-PAHs, and heterocyclic aromatic amines which have the potential to trigger mammary tumors in rodents (Martin, 2001).Toxins generated by Helicobacter pylori and Bacteroides fragilis can activate the human enzyme spermine oxidase, which causes the production of ROS and hydrogen peroxide to result in DNA damage (Vivarelli et al., 2019; Alvarez-Mercado et al., 2023). When E. coli and Campylobacter jejuni can release cytolethal distending toxin (CDT) near the gastrointestinal epithelium, resulting in the generation of DNA double-strand breaks in epithelial cells. This process leads to a temporary cell cycle arrest and creates an environment in which mutations can occur, potentially leading to the formation of tumors (Alvarez-Mercado et al., 2023; Gori et al., 2019). In a study performed from Urbaniak et al., Staphylococcus epidermidis, separated in BC patients, could induce DNA double-stranded breaks. Noteworthily, other Enterobacteriaceae family members are also capable of producing colibactin (Urbaniak et al., 2016; Scott et al., 2019; Alvarez-Mercado et al., 2023). Gut microbiome also plays a role in disrupting epigenetic regulation, which can interact with the tumor. Microorganisms in the gut can generate low-molecular-weight bioactive substances including folates, SCFAs, and biotin. These substances are actively related to various epigenetic processes, such as modifying substrates for methylation or synthesizing complexes for modifying epigenetic enzyme activities (Vitorino et al., 2021; Haque et al., 2022).
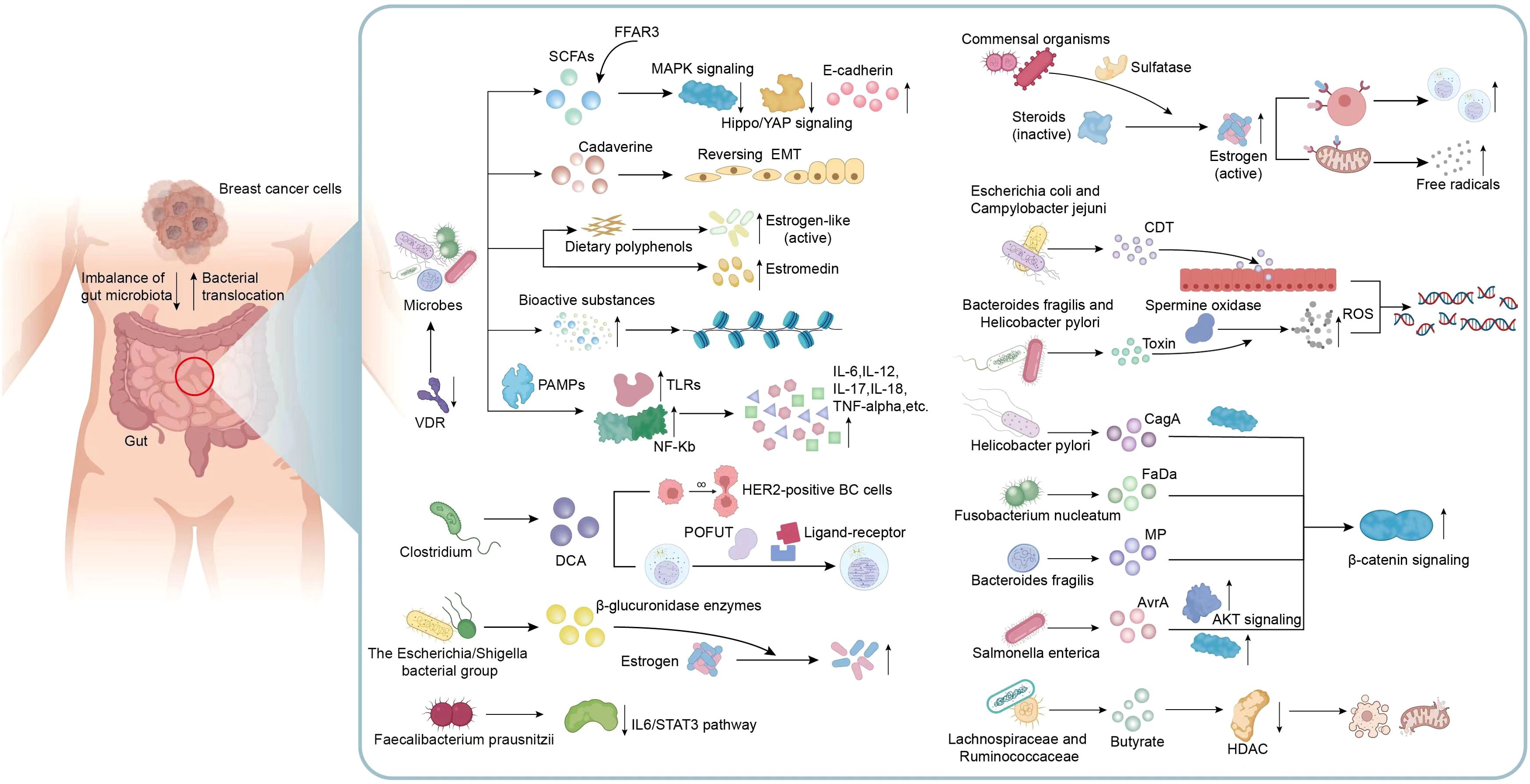
Figure 2. Mechanisms related to gut microbiota and metabolic dysbiosis in BC. Metabolic disorders exert a vital role in microbial dysbiosis, therefore indirectly affecting the initiation and progression of BC. Many microorganisms and their metabolites including VDR, SCFAs, Cadaverine and estrogen-like metabolites are involved in the mechanisms associated with the occurrence and development of BC, including the transition of invasive phenotypes, epithelial-mesenchymal transition, motility and metastatic characteristics, epigenetic processes, active estrogen production and inflammatory responses in the microenvironment. In addition, metabolites produced by certain specific bacteria are also involved in many signaling pathways associated with BC. For example, DCA specifically associated with Clostridium, enhances the proliferation of HER2-positive BC cells and regulate cell cycle; Faecalibacterium prausnitzii exhibited a suppressive effect on the growth of BC cells through inhibiting the IL6/STAT3 pathway; The toxins produced by Bacteroides fragilis, Helicobacter pylori, E. coli and Campylobacter jejuni can activate DNA damage and result in the formation of tumors; Metabolites from Helicobacter pylori, Fusobacterium nucleatum, Bacteroides fragilis, and Salmonella enterica possess the ability to activate β-catenin signaling, contributing to cancer; A reduction in the abundance of butyrate produced by Lachnospiraceae and Ruminococcaceae can attenuate anti-tumor activity. VDR, Vitamin D receptor; SCFAs, short-chain fatty acids; EMT, epithelial-mesenchymal transition; TLRs, Toll-like receptors; PAMPs, pathogen-associated molecular patterns; DCA, Metabolite deoxycholic acid; POFUT, peptide-O-fucosyltransferase; ROS, reactive oxygen species; CDT, cytolethal distending toxin; AKT, Protein kinase B, PKB; HDAC, histone deacetylase.
The promotion of BC by gut bacteria occurs through inducing chronic inflammation, and it is associated with tumor formation. Gut bacteria stimulate upregulation of Toll-like receptors (TLRs) and activation of NF-kB through pathogen-associated molecular patterns (PAMPs). NF-kB activation triggers secretion of various factors such as IL-6, IL-12, IL-17, IL-18, and tumor necrosis factor-alpha (TNF-alpha), causing sustained inflammation within tumor microenvironment (Vitorino et al., 2021). In previous studies, The H. pylori cagA gene product, engages in a physical interaction with E-cadherin, disrupts the complex formation between E-cadherin and beta-catenin, leading to the accumulation of beta-catenin in the cytoplasm and nucleus regardless of CagA’s tyrosine phosphorylation status (Murata-Kamiya et al., 2007). Fusobacterium nucleatum (Fn) attaches to E-cadherin, targeting a specific 11-amino-acid region, thereby activating β-catenin signaling and exerting differential regulation on inflammatory and oncogenic responses (Rubinstein et al., 2013). In addition, metalloproteinase (MP) toxin in Bacteroides fragilis, and Salmonella enterica’s virulence factor A (AvrA) possess the ability to activate β-catenin signaling, further modulate cell growth, and survival pathways, therefore facilitating cancer development (Alvarez-Mercado et al., 2023). CagA protein derived from Helicobacter pylori exerts its influence on the MAPK pathway, while the AvrA factor produced by Salmonella enterica promotes the MAPK and AKT pathways (Alvarez-Mercado et al., 2023; Bronte-Tinkew et al., 2009; Vivarelli et al., 2019). It was clearly revealed that Faecalibacterium prausnitzii exhibited a suppressive impact on BC cell proliferation through suppressing IL6/STAT3 pathway. The above result suggests a potential avenue for BC treatment (Ruo et al., 2021).
Furthermore, the gut microbiota profile could be significantly altered by diet, obesity, alcohol consumption, and changes in circulating hormonal levels, indicating that microbiota make-up is strongly associated with BC occurrence. The disrupted microbiota, leading to changed microbial-related activities, probably influences the risk of developing BC (Smith et al., 2021; Bouter et al., 2017; Teng et al., 2021). The high-fat diet and presence of obesity affect the gastrointestinal microorganism composition and contribute to increased estrogen levels (Toumazi et al., 2021). The gut microbiota that produces the enzyme β-glucuronidase (GUS) has the potential to enhance estrogen bioavailability by breaking down estrogen-glucuronide conjugates, thereby facilitating their reabsorption into the circulation. Consequently, this increase in circulating estrogens may contribute to the development of estrogen receptor-positive breast cancer (BC) (Arnone and Cook, 2022). Therefore, these factors may serve as risk factors for BC occurrence (Feng et al., 2021). It is widely suggested that BC is related to gut microbiome. However, future investigations are required to explore the detailed mechanisms of various clinical observations mentioned. These findings provide novel clues for potential intervention targets that could be used in clinical treatment for BC.
3.3 The oral-gut axis in breast cancer therapies
BC exhibits significant variability in its clinical progression. Different treatments including chemotherapy and immune checkpoint inhibitors (ICIs) have emerged as promising therapeutic approaches. However, patients’ responses vary due to the heterogeneity of individuals, distinct characteristics of their BC, and the tumor microenvironment. The predictive factors for anti-BC immunotherapeutic response are still insufficient (Vitorino et al., 2021). Recently, BC and anti-BC treatment has shown bidirectional interaction with gut microbiota. For examples, accumulating evidence suggests that the gut microbiota modulates hormone therapy efficacy in HR+BC. Firstly, gut microbiota influences immune tone, therefore impacting the antitumor immunity triggered by hormone therapy.
Secondly, gut bacteria are capable of synthesizing, recycling, or degrading estrogens, thus influencing cancer cell-supportive hormone concentrations. Specifically, an overabundance of Veillonella genus bacteria has been observed in women with endocrine-resistant HR+ BC (Terrisse et al., 2023; Lasagna et al., 2022). 16S rRNA sequencing indicated that acupuncture treatment in CRF mice after BC chemotherapy increased Candidatus Arthromitus, Lactobacillus, and Clostridia_UCG-014_unclassified abundances, and reduced Escherichia-Shigella, Burkholderia-Caballeronia-Paraburkholderia, and Streptococcus abundances. Therefore, gut microbiota-gut-brain axis is impacted by BC chemotherapy (Lv et al., 2022). Chemotherapy is vital for regulating fecal metabolomic profiles in BC cases. Amino acids show up-regulation, while lactate and fumaric acid exhibit down-regulation among patients in second and third cycles compared with those prior to treatment. SCFAs displayed significant differentiation among those studied groups (Zidi et al., 2021). Mice harboring special microbial species, including Akkermansia muciniphila, Bifidobacterium longum, Collinsella aerofaciens, and Faecalibacterium prausnitzii, exhibited a more favorable response to anti-PD-(L)1 therapy (Vitorino et al., 2021). Commensal microbiota with abundance of B. longum, Collinsella aerofaciens, and E. faecium was related to anti-PD-1 therapeutic effect on cancers (Matson et al., 2018; Alpuim Costa et al., 2021). A direct correlation is found between the levels of A. muciniphila in fecal samples and the efficacy of anti-PD-1 therapy. Therefore, pharmacomicrobiomics research may promote using gut microbiota analysis for predicting ICI response in patients, contributing to the personalized and precision medicine for cancers (Vitorino et al., 2021).
Gut microbiome may influence tumor growth and the effectiveness of cancer treatment, making it a viable target for both tumor prevention and treatment strategies (Zhang et al., 2022; Muhammad et al., 2024). Currently, fecal microbiota transplantation is the primary approach for intestinal microbial therapy. Another method to restore the balance of intestinal microecological environment is using probiotics that holds significant promise as an antitumor strategy, including BC (Eslami et al., 2019; Thu et al., 2023; Zhang et al., 2023d). The improved therapeutic effect in mice receiving fecal microbiota transplantation (FMT) with responder feces was associated with the augmented CD45+and CD8+T cell activation in intestinal region (Routy et al., 2018; Vitorino et al., 2021; Alpuim Costa et al., 2021). Several studies have indicated an increased risk of immune-mediated toxicity in patients harboring specific bacteria, such as Bacteroidaceae, Barnesiellaceae, and Rikenellaceae (Vitorino et al., 2021; Chaput et al., 2017). This suggested that intestinal microbiota metabolites may be used for the treatment of BC cells. Toshitaka Odamaki et al. conducted a preliminary investigation using a randomized controlled trial design. Participants consumed yogurt containing the probiotic strain Bifidobacterium longum BB536 (BB536Y group), while the control group was provided with regular milk. The group consuming probiotic yogurt showed significantly reduced enterotoxigenic Bacteroides fragilis (ETBF) abundance in gut microbiome. This suggests that probiotic yogurt may have the potential to eliminate ETBF from the microbial community, potentially lowering the risk of breast hyperplasia and BC progression to some extent (Odamaki et al., 2012). Zhang et al. confirmed that gut microbiota can be regulated by Lacticaseibacillus rhamnosus Probio-M9 (Probio-M9), efficiently inhibiting BC development in BC cell-transplanted mice (Zhang et al., 2022). In overweight BC patients, probiotic treatment can substantially change composition and diversity of the intestinal flora, particularly the phyla Bacteroidetes and Firmicutes (Feng et al., 2021; Pellegrini et al., 2020). It was reported that Probiotic bacteria might be the candidate anti-BC prevention strategy among menopausal women. Dietary fiber, the well-known prebiotic type, can be fermented by gut bacteria producing cancer-protective metabolites like butyrate. Currently, some intervention clinical trials are under way to study how new probiotics can affect breast and intestinal microbiota in cancer patients and life quality in BC patients (Ruo et al., 2021). For examples, herbal supplements including American ginseng generate cancer-protective metabolites and modulate gut microbial composition for preventing tumor genesis (Rea et al., 2018). Ganoderma lucidum spores also regulate gut microbiota and recover normobiosis (Su et al., 2018). Maternal GE use can effectively prevent obesity-related metabolic disorders and BC subsequently in life through the dynamic influence on the interaction of early-life gut microbiota with important microbial metabolite profiles and offspring epigenome (Chen et al., 2022a). Jessica R Lakritz et al. demonstrated that consuming fermentative microbes including Lactobacillus reuteri may inhibit mammary carcinogenesis in animal model through stimulating lymphocytes (Lakritz et al., 2014).
However, Alastair proposed that gut microbial disruption by antibiotics negatively influences BC development and outcomes. The loss of beneficial microbial species induced by antibiotics may accelerate tumor development by changing mast cell homing and/or function (Mckee et al., 2021). Preexisting dysbiosis triggered by antibiotics is the host-intrinsic regulatory factor for tumor cell invasion and tissue inflammation in BC (Zhang et al., 2021). As discovered by Shiao et al., gut bacteria depletion using the cocktail containing ampicillin, imipenem, cilastatin, and vancomycin prior to radio therapy accelerated tumor development and reduced the survival in tumor-bearing mice compared with RT alone (Shiao et al., 2021; Zhao et al., 2023). It is indicated that gut microbiota affects the radiotherapy effect, even though such effect is negative.
It is of note that microbiota manipulation is applicable for improving therapeutic outcomes of BC patients. LCA acted as intestinal bacterial derivatives, and decreased SREBP-1c, FASN and ACACA levels in BC cells, exhibiting anti-proliferative and pro-apoptotic effects (Luu et al., 2018). It can also induce apoptosis in MCF‐7 cells through the binding to receptor G protein‐coupled bile acid receptor 1 (GPBAR1) and prevent epithelial-to-mesenchymal transition in BC cells by suppressing vascular endothelial growth factor (VEGF) expression (Trah et al., 2020; Yang et al., 2023; Chattopadhyay et al., 2022; Mikó et al., 2019). S-equol, the specific enantiomeric configuration of soy isoflavone metabolite generated by the human intestinal microbiota, effectively inhibited MCF-7 BC cell growth in both time- and dose-dependent manners. Moreover, S-equol facilitated apoptosis in MCF-7 cells by upregulating miR-10a-5p expression and inhibiting the PI3K/AKT pathway (Zhang et al., 2019). Metabolites derived from the Faecalibacterium genus exert growth-inhibitory efficacy in BC cells through inhibiting IL-6/STAT3 signaling pathway (Ma et al., 2020; Yang et al., 2023). As a highly abundant SCFAs generated in gut microbiota, butyrate has presented with potential anticancer activity against BC. It can be generated naturally as the bacterial fermentation by-product of fermentable and non-digestible carbohydrates, like dietary fibers, in GIT. Particularly, low butyrate content can cause tumorigenesis in host, while high content can suppress tumorigenesis and tumor development (Jaye et al., 2022). Faecalibacterium prausnitzii can inhibit BC cell development by inhibiting IL6/STAT3 pathway; thus, more efforts are needed to develop the candidate intervention (Ma et al., 2020). In breast and intestinal environment, colonization of enterotoxigenic Bacteroides fragilis (ETBF) can secrete a toxin called Bacteroides fragilis toxin (BFT), which rapidly induces proliferation of mammary ductal tissues (usually presenting as precancerous lesions of BC). This suggests that enterotoxigenic Bacteroides fragilis (ETBF) in the gut can be another possible risk factor related to BC development (Parida et al., 2021). In fact, gut microbial species are suggested to translocate to breast tissue, and it is vital for maintaining breast health. Such translocation may occur through several pathways, such as nipple-oral contact through lactation (Jaye et al., 2022; Hieken et al., 2016). Interestingly, recent research has demonstrated significant changes in oral and gut bacterial community compositions in dogs impacted by canine mammary tumors. Specifically, there was a substantial increase in Bacteroides, serving as an important microbial biomarker for oral and gut bacterial communities. This suggests a potential migration of microbiota from the mouth to the intestine and finally reaching distant mammary tumor tissue. This study presents the novel microbiological concept for treating canine mammary tumors, also providing a theoretical basis for the study of human BC (Zheng et al., 2022).
Thus, more recent information will facilitate thinking of the correlation between the interactions of oral and gut microbiota and genesis, development, clinical treatment, and prognosis in BC. Similarly, BC may also influence the body’s microbiota, including the microbial population of the oral-gut axis. Oral cavity is the most common entry point for external substances in the body. Different foods, medications, and even breastfeeding during lactation period could affect normal distribution of microbial communities within oral cavity. In specific situations, certain external or oral microorganisms may overcome barriers between oral and gut environments and potentially migrate to intestines, thereby affecting the gut microbiota’s ecological balance. This leads to changes in the composition of gut bacterial communities. Gut microbiota and the metabolites may participate in the occurrence and progression of BC through various pathways, including influencing epithelial-mesenchymal transition, epigenetic processes, inflammatory responses in the microenvironment, estrogen production, and more. This eventually reaches breast tumor tissues. The microbial ecosystem surrounding breast tumor tissues may also undergo certain changes. Gut microbes show direct or indirect effects on oral microbial composition by regulating host’s immune function. In addition, at different stages of BC occurrence, development, and clinical treatment, the cancer itself may also cause alterations of oral and gut microbial compositions. Although research related to this topic still faces a lot of limitations, it is believed that more mechanisms will be elucidated in the future with technological advancements and increased attention to the association of oral-gut axis with microbiota in BC patients (Figure 3).
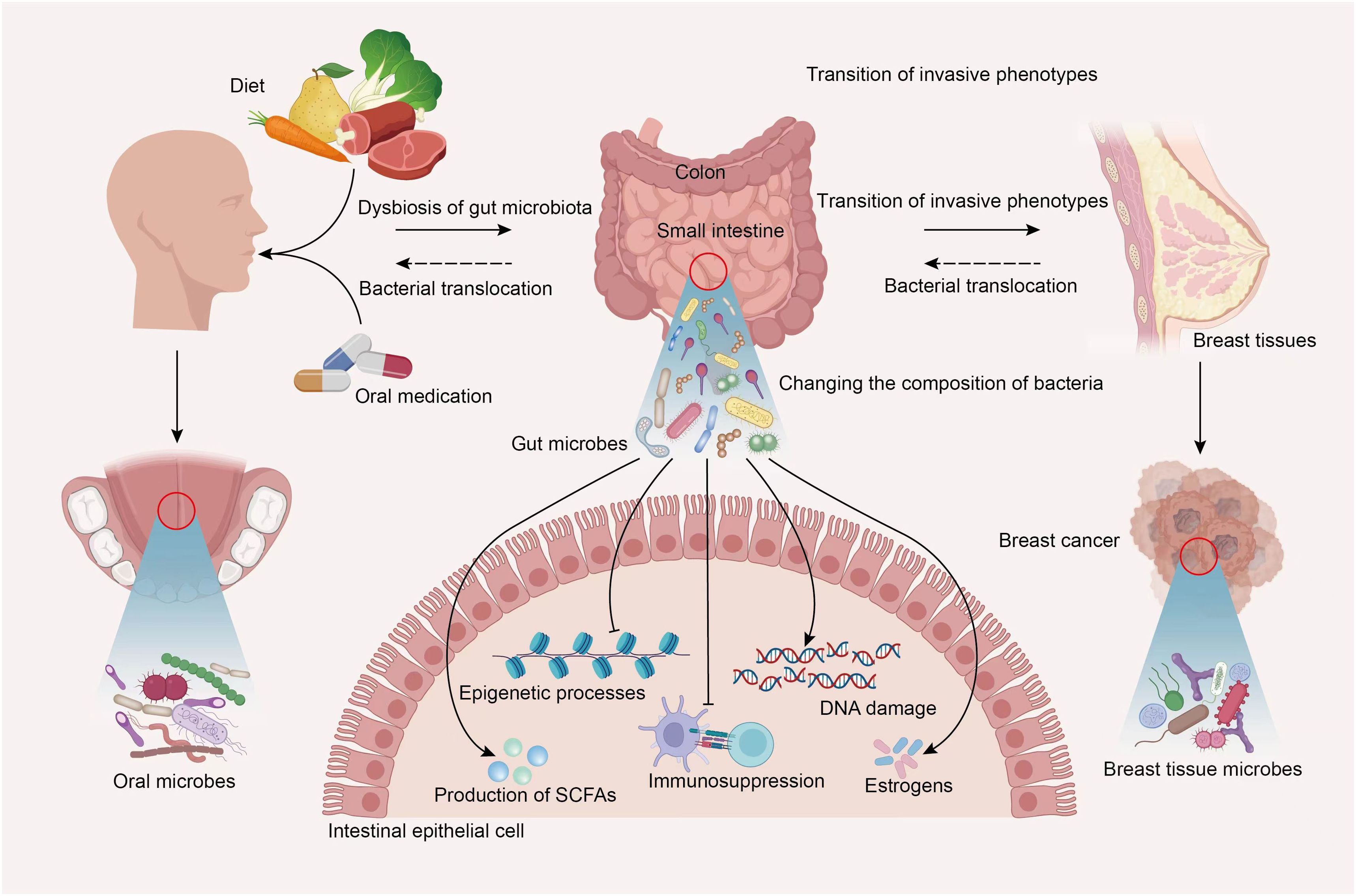
Figure 3. Hypothetical diagram of the interplay of microbiota within the oral-gut axis in BC. In specific situations, the oral microbiota profile could be significantly altered by diet, oral medication and more, certain external or oral microorganisms may overcome barriers between oral and gut environments and potentially migrate to intestines, thereby affecting the gut microbiota’s ecological balance. Gut microbiota and the metabolites may participate in the occurrence and progression of BC through various pathways, including influencing epithelial-mesenchymal transition, the transition of invasive phenotypes, DNA damage, epigenetic processes, inflammatory responses in the microenvironment, estrogen and short-chain fatty acids production, and more. This eventually reaches breast tumor tissues. The microbial ecosystem surrounding breast tumor tissues may also undergo certain changes. Gut microbes show direct or indirect effects on oral microbial composition by regulating host’s immune function. In addition, at different stages of BC occurrence, development, and clinical treatment, the cancer itself may also cause alterations of oral and gut microbial compositions.
4 Conclusion and perspectives
In conclusion, changes of gut and oral microbiota may facilitate the BC pathogenesis and its therapeutic treatment options. In addition, oral administration of probiotics or therapeutic drugs may provide an alternative approach to modulating gut microbiota. Monitoring changes of gut and oral microbiota can provide new opportunities for predicting, understanding, and treating BC. Existing research suggests that although the interplay of gut-oral axis microbiota with BC occurrence and treatment is not as well-established as the association of gut microbiota with gastrointestinal cancers, tumor microecology is inherently a complex network where microbial shifts and interactions often occur unexpectedly. As a direction in tumor microecology research, the study of microbiota in the gut-oral axis still holds significant prospects and research opportunities.
Author contributions
LH: Writing – original draft. CJ: Writing – original draft. MY: Writing – original draft. WW: Writing – original draft. SL: Writing – original draft. ZX: Writing – review & editing. JW: Writing – review & editing.
Funding
The author(s) declare financial support was received for the research, authorship, and/or publication of this article. This study was supported by the National Natural Science Foundation of China (No.82302626 and No. 82272396), Suzhou Medical and Health Science and Technology Innovation Project (No. SKY2022057 and KJXW2021043) and Gusu Health Talent Project of Suzhou (No. GSWS2022076).
Conflict of interest
The authors declare that the research was conducted in the absence of any commercial or financial relationships that could be construed as a potential conflict of interest.
Publisher’s note
All claims expressed in this article are solely those of the authors and do not necessarily represent those of their affiliated organizations, or those of the publisher, the editors and the reviewers. Any product that may be evaluated in this article, or claim that may be made by its manufacturer, is not guaranteed or endorsed by the publisher.
References
Alpuim Costa, D., Nobre, J. G., Batista, M. V., Ribeiro, C., Calle, C., Cortes, A., et al. (2021). Human microbiota and breast cancer-is there any relevant link?-A literature review and new horizons toward personalised medicine. Front. Microbiol. 12, 584332. doi: 10.3389/fmicb.2021.584332
Alvarez-Mercado, A. I., Del Valle Cano, A., Fernandez, M. F., Fontana, L. (2023). Gut microbiota and breast cancer: the dual role of microbes. Cancers (Basel) 15 (2), 443. doi: 10.3390/cancers15020443
Anderson, B. O., Ilbawi, A. M., Fidarova, E., Weiderpass, E., Stevens, L., Abdel-Wahab, M., et al. (2021). The global breast cancer initiative: A strategic collaboration to strengthen health care for non-communicable diseases. Lancet Oncol. 22, 578–581. doi: 10.1016/S1470-2045(21)00071-1
Arnold, M., Morgan, E., Rumgay, H., Mafra, A., Singh, D., Laversanne, M., et al. (2022). Current and future burden of breast cancer: global statistics for 2020 and 2040. Breast 66, 15–23. doi: 10.1016/j.breast.2022.08.010
Arnone, A. A., Cook, K. L. (2022). Gut and breast microbiota as endocrine regulators of hormone receptor-positive breast cancer risk and therapy response. Endocrinology 164, 15-23 doi: 10.1210/endocr/bqac177
Asili, P., Mirahmad, M., Rezaei, P., Mahdavi, M., Larijani, B., Tavangar, S. M. (2023). The association of oral microbiome dysbiosis with gastrointestinal cancers and its diagnostic efficacy. J. Gastrointest Cancer. 54 (4), 1082–1101. doi: 10.1007/s12029-022-00901-4
Atarashi, K., Suda, W., Luo, C., Kawaguchi, T., Motoo, I., Narushima, S., et al. (2017). Ectopic colonization of oral bacteria in the intestine drives T(H)1 cell induction and inflammation. Science 358, 359–365. doi: 10.1126/science.aan4526
Barrea, L., Verde, L., Auriemma, R. S., Vetrani, C., Cataldi, M., Frias-Toral, E., et al. (2023). Probiotics and prebiotics: any role in menopause-related diseases? Curr. Nutr. Rep. 12, 83–97. doi: 10.1007/s13668-023-00462-3
Bastos, A. R., Pereira-Marques, J., Ferreira, R. M., Figueiredo, C. (2023). Harnessing the microbiome to reduce pancreatic cancer burden. Cancers (Basel) 15 (9), 2629. doi: 10.3390/cancers15092629
Belkaid, Y., Hand, T. W. (2014). Role of the microbiota in immunity and inflammation. Cell 157, 121–141. doi: 10.1016/j.cell.2014.03.011
Blanco-Miguez, A., Marcos-Fernandez, R., Guadamuro-Garcia, L., Fdez-Riverola, F., Cubiella, J., Lourenco, A., et al. (2023). Targeted depletion of pks+ Bacteria from A fecal microbiota using specific antibodies. Msystems 8 (3), E0007923. doi: 10.1128/msystems.00079-23
Bobin-Dubigeon, C., Luu, H. T., Leuillet, S., Lavergne, S. N., Carton, T., Le Vacon, F., et al. (2021). Faecal microbiota composition varies between patients with breast cancer and healthy women: A comparative case-control study. Nutrients 13 (8), 2705. doi: 10.3390/nu13082705
Bouter, K. E., Van Raalte, D. H., Groen, A. K., Nieuwdorp, M. (2017). Role of the gut microbiome in the pathogenesis of obesity and obesity-related metabolic dysfunction. Gastroenterology 152, 1671–1678. doi: 10.1053/j.gastro.2016.12.048
Boyanova, L., Markovska, R., Yordanov, D., Gergova, R., Hadzhiyski, P. (2023). Anaerobes in specific infectious and noninfectious diseases: new developments. Anaerobe 81, 102714. doi: 10.1016/j.anaerobe.2023.102714
Bronte-Tinkew, D. M., Terebiznik, M., Franco, A., Ang, M., Ahn, D., Mimuro, H., et al. (2009). Helicobacter pylori cytotoxin-associated gene A activates the signal transducer and activator of transcription 3 pathway in vitro and in vivo. Cancer Res. 69, 632–639. doi: 10.1158/0008-5472.CAN-08-1191
Buchta Rosean, C., Bostic, R. R., Ferey, J. C. M., Feng, T. Y., Azar, F. N., Tung, K. S., et al. (2019). Preexisting commensal dysbiosis is A host-intrinsic regulator of tissue inflammation and tumor cell dissemination in hormone receptor-positive breast cancer. Cancer Res. 79, 3662–3675. doi: 10.1158/0008-5472.CAN-18-3464
Bui, D., Brown, H. E., Harris, R. B., Oren, E. (2016). Serologic evidence for fecal-oral transmission of helicobacter pylori. Am. J. Trop. Med. Hyg 94, 82–88. doi: 10.4269/ajtmh.15-0297
Cazzaniga, M., Zonzini, G. B., Di Pierro, F., Moricoli, S., Bertuccioli, A. (2022). Gut microbiota, metabolic disorders and breast cancer: could berberine turn out to be A transversal nutraceutical tool? A narrative analysis. Int. J. Mol. Sci. 23 (20), 12538. doi: 10.3390/ijms232012538
Chai, Y., Huang, Z., Shen, X., Lin, T., Zhang, Y., Feng, X., et al. (2023). Microbiota regulates pancreatic cancer carcinogenesis through altered immune response. Microorganisms 11 (5), 1240. doi: 10.3390/microorganisms11051240
Chapman, C. M. L., Kapinos, A., Rivera-Chavez, F. (2023). Modulation of host-microbe metabolism by cholera toxin. Infect. Immun. 91, E0043522. doi: 10.1128/iai.00435-22
Chaput, N., Lepage, P., Coutzac, C., Soularue, E., Le Roux, K., Monot, C., et al. (2017). Baseline gut microbiota predicts clinical response and colitis in metastatic melanoma patients treated with ipilimumab. Ann. Oncol. 28, 1368–1379. doi: 10.1093/annonc/mdx108
Chattopadhyay, I., Gundamaraju, R., Jha, N. K., Gupta, P. K., Dey, A., Mandal, C. C., et al. (2022). Interplay between dysbiosis of gut microbiome, lipid metabolism, and tumorigenesis: can gut dysbiosis stand as A prognostic marker in cancer? Dis. Markers 2022, 2941248. doi: 10.1155/2022/2941248
Chen, M., Li, S., Arora, I., Yi, N., Sharma, M., Li, Z., et al. (2022a). Maternal soybean diet on prevention of obesity-related breast cancer through early-life gut microbiome and epigenetic regulation. J. Nutr. Biochem. 110, 109119. doi: 10.1016/j.jnutbio.2022.109119
Chen, X., Bai, X., Xie, X., Huang, J., Chen, L., Song, L., et al. (2023). The anti-tumor efficiency of low-dose apatinib-based chemotherapy in pretreated her2-negative breast cancer with brain metastases. Ann. Med. 55, 2218647. doi: 10.1080/07853890.2023.2218647
Chen, X., Wang, N., Wang, J., Liao, B., Cheng, L., Ren, B. (2022b). The interactions between oral-gut axis microbiota and helicobacter pylori. Front. Cell Infect. Microbiol. 12, 914418. doi: 10.3389/fcimb.2022.914418
Dabek, M., Mccrae, S. I., Stevens, V. J., Duncan, S. H., Louis, P. (2008). Distribution of beta-glucosidase and beta-glucuronidase activity and of beta-glucuronidase gene gus in human colonic bacteria. FEMS Microbiol. Ecol. 66, 487–495. doi: 10.1111/j.1574-6941.2008.00520.x
De Graaf, M., Beck, R., Caccio, S. M., Duim, B., Fraaij, P., Le Guyader, F. S., et al. (2017). Sustained fecal-oral human-to-human transmission following A zoonotic event. Curr. Opin. Virol. 22, 1–6. doi: 10.1016/j.coviro.2016.11.001
Elghannam, M. T., Hassanien, M. H., Ameen, Y. A., Turky, E. A., Elattar, G. M., Elray, A. A., et al. (2023). Oral microbiota and liver diseases. Clin. Nutr. Espen 54, 68–72. doi: 10.1016/j.clnesp.2022.12.030
Eltay, E. G., Van Dyke, T. (2023). Resolution of inflammation in oral diseases. Pharmacol. Ther. 247, 108453. doi: 10.1016/j.pharmthera.2023.108453
Endogenous, H., Breast Cancer Collaborative, G, Key, T. J., Appleby, P. N., Reeves, G. K., Roddam, A. W., et al. (2011). Circulating sex hormones and breast cancer risk factors in postmenopausal women: reanalysis of 13 studies. Br. J. Cancer 105, 709–722. doi: 10.1038/bjc.2011.254
Eslami, M., Yousefi, B., Kokhaei, P., Hemati, M., Nejad, Z. R., Arabkari, V., et al. (2019). Importance of probiotics in the prevention and treatment of colorectal cancer. J. Cell Physiol. 234, 17127–17143. doi: 10.1002/jcp.v234.10
Fan, Z., Tang, P., Li, C., Yang, Q., Xu, Y., Su, C., et al. (2023). Fusobacterium nucleatum and its associated systemic diseases: epidemiologic studies and possible mechanisms. J. Oral. Microbiol. 15, 2145729. doi: 10.1080/20002297.2022.2145729
Fatima, F., Chourasiya, N. K., Mishra, M., Kori, S., Pathak, S., Das, R., et al. (2023). Curcumin and its derivatives targeting multiple signaling pathways to elicit anticancer activity: A comprehensive perspective. Curr. Med. Chem. 31 (24), 3668–3714. doi: 10.2174/0929867330666230522144312
Feng, Y., Duan, T. J., Huang, Q., Li, Z. Y., Liu, Y. P., Luo, M. S., et al. (2023). The clinicopathological characteristics of gastric cancer and precancerous conditions in gastric dlbcl and malt lymphoma patients: A multi-center retrospective study. Ann. Med. 55, 2193423. doi: 10.1080/07853890.2023.2193423
Feng, Z. P., Xin, H. Y., Zhang, Z. W., Liu, C. G., Yang, Z., You, H., et al. (2021). Gut microbiota homeostasis restoration may become A novel therapy for breast cancer. Invest. New Drugs 39, 871–878. doi: 10.1007/s10637-021-01063-z
Fernández, M. F., Reina-Pérez, I., Astorga, J. M., Rodríguez-Carrillo, A., Plaza-Díaz, J., Fontana, L. (2018). Breast cancer and its relationship with the microbiota. Int. J. Environ. Res. Public Health 15 (8), 1747. doi: 10.3390/ijerph15081747
Freudenheim, J. L., Genco, R. J., Lamonte, M. J., Millen, A. E., Hovey, K. M., Mai, X., et al. (2016). Periodontal disease and breast cancer: prospective cohort study of postmenopausal women. Cancer Epidemiol. Biomarkers Prev. 25, 43–50. doi: 10.1158/1055-9965.EPI-15-0750
Fujiwara, N., Kitamura, N., Yoshida, K., Yamamoto, T., Ozaki, K., Kudo, Y. (2020). Involvement of fusobacterium species in oral cancer progression: A literature review including other types of cancer. Int. J. Mol. Sci. 21 (17), 6207. doi: 10.3390/ijms21176207
Gaba, F. I., Gonzalez, R. C., Martinez, R. G. (2022). The role of oral fusobacterium nucleatum in female breast cancer: A systematic review and meta-analysis. Int. J. Dent. 2022, 1876275. doi: 10.1155/2022/1876275
Gaiser, R. A., Halimi, A., Alkharaan, H., Lu, L., Davanian, H., Healy, K., et al. (2019). Enrichment of oral microbiota in early cystic precursors to invasive pancreatic cancer. Gut 68, 2186–2194. doi: 10.1136/gutjnl-2018-317458
Geng, W., Cao, M., Dong, K., An, J., Gao, H. (2023). Shoc2 mediates the drug-resistance of triple-negative breast cancer cells to everolimus. Cancer Biol. Ther. 24, 2206362. doi: 10.1080/15384047.2023.2206362
Gevers, D., Kugathasan, S., Denson, L. A., Vazquez-Baeza, Y., Van Treuren, W., Ren, B., et al. (2014). The treatment-naive microbiome in new-onset crohn’s disease. Cell Host Microbe 15, 382–392. doi: 10.1016/j.chom.2014.02.005
Gori, S., Inno, A., Belluomini, L., Bocus, P., Bisoffi, Z., Russo, A., et al. (2019). Gut microbiota and cancer: how gut microbiota modulates activity, efficacy and toxicity of antitumoral therapy. Crit. Rev. Oncol. Hematol. 143, 139–147. doi: 10.1016/j.critrevonc.2019.09.003
Guo, S., Li, L., Xu, B., Li, M., Zeng, Q., Xiao, H., et al. (2018). A simple and novel fecal biomarker for colorectal cancer: ratio of fusobacterium nucleatum to probiotics populations, based on their antagonistic effect. Clin. Chem. 64, 1327–1337. doi: 10.1373/clinchem.2018.289728
Hao, Y., Peng, X., Zhou, X. D., Cheng, L. (2019). Research progress on the relationship between periodontal disease and common Malignancies. Hua Xi Kou Qiang Yi Xue Za Zhi 37 (3), 320–324. doi: 10.7518/hxkq.2019.03.018
Haque, S., Raina, R., Afroze, N., Hussain, A., Alsulimani, A., Singh, V., et al. (2022). Microbial dysbiosis and epigenetics modulation in cancer development - A chemopreventive approach. Semin. Cancer Biol. 86, 666–681. doi: 10.1016/j.semcancer.2021.06.024
Hasuda, H., Ikeda, T., Makizaki, Y., Yokota, H., Tanaka, Y., Ohno, H., et al. (2023). Alterations in the gut microbiome in patients with esophageal carcinoma in response to esophagectomy and neoadjuvant treatment. Surg. Today 53, 663–674. doi: 10.1007/s00595-022-02607-3
He, C., Liu, Y., Ye, S., Yin, S., Gu, J. (2021). Changes of intestinal microflora of breast cancer in premenopausal women. Eur. J. Clin. Microbiol. Infect. Dis. 40, 503–513. doi: 10.1007/s10096-020-04036-x
Heer, E., Harper, A., Escandor, N., Sung, H., Mccormack, V., Fidler-Benaoudia, M. M. (2020). Global burden and trends in premenopausal and postmenopausal breast cancer: A population-based study. Lancet Glob Health 8, E1027–E1037. doi: 10.1016/S2214-109X(20)30215-1
Helmink, B. A., Khan, M. A. W., Hermann, A., Gopalakrishnan, V., Wargo, J. A. (2019). The microbiome, cancer, and cancer therapy. Nat. Med. 25, 377–388. doi: 10.1038/s41591-019-0377-7
Hieken, T. J., Chen, J., Hoskin, T. L., Walther-Antonio, M., Johnson, S., Ramaker, S., et al. (2016). The microbiome of aseptically collected human breast tissue in benign and Malignant disease. Sci. Rep. 6, 30751. doi: 10.1038/srep30751
Hill, C., Guarner, F., Reid, G., Gibson, G. R., Merenstein, D. J., Pot, B., et al. (2014). Expert consensus document. The international scientific association for probiotics and prebiotics consensus statement on the scope and appropriate use of the term probiotic. Nat. Rev. Gastroenterol. Hepatol. 11, 506–514. doi: 10.1038/nrgastro.2014.66
Horigome, A., Okubo, R., Hamazaki, K., Kinoshita, T., Katsumata, N., Uezono, Y., et al. (2019). Association between blood omega-3 polyunsaturated fatty acids and the gut microbiota among breast cancer survivors. Benef Microbes 10, 751–758. doi: 10.3920/BM2019.0034
Hudson, A. W., Barnes, A. J., Bray, A. S., Ornelles, D. A., Zafar, M. A. (2022). Klebsiella pneumoniae L-fucose metabolism promotes gastrointestinal colonization and modulates its virulence determinants. Infect. Immun. 90, E0020622. doi: 10.1128/iai.00206-22
Imhann, F., Bonder, M. J., Vich Vila, A., Fu, J., Mujagic, Z., Vork, L., et al. (2016). Proton pump inhibitors affect the gut microbiome. Gut 65, 740–748. doi: 10.1136/gutjnl-2015-310376
Issrani, R., Reddy, R. J., El-Metwally, T. H., Prabhu, N. (2021). Periodontitis as A risk factor for breast cancer - what we know till date? Asian Pac J. Cancer Prev. 22 (10), 3109–3114. doi: 10.31557/APJCP.2021.22.10.3109
Iwauchi, M., Horigome, A., Ishikawa, K., Mikuni, A., Nakano, M., Xiao, J. Z., et al. (2019). Relationship between oral and gut microbiota in elderly people. Immun. Inflammation Dis. 7, 229–236. doi: 10.1002/iid3.2019.7.issue-3
Javier-Desloges, J., Mckay, R. R., Swafford, A. D., Sepich-Poore, G. D., Knight, R., Parsons, J. K. (2022). The microbiome and prostate cancer. Prostate Cancer Prostatic Dis. 25, 159–164. doi: 10.1038/s41391-021-00413-5
Jaye, K., Chang, D., Li, C. G., Bhuyan, D. J. (2022). Gut metabolites and breast cancer: the continuum of dysbiosis, breast cancer risk, and potential breast cancer therapy. Int. J. Mol. Sci. 23 (16), 9490. doi: 10.3390/ijms23169490
Jaye, K., Li, C. G., Bhuyan, D. J. (2021). The complex interplay of gut microbiota with the five most common cancer types: from carcinogenesis to therapeutics to prognoses. Crit. Rev. Oncol. Hematol. 165, 103429. doi: 10.1016/j.critrevonc.2021.103429
Jeong, J., Ahn, K., Mun, S., Yun, K., Kim, Y. T., Jung, W., et al. (2023). Understanding the bacterial compositional network associations between oral and gut microbiome within healthy koreans. J. Oral. Microbiol. 15, 2186591. doi: 10.1080/20002297.2023.2186591
Jia, M., Wu, Z., Vogtmann, E., O’brien, K. M., Weinberg, C. R., Sandler, D. P., et al. (2020). The association between periodontal disease and breast cancer in A prospective cohort study. Cancer Prev. Res. (Phila) 13, 1007–1016. doi: 10.1158/1940-6207.CAPR-20-0018
Kageyama, S., Sakata, S., Ma, J., Asakawa, M., Takeshita, T., Furuta, M., et al. (2023). High-resolution detection of translocation of oral bacteria to the gut. J. Dent. Res. 102, 752–758. doi: 10.1177/00220345231160747
Kato, I., Minkevitch, J., Sun, J. (2023). Oncogenic potential of campylobacter infection in the gastrointestinal tract: narrative review. Scand. J. Gastroenterol. 58 (12), 1–13. doi: 10.1080/00365521.2023.2228954
Kim, H. E., Kim, J., Maeng, S., Oh, B., Hwang, K. T., Kim, B. S. (2021). Microbiota of breast tissue and its potential association with regional recurrence of breast cancer in korean women. J. Microbiol. Biotechnol. 31, 1643–1655. doi: 10.4014/jmb.2106.06039
Klymiuk, I., Bilgilier, C., Mahnert, A., Prokesch, A., Heininger, C., Brandl, I., et al. (2022). Chemotherapy-associated oral microbiome changes in breast cancer patients. Front. Oncol. 12, 949071. doi: 10.3389/fonc.2022.949071
Kong, J., Liu, Y., Qian, M., Xing, L., Gao, S. (2023). The relationship between porphyromonas gingivalis and oesophageal squamous cell carcinoma: A literature review. Epidemiol. Infect. 151, E69. doi: 10.1017/S0950268823000298
Kost, Y., Rzepecki, A. K., Deutsch, A., Birnbaum, M. R., Ohri, N., Hosgood, H. D., et al. (2023). Association of staphylococcus aureus colonization with severity of acute radiation dermatitis in patients with breast or head and neck cancer. JAMA Oncol. 9 (7), 962–965. doi: 10.1001/jamaoncol.2023.0454
Koual, M., Tomkiewicz, C., Cano-Sancho, G., Antignac, J. P., Bats, A. S., Coumoul, X. (2020). Environmental chemicals, breast cancer progression and drug resistance. Environ. Health 19, 117. doi: 10.1186/s12940-020-00670-2
Kovács, T., Mikó, E., Vida, A., Sebő, É., Toth, J., Csonka, T., et al. (2019). Cadaverine, A metabolite of the microbiome, reduces breast cancer aggressiveness through trace amino acid receptors. Sci. Rep. 9 (1), 1300. doi: 10.1038/s41598-018-37664-7
Kwon, Y. J., Seo, E. B., Kim, S. K., Lee, H. S., Lee, H., Jang, Y. A., et al. (2023). Pharmacological anti-tumor effects of natural chamaecyparis obtusa (Siebold & Zucc.) endl. Leaf extracts on breast cancer. J. Ethnopharmacol 313, 116598. doi: 10.1016/j.jep.2023.116598
Lakritz, J. R., Poutahidis, T., Levkovich, T., Varian, B. J., Ibrahim, Y. M., Chatzigiagkos, A., et al. (2014). Beneficial bacteria stimulate host immune cells to counteract dietary and genetic predisposition to mammary cancer in mice. Int. J. Cancer 135, 529–540. doi: 10.1002/ijc.v135.3
Lam, G. A., Albarrak, H., Mccoll, C. J., Pizarro, A., Sanaka, H., Gomez-Nguyen, A., et al. (2022). The oral-gut axis: periodontal diseases and gastrointestinal disorders. Inflammation Bowel Dis. 29 (7), 1153–1164. doi: 10.1093/ibd/izac241
Lasagna, A., De Amici, M., Rossi, C., Zuccaro, V., Corbella, M., Petazzoni, G., et al. (2022). The bio-diversity and the role of gut microbiota in postmenopausal women with luminal breast cancer treated with aromatase inhibitors: an observational cohort study. Pathogens 11 (12), 1421. doi: 10.3390/pathogens11121421
Lee, K. M., Lee, M., Lee, J., Kim, S. W., Moon, H. G., Noh, D. Y., et al. (2016). Enhanced anti-tumor activity and cytotoxic effect on cancer stem cell population of metformin-butyrate compared with metformin hcl in breast cancer. Oncotarget 7, 38500–38512. doi: 10.18632/oncotarget.v7i25
Li, R., Hu, Y., Hou, S. (2022). An exploration of oral-gut pathogens mediating immune escape of pancreatic cancer via mir-21/pten axis. Front. Microbiol. 13, 928846. doi: 10.3389/fmicb.2022.928846
Liu, F., Gao, A., Zhang, M., Li, Y., Zhang, F., Herman, J. G., et al. (2024). Methylation of fam110c is A synthetic lethal marker for atr/chk1 inhibitors in pancreatic cancer. J. Of Trans. Internal Med. 12, 274–287. doi: 10.2478/jtim-2023-0128
Liu, F., Su, D., Zhang, H., Lin, H. C., Zhou, Q., Cao, B., et al. (2022). Clinical implications of the oral−Gut microbiome axis and its association with colorectal cancer (Review). Oncol. Rep. 48 (5), 192. doi: 10.3892/or.2022.8407
Loman, B. R., Russart, K. L. G., Grant, C. V., Lynch, A. J., Bailey, M. T., Pyter, L. M. (2022). Mammary tumors alter the fecal bacteriome and permit enteric bacterial translocation. BMC Cancer 22, 245. doi: 10.1186/s12885-022-09274-0
Lu, X., Wang, X., Cheng, H., Wang, X., Liu, C., Tan, X. (2023). Anti-triple-negative breast cancer metastasis efficacy and molecular mechanism of the sting agonist for innate immune pathway. Ann. Med. 55, 2210845. doi: 10.1080/07853890.2023.2210845
Luu, T. H., Bard, J. M., Carbonnelle, D., Chaillou, C., Huvelin, J. M., Bobin-Dubigeon, C., et al. (2018). Lithocholic bile acid inhibits lipogenesis and induces apoptosis in breast cancer cells. Cell Oncol. (Dordr) 41, 13–24. doi: 10.1007/s13402-017-0353-5
Lv, Z., Liu, R., Su, K., Gu, Y., Fang, L., Fan, Y., et al. (2022). Acupuncture ameliorates breast cancer-related fatigue by regulating the gut microbiota-gut-brain axis. Front. Endocrinol. (Lausanne) 13, 921119. doi: 10.3389/fendo.2022.921119
Ma, Z., Qu, M., Wang, X. (2022). Analysis of gut microbiota in patients with breast cancer and benign breast lesions. Pol. J. Microbiol. 71, 217–226. doi: 10.33073/pjm-2022-019
Ma, J., Sun, L., Liu, Y., Ren, H., Shen, Y., Bi, F., et al. (2020). Alter between gut bacteria and blood metabolites and the anti-tumor effects of faecalibacterium prausnitzii in breast cancer. BMC Microbiol. 20, 82. doi: 10.1186/s12866-020-01739-1
Makino, H. (2018). Bifidobacterial strains in the intestines of newborns originate from their mothers. Biosci. Microbiota Food Health 37, 79–85. doi: 10.12938/bmfh.18-011
Martin, F. L. (2001). Genotoxins and the initiation of sporadic breast cancer. Mutagenesis 16, 155–161. doi: 10.1093/mutage/16.2.155
Matson, V., Fessler, J., Bao, R., Chongsuwat, T., Zha, Y., Alegre, M. L., et al. (2018). The commensal microbiome is associated with anti-pd-1 efficacy in metastatic melanoma patients. Science 359, 104–108. doi: 10.1126/science.aao3290
Mckee, A. M., Kirkup, B. M., Madgwick, M., Fowler, W. J., Price, C. A., Dreger, S. A., et al. (2021). Antibiotic-induced disturbances of the gut microbiota result in accelerated breast tumor growth. Iscience 24, 103012. doi: 10.1016/j.isci.2021.103012
Mikó, E., Kovács, T., Sebő, É., Tóth, J., Csonka, T., Ujlaki, G., et al. (2019). Microbiome-microbial metabolome-cancer cell interactions in breast cancer-familiar, but unexplored. Cells 8 (4), 293. doi: 10.3390/cells8040293
Mikó, E., Vida, A., Kovács, T., Ujlaki, G., Trencsényi, G., Márton, J., et al. (2018). Lithocholic acid, A bacterial metabolite reduces breast cancer cell proliferation and aggressiveness. Biochim. Biophys. Acta Bioenerg 1859, 958–974. doi: 10.1016/j.bbabio.2018.04.002
Molina-Mateo, D., Valderrama, B. P., Zarate, R. V., Hidalgo, S., Tamayo-Leiva, J., Soto-Gonzalez, A., et al. (2023). Kanamycin treatment in the pre-symptomatic stage of A drosophila pd model prevents the onset of non-motor alterations. Neuropharmacology 236, 109573. doi: 10.1016/j.neuropharm.2023.109573
Muhammad, A. Y., Amonov, M., Baig, A. A., Alvi, F. J. (2024). Gut microbiome: an intersection between human genome, diet, and epigenetics. Advanced Gut Microbiome Res. 2024, 6707728. doi: 10.1155/2024/6707728
Munteanu, R., Feder, R. I., Onaciu, A., Munteanu, V. C., Iuga, C. A., Gulei, D. (2023). Insights into the human microbiome and its connections with prostate cancer. Cancers (Basel) 15 (9), 2539. doi: 10.3390/cancers15092539
Murata-Kamiya, N., Kurashima, Y., Teishikata, Y., Yamahashi, Y., Saito, Y., Higashi, H., et al. (2007). Helicobacter pylori caga interacts with E-cadherin and deregulates the beta-catenin signal that promotes intestinal transdifferentiation in gastric epithelial cells. Oncogene 26, 4617–4626. doi: 10.1038/sj.onc.1210251
Nakatsu, G., Li, X., Zhou, H., Sheng, J., Wong, S. H., Wu, W. K., et al. (2015). Gut mucosal microbiome across stages of colorectal carcinogenesis. Nat. Commun. 6, 8727. doi: 10.1038/ncomms9727
Nandi, D., Parida, S., Sharma, D. (2023). The gut microbiota in breast cancer development and treatment: the good, the bad, and the useful! Gut Microbes 15, 2221452. doi: 10.1080/19490976.2023.2221452
Niccolai, E., Baldi, S., Nannini, G., Gensini, F., Papi, L., Vezzosi, V., et al. (2023). Breast cancer: the first comparative evaluation of oncobiome composition between males and females. Biol. Sex Differ 14, 37. doi: 10.1186/s13293-023-00523-w
Niu, C., Tu, Y., Jin, Q., Chen, Z., Yuan, K., Wang, M., et al. (2023). Mapping the human oral and gut fungal microbiota in patients with metabolic dysfunction-associated fatty liver disease. Front. Cell Infect. Microbiol. 13, 1157368. doi: 10.3389/fcimb.2023.1157368
Nuzum, N. D., Szymlek-Gay, E. A., Loke, S., Dawson, S. L., Teo, W. P., Hendy, A. M., et al. (2023). Differences in the gut microbiome across typical ageing and in parkinson’s disease. Neuropharmacology 235, 109566. doi: 10.1016/j.neuropharm.2023.109566
Odamaki, T., Sugahara, H., Yonezawa, S., Yaeshima, T., Iwatsuki, K., Tanabe, S., et al. (2012). Effect of the oral intake of yogurt containing bifidobacterium longum bb536 on the cell numbers of enterotoxigenic bacteroides fragilis in microbiota. Anaerobe 18, 14–18. doi: 10.1016/j.anaerobe.2011.11.004
Ohadian Moghadam, S., Momeni, S. A. (2021). Human microbiome and prostate cancer development: current insights into the prevention and treatment. Front. Med. 15, 11–32. doi: 10.1007/s11684-019-0731-7
Parhi, L., Alon-Maimon, T., Sol, A., Nejman, D., Shhadeh, A., Fainsod-Levi, T., et al. (2020). Breast cancer colonization by fusobacterium nucleatum accelerates tumor growth and metastatic progression. Nat. Commun. 11 (1), 3259. doi: 10.1038/s41467-020-16967-2
Parida, S., Wu, S., Siddharth, S., Wang, G., Muniraj, N., Nagalingam, A., et al. (2021). A procarcinogenic colon microbe promotes breast tumorigenesis and metastatic progression and concomitantly activates notch and β-catenin axes. Cancer Discovery 11, 1138–1157. doi: 10.1158/2159-8290.CD-20-0537
Park, S. Y., Hwang, B. O., Lim, M., Ok, S. H., Lee, S. K., Chun, K. S., et al. (2021). Oral-gut microbiome axis in gastrointestinal disease and cancer. Cancers (Basel) 13 (9), 2124. doi: 10.3390/cancers13092124
Pellegrini, M., Ippolito, M., Monge, T., Violi, R., Cappello, P., Ferrocino, I., et al. (2020). Gut microbiota composition after diet and probiotics in overweight breast cancer survivors: A randomized open-label pilot intervention trial. Nutrition 74, 110749. doi: 10.1016/j.nut.2020.110749
Peters, B. A., Wu, J., Pei, Z., Yang, L., Purdue, M. P., Freedman, N. D., et al. (2017). Oral microbiome composition reflects prospective risk for esophageal cancers. Cancer Res. 77, 6777–6787. doi: 10.1158/0008-5472.CAN-17-1296
Plaza-Diaz, J., Álvarez-Mercado, A. I. (2023). The interplay between microbiota and chemotherapy-derived metabolites in breast cancer. Metabolites 13 (6), 703. doi: 10.3390/metabo13060703
Plaza-Díaz, J., Álvarez-Mercado, A. I., Ruiz-Marín, C. M., Reina-Pérez, I., Pérez-Alonso, A. J., Sánchez-Andujar, M. B., et al. (2019). Association of breast and gut microbiota dysbiosis and the risk of breast cancer: A case-control clinical study. BMC Cancer 19, 495. doi: 10.1186/s12885-019-5660-y
Porter, C. M., Shrestha, E., Peiffer, L. B., Sfanos, K. S. (2018). The microbiome in prostate inflammation and prostate cancer. Prostate Cancer Prostatic Dis. 21, 345–354. doi: 10.1038/s41391-018-0041-1
Portincasa, P., Bonfrate, L., Vacca, M., De Angelis, M., Farella, I., Lanza, E., et al. (2022). Gut microbiota and short chain fatty acids: implications in glucose homeostasis. Int. J. Mol. Sci. 23 (3), 1105. doi: 10.3390/ijms23031105
Pushalkar, S., Hundeyin, M., Daley, D., Zambirinis, C. P., Kurz, E., Mishra, A., et al. (2018). The pancreatic cancer microbiome promotes oncogenesis by induction of innate and adaptive immune suppression. Cancer Discovery 8, 403–416. doi: 10.1158/2159-8290.CD-17-1134
Qu, R., Zhang, Y., Ma, Y., Zhou, X., Sun, L., Jiang, C., et al. (2023). Role of the gut microbiota and its metabolites in tumorigenesis or development of colorectal cancer. Adv. Sci. (Weinh) 10 (23), E2205563. doi: 10.1002/advs.202205563
Rea, D., Coppola, G., Palma, G., Barbieri, A., Luciano, A., Del Prete, P., et al. (2018). Microbiota effects on cancer: from risks to therapies. Oncotarget 9, 17915–17927. doi: 10.18632/oncotarget.24681
Rinninella, E., Raoul, P., Cintoni, M., Franceschi, F., Miggiano, G. A. D., Gasbarrini, A., et al. (2019). What is the healthy gut microbiota composition? A changing ecosystem across age, environment, diet, and diseases. Microorganisms 7 (1), 14. doi: 10.3390/microorganisms7010014
Routy, B., Le Chatelier, E., Derosa, L., Duong, C. P. M., Alou, M. T., Daillère, R., et al. (2018). Gut microbiome influences efficacy of pd-1-based immunotherapy against epithelial tumors. Science 359, 91–97. doi: 10.1126/science.aan3706
Rubinstein, M. R., Wang, X., Liu, W., Hao, Y., Cai, G., Han, Y. W. (2013). Fusobacterium nucleatum promotes colorectal carcinogenesis by modulating E-cadherin/β-catenin signaling via its fada adhesin. Cell Host Microbe 14, 195–206. doi: 10.1016/j.chom.2013.07.012
Ruo, S. W., Alkayyali, T., Win, M., Tara, A., Joseph, C., Kannan, A., et al. (2021). Role of gut microbiota dysbiosis in breast cancer and novel approaches in prevention, diagnosis, and treatment. Cureus 13, E17472. doi: 10.7759/cureus.17472
Russo, E., Bellando-Randone, S., Carboni, D., Fioretto, B. S., Romano, E., Baldi, S., et al. (2023a). The differential crosstalk of the skin-gut microbiome axis as A new emerging actor in systemic sclerosis. Rheumatol. (Oxford). 63 (1), 226–234. doi: 10.1093/rheumatology/kead208
Russo, E., Gloria, L. D., Nannini, G., Meoni, G., Niccolai, E., Ringressi, M. N., et al. (2023b). From adenoma to crc stages: the oral-gut microbiome axis as A source of potential microbial and metabolic biomarkers of Malignancy. Neoplasia 40, 100901. doi: 10.1016/j.neo.2023.100901
Salimi, V., Shahsavari, Z., Safizadeh, B., Hosseini, A., Khademian, N., Tavakoli-Yaraki, M. (2017). Sodium butyrate promotes apoptosis in breast cancer cells through reactive oxygen species (Ros) formation and mitochondrial impairment. Lipids Health Dis. 16, 208. doi: 10.1186/s12944-017-0593-4
Saud Hussein, A., Ibraheem Salih, N., Hashim Saadoon, I. (2021). Effect of microbiota in the development of breast cancer. Arch. Razi Inst 76 (4), 761–768. doi: 10.22092/ari.2021.355961.1750
Schmidt, T. S., Hayward, M. R., Coelho, L. P., Li, S. S., Costea, P. I., Voigt, A. Y., et al. (2019). Extensive transmission of microbes along the gastrointestinal tract. Elife 8, e42693. doi: 10.7554/eLife.42693
Schwabe, R. F., Jobin, C. (2013). The microbiome and cancer. Nat. Rev. Cancer 13, 800–812. doi: 10.1038/nrc3610
Scott, A. J., Alexander, J. L., Merrifield, C. A., Cunningham, D., Jobin, C., Brown, R., et al. (2019). International cancer microbiome consortium consensus statement on the role of the human microbiome in carcinogenesis. Gut 68, 1624–1632. doi: 10.1136/gutjnl-2019-318556
Senthakumaran, T., Moen, A. E. F., Tannaes, T. M., Endres, A., Brackmann, S. A., Rounge, T. B., et al. (2023). Microbial dynamics with crc progression: A study of the mucosal microbiota at multiple sites in cancers, adenomatous polyps, and healthy controls. Eur. J. Clin. Microbiol. Infect. Dis. 42, 305–322. doi: 10.1007/s10096-023-04551-7
Sfreddo, C. S., Maier, J., De David, S. C., Susin, C., Moreira, C. H. C. (2017). Periodontitis and breast cancer: A case-control study. Community Dent. Oral. Epidemiol. 45, 545–551. doi: 10.1111/cdoe.2017.45.issue-6
Sharma, M., Tollefsbol, T. O. (2022). Combinatorial epigenetic mechanisms of sulforaphane, genistein and sodium butyrate in breast cancer inhibition. Exp. Cell Res. 416, 113160. doi: 10.1016/j.yexcr.2022.113160
Shiao, S. L., Kershaw, K. M., Limon, J. J., You, S., Yoon, J., Ko, E. Y., et al. (2021). Commensal bacteria and fungi differentially regulate tumor responses to radiation therapy. Cancer Cell 39, 1202–1213.E6. doi: 10.1016/j.ccell.2021.07.002
Silva-Boghossian, C. M., Cesario, P. C., Leao, A. T. T., Colombo, A. P. V. (2018). Subgingival microbial profile of obese women with periodontal disease. J. Periodontol 89, 186–194. doi: 10.1002/jper.2018.89.issue-2
Smith, K. S., Frugé, A. D., van der Pol, W., Caston, N. E., Morrow, C. D., Demark-Wahnefried, W., et al. (2021). Gut microbial differences in breast and prostate cancer cases from two randomised controlled trials compared to matched cancer-free controls. Benef Microbes 12, 239–248. doi: 10.3920/BM2020.0098
Su, J., Su, L., Li, D., Shuai, O., Zhang, Y., Liang, H., et al. (2018). Antitumor activity of extract from the sporoderm-breaking spore of ganoderma lucidum: restoration on exhausted cytotoxic T cell with gut microbiota remodeling. Front. Immunol. 9. doi: 10.3389/fimmu.2018.01765
Sun, Z., Ge, X., Qiu, B., Xiang, Z., Jiang, C., Wu, J., et al. (2023). Vulvovaginal candidiasis and vaginal microflora interaction: microflora changes and probiotic therapy. Front. In Cell. And Infection Microbiol. 13, 1123026. doi: 10.3389/fcimb.2023.1123026
Sun, J., Tang, Q., Yu, S., Xie, M., Xie, Y., Chen, G., et al. (2020). Role of the oral microbiota in cancer evolution and progression. Cancer Med. 9, 6306–6321. doi: 10.1002/cam4.v9.17
Sung, H., Ferlay, J., Siegel, R. L., Laversanne, M., Soerjomataram, I., Jemal, A., et al. (2021). Global cancer statistics 2020: globocan estimates of incidence and mortality worldwide for 36 cancers in 185 countries. CA Cancer J. Clin. 71, 209–249. doi: 10.3322/caac.21660
Tan, X., Wang, Y., Gong, T. (2023). The interplay between oral microbiota, gut microbiota and systematic diseases. J. Oral. Microbiol. 15, 2213112. doi: 10.1080/20002297.2023.2213112
Teng, N. M. Y., Price, C. A., Mckee, A. M., Hall, L. J., Robinson, S. D. (2021). Exploring the impact of gut microbiota and diet on breast cancer risk and progression. Int. J. Cancer 149, 494–504. doi: 10.1002/ijc.v149.3
Terrisse, S., Zitvogel, L., Kroemer, G. (2023). Impact of microbiota on breast cancer hormone therapy. Cell Stress 7, 12–19. doi: 10.15698/cst2023.03.277
Thirunavukkarasan, M., Wang, C., Rao, A., Hind, T., Teo, Y. R., Siddiquee, A. A., et al. (2017). Short-chain fatty acid receptors inhibit invasive phenotypes in breast cancer cells. PloS One 12, E0186334. doi: 10.1371/journal.pone.0186334
Thu, M. S., Ondee, T., Nopsopon, T., Farzana, I. A. K., Fothergill, J. L., Hirankarn, N., et al. (2023). Effect of probiotics in breast cancer: A systematic review and meta-analysis. Biol. (Basel) 12 (2), 280. doi: 10.3390/biology12020280
Thursby, E., Juge, N. (2017). Introduction to the human gut microbiota. Biochem. J. 474, 1823–1836. doi: 10.1042/BCJ20160510
Tilg, H., Zmora, N., Adolph, T. E., Elinav, E. (2020). The intestinal microbiota fuelling metabolic inflammation. Nat. Rev. Immunol. 20, 40–54. doi: 10.1038/s41577-019-0198-4
Toumazi, D., El Daccache, S., Constantinou, C. (2021). An unexpected link: the role of mammary and gut microbiota on breast cancer development and management (Review). Oncol. Rep. 45 (5), 80. doi: 10.3892/or.2021.8031
Trah, J., Arand, J., Oh, J., Pagerols-Raluy, L., Trochimiuk, M., Appl, B., et al. (2020). Lithocholic bile acid induces apoptosis in human nephroblastoma cells: A non-selective treatment option. Sci. Rep. 10, 20349. doi: 10.1038/s41598-020-77436-w
Urbaniak, C., Cummins, J., Brackstone, M., Macklaim, J. M., Gloor, G. B., Baban, C. K., et al. (2014). Microbiota of human breast tissue. Appl. Environ. Microbiol. 80, 3007–3014. doi: 10.1128/AEM.00242-14
Urbaniak, C., Gloor, G. B., Brackstone, M., Scott, L., Tangney, M., Reid, G. (2016). The microbiota of breast tissue and its association with breast cancer. Appl. Environ. Microbiol. 82, 5039–5048. doi: 10.1128/AEM.01235-16
Van Der Merwe, M., Van Niekerk, G., Botha, A., Engelbrecht, A. M. (2021). The onco-immunological implications of fusobacterium nucleatum in breast cancer. Immunol. Lett. 232, 60–66. doi: 10.1016/j.imlet.2021.02.007
Vergara, D., Simeone, P., Damato, M., Maffia, M., Lanuti, P., Trerotola, M. (2019). The cancer microbiota: emt and inflammation as shared molecular mechanisms associated with plasticity and progression. J. Oncol. 2019, 1253727. doi: 10.1155/2019/1253727
Vitorino, M., Alpuim Costa, D., Vicente, R., Caleça, T., Santos, C. (2022). Local breast microbiota: A “New. Player On Block. Cancers (Basel) 14 (15), 3811. doi: 10.3390/cancers14153811
Vitorino, M., Baptista De Almeida, S., Alpuim Costa, D., Faria, A., Calhau, C., Azambuja Braga, S. (2021). Human microbiota and immunotherapy in breast cancer - A review of recent developments. Front. Oncol. 11, 815772. doi: 10.3389/fonc.2021.815772
Vivarelli, S., Salemi, R., Candido, S., Falzone, L., Santagati, M., Stefani, S., et al. (2019). Gut microbiota and cancer: from pathogenesis to therapy. Cancers (Basel) 11 (1), 38. doi: 10.3390/cancers11010038
Wade, W. G. (2013). The oral microbiome in health and disease. Pharmacol. Res. 69, 137–143. doi: 10.1016/j.phrs.2012.11.006
Wang, B., Hao, X., Yan, J., Li, X., Zhao, M., Han, T. (2024). A bibliometric analysis of immune-related adverse events in cancer patients and A meta-analysis of immune-related adverse events in patients with hepatocellular carcinoma. J. Of Trans. Internal Med. 12 (3), 225–243. doi: 10.2478/jtim-2024-0003
Wang, Y., Hu, P. C., Ma, Y. B., Fan, R., Gao, F. F., Zhang, J. W., et al. (2016). Sodium butyrate-induced apoptosis and ultrastructural changes in mcf-7 breast cancer cells. Ultrastruct Pathol. 40, 200–204. doi: 10.3109/01913123.2016.1170083
Wang, X., Jia, Y., Wen, L., Mu, W., Wu, X., Liu, T., et al. (2021). Porphyromonas gingivalis promotes colorectal carcinoma by activating the hematopoietic nlrp3 inflammasome. Cancer Res. 81, 2745–2759. doi: 10.1158/0008-5472.CAN-20-3827
Wang, Y., Wu, J., Xu, J., Lin, S. (2019). Clinical significance of high expression of stanniocalcin-2 in hepatocellular carcinoma. Bioscience Rep. 39, Bsr20182057. doi: 10.1042/BSR20182057
Wang, N., Yang, J., Han, W., Han, M., Liu, X., Jiang, L., et al. (2022). Identifying distinctive tissue and fecal microbial signatures and the tumor-promoting effects of deoxycholic acid on breast cancer. Front. Cell Infect. Microbiol. 12, 1029905. doi: 10.3389/fcimb.2022.1029905
Wei, L., Zhang, L., Zhang, Y., Yan, L., Liu, B., Cao, Z., et al. (2023). Intestinal escherichia coli and related dysfunction as potential targets of traditional chinese medicine for respiratory infectious diseases. J. Ethnopharmacol 313, 116381. doi: 10.1016/j.jep.2023.116381
Wirbel, J., Pyl, P. T., Kartal, E., Zych, K., Kashani, A., Milanese, A., et al. (2019). Meta-analysis of fecal metagenomes reveals global microbial signatures that are specific for colorectal cancer. Nat. Med. 25, 679–689. doi: 10.1038/s41591-019-0406-6
Wong, C. C., Yu, J. (2023). Gut microbiota in colorectal cancer development and therapy. Nat. Rev. Clin. Oncol. 20, 429–452. doi: 10.1038/s41571-023-00766-x
Wu, J., Bortolanza, M., Zhai, G., Shang, A., Ling, Z., Jiang, B., et al. (2022b). Gut microbiota dysbiosis associated with plasma levels of interferon-Γ And viral load in patients with acute hepatitis E infection. J. Of Med. Virol. 94, 692–702. doi: 10.1002/jmv.27356
Wu, Z., Byrd, D. A., Wan, Y., Ansong, D., Clegg-Lamptey, J. N., Wiafe-Addai, B., et al. (2022c). The oral microbiome and breast cancer and nonmalignant breast disease, and its relationship with the fecal microbiome in the Ghana breast health study. Int. J. Cancer 151, 1248–1260. doi: 10.1002/ijc.v151.8
Wu, F., Yang, L., Hao, Y., Zhou, B., Hu, J., Yang, Y., et al. (2022a). Oral and gastric microbiome in relation to gastric intestinal metaplasia. Int. J. Cancer 150, 928–940. doi: 10.1002/ijc.v150.6
Xia, C., Su, J., Liu, C., Mai, Z., Yin, S., Yang, C., et al. (2023). Human microbiomes in cancer development and therapy. Medcomm (2020) 4, E221. doi: 10.1002/mco2.v4.2
Xiang, Z., Wu, J., Li, J., Zheng, S., Wei, X., Xu, X. (2023). Gut microbiota modulation: A viable strategy to address medical needs in hepatocellular carcinoma and liver transplantation. Engineering 29, 59–72. doi: 10.1016/j.eng.2022.12.012
Xuan, C., Shamonki, J. M., Chung, A., Dinome, M. L., Chung, M., Sieling, P. A., et al. (2014). Microbial dysbiosis is associated with human breast cancer. PloS One 9, E83744. doi: 10.1371/journal.pone.0083744
Yang, H. J. (2023). Gastric cancer and gastric microbiome. Korean J. Gastroenterol. 81, 235–242. doi: 10.4166/kjg.2023.055
Yang, Y., Long, J., Wang, C., Blot, W. J., Pei, Z., Shu, X., et al. (2022). Prospective study of oral microbiome and gastric cancer risk among asian, african american and european american populations. Int. J. Cancer 150, 916–927. doi: 10.1002/ijc.v150.6
Yang, Q., Wang, B., Zheng, Q., Li, H., Meng, X., Zhou, F., et al. (2023). A review of gut microbiota-derived metabolites in tumor progression and cancer therapy. Adv. Sci. (Weinh) 10, E2207366. doi: 10.1002/advs.202207366
Zeng, H., He, S., Xiong, Z., Su, J., Wang, Y., Zheng, B., et al. (2023a). Gut microbiota-metabolic axis insight into the hyperlipidemic effect of lotus seed resistant starch in hyperlipidemic mice. Carbohydr Polym 314, 120939. doi: 10.1016/j.carbpol.2023.120939
Zeng, J., Yang, K., Nie, H., Yuan, L., Wang, S., Zeng, L., et al. (2023b). The mechanism of intestinal microbiota regulating immunity and inflammation in ischemic stroke and the role of natural botanical active ingredients in regulating intestinal microbiota: A review. BioMed. Pharmacother. 157, 114026. doi: 10.1016/j.biopha.2022.114026
Zhang, H., Jin, K., Xiong, K., Jing, W., Pang, Z., Feng, M., et al. (2023b). Disease-associated gut microbiome and critical metabolomic alterations in patients with colorectal cancer. Cancer Med. 12 (14), 15720–15735. doi: 10.1002/cam4.6194
Zhang, S., Kong, C., Yang, Y., Cai, S., Li, X., Cai, G., et al. (2020b). Human oral microbiome dysbiosis as A novel non-invasive biomarker in detection of colorectal cancer. Theranostics 10, 11595–11606. doi: 10.7150/thno.49515
Zhang, T., Li, N., Wang, R., Sun, Y., He, X., Lu, X., et al. (2023c). Enhanced therapeutic efficacy of doxorubicin against multidrug-resistant breast cancer with reduced cardiotoxicity. Drug Delivery 30, 2189118. doi: 10.1080/10717544.2023.2189118
Zhang, J., Lu, R., Zhang, Y., Matuszek, Ż., Zhang, W., Xia, Y., et al. (2020a). Trna queuosine modification enzyme modulates the growth and microbiome recruitment to breast tumors. Cancers (Basel) 12 (3), 628. doi: 10.21203/rs.2.21087/v1
Zhang, J., Ren, L., Yu, M., Liu, X., Ma, W., Huang, L., et al. (2019). S-equol inhibits proliferation and promotes apoptosis of human breast cancer mcf-7 Cells via regulating mir-10a-5p and pi3k/akt pathway. Arch. Biochem. Biophys. 672, 108064. doi: 10.1016/j.abb.2019.108064
Zhang, J., Xia, Y., Sun, J. (2021). Breast and gut microbiome in health and cancer. Genes Dis. 8, 581–589. doi: 10.1016/j.gendis.2020.08.002
Zhang, Y. G., Xia, Y., Zhang, J., Deb, S., Garrett, S., Sun, J. (2023d). Intestinal vitamin D receptor protects against extraintestinal breast cancer tumorigenesis. Gut Microbes 15, 2202593. doi: 10.1080/19490976.2023.2202593
Zhang, W., Zhang, Y., Li, Y., Ma, D., Zhang, H., Kwok, L. Y. (2022). Lacticaseibacillus rhamnosus probio-M9-driven mouse mammary tumor-inhibitory effect is accompanied by modulation of host gut microbiota, immunity, and serum metabolome. Nutrients 15 (1), 5. doi: 10.3390/nu15010005
Zhang, C. Y., Zhang, L., Wang, Z. M., Ren, D. H., Zheng, H. C. (2023a). The bioinformatics analysis of the clinicopathological and prognostic significances of reg4 mrna in gynecological cancers. J. Obstet Gynaecol 43, 2213764. doi: 10.1080/01443615.2023.2213764
Zhang, Z., Zhu, T., Zhang, L., Xing, Y., Yan, Z., Li, Q. (2023e). Critical influence of cytokines and immune cells in autoimmune gastritis. Autoimmunity 56, 2174531. doi: 10.1080/08916934.2023.2174531
Zhao, L. Y., Mei, J. X., Yu, G., Lei, L., Zhang, W. H., Liu, K., et al. (2023). Role of the gut microbiota in anticancer therapy: from molecular mechanisms to clinical applications. Signal Transduct Target Ther. 8, 201. doi: 10.1038/s41392-023-01406-7
Zheng, H. H., Du, C. T., Yu, C., Tang, X. Y., Huang, R. L., Zhang, Y. Z., et al. (2022). The relationship of tumor microbiome and oral bacteria and intestinal dysbiosis in canine mammary tumor. Int. J. Mol. Sci. 23 (18), 10928. doi: 10.3390/ijms231810928
Zhu, J., Liao, M., Yao, Z., Liang, W., Li, Q., Liu, J., et al. (2018). Breast cancer in postmenopausal women is associated with an altered gut metagenome. Microbiome 6, 136. doi: 10.1186/s40168-018-0515-3
Keywords: oral-gut microbiome axis, gut microbiota, oral microbiota, BC, tumor microecology
Citation: Huang L, Jiang C, Yan M, Wan W, Li S, Xiang Z and Wu J (2024) The oral-gut microbiome axis in breast cancer: from basic research to therapeutic applications. Front. Cell. Infect. Microbiol. 14:1413266. doi: 10.3389/fcimb.2024.1413266
Received: 07 April 2024; Accepted: 01 November 2024;
Published: 21 November 2024.
Edited by:
Cecilia Ana Suarez, National Scientific and Technical Research Council (CONICET), ArgentinaCopyright © 2024 Huang, Jiang, Yan, Wan, Li, Xiang and Wu. This is an open-access article distributed under the terms of the Creative Commons Attribution License (CC BY). The use, distribution or reproduction in other forums is permitted, provided the original author(s) and the copyright owner(s) are credited and that the original publication in this journal is cited, in accordance with accepted academic practice. No use, distribution or reproduction is permitted which does not comply with these terms.
*Correspondence: Jian Wu, d3VqaWFuZ2xpbnhpbmdAMTYzLmNvbQ==