- 1Department of Laboratory Medicine, Seoul National University Bundang Hospital and Seoul National University College of Medicine, Seoul, Republic of Korea
- 2Department of Laboratory Medicine and Research Institute of Bacterial Resistance, Yonsei University College of Medicine, Seoul, Republic of Korea
- 3Department of Laboratory Medicine and Paik Institute for Clinical Research, Inje University College of Medicine, Busan, Republic of Korea
- 4Department of Laboratory Medicine, National Health Insurance Service Ilsan Hospital, Goyang, Republic of Korea
- 5Department of Laboratory Medicine, Yonsei University Wonju College of Medicine, Wonju, Republic of Korea
- 6Department of Laboratory Medicine, Chonnam National University Medical School, Gwangju, Republic of Korea
Introduction: The rapid spread of COVID-19 worldwide within 2 months demonstrated the vulnerability of the world’s population to infectious diseases. In 2015, the Global Antimicrobial Resistance and Use Surveillance System (GLASS) was launched to combat antimicrobial resistance (AMR). However, there has been no comprehensive assessment of the decade-long global battle against AMR based on GLASS data.
Methods: South Korea established Kor-GLASS (Korean-GLASS) to proactively monitor data quality and enable international collaborations. A unique feature of Kor-GLASS is the quality control center (QCC), which uses network hubs and ensures standardized, high-quality data through interlaboratory proficiency testing (IPT) and external quality assessment (EQA). In addition, the QCC multifaceted endeavors for integrated data quality management.
Results: Since 2020, high-quality AMR data have indicated fluctuating antibiotic resistance rates in South Korea. This trend does not align with the decrease in antibiotic usage seen in humans but coincides with non-human antibiotic sales, indicating a need for greater monitoring of non-human antibiotic resistance. Comprehensive and robust management taking account of the intricate interplay among humans, animals, and the environment is essential. Kor-GLASS has been expanded into a “One Health” multiagency collaborative initiative.
Discussion: Although a standardized solution is not suitable for all countries, it must align with the local context and international standards. A centralized top-down management structure such as that of the QCC is essential to ensure continuous data quality coordination. Sustained efforts and surveillance systems are crucial for monitoring and managing AMR and safeguarding human health.
Introduction
In 2014, the O’Neill report highlighted the global threat posed by antimicrobial resistance (AMR) (O’Neill, 2014). Subsequently, in 2015, during the 68th World Health Assembly, the Global Antimicrobial Resistance and Use Surveillance System (GLASS) was established as a worldwide action plan by the World Health Organization (WHO) (Organization WHO, 2014). The rapid and widespread outbreak of COVID-19 in 2019, affecting over 200 countries within a span of two months, demonstrated the vulnerability of the world’s population to infectious diseases (Lim, 2021). This experience underscores the importance of robust surveillance systems for managing public health threats.
South Korea has been actively involved in AMR management since 1997, prior to the establishment of GLASS (Supplementary Figure S1, Supplementary Table S1). In 2016, South Korea launched the Korean Global Antimicrobial Resistance Surveillance System (Kor-GLASS), which is based on the GLASS platform but incorporates additional features aimed at enhancing data quality and management. Kor-GLASS operates under a “One Health” approach (KDCA, 2017-2021; Lee et al., 2018; Liu et al., 2019), which integrates human, animal, and environmental health sectors to comprehensively address AMR.
The primary objective of Kor-GLASS is to provide standardized, high-quality data that can be used for further analysis, interpretation, and actions by various stakeholders. The system includes key components such as external quality assessment (EQA) and interlaboratory proficiency testing (IPT) to ensure data quality. The Quality Control Center (QCC) plays a central role in managing these components and maintaining the integrity of the data collected.
This study aims to provide a detailed overview of the Kor-GLASS system, highlighting its unique features, innovative approaches, and contributions to the global fight against AMR. By comparing Kor-GLASS with other national AMR surveillance systems, this paper seeks to emphasize the system’s unique contributions and improvements, and to discuss its potential impact on AMR management.
Methods
Network organization of Kor-GLASS
Kor-GLASS was established to collect standardized AMR surveillance data in accordance with the GLASS guidelines, toward the construction of a global database. This initiative is steered by the Korea Disease Control and Prevention Agency (KDCA), a government agency. To ensure advancement of the AMR surveillance system, the government has appointed experts within a hierarchical framework. Kor-GLASS is based on four principles: representativeness, specialization, harmonization, and localization (Lee et al., 2018). The system is divided into five stages (Figure 1), systematically integrating specimen collection, data generation, and data quality assessment. Testing methods and results are standardized across all centers, and these uniform results are uploaded to the Kor-GLASS database (https://is.kdca.go.kr/). The operational framework for each hub ensures a consistent flow of specimens and data, with detailed processes outlined in Figure 2 and Supplementary Table S2.
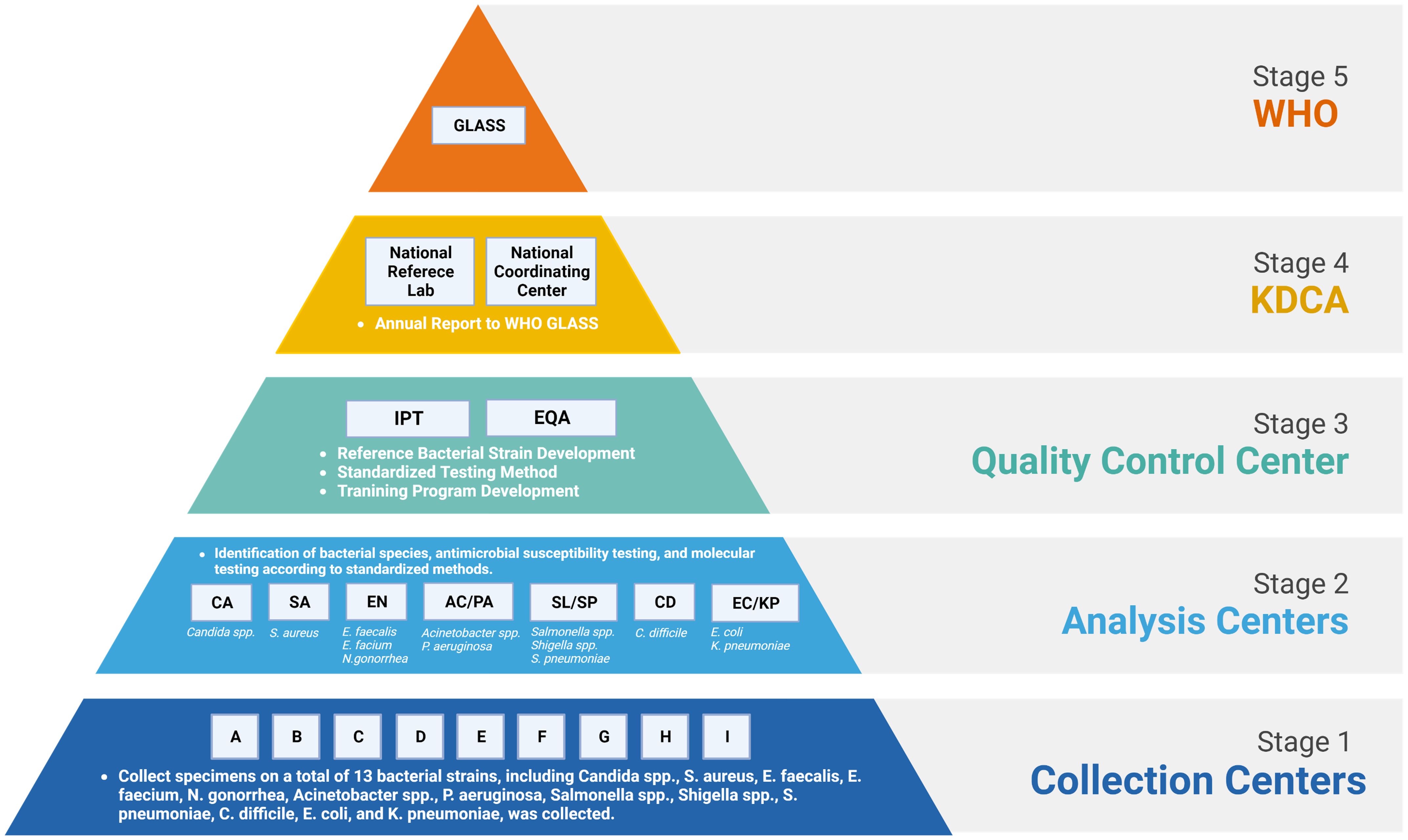
Figure 1 Organizational structure, relationships, and government and international institutions within Kor-GLASS. WHO, World Health Organization; GLASS, Global Antimicrobial Resistance and Use Surveillance System; KDCA, Korea Disease Control and Prevention Agency; IPT, interlaboratory proficiency testing; EQA, external quality assessment. Figures were created with BioRender.
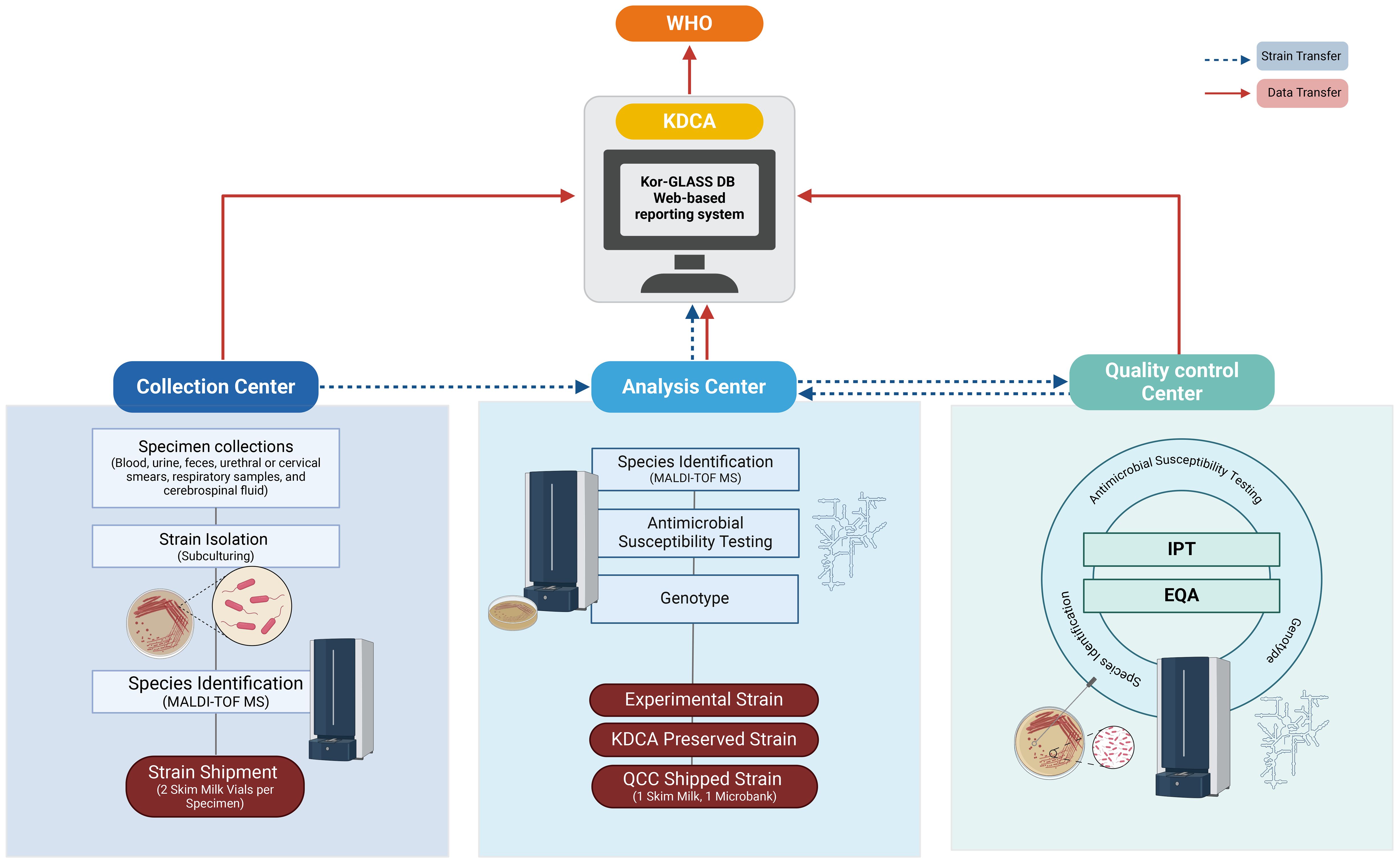
Figure 2 Flowchart of Strain Collection and Data Flow. The flowchart illustrates the process of strain collection and data flow, following two primary pathways: one from the collection center to either the analysis center or the quality control center, covering processes from identification to test management, and another from the analysis center to KDCA for strain preservation. Data collected by each center is uploaded to the Kor-GLASS database (https://is.kdca.go.kr/) in the designated format. This data is managed by KDCA, which reports the annual results to WHO in the GLASS format, ensuring precise tracking and reporting for high data integrity and quality control. Figures were created with BioRender.
Quality control center
A distinctive feature of Kor-GLASS is the establishment of a national-level Quality Control Center (QCC), which is independent of the GLASS system. This centralized QCC bridges gaps between network hubs, ensuring the generation of standardized, high-quality AMR surveillance data through unified criteria, and facilitating rapid improvements and issue resolution across the system.
Designated by the KDCA, the QCC operates independently of collection and analysis centers. It is a tertiary hospital laboratory with expertise in analysis, staffed with a dedicated on-site microbiology expert, and holds ISO 9001:2015 certification, ensuring adherence to stringent international standards. The QCC standardizes processes among analysis centers and manages the collected data, striving for network-wide consistency (Figure 3).
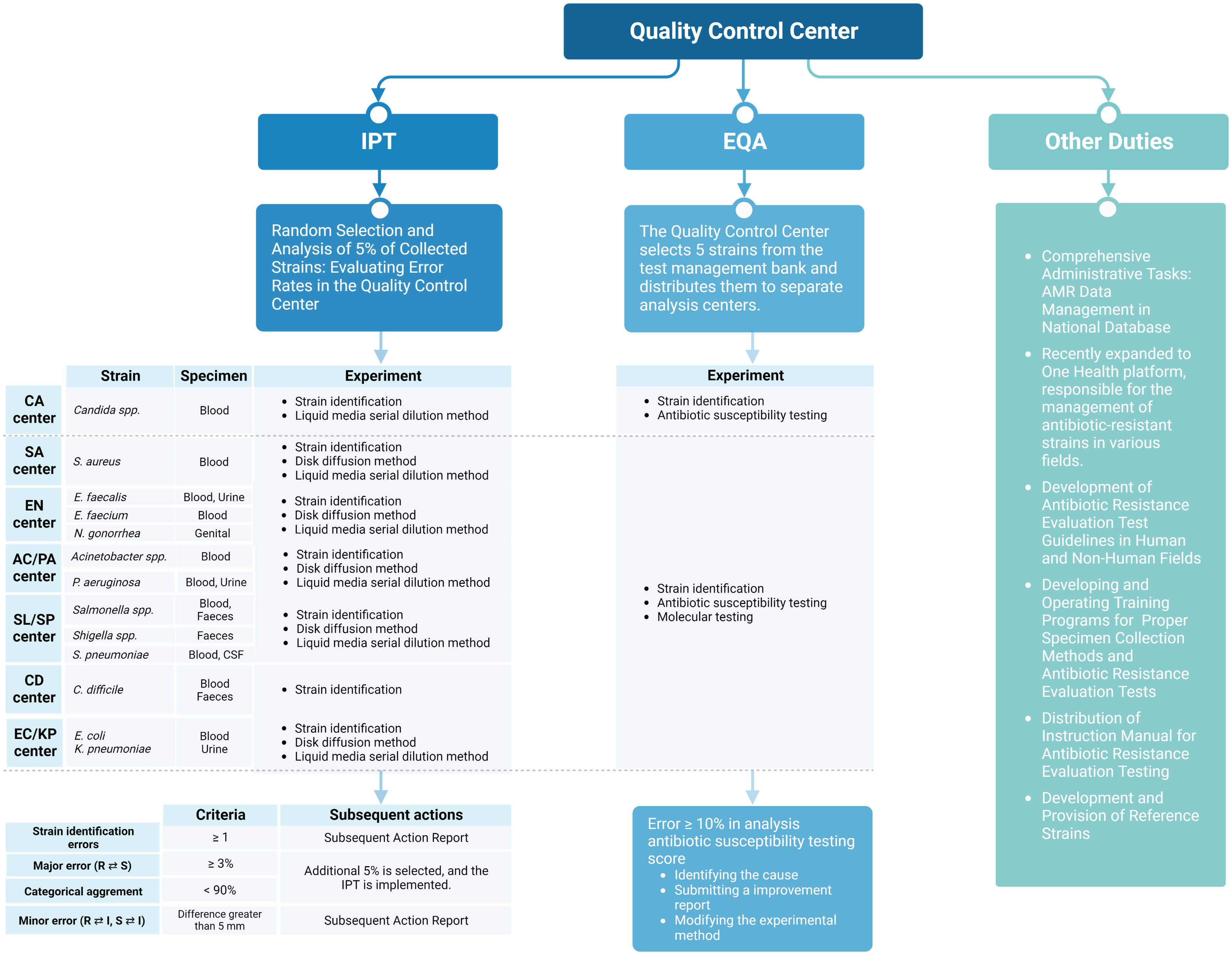
Figure 3 Role of the quality control center in antibiotic-resistant surveillance in Korea. Figures were created with BioRender.
Additionally, the QCC ensures rigorous data quality management through IPT and EQA. Unlike other national AMR surveillance systems in countries such as the United States (Robillard et al., 2024), the United Kingdom (Bennani et al., 2021), and Australia (Coombs et al., 2024), the centralized QCC of Kor-GLASS operates autonomously, maintaining high standards across multiple centers.
Initial integrated data quality management: IPT and EQA
Monitoring of the seven analysis centers responsible for generating species-specific AMR data under the guidance of the QCC is essential for integrated data quality management. Antibiotic susceptibility testing (AST) is performed using disk diffusion or broth microdilution methods in accordance with the recommendations of the Clinical Laboratory Standard Institute and the European Committee on Antimicrobial Susceptibility Testing (Matuschek et al., 2014; Wayne and Clinical and Laboratory Standards Institute, 2016). For data quality management, the QCC has implemented bidirectional management through IPT and EQA, ensuring comprehensive data quality control.
IPT is typically conducted on a monthly basis for samples delivered from analysis centers to the QCC. The QCC evaluates the error rate by performing AST on randomly selected isolates (5% for all species) from the strains collected from each analysis center. Errors are categorized as major, involving misclassification of resistant strains as susceptible or vice versa, or minor, involving intermediate classifications. The accepted threshold for major errors is < 3%, and the categorical agreement for the classification of susceptible, intermediate, and resistant strains should be > 90%. In cases where major errors exceed 3% or categorical agreement falls below 90%, an additional 5% of randomly selected strains for the specified period are tested at the QCC. Causes of errors are investigated, and corrective actions are implemented.
EQA is conducted on a quarterly basis and involves the distribution of fully identified strains from the QCC to each analysis center. Five strains are selected from the QCC strain bank, and analysis centers undertake testing within a predefined scope, encompassing tasks such as species identification and AST. The QCC assesses the data generated by the analysis centers, presenting the results as percentages, with a weight of 5 assigned to the degree of agreement with the identification results. If the error rate exceeds 10%, causes of the errors are investigated, and corrective actions are implemented.
Enhanced data quality management
The AMR data provided by the seven analysis center hubs, which conform to uniform testing criteria and quality standards, are aggregated within the Disease Control and Prevention Integrated Management System database, which is managed by the KDCA (Kor-GLASS database: https://is.kdca.go.kr/). Access to this database is restricted to designated personnel within the Kor-GLASS network hubs. The QCC uploads findings from its oversight activities, ensuring high-quality national AMR data are reported to the WHO annually through the KDCA. Furthermore, comprehensive AMR management data are disclosed transparently and are accessible for public scrutiny via the KDCA platform (https://nih.go.kr/nohas/common/main.do).
Multifaceted endeavors for data quality management: training and education
To minimize errors made during specimen collection and testing, the QCC provides ongoing education and develops guidelines for standardized collection methods. Biannual visits to participating laboratories are conducted to assess infrastructure and techniques, followed by training to address identified issues. The QCC also educates on updated molecular microbiology testing techniques, which are incorporated into standardized experimental guidelines.
For new participant laboratories, it evaluates adherence to guidelines, provides the necessary training, and conducts EQA testing. The QCC meticulously characterized the phenotypic and genetic resistance profiles for the scrutiny of reference strains. Subsequently, QCC leverages preserved reference strains for ongoing research endeavors and proficiency assessments, such as EQA, among laboratories involved in the management of resistant bacteria.
This process involves the use of matrix-assisted laser desorption/ionization time-of-flight mass spectrometry (MALDI-TOF MS) and 16S rRNA sequencing techniques for species identification. In certain challenging cases, Acinetobacter strains are identified through additional sequencing of the nuc and rpoB genes. SCCmec typing and analysis of Staphylococcus aureus strains involves examining the mecA and SCCmec genes using 18 primers. Additional tests include TSST-1 gene identification, analysis of eta and etb genes, multilocus sequence typing, spa typing, and whole-genome analysis using Pacific Biosciences equipment.
To develop strains for extended-spectrum beta-lactamase or carbapenemase proficiency testing, several genes are evaluated. In particular, TEM, SHV, and CTX genes are examined for extended-spectrum beta-lactamases, while CMY, DHA, and CIT genes are tested for plasmid-mediated AmpC β-lactamases. For carbapenemases, the KPC, GES, VIM, IMP, and NDM genes are analyzed. Additionally, mcr-1 PCR and sequence analysis, along with multilocus sequence typing, are performed.
Results
Outcomes of integrated data quality management by the QCC
IPT implementation and findings
IPT is the most important responsibility of the QCC because it ensures quality assurance and standardization of data generated by the analysis centers. The frequency of IPT implementation varies across centers depending on the number of collected specimens. Between 2020 and 2021, the following specimens underwent AST testing and analysis of species-specific bacterial-antimicrobial combinations through IPT:
− For E. coli, 1,464 of 19,568 specimens underwent AST, resulting in the testing of 29,500 bacterial-antimicrobial combinations.
− For Klebsiella pneumonia, 364 of 4,608 specimens underwent AST, resulting in the testing of 6,973 bacterial-antimicrobial combinations.
− For S. aureus, 156 of 1,506 specimens underwent AST, resulting in the testing of 2,214 bacterial-antimicrobial combinations.
− For Enterococcus faecium, 94 of 761 specimens underwent AST, resulting in the testing of 1,119 bacterial-antimicrobial combinations.
− For Enterococcus faecalis, 62 of 447 specimens underwent AST, resulting in the testing of 802 bacterial-antimicrobial combinations.
− For Acinetobacter baumannii, 103 of 412 specimens underwent AST, resulting in the testing of 1,468 bacterial-antimicrobial combinations.
− For Pseudomonas aeruginosa, 69 of 442 specimens underwent AST, resulting in the testing of 925 bacterial-antimicrobial combinations.
During this period, no species identification errors occurred in any of the centers. As depicted in Figure 4, the majority of bacterial species were subjected to monthly IPT. The AST results demonstrated categorical agreement within 90%, and no instances where major error rates exceeded 10%. However, some analysis centers had minor error rates > 10%, resulting in categorical agreement < 90%. In particular, minor error rates > 10% were noted in the results for A. baumanni in February and October 2021, as well as for P. aeruginosa in January 2022. These errors were attributed to inaccurate in determination of the size of inhibition zones according to EUCAST guidelines (EUCAST, 2024); subsequent education and retesting were performed to rectify these errors (See Supplementary Figure S2 for error photos in Disk diffusion test across various strains.). In subsequent IPTs, the error rate had stabilized within an acceptable range, confirming the production of consistently high-quality data.
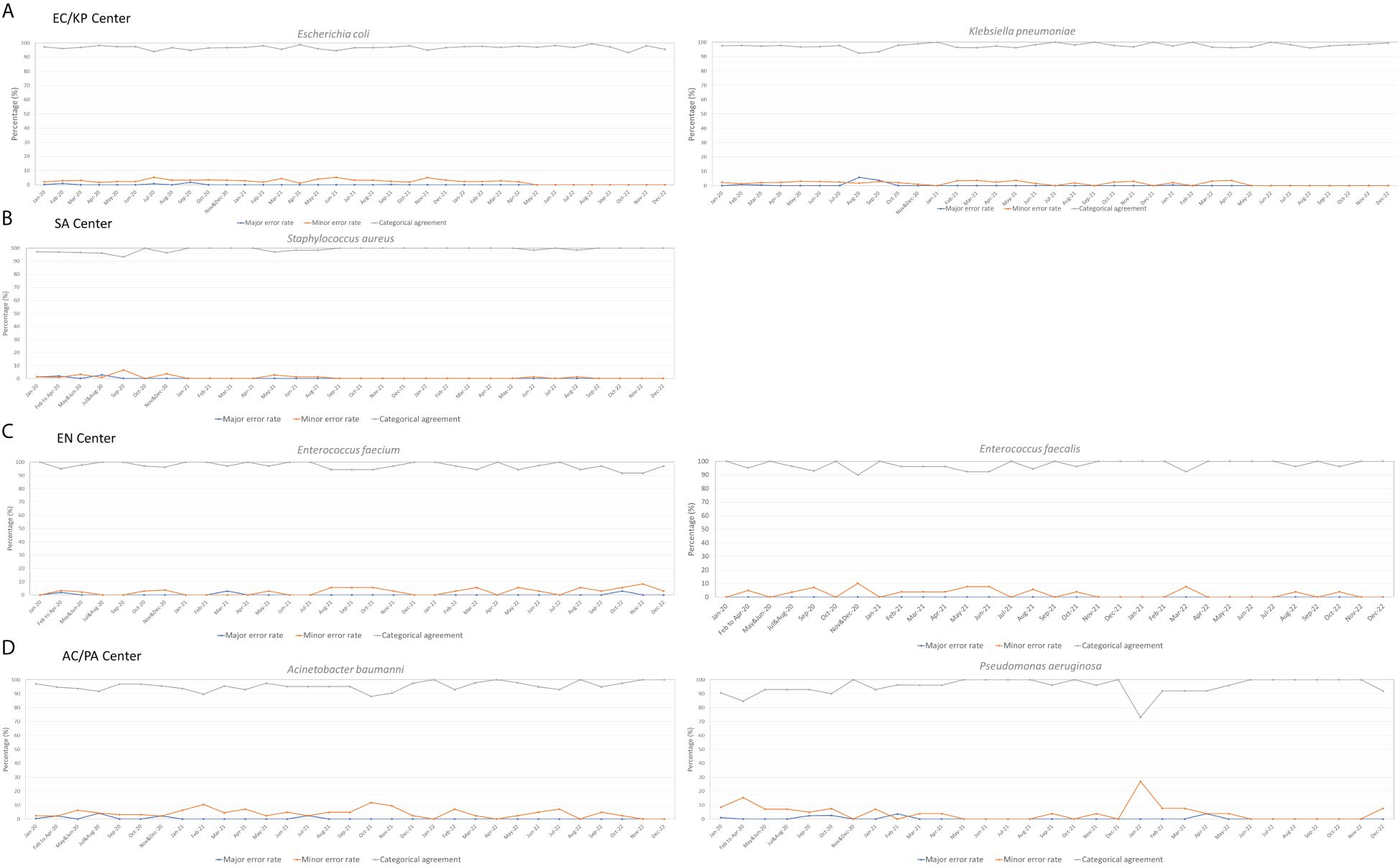
Figure 4 Three-Year Management Outcomes of the Quality Control Center: Ensuring Consistent Data Quality in Each Analysis Center. (A) EC/KP (Escherichia coli/Klebsiella pneumoniae) - Management outcomes of Escherichia coli and Klebsiella pneumoniae in the center; (B) SA (Staphylococcus aureus) - Management outcomes of Staphylococcus aureus in the center; (C) EN (Enterococcus) - Management outcomes of Enterococcus faecalis and Enterococcus faecium in the center; (D) AC/PA (Acinetobacter spp./Pseudomonas aeruginosa) - Management outcomes of Acinetobacter spp. and Pseudomonas aeruginosa in the center.
For centers with a lower volume of collected specimens, including SL/SP, CD, and CA centers where IPT procedures were performed every 3 months, the numbers of specimens that underwent IPT between 2020 and 2021 were as follows: 63 for Candida spp., 54 for Clostridium difficile, 41 for Salmonella spp., and 20 for Streptococcus pneumoniae. Notably, no errors or major discrepancies were observed in species identification among these specimens. For Candida spp., minor error rates were consistently low (average of 0.18%). Similarly, Salmonella spp. displayed a modest average error rate of 1.0%. However, S. pneumoniae showed a slight increase in minor identification errors during the initial half of 2021, with an average rate of 10.5%.
EQA performance and data quality
EQA is conducted quarterly and involves the distribution of fully identified strains from the QCC to each analysis center. The results of the EQA are presented as percentages. The error rates for all tested species were consistently low, indicating reliable data quality.
AMR trends in South Korea
A comprehensive analysis of AMR fluctuations in South Korea revealed distinct patterns among various strains. High levels of AMR were observed in critical priority pathogens (Organization WHO, 2017), including A. baumannii, P. aeruginosa, and multiple Enterobacteriaceae (including K. pneumoniae, E. coli, Serratia, and Proteus), indicating the need for continuous monitoring and management (Figure 5). Notably, the detection rate of extensively drug-resistant P. aeruginosa surged from 20.87% in 2020 to 26.61% in 2021, remaining high at 18.63% in 2022. Similarly, the proportion of extensively drug-resistant K. pneumoniae increased from 5.75% in 2020 to 9.72% in 2021, slightly decreasing to 8.87% in 2022. Considering that Kor-GLASS gathers data from medium to large hospitals with 500-1000 beds, the sudden surge in antibiotic resistance witnessed in 2021 cannot dismiss the potential for a temporary shift linked to COVID-19 in the respiratory intensive care units (La et al., 2022).
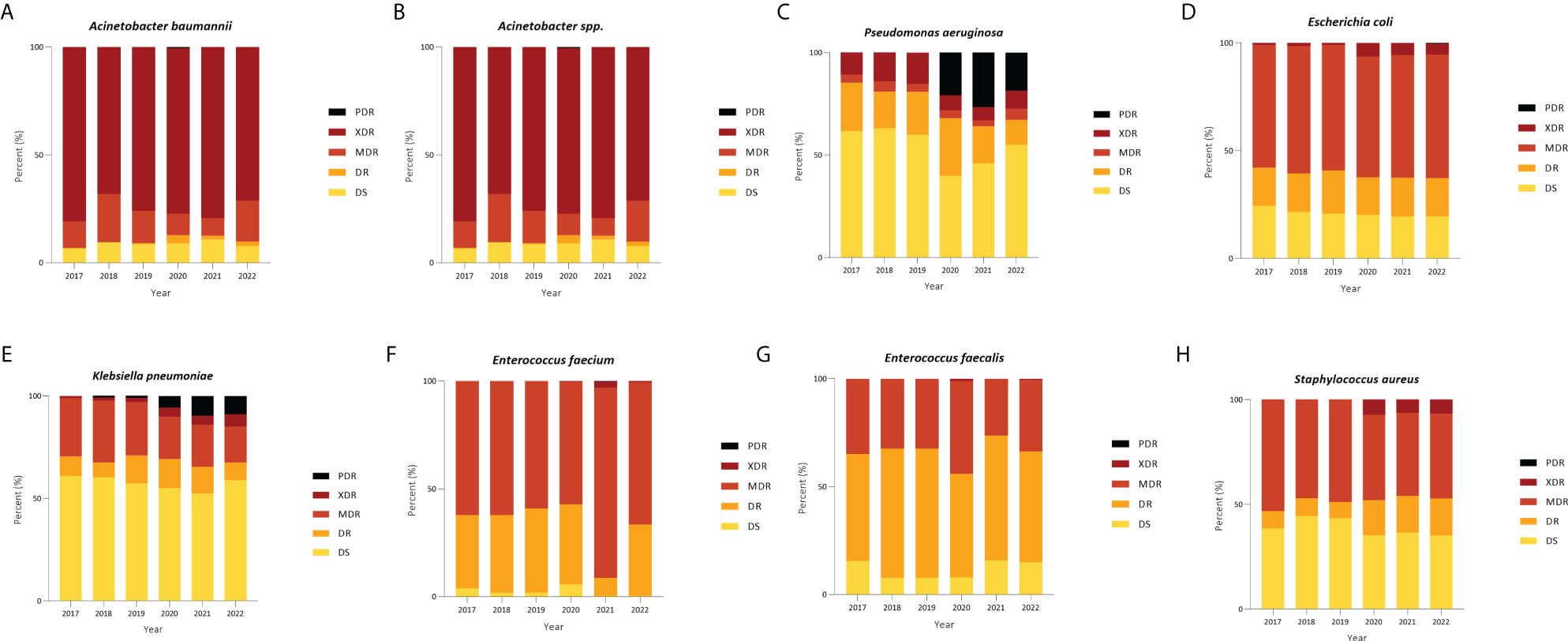
Figure 5 Distribution of categories of resistance among strains in blood. (A) Acinetobacter baumannii, (B) Acinetobacter spp., (C) Pseudomonas aeruginosa, (D) Escherichia coli, (E) Klebsiella pneumoniae, (F) Enterococcus faecium, (G) Enterococcus faecalis, and (H) Staphylococcus aureus. PDR, pan drug resistance; XDR, extensive drug resistance; MDR, multi-drug resistance; DR, drug resistance; DS, drug-sensitive.
Expansion into one health initiative
High-quality AMR data revealed that the AMR rate in Korea displayed marked fluctuations. Conversely, data from the Health Insurance Review and Assessment Service (HIRA, 2017-2021) indicated a gradual decline in antibiotic utilization in hospitals and pharmacies over the years. This decline is measured in terms of the daily dose per 1,000 Inhabitants per day (DID), which was 23.65 in 2017, 26.92 in 2018, 26.51 in 2019, 20.93 in 2020, and 19.58 in 2021. In contrast to these declining trends, Kor-GLASS verification data revealed fluctuating patterns, rather than the expected decrease in antibiotic resistance rates.
The antibiotic sales volume data provided by the Animal and Plant Quarantine Agency (2017-2021) revealed a fluctuating pattern: 1,003,678 kg in 2017, 960,663 kg in 2018, 903,476 kg in 2019, 894,999 kg in 2020, and 1,035,850 kg in 2021. The observed fluctuations in AMR rates within the Kor-GLASS data can be attributed to the increasing antibiotic use in non-human sectors.
Antibiotics are used for humans, animals, and the environment. Consequently, bacteria with antibiotic resistance can spread across the entire ecosystem, transcending boundaries (McEwen and Collignon, 2018; Collignon and McEwen, 2019; White and Hughes, 2019). In response, Kor-Glass expanded its AMR surveillance in 2019 to encompass organisms found in humans, animals, and the environment, operating as a One Health platform to monitor resistant strains across these domains [Korea Disease Control and Prevention Agency (KDCA), 2017-2021]. Kor-GLASS has thus evolved into a comprehensive “One Health” multiagency collaborative initiative (Figure 6). This approach ensures seamless data sharing and collaborative efforts across the human, animal, and environmental health sectors.
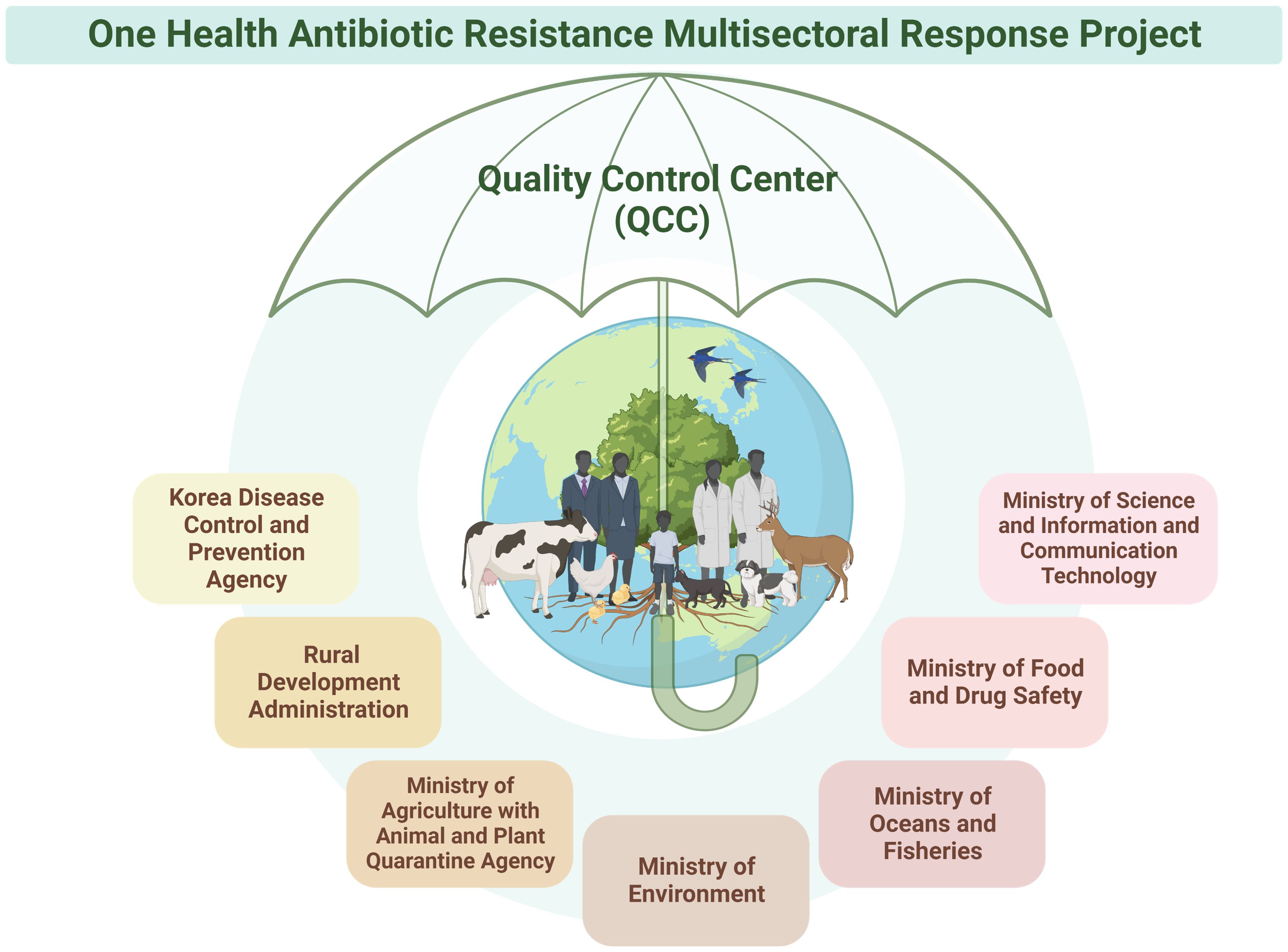
Figure 6 Collaboration with Seven Ministries in the One Health Antibiotic Resistance Multisectoral Response Project. The Korea Disease Control and Prevention Agency has embraced a One Health approach, forming partnerships with seven ministries. This collaborative initiative involves multidisciplinary research and development across human, animal, and environmental domains, addressing antibiotic resistance comprehensively. The project has set up an integrated network among the participating ministries to ensure coordinated management in line with research targets and objectives. Furthermore, each ministry maintains its own self-management system, with all data and management overseen by the quality control center. Figures were created with BioRender.
The KDCA adopted a One Health approach, collaborating with seven ministries, including the Ministry of Science and ICT, Ministry of Agriculture with the Animal and Plant Quarantine Agency, Ministry of Environment, Ministry of Oceans and Fisheries, Ministry of Food and Drug Safety, and the Rural Development Administration. This collaborative initiative involves multidisciplinary research and development across human, animal, and environmental domains.
Currently, this initiative is in its early stages, undergoing a systematization process similar to Kor-GLASS, with collection centers and analysis centers being established in the animal and environmental sectors. One Health analysis centers include laboratories within regional universities, where the QCC also conducts IPT and EQA. All ministries hold quarterly working-level meetings to monitor antibiotic resistance trends and publish biannual reports discussing future policy changes and budget allocations.
Discussion
The findings presented in this study underscore the critical importance of data quality management in AMR surveillance systems, specifically within the framework of Kor-GLASS. By expanding upon the One Health approach, Kor-GLASS has integrated data from human, animal, and environmental sectors, ensuring a comprehensive understanding of AMR trends. This integrated system, supported by a robust QCC, enables seamless data sharing and collaborative efforts among various health sectors, thereby enhancing the overall efficacy of AMR surveillance.
Kor-GLASS distinguishes itself from other national AMR surveillance systems (Bennani et al., 2021; Coombs et al., 2024; Robillard et al., 2024), through its centralized QCC. This unique feature ensures the standardization and high quality of AMR data across multiple centers. The QCC operates autonomously, providing rigorous oversight through IPT and EQA. This approach not only maintains high standards but also facilitates the rapid identification and resolution of any discrepancies or issues in the data. A recent report on the policy design, implementation, monitoring, and evaluation of national AMR action plans across 114 countries noted the absence of processes that guarantee the acquisition of standardized, high-quality data (Patel et al., 2023). This gap in systematization might explain why, even after a decade of GLASS operation, conducting a comprehensive analysis of the global AMR situation based on the available data remains challenging (Diseases, 2023).
The adoption of the One Health approach by Kor-GLASS marks a significant advancement in AMR surveillance, aligning with recent trends (Ruckert et al., 2024; Sabbatucci et al., 2024). Through this expansion, collaboration among all government departments involved in antibiotic use is ensured, enabling a comprehensive and multidisciplinary response to AMR. This collaboration facilitates the integration of diverse data sets and promotes a holistic understanding of AMR dynamics across different ecosystems.
A key strength of Kor-GLASS lies in its rigorous process and data level standardization, including the standardization of testing panels. Detailed protocols and procedures for IPT and EQA have been established and are consistently followed across all participating centers. The standardized operational framework ensures that specimen collection, data generation, and quality assessment are conducted uniformly, enhancing the reliability and comparability of the data. Additionally, the adoption of standardized data formats, ontologies, and semantics supports robust data management practices and facilitates international comparisons. The commitment to consistency and reliability is further underscored by the uniform implementation of species identification using MALDI-TOF and AST using disk diffusion methods, as detailed in Supplementary Table S2. This ensures that all analysis centers adhere to the same high standards.
While the client-server architecture employed by Kor-GLASS database may appear traditional, it offers several advantages, including centralized control, enhanced data security, and streamlined data quality management through the QCC. These benefits are crucial for maintaining the integrity and consistency of AMR data. The centralized approach allows for efficient oversight and rapid response to any data quality issues, ensuring that the system remains robust and reliable. Although the client-server model has limitations, such as potential scalability issues and reliance on a central point of control, these are mitigated within the Kor-GLASS framework through rigorous data validation and real-time monitoring capabilities, ensuring continuous system performance and reliability.
Since the first antibiotic was developed in the 1960s, only six new antibiotics have been introduced for clinical use (Walesch et al., 2023). As antibiotic development has reached its limits, the appropriate utilization and management of existing antibiotics are essential. Preventing AMR and protecting human health require sustainable efforts and surveillance systems. It is imperative to optimize the enhancement and utilization of GLASS data globally and prioritize overcoming this grave threat through concerted efforts across academia, commerce, public health, and policy sectors (Pallett et al., 2024). Amid slow antibiotic development and the relentless threat of superbugs, there is a need to comprehensively manage the complex interactions between humans, animals, and the environment while supporting robust data management practices. Therefore, continuous efforts to reduce AMR and strengthen global surveillance are necessary to create a sustainable future.
In conclusion, while economic and political disparities, as well as resource limitations among countries, must be taken into account when developing a global AMR management framework (Charani et al., 2023; Diseases, 2023), the enhanced functionalities of the Kor-GLASS system—particularly the integration of the One Health approach and the establishment of a centralized QCC—represent substantial advancements in the field of AMR surveillance. By addressing the critical aspects of data and process standardization, Kor-GLASS provides a model for high-quality, comprehensive AMR monitoring that can inform policy decisions and public health strategies. The ongoing collaboration among multiple ministries and the rigorous data quality management practices ensure that Kor-GLASS remains at the forefront of AMR surveillance, contributing valuable insights to the global effort to combat antimicrobial resistance.
Data availability statement
The raw data supporting the conclusions of this article will be made available by the authors, without undue reservation.
Ethics statement
The study protocol was approved by the Institutional Review Board of Seoul National University Bundang Hospital (no. X-2308-846-903).
Author contributions
HyK: Data curation, Formal analysis, Validation, Visualization, Writing – original draft, Writing – review & editing. JP:Conceptualization, Data curation, Investigation, Methodology, Project administration, Validation, Writing – review & editing. DK: Project administration, Resources, Writing – review & editing. HeK: Project administration, Resources, Writing – review & editing. JeS: Project administration, Resources, Writing – review & editing. YK: Project administration, Resources, Writing – review & editing. YU: Project administration, Resources, Writing – review & editing. SK: Project administration, Resources, Writing – review & editing. JoS: Project administration, Resources, Writing – review & editing. SJ: Project administration, Resources, Writing – review & editing. KP: Conceptualization, Data curation, Funding acquisition, Investigation, Methodology, Project administration, Resources, Supervision, Validation, Writing – review & editing.
Funding
The author(s) declare financial support was received for the research, authorship, and/or publication of this article. This work was supported by the research fund 2023-ER2202-00 of the Korea Disease Control and Prevention Agency.
Acknowledgments
We extend our deepest gratitude to the collection centers, analysis centers, KDCA, and all individuals associated with Kor-GLASS and One Health for their unwavering dedication and tireless efforts to address the critical national issue of antibiotic resistance.
Conflict of interest
The authors declare that the research was conducted in the absence of any commercial or financial relationships that could be construed as a potential conflict of interest.
Publisher’s note
All claims expressed in this article are solely those of the authors and do not necessarily represent those of their affiliated organizations, or those of the publisher, the editors and the reviewers. Any product that may be evaluated in this article, or claim that may be made by its manufacturer, is not guaranteed or endorsed by the publisher.
Supplementary material
The Supplementary Material for this article can be found online at: https://www.frontiersin.org/articles/10.3389/fcimb.2024.1411145/full#supplementary-material
References
Animal and Plant Quarantine Agency. (2017-2021). Available online at: http://qia.go.kr/anp/rchStatus/listwebQiaCom.do?type=90.
Bennani, H., Cornelsen, L., Stärk, K. D., Häsler, B. (2021). Characterisation and mapping of the surveillance system for antimicrobial resistance and antimicrobial use in the United Kingdom. Veterinary Rec. 188, e10. doi: 10.1002/vetr.10
Charani, E., Mendelson, M., Pallett, S. J., Ahmad, R., Mpundu, M., Mbamalu, O., et al. (2023). An analysis of existing national action plans for antimicrobial resistance—gaps and opportunities in strategies optimising antibiotic use in human populations. Lancet Global Health 11, e466–ee74. doi: 10.1016/S2214-109X(23)00019-0
Collignon, P. J., McEwen, S. A. (2019). One health—its importance in helping to better control antimicrobial resistance. Trop. Med. Infect. disease. 4, 22. doi: 10.3390/tropicalmed4010022
Coombs, G. W., Daley, D. A., Bell, J. M., Mowlaboccus, S., Iredell, J. R. (2024). The Australian group on antimicrobial resistance. Microbiol. Aust. 45 (2). doi: 10.1071/MA24018
Diseases, T. L. I. (2023). Antimicrobial resistance through the looking-GLASS. The Lancet. Infectious diseases 23 (2), 131. doi: 10.1016/S1473-3099(23)00012-9
EUCAST (2024). Antimicrobial susceptibility testing-EUCAST disk diffusion method (EUCAST). Available at: https://www.eucast.org/ast_of_bacteria/disk_diffusion_methodology.
HIRA. (2017-2021). Available online at: https://opendata.hira.or.kr/home.do.
KDCA. (2017-2021). One Health Antimicrobial resistant oraganism (Korea Centers for Disease Control and Prevention). Available at: https://www.kdca.go.kr/nohas/en/common/main.do.
Korea Disease Control and Prevention Agency (KDCA). (2017-2021). Ministry of Agriculture with the Animal and Plant Quarantine Agency. Available online at: https://www.kdca.go.kr/nohas/common/main.do.
La, Y., Hong, J. Y., Lee, H. S., Lee, E. H., Lee, K. H., Song, Y. G., et al. (2022). Increase of multidrug-resistant bacteria after the COVID-19 pandemic in South Korea: Time-series analyses of a long-term multicenter cohort. J. Infection. 85, 702. doi: 10.1016/j.jinf.2022.09.026
Lee, H., Yoon, E.-J., Kim, D., Jeong, S. H., Shin, J. H., Shin, J. H., et al. (2018). Establishment of the South Korean national antimicrobial resistance surveillance system, Kor-GLASS, in 2016. Eurosurveillance. 23, 1700734. doi: 10.2807/1560-7917.ES.2018.23.42.1700734
Lim, W. M. (2021). History, lessons, and ways forward from the COVID-19 pandemic. Int. J. Qual. Innovation. 5, 101–108.
Liu, C., Yoon, E.-J., Kim, D., Shin, J. H., Shin, J. H., Shin, K. S., et al. (2019). Antimicrobial resistance in South Korea: A report from the Korean global antimicrobial resistance surveillance system (Kor-GLASS) for 2017. J. Infection Chemotherapy. 25, 845–859. doi: 10.1016/j.jiac.2019.06.010
Matuschek, E., Brown, D. F., Kahlmeter, G. (2014). Development of the EUCAST disk diffusion antimicrobial susceptibility testing method and its implementation in routine microbiology laboratories. Clin. Microbiol. infection 20, O255–OO66. doi: 10.1111/1469-0691.12373
McEwen, S. A., Collignon, P. J. (2018). Antimicrobial resistance: a one health perspective. Microbiol Spectr. 6 (2). doi: 10.1128/microbiolspec.ARBA-0009-2017
O’Neill, J. (2014). Review on antimicrobial resistance. Antimicrobial resistance: tackling crisis Health wealth nations 2014. Available at: https://amr-review.org/sites/default/files/AMR%20Review%20Paper%20-%20Tackling%20a%20crisis%20for%20the%20health%20and%20wealth%20of%20nations_1.pdf.
Organization WHO (2014). Antimicrobial resistance: global report on surveillance (World Health Organization).
Organization WHO (2017). Prioritization of pathogens to guide discovery, research and development of new antibiotics for drug-resistant bacterial infections, including tuberculosis (World Health Organization).
Pallett, S. J. C., Charani, E., Lamb, G., Mazzella, A., Alqahtani, S. A., Moore, L. S. P. (2024). Optimising utilisation of GLASS data to inform cross-border antimicrobial resistance strategy is a priority. Lancet Regional Health–Americas. 29, 100655. doi: 10.1016/j.lana.2023.100655
Patel, J., Harant, A., Fernandes, G., Mwamelo, A. J., Hein, W., Dekker, D., et al. (2023). Measuring the global response to antimicrobial resistance, 2020–21: a systematic governance analysis of 114 countries. Lancet Infect. Diseases. 23, 706–718. doi: 10.1016/S1473-3099(22)00796-4
Robillard, D. W., Sundermann, A. J., Raux, B. R., Prinzi, A. M. (2024). Navigating the network: a narrative overview of AMR surveillance and data flow in the United States. Antimicrobial Stewardship Healthcare Epidemiol. 4, e55. doi: 10.1017/ash.2024.64
Ruckert, A., Harris, F., Aenishaenslin, C., Aguiar, R., Boudreau-LeBlanc, A., Carmo, L. P., et al. (2024). One Health governance principles for AMR surveillance: a scoping review and conceptual framework. Res. Directions: One Health 2, e4. doi: 10.1017/one.2023.13
Sabbatucci, M., Ashiru-Oredope, D., Barbier, L., Bohin, E., Bou-Antoun, S., Brown, C., et al. (2024). Tracking progress on antimicrobial resistance by the quadripartite country self-assessment survey (TrACSS) in G7 countries, 2017–2023: opportunities and gaps. Pharmacol. Res. 204, 107188. doi: 10.1016/j.phrs.2024.107188
Walesch, S., Birkelbach, J., Jézéquel, G., Haeckl, F. J., Hegemann, J. D., Hesterkamp, T., et al. (2023). Fighting antibiotic resistance—strategies and (pre) clinical developments to find new antibacterials. EMBO Rep. 24, e56033. doi: 10.15252/embr.202256033
Wayne, P., Clinical and Laboratory Standards Institute (2016). Performance standards for antimicrobial susceptibility testing: 26th informational supplement. CLSI document M100-S26.
Keywords: antimicrobial resistance, Kor-GLASS, quality control center, IPT, EQA
Citation: Kim H, Park JS, Kim D, Kim HJ, Shin JH, Kim YA, Uh Y, Kim SH, Shin JH, Jeong SH and Park KU (2024) Standardization of an antimicrobial resistance surveillance network through data management. Front. Cell. Infect. Microbiol. 14:1411145. doi: 10.3389/fcimb.2024.1411145
Received: 02 April 2024; Accepted: 02 July 2024;
Published: 29 July 2024.
Edited by:
Vineet Kumar, Central University of Rajasthan, IndiaReviewed by:
Leonardo Gabriel Panunzi, CEA Saclay, FranceHarpreet Singh, Indian Council of Medical Research (ICMR), India
Copyright © 2024 Kim, Park, Kim, Kim, Shin, Kim, Uh, Kim, Shin, Jeong and Park. This is an open-access article distributed under the terms of the Creative Commons Attribution License (CC BY). The use, distribution or reproduction in other forums is permitted, provided the original author(s) and the copyright owner(s) are credited and that the original publication in this journal is cited, in accordance with accepted academic practice. No use, distribution or reproduction is permitted which does not comply with these terms.
*Correspondence: Kyoung Un Park, bTkxdzk1cGZAc251LmFjLmty
†These authors have contributed equally to this work and share first authorship
‡ORCID: Kyoung Un Park, orcid.org/0000-0002-2402-7633