- 1Laboratory of Microbiology, Department of Clinical Laboratories; Escuela de Medicina, Pontificia Universidad Católica de Chile, Santiago, Chile
- 2Instituto de Ciencias e Innovación en Medicina (ICIM), Facultad de Medicina, Universidad del Desarrollo. Millennium Initiative for Collaborative Research on Bacterial Resistance (MICROB-R), Santiago, Chile
- 3Grupo de Resistencia Antimicrobiana en Bacterias Patógenas y Ambientales (GRABPA), Instituto de Biología, Pontificia Universidad Católica de Valparaíso, Valparaíso, Chile
- 4Instituto de Biomedicina de Sevilla, Hospital Universitario Virgen Macarena, Consejo Superior de Investigaciones Científicas (CSIC), Universidad de Sevilla, Sevilla, Spain
- 5Centro de Investigación Biomédica en Red de Enfermedades Infecciosas, Instituto de Salud Carlos III, Madrid, Spain
- 6Center for Bioinformatics and Integrative Biology, Facultad de Ciencias de la Vida, Universidad Andrés Bello, Santiago, Chile
- 7Genomics and Resistant Microbes Group (GeRM), Instituto de Ciencias e Innovación en Medicina (ICIM), Facultad de Medicina Clínica Alemana, Universidad del Desarrollo, Santiago, Chile
- 8Australian Institute for Microbiology and Infection, Faculty of Science, University of Technology Sydney, Sydney, NSW, Australia
- 9Clinical Laboratories Network, Red de Salud UC-CHRISTUS, Santiago, Chile
Introduction: Ceftazidime/avibactam (CZA) is indicated against multidrug-resistant Pseudomonas aeruginosa, particularly those that are carbapenem resistant. CZA resistance in P. aeruginosa producing PER, a class A extended-spectrum β-lactamase, has been well documented in vitro. However, data regarding clinical isolates are scarce. Our aim was to analyze the contribution of PER to CZA resistance in non-carbapenemase-producing P. aeruginosa clinical isolates that were ceftazidime and/or carbapenem non-susceptible.
Methods: Antimicrobial susceptibility was determined through agar dilution and broth microdilution, while blaPER gene was screened through PCR. All PER-positive isolates and five PER-negative isolates were analyzed through Whole Genome Sequencing. The mutational resistome associated to CZA resistance was determined through sequence analysis of genes coding for PBPs 1b, 3 and 4, MexAB-OprM regulators MexZ, MexR, NalC and NalD, AmpC regulators AmpD and AmpR, and OprD porin. Loss of blaPER-3 gene was induced in a PER-positive isolate by successive passages at 43°C without antibiotics.
Results: Twenty-six of 287 isolates studied (9.1%) were CZA-resistant. Thirteen of 26 CZA-resistant isolates (50%) carried blaPER. One isolate carried blaPER but was CZA-susceptible. PER-producing isolates had significantly higher MICs for CZA, amikacin, gentamicin, ceftazidime, meropenem and ciprofloxacin than non-PER-producing isolates. All PER-producing isolates were ST309 and their blaPER-3 gene was associated to ISCR1, an insertion sequence known to mobilize adjacent DNA. PER-negative isolates were classified as ST41, ST235 (two isolates), ST395 and ST253. PER-negative isolates carried genes for narrow-spectrum β-lactamases and the mutational resistome showed that all isolates had one major alteration in at least one of the genes analyzed. Loss of blaPER-3 gene restored susceptibility to CZA, ceftolozane/tazobactam and other β-lactamsin the in vitro evolved isolate.
Discussion: PER-3-producing ST309 P. aeruginosa is a successful multidrug-resistant clone with blaPER-3 gene implicated in resistance to CZA and other β-lactams.
Introduction
Carbapenem-resistant Pseudomonas aeruginosa is a global public health problem. Ceftazidime/avibactam (CZA) is a combination of a third-generation cephalosporin and an inhibitor of classes A, C, and some class D β-lactamases (Papp-Wallace et al., 2020). CZA was approved by the Food and Drug Administration (FDA) in 2015 and has become an alternative for treating infections caused by carbapenem-resistant bacteria (Papp-Wallace et al., 2020). Multiple mechanisms of resistance to CZA have been described in P. aeruginosa. It has been reported that resistance to CZA may be acquired through mutation of penicillin-binding proteins (PBPs) (Castanheira et al., 2019), overexpression and/or structural modifications in the Ω-loop region of the chromosomally-encoded AmpC β-lactamase (Ruedas-López et al., 2022). Experimental in vitro evolution assays and in vivo evidence showed that mutations related to overexpression and/or structural modification of MexAB-OprM and MexCD-OprJ efflux pump are associated with resistance to CZA (Chalhoub et al., 2018; Sanz-García et al., 2018; Castanheira et al., 2019). These mutations often involve inactivation of MexR, NalC, or NalD which are regulators of MexAB-OprM, and cause the overexpression of these efflux pumps resulting in 2- to 16- fold MIC increases to all the pump substrates compared to baseline levels (Adewoye et al., 2002). OprD porin inactivation was observed in CZA resistant clinical isolates of P. aeruginosa (Zamudio et al., 2019). Some variants of extended-spectrum β-lactamases (ESBLs) have also been associated with CZA resistance, including VEB-1, VEB-9, GES-26, GES-19, GES-7, SHV-2, SHV-5 and PER-1 (Kazmierczak et al., 2018; Khan et al., 2019; Mendes et al., 2019). PER (Pseudomonas-Extended-Resistant) is a class A ESBL that confers resistance to penicillins, broad-spectrum cephalosporins, and aztreonam (Philippon et al., 2016). In total, 16 variants of PER have been described. PER-1 is the most prevalent PER type in Europe and Asia, whereas PER-2 is frequent in South America (Xie et al., 2016). PER-3 has been reported in Aeromonas sp (Xie et al., 2016), and P. aeruginosa (Mojica et al., 2023), among other species. The blaPER gene was reported to be in the chromosome (Proteus mirabilis) and in plasmids (P. aeruginosa, P. mirabilis, Aeromonas sp and Enterobacter cloacae) (Xie et al., 2016). Although avibactam efficiently inhibits most class A β-lactamases, its efficacy against PER-type enzymes is reduced compared to other β-lactamases of the same class. The hydrophobicity of PER’s active site hampers the binding of water molecules that are crucial for the acylation step required for an efficient enzymatic inhibition (Ruggiero et al., 2019). PER-mediated resistance to CZA in P. aeruginosa has been demonstrated in vitro by introducing blaPER-1 in the reference strain PAO1 (Poirel et al., 2022; Ortiz de la Rosa et al., 2019). However, evidence of the contribution of PER to CZA resistance in clinical isolates of P. aeruginosa is scarce.
In 2018 we obtained the first CZA resistant clinical isolate of P. aeruginosa that produced PER-3. During the COVID-19 pandemic, the use of carbapenems and CZA increased significantly and carbapenem-resistant P. aeruginosa increased as well (Allel et al., 2023). In this pandemic period, several PER-producing P. aeruginosa clinical isolates were obtained. This is of major concern because the effectiveness of CZA, approved in 2019 for clinical use in Chile, is being compromised. Our objective was to analyze the contribution of PER to CZA resistance through determining the prevalence of CZA resistance and blaPER gene in non-carbapenemase producing clinical isolates of P. aeruginosa, which were non-susceptible to carbapenems and/or ceftazidime, and to characterize the genetic context of blaPER gene. Moreover, we analyzed the contribution of PER to CZA resistance in a clinical isolate that was induced to lose the blaPER gene and restoration of β-lactam susceptibility was analyzed thereof.
Materials and methods
Clinical isolates
The first clinical isolate of PER-producing P. aeruginosa (MF-1) in the University Hospital of Pontificia Universidad Católica de Chile was obtained in 2018 from blood cultures of a 14-year-old male patient. MF-1 was resistant to CZA (MIC >16 μg/ml), according to Clinical Laboratory Standards Institute (CLSI 2023) breakpoints (Clinical and Laboratory Standards Institute, 2023). CZA was not administered to the patient, but he had received meropenem and vancomycin because of polymicrobial infection with Enterococcus and ESBL-producing Enterobacteriaceae.
In the present work, we screened for all non-carbapenemase producing clinical isolates of P. aeruginosa with a non-susceptible phenotype to ceftazidime and/or carbapenems (imipenem and meropenem) obtained in the Laboratory of Microbiology of the University Hospital between January-December 2021. The total number of P. aeruginosa isolates obtained during this period was 1314, and 287 isolates fulfilled the above-mentioned criteria. The source of the isolates were respiratory specimens (119), urine (91), wounds (33), blood (19), tissue (11), skin (6), abscesses (5), peritoneal fluid (2), and cerebrospinal fluid (1). All 287 isolates had a negative carba-NP test performed according to CLSI recommendations (Clinical and Laboratory Standards Institute, 2023). All isolates were screened for the carbapenemase genes blaKPC, blaOXA-48, blaNDM, blaIMP, blaSPM, blaGES, blaVIM through conventional PCR as described (Wozniak et al., 2021). Only the first isolate of each patient that fulfilled the above-described criteria was included. Surveillance isolates were excluded from this study. Bacterial species were identified through Matrix Assisted Laser Desorption - Time of Flight Mass Spectrometry (MALDI-TOF) (Bruker-Daltonics, Bremen, Germany). All the 287 isolates were screened for blaPER and blaVEB genes through PCR using primers and conditions as described (Fazeli et al., 2015). This study was approved by the Ethics and Biosafety Committee of Pontificia Universidad Católica de Chile.
In vitro evolution of blaPER-3-positive isolates
To induce the loss of blaPER-3, isolate MF-1 (the first PER-producing isolate obtained in 2018) was grown in 25 mL of tryptic soy broth (TSB) (Beckton Dickinson) without antibiotics, and incubated at 37°C or 43°C with constant shaking. The culture broth was daily sub-cultured (dilution 1/100) in fresh medium for 14 days, and each day, 10 µl from the culture broth were seeded in PIA plates (Pseudomonas Isolation Agar) and incubated for 24 h at 37°C. Every following day, a total of 16 colonies were screened through PCR for detection of the blaPER gene. No PER-negative colonies were obtained at 37°C. One PER-negative colony was obtained at 43°C. This in vitro evolved isolate was named MF-2.
Antimicrobial susceptibility testing
Agar dilution method
Susceptibility to CZA, ceftazidime, imipenem, meropenem, cefoperazone/sulbactam, piperacillin/tazobactam, ciprofloxacin, and amikacin of all isolates was determined through the agar dilution method as per CLSI 2023 recommendations and breakpoints (Clinical and Laboratory Standards Institute, 2023). Briefly, bacterial suspensions were adjusted to McFarland 0.5 and dispensed in the seeding-tray wells of a Cathra Replicator System (32 wells). To determine minimum inhibitory concentration (MIC), Mueller-Hinton agar plates (BBL™ BD, Sparks, MD) supplemented with doubling antibiotic concentrations were seeded using 1 mm-pins. To determine the MIC of CZA, the doubling dilution range for ceftazidime spanned from 0.125 μg/ml to 16 μg/ml, with a fixed avibactam concentration of 4 μg/ml. Plates were incubated at 37°C for 18–20 h before reading. Breakpoints used for cefoperazone/sulbactam were those indicated in the package insert of Sulperazon (Pfizer). All assays were performed in triplicate. All antimicrobial compounds were obtained from USP (United States Pharmacopeia), except for ceftazidime that was obtained from Sigma-Aldrich, and avibactam that was provided by Pfizer in the context of a Pure Compound Grant (PCG) ID#75444555 awarded to AW.
Broth microdilution method
Susceptibility of MF-1 and its respective in vitro evolved counterpart MF-2, was determined through broth microdilution using SensiTitre Antimicrobial Susceptibility Testing System DKMGN according to manufacturer`s instructions (Thermo Fisher Scientific, United States). Susceptibility of all PER-positive isolates to aztreonam and ceftolozane/tazobactam was analyzed through broth microdilution. MIC values were determined after incubation for 18–20 h at 37°C. Susceptibility categorization was performed using the breakpoints suggested by CLSI 2023 (Clinical and Laboratory Standards Institute, 2023). Quality control (QC) tests were performed with reference standard strains P. aeruginosa ATCC 27853 and Escherichia coli ATCC 25922. QC MIC values for all standard strains were within acceptable ranges.
Whole-genome sequencing and bioinformatic analysis
Isolate MF-1 was sequenced using long and short read approaches (Illumina and Oxford Nanopore, respectively). Long reads were obtained in a GridION Flow cell at the Garvan Institute of Medical Research (Australia). Short read library preparation was performed using Hackflex protocol (Gaio et al., 2022), and sequencing was conducted through Illumina MiSeq technology at the University of Technology Sydney (Australia). MF-1 genome was assembled using Raven, Flye, and Canu (Murigneux et al., 2020). Finally, assemblies were consolidated using Trycycler, in which short reads were used to polish long reads (Wick et al., 2021). MF-2 and all 14 PER-positive isolates were sequenced through a short read approach. Briefly, sample library was prepared using the Illumina DNA Prep kit and IDT 10 bp UDI indices and sequenced on an Illumina NextSeq-2000. The assembled genomes were annotated using Bakta (Schwengers et al., 2021), and antimicrobial resistance genes were predicted using AMRFinder (Feldgarden et al., 2019). To identify single nucleotide polymorphisms (SNPs) as well as structural differences between parental MF-1 and in vitro evolved MF-2 genomes we used the circularized genome as a reference and mapped the short reads using Breseq (Deatherage and Barrick, 2014). Multi-Locus Sequence Typing (MLST) was performed using the Galaxy-Australia platform (Galaxy Australia (Australian Biocommons and partners), [[NoYear]]). Circular genome visualization was performed using pyCirclize (Shimoyama, 2022). The non-synonymous polymorphisms of genes associated with mutational resistome of P. aeruginosa (López-Causapé et al., 2017) were identifed by calling SNPs with Snippy v4.6.0 software (https://github.com/tseemann/snippy), mapping the trimmed raw reads of each bacterial isolate with respect the PAO1 reference genome (NC_002516.2) (Delgado-Valverde et al., 2024). In addition, amino acid sequences were compared with that of susceptible isolates of the same STs using ClustalW. Amino acid substitutions that were present in PAO1 and/or in the susceptible isolate of the same ST were considered polymorphisms and were not reported as SNPs. The genomes of susceptible isolates were obtained from PATRIC database and their Genome IDs were 1402550.3 (ST309), 652611.13 (ST253, PA14 genome), 1447536.3 (ST395), 1402496.3 (ST235) and 1163395.3 (ST41). The impact of amino acid substitutions in protein functionality was analyzed with SIFT algorithm (Sorting Intolerant From Tolerant) allowing a prediction of functional impact of mutations as “deleterious” (score ≤ 0.05) or “neutral” (score >0.05) (Vaser et al., 2016).
Results
Susceptibility to CZA and blaPER gene occurrence
A total of 287 non-carbapenemase-producing P. aeruginosa isolates that were non-susceptible to ceftazidime and/or carbapenems, were included in the study. All isolates had a negative PCR for carbapenemase genes. Twenty-six of them (9.1%) were resistant to CZA (MIC≥16 μg/ml), as determined through agar dilution. Thirteen of the 26 CZA-resistant isolates (50%) carried the blaPER gene. Isolate N° 1224 carried blaPER gene but was susceptible to CZA (MIC=4 μg/ml). The prevalence of blaPER among the cohort analyzed was 4.8% (14/287), whereas 92.8% (13/14) of PER-positive isolates were CZA-resistant. To compare MIC values between PER-positive and PER-negative isolates, the following isolate after each PER-positive isolate was selected. PER-positive isolates had MIC values for CZA, amikacin, gentamicin, ceftazidime, meropenem and ciprofloxacin that were significantly higher than PER-negative isolates (Table 1). In contrast, MICs for cefoperazone/sulbactam, piperacillin/tazobactam, colistin and imipenem were not significantly different between PER-positive and PER-negative isolates (Table 1). All PER-positive isolates were further analyzed for their susceptibility to ceftolozane/tazobactam and aztreonam through broth microdilution. Thirteen out of fourteen PER-positive isolates were resistant to ceftolozane/tazobactam, whereas isolate 1224 was intermediately resistant (MIC = 8 μg/ml), and all isolates were resistant to aztreonam.
Genomic analysis of PER-positive and PER-negative isolates
All the 14 PER-positive isolates were sequenced through short reads WGS. MLST analysis classified them as sequence type (ST) 309. The blaPER gene of all the isolates was PER-3 type. PlasmidSpades was run for all PER-positive isolates and no plasmids were found. In all isolates except 1368, the blaPER-3 gene was associated with an insertion sequence formed by an ISCR1 gene that encodes a site-specific recombinase and two adjacent 28 bp-target sequences (direct repeats) that are recognized by the ISCR1 recombinase (Arduino et al., 2002; Partridge and Hall, 2003). Isolate 1368 had its ISCR1 recombinase gene in a different contig. Downstream the blaPER-3 gene all isolates except 1263 and 1486 carried genes coding for an ABC transporter ATP-binding protein and an ISPa33-family transposase (Figure 1). According to AMRFinder, all 14 PER-positive isolates carried the resistance genes blaPER-3 and blaOXA-4 (Figure 1). All isolates carried the same alleles for the intrinsic P. aeruginosa β-lactamases AmpC and OXA-50: the blaPDC (AmpC) allele was PDC-19a and the blaOXA-50 allele was OXA-1035. Mutations in chromosomal genes known to be associated to CZA resistance were analyzed and are referred as mutational resistome (López-Causapé et al., 2017) (Table 2). Genes analyzed were those coding for PBPs 1b, 3 and 4, MexAB-OprM regulators MexZ, MexR, NalC and NalD, AmpC regulators AmpD and AmpR, and OprD porin. Sequences were compared to wild type sequences of PAO1 and to CZA susceptible isolates of the same STs. Several amino acid substitutions were found in PER-positive ST309 isolates respect to PAO1, but most of them were also found in the CZA susceptible ST309 strain, suggesting that they are ST-related polymorphisms, and they were not reported here. Major alterations not observed in the CZA susceptible strain i.e., insertions, premature stop codons, amino acid substitutions, or frameshift mutations were detected in MexZ of isolates 1673, 1169, 1263, 1270 and 1368. MexR, NalD and NalC had no major alterations except for isolate 1263 that had the amino acid substitution T11N in NalD (Table 2). Penicillin-binding proteins PBP1b, PBP3 and PBP4, and regulators of AmpC expression AmpD and AmpR, and OprD porin had no major alterations in none of the PER-positive isolates. The CZA susceptible isolate 1224 had no major alterations in the genes analyzed (Table 2). Moreover, this isolate had an intact blaPER-3 gene and promoter and has the same resistance genes as the other PER-positive isolates. The analysis of mutational resistome showed that this isolate had amino acid changes in PmrA and CprS (E191K and D141N respectively), a response regulator and a sensor kinase respectively, of two-component systems involved in lipopolysaccharide modification causing polymyxin resistance (Fernández et al., 2012). Regarding resistance to non-β-lactam antibiotics, notably, all PER-positive isolates had two genes for aminoglycoside modifying enzymes that may account for their resistance to amikacin and gentamicin (Figure 1). Regarding genes associated with fluoroquinolone resistance, one isolate had an amino acid change in GyrA (D87N) and 4 isolates had an amino acid change in ParC (data not shown). Besides this, 3 isolates had a crpP-like gene that is known to contribute to ciprofloxacin resistance (Chávez-Jacobo et al., 2018) (Figure 1).
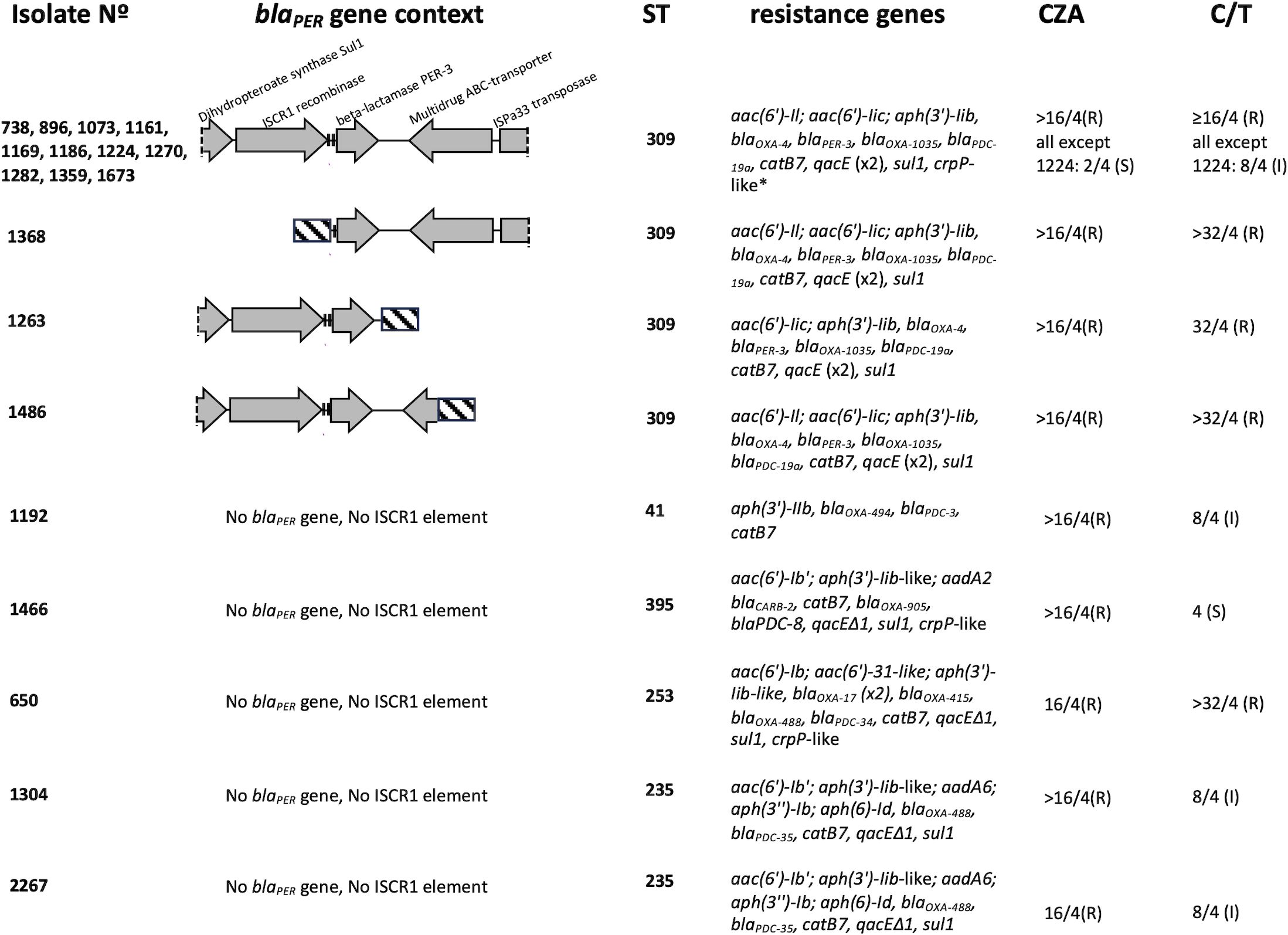
Figure 1 Genetic characteristics of PER-positive and PER-negative isolates. All PER-positive isolates except 1368 had an ISCR1 element upstream blaPER-3 gene composed by the ISCR1 gene coding for a site-specific recombinase and two adjacent 28bp-target sequences (vertical bars). *detected only in isolate 1673. Striped bars represent sequences that are non-homologous among isolates. CZA, ceftazidime-avibactam; C/T, ceftolozane-tazobactam.
Five PER-negative CZA resistant isolates were analyzed through short reads WGS. Their sequence types were ST41, ST235 (two isolates), ST395 and ST253. The blaPDC alleles were PDC-3, PDC-35 (two isolates), PDC-8 and PDC-34, respectively. PER-negative isolates carried genes for narrow-spectrum β-lactamases: isolate 650 had two copies of blaOXA-17 (OXA-10-like) and one copy of blaOXA-415 (OXA-2-like); isolate 1466 had a blaCARB-2 gene; isolates 1304, 2267 and 650 carried blaOXA-488 (OXA-50-like) (Figure 1). In contrast to PER-positive isolates, the mutational resistome of PER-negative isolates showed that all isolates had one major alteration in at least one of the genes analyzed (Table 2). Isolates 1304 and 2267 had a mutation in the stop codon of MexZ, such that the protein was 36 amino acids longer than the wild type one and isolate 650 had the amino acid substitution T5A. MexR had the amino acid substitution R63H in isolate 1192. NalD had a frameshift mutation in position V151 in isolate 1466, and it was truncated in isolates 1304 and 1267. In contrast to PER-positive isolates, PER-negative isolates had major alterations in penicillin-binding proteins as specified in Table 2. Isolates 1304 and 2267 had the amino acid change E120K in AmpD (Table 2). Only isolate 1192 had a wild type OprD, the other four isolates had a frameshift mutation or were truncated.
In vitro evolved isolate that lost the blaPER-3 gene became susceptible to CZA and other β-lactams
To investigate the contribution of PER-3 to β-lactam resistance, loss of the blaPER-3 gene was induced in the PER-3-positive isolate MF-1, by successive subcultures at 37°C or 43°C without antibiotics. Colony PCR was performed to determine the presence/absence of the blaPER-3 gene. No PER-negative colonies were obtained at 37°C after 14 days of subcultures. In contrast, a PER-negative colony was obtained the first day of subculture at 43°C. Loss of blaPER-3 gene was determined by a negative amplification in the blaPER-targeted PCR. This in vitro evolved isolate was designated MF-2. Susceptibility of the parental isolate and it’s in vitro evolved isogenic counterpart was determined through broth microdilution and is shown in Table 2. Loss of blaPER restored the susceptibility to ceftazidime, CZA and ceftolozane/tazobactam in isolate MF-2. Furthermore, MICs of aztreonam and cefepime decreased from resistant to intermediately resistant (Table 3). In contrast, MICs of piperacillin/tazobactam and carbapenems as well as the other antimicrobials tested were not affected upon loss of blaPER-3 (Table 3).
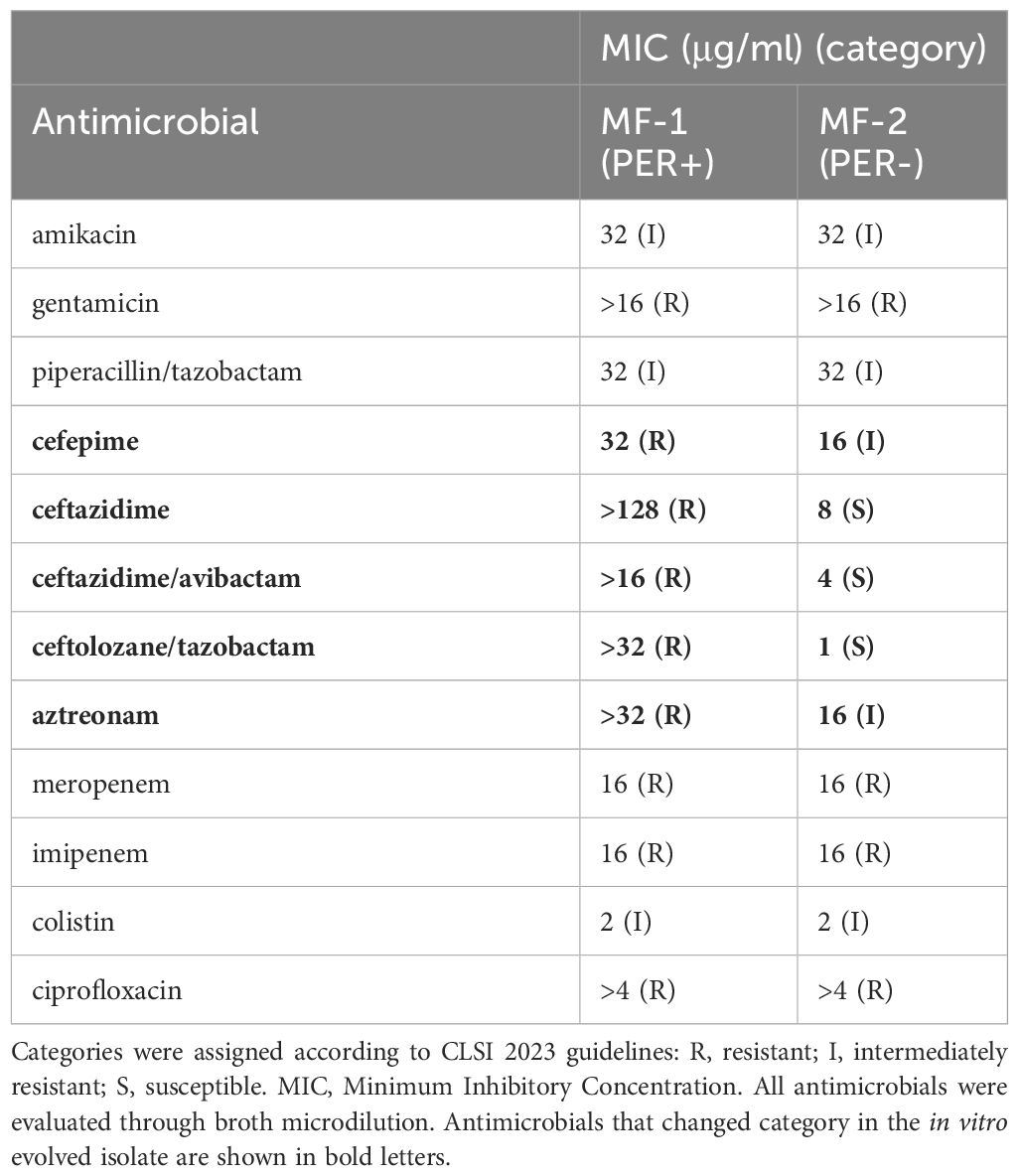
Table 3 Antimicrobial susceptibility profile of parental PER-positive isolate MF-1 and its in vitro evolved PER-negative counterpart MF-2.
Comparative genomic analysis of parental and in vitro evolved isolate
MF-1 was sequenced through short and long reads WGS to fully close its genome. The hybrid assembly of MF-1 produced a single circularized contig of 7,043,257 bp that corresponded to the bacterial chromosome (Figure 2A). The blaPER-3 gene was associated to an ISCR1 element, the same found in the other PER-positive isolates (Figures 1, 2B). Sequence analysis showed that blaPER-3 gene was embedded in a particular type of class 1 integron harboring two copies of the 3`CS (Conserved Sequence, containing the sul1 gene), with blaPER-3 gene located between both 3`CS copies (3`CS-1 and 3`CS-2) (Figure 2B) (Partridge and Hall, 2003). MF-2 was sequenced through short reads WGS. To compare MF-1 and MF-2 genomes, MF-2 reads were mapped against the closed chromosome of MF-1. MF-2 short reads covered the MF-1 genome entirely, except for a 46,933 bp fragment (Figure 2A), that was lost in MF-2 isolate. This DNA fragment included the blaPER-3 gene and several genes encoding efflux pumps, ABC transporters and transcriptional regulators, among other traits (Figure 2B). The complete list of genes included in the 47 kb fragment is shown in Supplementary Figure S1. MF-2 harbored the same resistance genes as MF-1, except for the absence of blaPER-3. In addition, mapping analysis identified 44 SNPs between MF-1 and MF-2: 6 intergenic, 1 nonsense, 12 non-synonymous, 23 in pseudogenes, and 2 synonymous (Supplementary Figure S2). However, none of these polymorphisms was identified in genes previously associated with CZA resistance or their regulatory elements. Sequences of MF-1 and MF-2 were deposited in the Genbank database with accession numbers CP117527 and NZ_JARFMG000000000 respectively.
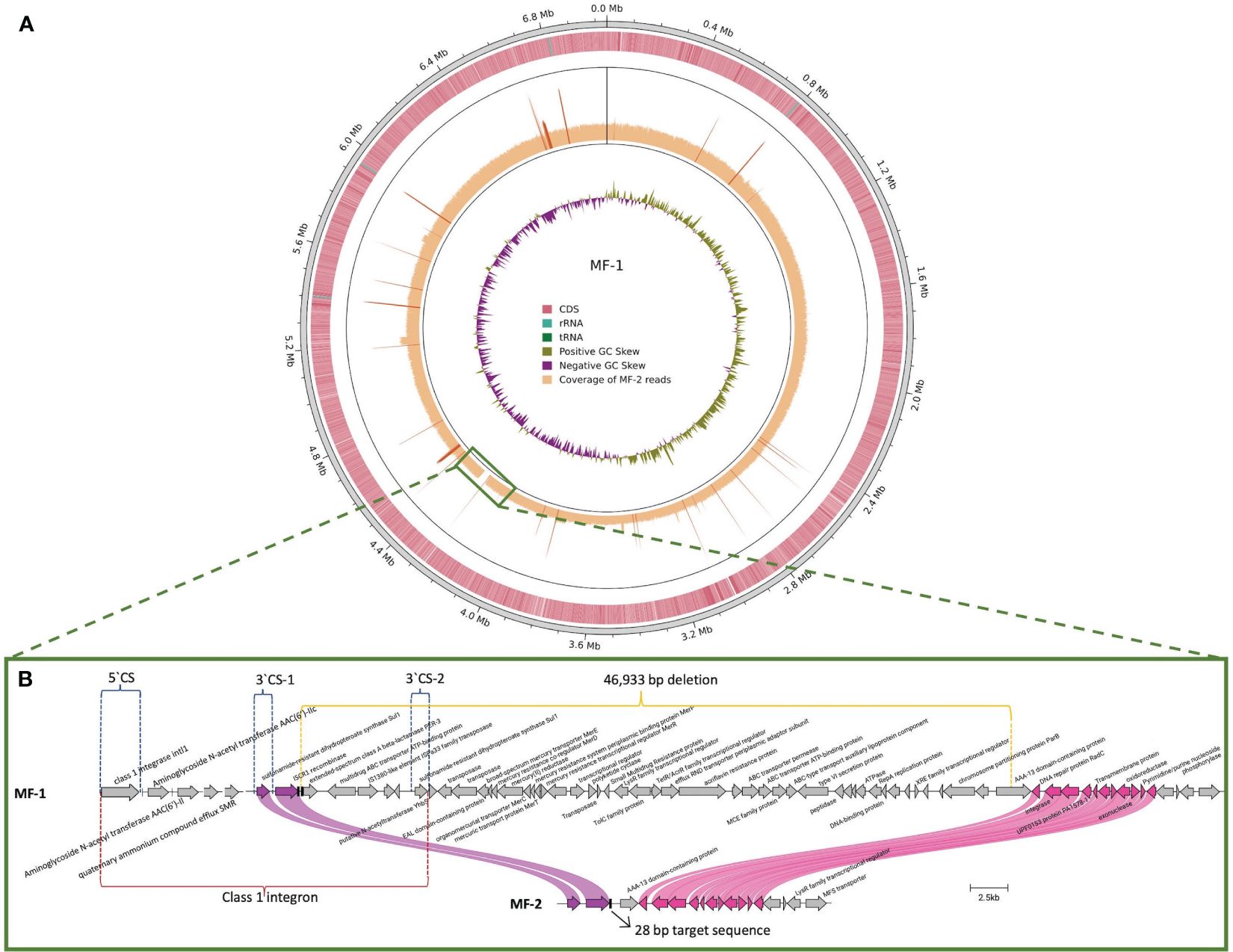
Figure 2 Genomic regions involved in loss of blaPER-3 gene in isolate MF-1 and in vitro evolved isolate MF-2. (A) MF-1 chromosome was obtained through hybrid alignment of long+short reads. Coverage of MF-2 short reads mapped against MF-1 chromosome is shown. The green square indicates the uncovered region. (B) The genetic content of the 46,933 bp deletion is shown. The blaPER-3 gene is embedded in a class 1 integron (red lines). The 3`CS (conserved segment) of the integron is duplicated as 3`CS-1 and 3`CS-2 and the 5`CS includes the class 1 integrase (blue lines). Purple and pink curved lines show the upstream and downstream limits of the deletion. Black vertical bars: 28 bp-target sequences of ISCR1.
Discussion
The prevalence of blaPER among the isolates studied here was 4.8% (14/287), whereas it was 15.2% (14/92) among ceftazidime non-susceptible isolates. In contrast, the prevalence of blaPER among ceftazidime non-susceptible isolates reported in other regions is considerably higher, namely 29% in Europe (Mendes et al., 2019), 86% in Turkey (Aktaş et al., 2005), and 81% in Iran (Haghighi and Reza Goli, 2022). Notably, we did not detect VEB and GES, which are highly prevalent in regions like Asia-Pacific and Thailand, with frequencies of 80% and 100%, respectively (Karlowsky et al., 2018).
In this work 13 of 14 PER-positive isolates were CZA resistant. Moreover, the in vitro evolved isolate that lost blaPER-3 became susceptible to CZA, ceftolozane/tazobactam and other antipseudomonal β-lactams. Although the SNP analysis showed that in vitro evolved isolate MF-2 did not acquire mutations in genes associated to CZA resistance, it is reasonable to think that other mutations induced by high temperature could be contributing to susceptibility to CZA. The evidence presented in this work about clinical isolates reinforces the results obtained with isogenic mutants expressing PER that indicate that PER-3 is the main responsible for the resistance to CZA and may compromise the success of new lines of therapies.
CZA resistance in PER-negative isolates is most likely associated with narrow-spectrum β-lactamases and/or mutations in genes associated to CZA resistance i.e. mexR, mexZ, nalD, ponB, ftsI, dacB, ampD, oprD. OXA-488 and CARB-2 have been reported in CZA resistant P. aeruginosa clinical isolates (Sid Ahmed et al., 2022; Babouee Flury et al., 2023). OXA-17 is an OXA-10-like oxacillinase that has been associated with resistance to CZA and ceftolozane/tazobactam (Sid Ahmed et al., 2022). The mutational resistome of PER-negative isolates showed that they had mutations in AmpC, efflux pump regulators, PBPs and OprD porin; some of them have been reported in CZA resistant P. aeruginosa isolates that did not have other carbapenemases (Babouee Flury et al., 2023). Isolate 1192 has an AmpC variant not associated with CZA resistance and has no narrow-spectrum β-lactamases but it has a mutation in PBP4 that was predicted as deleterious. PBP4 mutants were shown to have increased expression of AmpC which may account for its CZA resistance (Zamorano et al., 2010). These findings are in accordance with the multifactorial nature of CZA resistance, particularly in P. aeruginosa; several determinants acting together is needed to achieve resistance, e.g., narrow-spectrum β-lactamases or carbapenemases, increased efflux pump expression, and altered permeability of antimicrobials (Wang et al., 2020; Babouee Flury et al., 2023). The fact that the CZA susceptible isolate 1224 had the same resistance genes and similar mutational resistome as the other PER-positive CZA resistant isolates, indicates that other mechanisms may be related to CZA susceptibility. For instance, mutations in PmrA and CprS that modulate the composition of lipopolysaccharide in the outer membrane, could confer hyper-susceptibility to CZA in isolate 1224.
The role of ISCR1 in blaPER mobilization has been reported in a clinical isolate of Vibrio cholerae (Wu et al., 2015). The excision of the 47 kb fragment in MF-2 occurred between the two 28 bp target sequences of ISCR1, suggesting that this recombinase is likely involved in this deletion. The loss of blaPER-3 in MF-1 by the action of ISCR1 recombinase suggests that the stress conditions imposed by high temperature (43°C) may have induced the IS-dependent mobilization of resistance genes (Guerin et al., 2009). Although further research needs to be carried out, it is remarkable that stability of chromosomal resistance genes, which are usually considered more stable than plasmid-located ones, could be compromised in response to temperature-induced stress.
The fact that all PER-3-producing isolates belong to the same clone (ST309) and have the same resistance genes and genetic environment of the blaPER-3 gene, suggests that ST309 is a relevant vehicle of PER-3. Moreover, our results show that PER-3-producing ST309 lineage is associated with resistance to amikacin, meropenem, ciprofloxacin, and ceftolozane/tazobactam. Intensive use of CZA may select for the PER-3 variant, increasing CZA resistance levels further, and exacerbating the lack of options for the treatment of infections caused by multidrug resistant bacteria. This is particularly serious in our setting because there are no therapeutic options for infections caused by organisms like this; cefiderocol or meropenem/vaborbactam are not available in Chile yet. ST309 is not among the top high-risk clones described worldwide that include ST111, ST235, ST175, ST375 (del Barrio-Tofiño et al., 2020). However, ST309 is now being considered a newly emerging high-risk clone: it has been reported in severe infections associated with resistance to CZA and ceftolozane/tazobactam in Mexico, United States (Khan et al., 2019), and Brazil (Fonseca et al., 2022). It is of major importance to know the main circulating STs, resistance mechanisms and genetic platforms to detect the potential emergence of new high-risk clones and predict what could happen in other regions.
Data availability statement
The datasets presented in this study can be found in online repositories. The names of the repository/repositories and accession number(s) can be found below:https://www.ncbi.nlm.nih.gov/genbank/, CP117527 https://www.ncbi.nlm.nih.gov/genbank/, NZ_JARFMG000000000.
Ethics statement
The studies involving humans were approved by Comité Ético Científico de Ciencias de la salud UC. The studies were conducted in accordance with the local legislation and institutional requirements. The human samples used in this study were acquired from primarily isolated as part of your previous study for which ethical approval was obtained. Written informed consent for participation was not required from the participants or the participants’ legal guardians/next of kin in accordance with the national legislation and institutional requirements.
Author contributions
KS: Data curation, Formal analysis, Methodology, Project administration, Validation, Writing – original draft. MA-R: Conceptualization, Formal analysis, Investigation, Methodology, Project administration, Resources, Software, Supervision, Validation, Writing – review & editing. JU: Data curation, Formal analysis, Investigation, Methodology, Resources, Software, Visualization, Writing – review & editing. JO-P: Formal analysis, Investigation, Methodology, Resources, Supervision, Writing – review & editing. VQ: Data curation, Investigation, Methodology, Writing – review & editing. BB: Data curation, Formal analysis, Funding acquisition, Investigation, Methodology, Software, Visualization, Writing – review & editing. LR: Data curation, Investigation, Methodology, Project administration, Resources, Supervision, Writing – review & editing. JM: Conceptualization, Formal analysis, Funding acquisition, Investigation, Project administration, Resources, Supervision, Writing – review & editing. PG: Conceptualization, Formal analysis, Funding acquisition, Project administration, Resources, Supervision, Validation, Writing – review & editing. AW: Conceptualization, Data curation, Formal analysis, Funding acquisition, Investigation, Methodology, Project administration, Resources, Supervision, Validation, Writing – original draft.
Funding
The author(s) declare financial support was received for the research, authorship, and/or publication of this article. This work was supported by research funds from SENTRY (Antimicrobial Resistance Surveillance Program), the Red de Salud UC-Christus and the Department of Clinical Laboratories at the School of Medicine of Pontificia Universidad Católica de Chile. Funding for whole-genome sequencing was obtained from the Key Technology Partnership Program from the University of Technology Sydney, and from Instituto de Ciencias e Innovación en Medicina (ICIM), Facultad de Medicina, Universidad del Desarrollo. Santiago, Chile. Pfizer provided avibactam in the context of a Pure Compound Grant (PCG) ID#75444555 awarded to AW. This work was partially funded by the Agencia Nacional de Investigación y Desarrollo (ANID, Chile) through FONDECYT Postoctoral Grant N°3200798 awarded to MA-R. JU was funded by ANID-Fondecyt Regular 1221209 and ANID-Anillo ATE 220061.
Acknowledgments
We thank the staff of the Microbiology Laboratory of the Red de Salud UC-CHRISTUS for their help in the technical aspects of this work. We also thank the Millennium Science Initiative of the Ministry of Economy, Development and Tourism, Government of Chile. We would like to express our gratefulness to the “Pseudomonas group”, a collaborative team including researchers from various Chilean Universities: Pontificia Universidad Católica de Chile, Pontificia Universidad Católica de Valparaíso, Universidad de Concepción; Universidad del Desarrollo. We are very grateful to Joyce To and Kay Anantanawat from the UTS sequencing facility, who helped with the wet-lab work for WGS.
Conflict of interest
The authors declare that the research was conducted in the absence of any commercial or financial relationships that could be construed as a potential conflict of interest.
Publisher’s note
All claims expressed in this article are solely those of the authors and do not necessarily represent those of their affiliated organizations, or those of the publisher, the editors and the reviewers. Any product that may be evaluated in this article, or claim that may be made by its manufacturer, is not guaranteed or endorsed by the publisher.
Supplementary material
The Supplementary Material for this article can be found online at: https://www.frontiersin.org/articles/10.3389/fcimb.2024.1410834/full#supplementary-material
References
Adewoye, L., Sutherland, A., Srikumar, R., Poole, K. (2002). The mexR repressor of the mexAB-oprM multidrug efflux operon in Pseudomonas aeruginosa: characterization of mutations compromising activity. J. Bacteriol. 184, 4308–4312. doi: 10.1128/JB.184.15.4308-4312.2002
Aktaş, Z., Poirel, L., Şalcloğlu, M., Ozcan, P. E., Midilli, K., Bal, C., et al. (2005). PER-1- and OXA-10-like β-lactamases in ceftazidime-resistant Pseudomonas aeruginosa isolates from intensive care unit patients in Istanbul, Turkey. Clin. Microbiol. Infect. 11, 193–198. doi: 10.1111/j.1469–0691.2004.01067.x
Allel, K., Peters, A., Conejeros, J., Martínez, J. R. W., Spencer-Sandino, M., Riquelme-Neira, R., et al. (2023). Antibiotic consumption during the coronavirus disease 2019 pandemic and emergence of carbapenemase-producing klebsiella pneumoniae lineages among inpatients in a Chilean hospital: A time-series study and phylogenomic analysis. Clin. Infect. Dis. 77, S20–S28. doi: 10.1093/cid/ciad151
Arduino, S. M., Roy, P. H., Jacoby, G. A., Orman, B. E., Pineiro, S. A., Centron, D. (2002). blaCTX-M-2 is located in an unusual class 1 integron (In35) which includes Orf513. Antimicrob. Agents Chemother. 46, 2303–2306. doi: 10.1128/AAC.46.7.2303-2306.2002
Babouee Flury, B., Bösch, A., Gisler, V., Egli, A., Seiffert, S. N., Nolte, O., et al. (2023). Multifactorial resistance mechanisms associated with resistance ceftazidime-avibactam in clinical Pseudomonas aeruginosa isolates from Switzerland. 2023 Front. Cell. Infect. Microbiol. 13. doi: 10.3389/fcimb.2023.1098944
Castanheira, M., Doyle, T. B., Smith, C. J., Mendes, R. E., Sader, H. S. (2019). Combination of MexAB-OprM overexpression and mutations in efflux regulators, PBPs and chaperone proteins is responsible for ceftazidime/avibactam resistance in Pseudomonas aeruginosa clinical isolates from US hospitals. J. Antimicrob. Chemother. 74, 2588–2595. doi: 10.1093/jac/dkz243
Chalhoub, H., Sáenz, Y., Nichols, W. W., Tulkens, P. M., Van Bambeke, F. (2018). Loss of activity of ceftazidime-avibactam due to MexAB-OprM efflux and overproduction of AmpC cephalosporinase in Pseudomonas aeruginosa isolated from patients suffering from cystic fibrosis. Int. J. Antimicrob. Agents 52, 697–701. doi: 10.1016/j.ijantimicag.2018.07.027
Chávez-Jacobo, V. M., Hernández-Ramírez, K. C., Romo-Rodríguez, P., Pérez-Gallardo, R. V., Campos-García, J., Gutiérrez-Corona, J. F., et al. (2018). CrpP Is a Novel Ciprofloxacin-Modifying Enzyme Encoded by the Pseudomonas aeruginosa pUM505 Plasmid. Antimicrob. Agents Chemother. 62, e02629–e02617. doi: 10.1128/AAC.02629-17
Clinical and Laboratory Standards Institute (2023). Performance Standards for Antimicrobial Susceptibility Testing. 33rd Edition (USA: CLSI Supplement M100).
Deatherage, D. E., Barrick, J. E. (2014). Identification of mutations in laboratory-evolved microbes from next-generation sequencing data using breseq. Methods Mol. Biol. 1151, 165–188. doi: 10.1007/978–1-4939–0554-6_12
del Barrio-Tofiño, E., López-Causapé, C., Oliver, A. (2020). Pseudomonas aeruginosa epidemic high-risk clones and their association with horizontally-acquired β-lactamases: 2020 update. Int. J. Antimicrob. Agents 56, 106196. doi: 10.1016/j.ijantimicag.2020.106196
Delgado-Valverde, M., Portillo-Calderón, I., Alcalde-Rico, M., Conejo, M. C., Hidalgo, C., Del Toro Esperón, C. (2024). Activity of imipenem/relebactam and comparators against KPC-producing Klebsiella pneumoniae and imipenem-resistant Pseudomonas aeruginosa. Eur. J. Clin. Microbiol. Infect. Dis. 43, 445–457. doi: 10.1007/s10096-023-04735-1
Fazeli, H., Kamali Dolatabadi, R., Taraghian, A., Isfahani, B. N., Moghim, S. (2015). Genetic Characterization of blaSHV/VEB/PER Genes in ESBL-producing MDR Klebsiella pneumoniae Strains Isolated from Patients in Isfahan, Iran. Eur. Online J. Of Natural And Soc. Sciences 4, 191–202. Avaiable at: https://api.semanticscholar.org/CorpusID:31766986.
Feldgarden, M., Brover, V., Haft, D. H., Prasad, A. B., Slotta, D. J., Tolstoy, I., et al. (2019). Validating the AMRFinder tool and resistance gene database by using antimicrobial resistance genotype-phenotype correlations in a collection of isolates. Antimicrob. Agents Chemother. 63, e00483-19. doi: 10.1128/AAC.00483-19
Fernández, L., Jenssen, H., Bains, M., Wiegand, I., Gooderham, W. J., Hancock, R. E. (2012). The two-component system CprRS senses cationic peptides and triggers adaptive resistance in Pseudomonas aeruginosa independently of ParRS. Antimicrob. Agents Chemother. 56, 6212–6222. doi: 10.1128/AAC.01530-12
Fonseca, ÉL, Morgado, S. M., Caldart, R. V., Freitas, F., Vicente, A. C. P. (2022). Emergence of a VIM-2-producing extensively drug-resistant (XDR) Pseudomonas aeruginosa ST309 in South America: a comparative genomic analysis. Int. J. Antimicrob. Agents 59, 106507. doi: 10.1016/j.ijantimicag.2021.106507
Gaio, D., Anantanawat, K., To, J., Liu, M., Monahan, L., Darling, A. E. (2022). Hackflex: low-cost, high-throughput, Illumina Nextera Flex library construction. Microb. Genom. 8, e000744. doi: 10.1099/mgen.0.000744
Galaxy Australia (Australian Biocommons and partners). Funded by Bioplatforms Australia and the Australian research data commons (Melbourne, Australia: The University of Melbourne and Queensland Government RICF funding). doi: 10.47486/PL105
Guerin, E., Cambray, G., Sanchez-Alberola, N., Campoy, S., Erill, I., Da Re, S., et al. (2009). The SOS response controls integron recombination. Sci. 22 324, 1034. doi: 10.1126/science.1172914
Haghighi, S., Reza Goli, H. (2022). High prevalence of blaVEB, blaGES and blaPER genes in β-lactam resistant clinical isolates of Pseudomonas aeruginosa. AIMS Microbiol. 8, 153–166. doi: 10.3934/microbiol.2022013
Karlowsky, J. A., Kazmierczak, K. M., Bouchillon, S. K., Boudewijn, L. M., Stone, G. G., Sahm, D. F. (2018). In Vitro Activity of Ceftazidime-Avibactam against Clinical Isolates of Enterobacteriaceae and Pseudomonas aeruginosa Collected in Asia-Pacific Countries: Results from the INFORM Global Surveillance Program, 2012 to 2015. Antimicrob. Agents Chemother. 62, e2517–e2569. doi: 10.1128/AAC.02569-17
Kazmierczak, K. M., de Jonge, B., Stone, G. G., Sahm, D. F. (2018). In Vitro Activity of Ceftazidime/Avibactam against Isolates of Pseudomonas aeruginosa Collected in European Countries: INFORM Global Surveillance 2012–15. J. Antimicrob. Chemother. 73, 2777–2781. doi: 10.1093/jac/dky267
Khan, A., Tran, T. T., Rios, R., Hanson, B., Shropshire, W. C., Sun, Z., et al. (2019). Extensively drug-resistant pseudomonas aeruginosa ST309 harboring tandem guiana extended spectrum β-lactamase enzymes: A newly emerging threat in the United States. Open Forum Infect. Dis. 6, ofz273. doi: 10.1093/ofid/ofz273
López-Causapé, C., Sommer, L. M., Cabot, G., Rubio, R., Ocampo-Sosa, A. A., Johansen, H. K., et al. (2017). Evolution of the Pseudomonas aeruginosa mutational resistome in an international Cystic Fibrosis clone. Sci. Rep. 7, 5555. doi: 10.1038/s41598–017-05621–5
Mendes, R. E., Castanheira, M., Woosley, L. N., Stone, G. G., Bradford, P. A., Flamm, R. K. (2019). Characterization of β-Lactamase Content of Ceftazidime-Resistant Pathogens Recovered during the Pathogen-Directed Phase 3 REPRISE Trial for Ceftazidime-Avibactam: Correlation of Efficacy against β-Lactamase Producers. Antimicrob. Agents Chemother. 63, e02655–e02618. doi: 10.1128/AAC.02655-18
Mojica, M. F., de la Cadena, E., García-Betancur, J. C., Porras, J., Novoa-Caicedo, I., Páez-Zamora, L., et al. (2023). Molecular mechanisms of resistance to ceftazidime/avibactam in clinical isolates of enterobacterales and pseudomonas aeruginosa in latin American hospitals. mSphere 8, e0065122. doi: 10.1128/msphere.00651-22
Murigneux, V., Rai, S. K., Furtado, A., Bruxner, T. C., Tian, W., Harliwong, I., et al. (2020). Comparison of long-read methods for sequencing and assembly of a plant genome. GigaScience 9, giaa146. doi: 10.1093/gigascience/giaa146
Ortiz de la Rosa, J. M., Nordmann, P., Poirel, L. (2019). ESBLs and resistance to ceftazidime/avibactam and ceftolozane/tazobactam combinations in Escherichia coli and Pseudomonas aeruginosa. J. Antimicrob. Chemother. 74, 1934–1939. doi: 10.1093/jac/dkz149
Papp-Wallace, K. M., Mack, A. R., Taracila, M. A., Bonomo, R. A. (2020). Resistance to novel β-lactam-β-lactamase inhibitor combinations: the “Price of progress”. Infect. Dis. Clin. North Am 34, 773–819. doi: 10.1016/j.idc.2020.05.001
Partridge, S. R., Hall, R. M. (2003). In34, a complex In5 family class 1 integron containing orf513 and dfrA10. Antimicrob. Agents Chemother. 47, 342–349. doi: 10.1128/AAC.47.1.342-349.2003
Philippon, A., Slama, P., Dény, P., Labia, R. (2016). A structure-based classification of class A β-lactamases, a broadly diverse family of enzymes. Clin. Microbiol. Rev. 29, 29–57. doi: 10.1128/CMR.00019-15
Poirel, L., Ortiz de la Rosa, J. M., Sadek, M., Nordmann, P. (2022).Impact of Acquired Broad-Spectrum β-Lactamases on Susceptibility to Cefiderocol and Newly Developed β-Lactam/β-Lactamase Inhibitor Combinations in Escherichia coli and Pseudomonas aeruginosa. Antimicrob. Agents Chemother. 66, e0003922. doi: 10.1128/aac.00039–22
Ruedas-López, A., Alonso-García, I., Lasarte-Monterrubio, C., Guijarro-Sánchez, P., Gato, E., Vázquez-Ucha, J. C., et al. (2022). Selection of AmpC β-Lactamase Variants and Metallo-β-Lactamases Leading to Ceftolozane/Tazobactam and Ceftazidime/Avibactam Resistance during Treatment of MDR/XDR Pseudomonas aeruginosa Infections. Antimicrob. Agents Chemother. 66, e0206721. doi: 10.1128/AAC.02067–21
Ruggiero, M., Papp-Wallace, K. M., Brunetti, F., Barnes, M. D., Bonomo, R. A., Gutkind, G., et al. (2019). Structural insights into the inhibition of the extended-spectrum β-lactamase PER-2 by avibactam. Antimicrob. Agents Chemother. 63, e00487–e00419. doi: 10.1128/AAC.00487-19
Sanz-García, F., Hernando-Amado, S., Martínez, J. L. (2018). Mutation-Driven Evolution of Pseudomonas aeruginosa in the Presence of either Ceftazidime or Ceftazidime-Avibactam. Antimicrob. Agents Chemother. 62, e01379–e01318. doi: 10.1128/AAC.01379-18
Schwengers, O., Jelonek, L., Dieckmann, M. A., Beyvers, S., Blom, J., Goesmann, A. (2021). Bakta: rapid and standardized annotation of bacterial genomes via alignment-free sequence identification. Microb. Genom. 7, 685. doi: 10.1101/2021.09.02.458689
Shimoyama, Y. (2022). pyCirclize: Circular visualization in Python [Computer software]. Available online at: https://github.com/moshi4/pyCirclize.
Sid Ahmed, M. A., Khan, F. A., Hadi, H. A., Skariah, S., Sultan, A. A., Salam, A., et al. (2022). Association of blaVIM-2, blaPDC-35, blaOXA-10, blaOXA-488 and blaVEB-9 β-Lactamase Genes with Resistance to Ceftazidime-Avibactam and Ceftolozane-Tazobactam in Multidrug-Resistant Pseudomonas aeruginosa. Antibiotics (Basel) 11, 130. doi: 10.3390/antibiotics11020130
Vaser, R., Adusumalli, S., Leng, S., Sikic, M., Ng, P. (2016). SIFT missense predictions for genomes. Nat. Protoc. 11, 1–9. doi: 10.1038/nprot.2015.123
Wang, Y., Wang, J., Wang, R., Cai, Y. (2020). Resistance to ceftazidime–avibactam and underlying mechanisms. J. Glob. Antimicrob. Res. 22, 18–27. doi: 10.1016/j.jgar.2019.12.009
Wick, R. R., Judd, L. M., Cerdeira, L. T., Hawkey, J., Méric, G., Vezina, B., et al. (2021). Trycycler: consensus long-read assemblies for bacterial genomes. Genome Biol. 22, 266. doi: 10.1186/s13059-021-02483-z
Wozniak, A., Rodríguez, N., Alcalde-Rico, M., Castillo, C., García, P. (2021). First clinical isolate of Pseudomonas aeruginosa producing Sao Paulo metallo-β-lactamase (SPM-1) in a Chilean patient. Rev. chil. infectol. 38, 724–726. doi: 10.4067/s0716-10182021000500724
Wu, J., Xie, L., Zhang, F., Ni, Y., Sun, J. (2015). Molecular characterization of ISCR1-mediated blaPER-1 in a non-O1, non-O139 Vibrio cholerae strain from China. Antimicrob. Agents Chemother. 59, 4293–4295. doi: 10.1128/AAC.00166-15
Xie, L., Wu, J., Zhang, F., Han, L., Guo, X., Ni, Y., et al. (2016). Molecular epidemiology and genetic characteristics of various blaPER genes in Shanghai, China. Antimicrob. Agents Chemother. 60, 3849–38453. doi: 10.1128/AAC.00258-16
Zamorano, L., Moyá, B., Juan, C., Oliver, A. (2010). Differential β-lactam resistance response driven by ampD or dacB (PBP4) inactivation in genetically diverse Pseudomonas aeruginosa strains. J. Antimicrob. Chemother. 65, 1540–1542. doi: 10.1093/jac/dkq142
Zamudio, R., Hijazi, K., Joshi, C., Aitken, E., Oggioni, M. R., Gould, I. M. (2019). Phylogenetic analysis of resistance to ceftazidime/avibactam, ceftolozane/tazobactam and carbapenems in piperacillin/tazobactam-resistant Pseudomonas aeruginosa from cystic fibrosis patients. Int. J. Antimicrob. Agents 53, 774–780. doi: 10.1016/j.ijantimicag.2019.02.022
Keywords: Pseudomonas aeruginosa, blaPER-3 gene, PER-3 extended-spectrum β-lactamase, ceftazidime/avibactam resistance, mutations conferring CZA resistance
Citation: Soto KD, Alcalde-Rico M, Ugalde JA, Olivares-Pacheco J, Quiroz V, Brito B, Rivas LM, Munita JM, García PC and Wozniak A (2024) Ceftazidime/avibactam resistance is associated with PER-3-producing ST309 lineage in Chilean clinical isolates of non-carbapenemase producing Pseudomonas aeruginosa. Front. Cell. Infect. Microbiol. 14:1410834. doi: 10.3389/fcimb.2024.1410834
Received: 01 April 2024; Accepted: 13 May 2024;
Published: 05 June 2024.
Edited by:
Rima El-Herte, Creighton University, United StatesReviewed by:
Vittoria Mattioni Marchetti, University of Pavia, ItalyChristopher Mark Longshaw, Shionogi Europe, United Kingdom
Copyright © 2024 Soto, Alcalde-Rico, Ugalde, Olivares-Pacheco, Quiroz, Brito, Rivas, Munita, García and Wozniak. This is an open-access article distributed under the terms of the Creative Commons Attribution License (CC BY). The use, distribution or reproduction in other forums is permitted, provided the original author(s) and the copyright owner(s) are credited and that the original publication in this journal is cited, in accordance with accepted academic practice. No use, distribution or reproduction is permitted which does not comply with these terms.
*Correspondence: Aniela Wozniak, YW5pZWxhLndvem5pYWtAZ21haWwuY29t; YXdvem5pYWtAdWMuY2w=
†These authors have contributed equally to this work