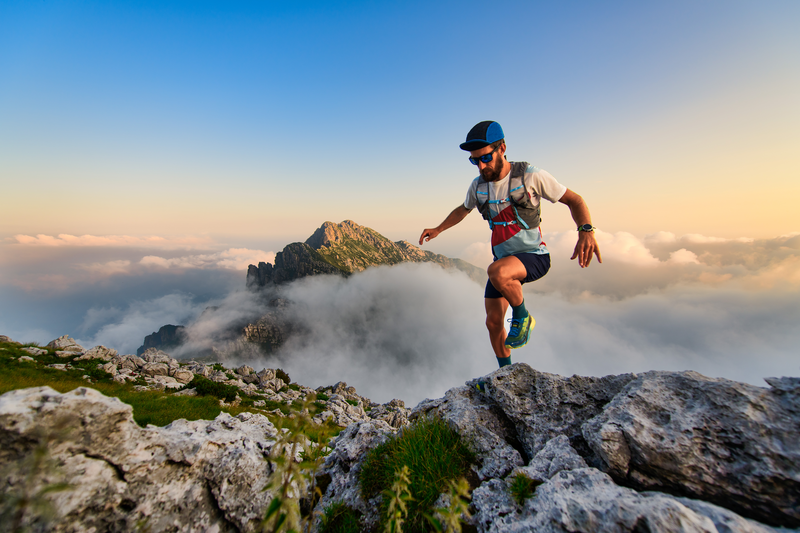
95% of researchers rate our articles as excellent or good
Learn more about the work of our research integrity team to safeguard the quality of each article we publish.
Find out more
ORIGINAL RESEARCH article
Front. Cell. Infect. Microbiol. , 13 June 2024
Sec. Bacteria and Host
Volume 14 - 2024 | https://doi.org/10.3389/fcimb.2024.1408362
This article is part of the Research Topic Interactions between Insects, Gut Flora, and Pathogens: Insights from Vector Insects View all 5 articles
The Asian citrus psyllid (ACP) Diaphorina citri Kuwayama is the leading vector of Candidatus Liberibacter asiaticus (CLas), the causative agent of citrus Huanglongbing (HLB) disease. The distribution and dynamics of CLas within ACP are critical to understanding how the transmission, spread and infection of CLas occurs within its host vector in nature. In this study, the distribution and titer changes of CLas in various tissues of ACP 5th instar nymphs and adults were examined by fluorescence in situ hybridization (FISH) and real-time quantitative PCR (qPCR) techniques. Results demonstrated that 100% of ACP 5th instar nymphs and adults were infected with CLas following feeding on infected plants, and that CLas had widespread distribution in most of the tissues of ACP. The titers of CLas within the midgut, salivary glands and hemolymph tissues were the highest in both 5th instar nymphs and adults. When compared with adults, the titers of CLas in these three tissues of 5th instar nymphs were significantly higher, while in the mycetome, ovary and testes they were significantly lower than those of adults. FISH visualization further confirmed these findings. Dynamic analysis of CLas demonstrated that it was present across all the developmental ages of ACP adults. There was a discernible upward trend in the presence of CLas with advancing age in most tissues of ACP adults, including the midgut, hemolymph, salivary glands, foot, head, cuticula and muscle. Our findings have significant implications for the comprehensive understanding of the transmission, dissemination and infestation of CLas, which is of much importance for developing novel strategies to halt the spread of CLas, and therefore contribute to the efficient prevention and control of HLB.
The Asian citrus psyllid (ACP) Diaphorina citri Kuwayama (Hemiptera: Sternorrhyncha: Psylloidea: Liviidae) is a phloem-feeding insect and major pest of citrus plants (Hall et al., 2013). It is well documented as being the primary insect vector of ‘Candidatus Liberibacter asiaticus’ (CLas), the bacterial pathogen associated with citrus Huanglongbing (HLB) or citrus greening disease, which is the most serious disease of citrus worldwide (Bové, 2006; Carmo-Sousa et al., 2020). To date, HLB has spread to more than 40 countries in Asia, Oceania, North and South America (Bové, 2006; Croxton and Stansly, 2014; Da Graça et al., 2016). Although research on CLas has attracted significant attention, the potential control of CLas itself remains largely unknown currently due to the lack of pure cultures of CLas, therefore, controlling the insect vector is the most critical step for citrus HLB prevention (Nava et al., 2007; Hall et al., 2013). Given that reducing populations of ACP is critical to HLB management, the biology of the vector and mechanisms of CLas acquisition and transmission in ACP hosts must be fully understood.
CLas is acquired by ACP nymphs or adults during feeding on the phloem of infected citrus plants and the resulting ingestion of CLas into the ACP’s midgut. This is followed by its translocation and probable multiplication into various tissues including the hemolymph and salivary glands, where it is then inoculated into a new plant during feeding (Hall et al., 2013). The ACP nymphs are more efficient at acquiring CLas than adults and can transmit HLB efficiently when acquiring CLas in the nymphal stage, whereas they are inefficient at transmitting HLB when acquiring CLas in the adult stage (Ammar et al., 2011b; Carmo-Sousa et al., 2020). Therefore, it is proposed that CLas mainly uses ACP nymphs to acquire pathogens, and ACP adults to inoculate and transmit pathogens (Ammar et al., 2020). When acquired by ACP nymphs, CLas is transmitted in a persistent propagative manner with it even accompanying the psyllid throughout its entire life cycle (Inoue et al., 2009; Pelz-Stelinski et al., 2010). During the circulative journey, CLas successfully overcomes multiple physiological barriers, including the midgut-infection barrier, dissemination barrier, and salivary-escape barrier. Using quantitative polymerase chain reaction (qPCR), electron microscopy scanning, and fluorescence in situ hybridization (FISH), it has been determined that CLas is detectable in various ACP tissues including the filter chamber, midgut, hemolymph, salivary glands, ovary, and muscles (Ammar et al., 2011a, b). Hosseinzadeh et al. (2019b) quantified CLas titer in multiple tissues of ACP and found that CLas were detected in various insect tissues. More recently, several studies have used qPCR to describe CLas accumulation in hemolymph and salivary glands, suggesting propagation of CLas within insect tissues (Pelz-Stelinski et al., 2010; Ammar et al., 2016; Wu et al., 2018). However, previous studies have primarily focused on the early-stage detection of CLas within ACP; limited information is available regarding the distribution and titers of CLas among various tissues of an ACP nymph and adult during its development, and indeed the multiplication of CLas within various ACP tissues remains poorly understood (Pelz-Stelinski et al., 2010; Ammar et al., 2011a, b, 2016; Hosseinzadeh et al., 2019b; Nian et al., 2023).
Over a prolonged period of co-evolution, an intricate and mutually advantageous symbiotic relationship has evolved among vector insects, pathogenic microorganisms, and receptor plants (Hogenhout et al., 2008); the plant-CLas-ACP association system in our current study is also included. Understanding the titers and distribution of pathogens within insects is crucial for elucidating the underlying mechanisms through which pathogenic microorganisms impact the physiological functions of their hosts (Hogenhout et al., 2008; Carmo-Sousa et al., 2020).
It is known that CLas multiplies in the host bodies after it acquisition by ACP 5th instar nymph,. however, after the acquisition of CLas by ACP adult, the persistence of CLas was found to be limited and even eliminated, suggesting that only those ACP adults that were infected CLas during their 5th instar nymph stage have high efficiency to transmit CLas. The exact mode of infection in different tissues is still unclear to date, to address the knowledge gaps identified above, the presence and distribution of CLas in various tissues of an ACP 5th instar nymph and adult were systematically detected through qPCR and FISH. The dynamics of CLas in different tissues of an ACP adult was also investigated. The results are expected to aid in further understanding the phenomenon of propagation of CLas within the body of an ACP, providing theoretical support for understanding the underlying mechanism behind CLas transmission by these insects.
The colony of ACP was continuously reared at the Guangdong Laboratory for Lingnan Modern Agriculture, Guangzhou, China. The CLas-uninfected ACP colony were reared on healthy, CLas negative orange jasmine (Murraya exotica L.) plants, while the CLas positive ACP colony was obtained by inoculating CLas-uninfected ACP to a CLas-infected tree of Citrus reticulata “Shatangju” at Fruit Research Institute of Zhaoqing University (Guangdong, China) for 3 weeks before experiments. Experimental plants were tested periodically as CLas-negative or CLas-positive by PCR, with nested-PCR also used to ensure the CLas infection rate within the ACP as outlined by Guo et al. (2021).
The 5th instar ACP nymphs were reared on CLas-infected Shatangju plants in plant growth chambers (Jiangnan Instrument Co. Ltd., RXZ-380A) at Guangdong Laboratory for Lingnan Modern Agriculture at 26 ± 1°C, RH 80 ± 10% with L:D = 14:10 photoperiods. Following emergence (which was marked as 1 day after eclosion), the CLas-infected adults were collected and reared on the CLas-uninfected Shatangju plants. Five different developmental stages (1 d, 5 d, 10 d, 15 d and 20 d old) of adults were prepared.
The above collected ACP 5th instar nymphs and adults of different ages were dissected, and various tissues were collected. The hemolymph was collected with a 10 µL pipette tip using the method of Killiny et al (Killiny et al., 2017). For other tissues including midgut, salivary gland, mycetome, ovary, testes, foot, head, cuticula and muscle, dissections were performed in 1×phosphate buffer saline (with 75% RNAfollow All, Yuanye, Shanghai, China, R40036) on ice and collected within a maximum of one hour. After the dissection process, the obtained samples were subsequently divided into two distinct portions to facilitate the conjoint analysis. One portion was utilized for FISH in order to detect the distribution of CLas, while the other portion was allocated for qPCR to determine the relative titers of CLas. Three biological replications were conducted, wherein each replication consisted of 30–40 individuals for qPCR analysis; 5 tissue samples including midgut, salivary glands, testis, and ovary of ACP 5th instar nymphs and adults were imaged in FISH detection. Five replications for each tissue type were repeated to verify the repeatability.
DNA extraction of ACP: Each isolated tissue, including midgut, salivary glands, head, muscle, cuticula, foot, mycetome, ovary and testes, was washed twice in 1×PBS and each time was 5 minutes, afterward, the tissues and collected hemolymph were transferred to 50µL GA buffer lysate using a dissecting needle. The DNA extraction of the different ACP tissues were followed the instructions of the TIANamp Micro DNA Kit (Tiangen, Beijing, China, DP316).
FISH detection of CLas: In order to gain further insights into the distribution of CLas within the main tissues, FISH determination was used to visualize the presence of CLas within the anatomical structures of the midgut, salivary gland, ovary and testis of the ACP 5th instar nymphs and adults following the protocol of Guo et al. (2023). After a series of fixation, washing, decolorization and hybridization, the nuclei of dissected tissues were stained with DAPI (4’, 6-diamidino-2-phenylindole; 0.1 mg/mL; ThermoFisher Scientific, Waltham, MA, USA, 62248) for 10 min and then rinsed with PBST for 10 min. The stained samples were then observed and photographed under a Nikon Eclipse Ti-U inverted microscope (Nikon Instruments Inc., Tokyo, Japan). The CLas probe 5’-Cy3-GCCTCGCGACTTCGCAACCCAT-3’ (targeting the 16S rDNA gene of CLas) was labeled at the 5’-terminal with fluorescence labeling of Cy3.
The titer of CLas in different tissues of ACP, including midgut, salivary glands, hemolymph, mycetome, ovary, head, foot, cuticula, muscle and fat body, at different developmental times (1d, 5d, 10d, 15d and 20d) were detected using qPCR. The CLas primers (targeting CLas prophage genes) used for qPCR were F: 5’-GCCGTTTTAACACAAAAGATGAATATC-3’, R: 5’-ATAAATCAATTTGTTCTAGTTTACGAC-3’ (Morgan et al., 2012), and the β-Actin gene of ACP was chosen as a reference gene for the purpose of normalizing and quantifying the data: whose primers were F: 5’-CCCTGGACTTTGAACAGGAAA-3’, R: 5’-CTCGTGGATAC CG CAAGATT-3’ (Tiwari et al., 2012). The reaction system of qPCR was as follows: 5 µL SYBR Premix Ex Taq (Takara, Dalian, China, RR820A), 2 µL DNA template, 0.5 µL (10 µmol/L) forward/reverse primer and 2 µL ddH2O, at a total reaction volume of 10 µL. The reactions were conducted using a Bio-Rad CFX Co-nnect™ thermocycler (Bio-Rad Laboratories Inc., Hercules, CA, USA) according to the following protocol: initial denaturation at 95°C for 3 min, followed by 40 cycles at 95°C for 10 s, 60°C for 20 s and 72°C for 30 s. The relative titers of CLas (relative expression of CLas) was calculated using the 2-ΔΔCt method (Livak and Schmittgen, 2001).
The CLas in the saliva secreted by the different stages (1 d, 5 d, 10 d, 15 d and 20 d) of CLas-infected ACP adults was detected by nested PCR. Thirty CLas-infected ACP adults were collected and fed with artificial diet (containing 15% sucrose solution, 0.1% green food dyes and 0.4% yellow food dyes) using the Parafilm double-film method (Galdeano et al., 2017). The ACP adults without CLas were used as a parallel control. Following 3 days of feeding, the genomic DNA was extracted from the artificial diet using a micro-scale genomic DNA extraction kit (Tiangen, Beijing, China, DP316) for CLas detection, following the instructions provided with the kit for “Extraction of Genomic DNA from Mouthwash”. The template DNA was amplified using a rolling circle amplification (RCA) kit (Haigene, Haerbin, China, A3702a) to increase its concentration, and then nested PCR was used to detect CLas within the saliva.
The primer sequences for nested PCR (targeting the β-operon gene of CLas) were P1, P2, P3 and P4 (P1: 5’-TCTGTTTTCTTCGAGGTTGGTGAG-3’, P2: 5’-ACCGCAAGACTCCTTACCAG GAAG-3’, P3: 5’-GCGTTCATGTAGAAGTTGTG-3’, P4: 5’-CTTACAGGTGGCTGACTCAT-3’). The first round of PCR reaction included 12 μL Taq enzyme mix (Mei5bio, Beijing, China, MF002-plus-10), 10 μL ddH2O, 1 μL each of P1 and P2 primers and 1 μL DNA template. The PCR protocol consisted of an initial denaturation step at 94°C for 5 minutes, followed by 20 cycles of denaturation at 94°C for 30 seconds, annealing at 50°C for 30 seconds, and extension at 72°C for 1 minute, with a final extension step at 72°C for 5 minutes. The second round of PCR reaction was comprised of 12 μL Taq enzyme mix, 9 μL ddH2O, 1 μL each of the P3 and P4 primers (10 μmol/L) and 2 μL of DNA template (i.e., the first-round PCR product). The PCR program included an initial denaturation step at 94°C for 5 min, followed by 35 cycles of denaturation at 94°C for 30s, annealing at 50°C for 30s, and extension at 72°C for 30s. A final extension step at 72°C lasting five minutes concluded the PCR process.
Relative titer of CLas in different tissues of ACP were calculated using the method of 2-ΔΔCt. ACP β-Actin was used as the internal control for normalization. The column and error bars represent the fold change (titer) in Mean ± SE. Different letters indicate significant differences in CLas gene expression among different treatments (P <0.05).
In order to comprehensively understand the copy number of CLas within an infected ACP, we took into account the scenario where the CtActin value exceeded the CtCLas value by employing the formula-POWER (2, (CtActin-CtCLas)) to calculate the relative number of CLas within individual cells of various tissues, where CtCLas is the cycle threshold of the gene of CLas and CtActin is the cycle threshold value of the housekeeping gene (β-actin). If the CLas content within a single cell surpasses the copy number of the reference Actin gene, this means the CLas content within a single cell > 1.0.
All fluorescent images were prepared for final presentation using Adobe Photoshop CS5. It is imperative to assess the normality and homogeneity of variance for all the data prior to utilization. The data analyses were conducted utilizing SPSS 18.0 software (SPSS Inc., Chicago, IL, USA). Statistical analysis was performed via Student’s t-test or One-Way Analysis of Variance (ANOVA). Graphs were drawn using GraphPad Prism 6.0 and Microsoft Excel software.
To assess the acquisition rate of CLas by ACP, CLas-free ACP adults were introduced to oviposit on the CLas-infected citrus plants. After the progeny developed through to 5th instar nymphs and teneral adults, the infection rate of CLas was detected. Results demonstrated that 100% of 5th instar nymphs and adults were infected with CLas when they developed on CLas-infected citrus plants (Table 1); indicating a high ability of ACP nymphs to acquire CLas.
The relative titers of CLas within CLas-infected 5th instar nymphs and adults were investigated by qPCR. The results demonstrated that CLas infested all the tissues, including midgut, salivary gland, hemolymph, head, muscle, cuticula, foot, mycetome, ovary and testes. In addition, midgut, salivary glands and hemolymph were the tissues with the highest titers of CLas; the titer of CLas in the midgut was 4.1 times higher than that in the salivary glands, and 7.6 times higher than in the hemolymph (Figure 1).
Figure 1 Relative titer of CLas in different tissues of ACP 5th instar nymphs. ACP β-Actin was used as the internal control for normalization. The relative titers were calculated using the method of 2-ΔΔCt. The relative titer of CLas in other tissues is relative to the titer in the head. The column and error bars represent the fold change (titer) in Mean ± SE. Different letters indicate significant differences in CLas gene expression among different treatments (P <0.05).
Similarly, CLas was detected in all tissues of teneral adults. Among these tissues, the midgut and salivary glands exhibited higher levels of CLas, with the midgut displaying the highest concentration, surpassing that of the salivary glands by a factor of 7.6. Furthermore, the concentration of CLas in the midgut was 18.5 times higher than that in the mycetome, and 21.1 times higher than that in the hemolymph (Figure 2). Conversely, lower titers of CLas were detected in the head, muscle, cuticula, foot, ovary and testes.
Figure 2 Relative titer of CLas in different tissues of ACP teneral adults. ACP β-Actin was used as the internal control for normalization. The relative titers were calculated using the method of 2-ΔΔCt. The relative titer of CLas in other tissues is relative to the titer in the muscle. The column and error bars represent the fold change (titer) in Mean ± SE. Different letters indicate significant differences in CLas gene expression among different treatments (P <0.05).
To comprehend the alterations of CLas titers in different tissues of 5th instar nymphs and adults, the titers of CLas in the midgut, salivary glands, hemolymph, mycetome, ovary and testes were detected using qPCR. Results revealed a significant disparity in CLas titers between the 5th instar nymphs and adults, for example, the CLas titers in the midgut, salivary glands and hemolymph of 5th instar nymphs were significantly higher than those in adults, while the mycetome, ovary, and testes of 5th instar nymphs exhibited significantly lower CLas titers compared to that of teneral adults (Figure 3), which suggests that during the development from nymph to adult, the content of CLas in different tissues undergoes different trends of change.
Figure 3 Relative titer of CLas in different tissues of ACP 5th instar nymphs and teneral adults. (A) midgut; (B) hemolymph; (C) salivary gland; (D) mycetome; (E) ovary; (F) testes. ACP β-Actin was used as the internal control for normalization. The relative titers were calculated using the method of 2-ΔΔCt. The relative titer of CLas in adults is relative to the titer in nymphs. The column and error bars represent the fold change (titer) in Mean ± SE. Different letters indicate significant differences in CLas gene expression among different treatments P <0.05 (independent-sample t-test).
Based on the aforementioned result, it is evident that the midgut and salivary glands of the ACP serve as the primary sites of CLas infection, there by acting as significant barriers to the transmission of CLas, additionally, the ovaries and testis also were infected with CLas in moderate quantities. The FISH assay results demonstrated that the fluorescence signals of CLas in the midgut were more pronounced in 5th instar nymphs compared to teneral adults (Figures 4A, B), which was consistent with the CLas titer results of qPCR analysis (Figure 3A), which indicated a higher CLas titer in the filter chamber. CLas fluorescence signals were both found in the salivary glands of 5th instar nymphs and teneral adults. CLas was exclusively localized within particular cells of the salivary glands of nymphs and adults. Additionally, the salivary glands of 5th instar nymphs exhibited stronger CLas fluorescence signals compared to those of teneral adults (Figures 4C, D).
Figure 4 Localization of CLas in different tissues of 5th instar nymphs and adults of ACP. (A) midgut of 5th instar nymph; (B) midgut of adult; (C) salivary gland of 5th instar nymph; (D) salivary glands of adult; (E) testes of 5th instar nymph; (F) testes of the adult;(G) ovary of 5th instar nymph; (H) ovary of adult. Nuclear was stained with DAPI (blue); CLas was stained with CLas-Cy3 probe (red).
The fluorescence signals of CLas in the testes of 5th instar nymphs and teneral adults exhibited diminished intensity and a dispersed pattern (Figures 4E, F). The fluorescence signals of CLas in the ovaries of 5th instar nymphs and adults were also relatively weak, with only a few discrete fluorescence signals observed (Figures 4G, H). However, in the ovaries of teneral adults, it was evident that CLas primarily localized around the oocyte, while only a limited number of CLas infiltrated the interior (Figure 4H). In contrast, the fluorescent signal of CLas was not detected in the CLas-negative control group (Figure S1).
The relative titers of CLas in various tissues of infected ACP spanning a period of 1 to 20 days from emergence is shown in Figure 5. Results demonstrated that CLas was presented across all the developmental ages of ACP adults tested, and that its relative titers varied among different tissues. In general, there was a discernible upward trend in the presence of CLas with advancing age in most tissues of ACP adults, including midgut, hemolymph, salivary glands, foot, head, cuticula and muscle, which suggests that CLas has the ability to propagate and reproduce within these specific anatomical structures (Figures 5A-C, G-J). In mycetome, ovary and testes, the CLas titers showed a trend of decreasing-increasing-f-decreasing (Figures 5D–F), but the CLas titers in mycetome and testes on days 15 and 20 were significantly higher than those of days (1, 5 and 10) (Figures 5D, F), while the CLas titers in an adult ovary on day (5, 10, 15 and 20) were significantly lower than that of day 1 (Figure 5E).
Figure 5 Dynamics of CLas multiplication in eleven tissues of adult ACP. (A) midgut; (B) hemolymph; (C) salivary gland; (D) mycetome; (E) ovary; (F) testes; (G) foot; (H) head; (I) cuticula; (J) muscle; (K) fat body. ACP β-Actin was used as the internal control for normalization. The relative titers were calculated using the method of 2-ΔΔCt. The column and error bars represent the fold change (titer) in Mean ± SE. Different letters indicate significant differences in CLas gene expression among different treatments (P <0.05).
The gel results demonstrated that CLas was detected in the saliva of CLas- infected ACP at various developmental stages (Figure 6). In addition, the PCR bands exhibited a progressive enhancement as the age of the ACP increased, thereby suggesting a corresponding increase in the transmission capacity of CLas by the ACP with advancing age.
Figure 6 CLas detection in the saliva of citrus psyllid. CLas in ACP saliva was detected using nested PCR. M: DNA marker; Lane 1: saliva of CLas-free ACP, Lanes 2–6: saliva of ACP adults on day 1, 5, 10, 15, 20.
The relative copy of CLas and Actin genes in the cells of different tissues of ACP are compared in Figure 7. Results showed that the values of CLas in the salivary glands (days 5–20), midgut (days 10–20) and hemolymph (days 10–20) were all > 1.0, which indicated relative higher amounts of CLas in the individual cells of these three tissues. Conversely, the remaining tissues (ovary, testes, mycetome, foot, head, cuticula, muscle and fat body) displayed a relatively lower amount of CLas in single cells, consistent with our other results. These observations further support the notion that the salivary glands, midgut and hemolymph serve as the primary sites for CLas proliferation and replication.
Figure 7 Relative copies of CLas in cells of each tissue compared to ACP β-Actin gene. The formula POWER (2, (CtActin- CtCLas)) was used to calculate the relative number of CLas in a single cell of each tissue. When the value is greater than 1, it indicates that the tissue has more than 1 unit CLas within a single cell. Red arrowheads: The value of relative copies of CLas is greater than 1.
Citrus Huanglongbing (HLB) is a serious disease of citrus and has caused destructive loss to the citrus industry worldwide for many decades (Bové, 2006; Carmo-Sousa et al., 2020). As the pathogen of HLB, CLas is transmitted by ACP in a persistent and propagative manner, completing its circulate transmission from CLas-positive plants to healthy plants (Ammar et al., 2016). The transmission mechanism consists of multiple phases, which encompass: (a) the ingestion of the pathogen and its migration into the midgut of ACP while feeding on an CLas-infected citrus plant, (b) the transfer of CLas from the midgut into the hemolymph and different tissues, including the salivary glands, with potential pathogen replication in these tissues, and (c) the introduction of the CLas through injection within salivary secretions during subsequent feeding on a healthy plant that is susceptible to infection (Hogenhout et al., 2008; Mercer et al., 2010; Galdeano et al., 2020). Over the past decade, the acquisition of CLas by ACP has been found to be a significant factor in pathogen transmission, and it was influenced by various factors such as temperature, citrus susceptibility, life stage, the sex of the psyllid, season, sampling method, time of acquisition and plant species, resulting in infection rates ranging from 1 to 90% (Inoue et al., 2009; Pelz-Stelinski et al., 2010; Perilla-Henao and Casteel, 2016; Wu et al., 2018). However, our findings revealed a 100% infection rate among ACP. The observed high infection rate could potentially be attributed to the continuous consumption of CLas-infected citrus plants by the ACP population under a laboratory experimental design. This prolonged feeding behavior provided an advantageous circumstance for CLas to effectively infiltrate all tissues, thereby reinforcing the notion that the nymph stage of ACP exhibits a notable proficiency in acquiring CLas (Pelz-Stelinski et al., 2010; Ammar et al., 2016).
Understanding the impact of infection titer and distribution of CLas in ACP is of utmost significance. Previously, CLas has been identified in the salivary glands, alimentary canal, and overall body of ACP adults through the utilization of qPCR (Ammar et al., 2011b). Additional investigations employing FISH have demonstrated the presence of CLas in most tissues of ACP, in addition to those previously mentioned (Ammar et al., 2011a; Coletta et al., 2014; Ammar et al., 2019; Nian et al., 2023). Most of the prior research primarily focused on the detection CLas infection in limited number of tissues of ACP adults, neglecting CLas infection in different tissues of ACP 5th instar nymphs and lacking a thorough and systematic examination of the infection pattern of CLas in various tissues of ACP adults at different instars. In the present study, we conducted a thorough examination of various tissues of ACP 5th instar nymphs and adults to detect CLas for the first time, and it was observed that CLas also exhibited a systemic invasion in all dissected tissues of an ACP 5th instar nymphs and adults, including the midgut, salivary gland, hemolymph, head, muscle, cuticula, foot, mycetome, ovary and testes. Our findings indicated that CLas has the ability to infest various tissues of the ACP in a systematic manner when acquired during the ACP 5th instar nymphs, thus supporting the hypothesis that the ACP 5th instar nymph is proficient in disseminating and acquiring Clas than adult (Ammar et al., 2011b, 2020; Carmo-Sousa et al., 2020). Our study also identified notable variations in the CLas titers attributed to the life stage of ACP, wherein the titer of CLas in the midgut, salivary glands, and hemolymph of ACP 5th instar nymphs was significantly higher compared to that of adults. The significantly higher efficiency of CLas acquisition by ACP nymphs compared to adults, is possibly due to their higher frequency and longer duration of phloem feeding (Inoue et al., 2009; Pelz-Stelinski et al., 2010; Ammar et al., 2016; Mann et al., 2018). However, it is also important to consider that successful acquisition may be influenced by other factors such as disparities in innate immunity between nymphs and adults (Mann et al., 2011; Ghanim et al., 2017; George et al., 2018).
Two tissues, midgut and salivary glands, are recognized as the most important transmission barriers of persistently and propagatively transmitted plant pathogens (Ramsey et al., 2017; Mishra and Ghanim, 2022). During CLas infection, the pathogen must traverse the intestinal barrier or evade immune responses in order to reach the salivary glands for efficient transmission. Ammar et al. observed that the levels of CLas in the midgut and salivary glands of ACP adults were considerably greater compared to those in other tissues (Ammar et al., 2011a, b). In the current study, similar findings were observed in 5th instar nymphs and teneral adults; i.e. significantly greater titers of CLas in the midgut and salivary glands compared to other bodily tissues. Additionally, our study also demonstrated that the salivary glands (days 5–20), midgut (days 10–20) and hemolymph (days 10–20) exhibited a significantly elevated copy number of CLas in individual cells (Figure 7), consistent with the results that the relative copy number of CLas genomes was significantly higher in both midgut and salivary glands compared to the reference genes of ACP genomes in each sample (Ammar et al., 2011b). The elevated concentration of CLas in the hemolymph may increase the likelihood of its infection in the salivary glands.
From another perspective, our results align with previous studies on vector insects being responsible for transmitting plant pathogens and confirmed that the midgut and salivary glands of insects serve as a significant impediment for pathogen transmission (Hogenhout et al., 2008; Wang et al., 2023). A significant indication of the transmission capability of an insect vector related to plant pathogens, is the ability of the pathogen to infiltrate particular cells within the salivary glands of vector insects, and amass in substantial quantities, thereby attaining a sufficient concentration to facilitate transmission of the plant pathogen (Weintraub and Beanland, 2005; Galdeano et al., 2020; Wang et al., 2023).
In this study, the FISH technique was employed to ascertain that CLas was exclusively localized within particular cells of the salivary glands of 5th instar nymphs and adults of ACP. The results, combined with qPCR data, indicate that the salivary glands exhibited a higher accumulation of CLas compared to other tissues (excluding the midgut). By conducting further testing, it was discovered that the titer of CLas within the saliva of the ACP adults gradually increased with age. Consequently, it can be inferred that ACP adults possess the ability to transmit CLas during various developmental stages.
Previous studies have revealed the infrequent infection of CLas in the ovaries of ACP through qPCR (Weintraub and Beanland, 2005; Ammar et al., 2011a, b). Hosseinzadeh et al. further observed occasional existence of CLas in the follicle cells of females (Hosseinzadeh et al., 2019b). In the current study, the presence of CLas was also identified in ovary tissues through the utilization of qPCR and FISH, albeit at a low level. It is worth noting that CLas exhibited predominant localization around the oocyte in the ovaries of ACP adults, with only a minimal infiltration into the oocyte, which may provide a possible explanation that usually extremely low CLas can be maternally transmitted to the next generation (Ghanim et al., 2017; Carmo-Sousa et al., 2020).
The transmission dynamics of CLas in ACP suggest a durable association between the pathogen and vector, suggesting that the bacterium responsible for HLB circulates, infects, and potentially replicates within multiple tissues of the vector (Pelz-Stelinski et al., 2010; Wu et al., 2018; Hosseinzadeh et al., 2019b; Nian et al., 2023), which has received constant attention. For instance, Wu et al. reported that CLas multiplication was detected in the hemolymph and salivary glands of ACP adults after the bacterium was acquired by nymphs (Wu et al., 2018). Nian et al. reported that the relative CLas titer in the midgut and ovaries obviously increased with development (Nian et al., 2023). Based on this premise, we conducted a more detailed detection of the CLas infection pattern in various tissues of ACP at different developmental stages. In the present study, it was found that the relative titer in midgut, hemolymph, salivary glands, foot, head, cuticula, and muscle were of a discernible upward trend with advancing age. This observation implies that CLas possesses the capacity to propagate and reproduce within these particular anatomical structures. Alternatively, the fluctuation in CLas titer observed in the mycetome cells and fat body of ACP may potentially be associated with endosymbiosis and immune responses within the insect (Hosseinzadeh et al., 2019b).
Previous work has found that vitellogenin-like protein (Vg-VWD) in ACP interacts with a CLas flagellum (flaA) protein, which is upregulated in CLas-infected ACP (Hosseinzadeh et al., 2019a). This implies that the presence of an adequate quantity of Vg within the ACP facilitates the binding of CLas. As widely acknowledged, vitellogenin (Vg) is expressed in the fat body in an insect, secreted into the hemolymph, and then taken up by developing oocytes (Hosseinzadeh et al., 2019a). According to our previous study (Tufail et al., 2014), the fecundity peak appeared on approximately day 15. Consequently, it is necessary for the fat body to increase its production of Vg during this specific period. However, the titer of CLas in the ovary exhibited a gradual decline, whereas the CLas titer in the testes initially decreased and subsequently increased after day 15. Whatever the case may be, it is noteworthy that the CLas titer in the ovary and testes were comparatively lower when compared to other tissues (Figure 2), which also provides a possible explanation that extremely low levels of CLas can be maternally transmitted to progeny (Ren et al., 2016; Ghanim et al., 2017). Therefore, regulation of Vg-related immune pathways may serve as a mechanism to modulate the vector competence of ACP for CLas, suggesting a potential role for elevated Vg expression in females as a defense against ovary infection by CLas. Nevertheless, these hypotheses necessitate additional empirical verification.
In conclusion, this study reveals that during the nymph stage, ACP can acquire CLas by feeding on a CLas-infected citrus plant with high efficiency, and then CLas consequently reaches various tissues of ACP in a systematic and comprehensive way, so revealing its ability to replicate or accumulate in the diverse tissues of ACP hosts including midgut, hemolymph, salivary glands, foot, head, cuticula, and muscle. These results aid in comprehensively understanding the different dynamic changes of titers in different tissues of ACP, which is important for developing in-depth research and innovation towards better “CLas transmission blocking” strategies for HLB management.
The original contributions presented in the study are included in the article/Supplementary Material. Further inquiries can be directed to the corresponding author.
The manuscript presents research on animals that do not require ethical approval for their study.
CG: Conceptualization, Formal analysis, Investigation, Methodology, Software, Writing – original draft, Writing – review & editing. WK: Investigation, Methodology, Software, Writing – original draft, Writing – review & editing. MM: Conceptualization, Methodology, Writing – review & editing. YH: Conceptualization, Investigation, Resources, Writing – review & editing. YL: Conceptualization, Resources, Supervision, Writing – review & editing. WS: Conceptualization, Methodology, Supervision, Writing – review & editing. BQ: Funding acquisition, Resources, Supervision, Writing – original draft, Writing – review & editing.
The author(s) declare financial support was received for the research, authorship, and/or publication of this article. This work was supported by the National Joint Fund for Regional Innovation and Development (U22A20481), the Guangdong Laboratory of Lingnan Modern Agriculture Project (NT2021003) to BLQ. CFG acknowledges support from both China Postdoctoral Science Foundation (No.2022M711208) and Basic and Applied Basic Research Fund of Guangdong Province (2022A1515110401). The funders had no role in the study design, data collection and interpretation, or in the decision to submit the work for publication.
The authors thank Dr. Andrew G S Cuthbertson (York, UK) for valuable comments on an earlier version of the manuscript. We also thank the reviewers for their constructive comments and suggestions.
The authors declare that the research was conducted in the absence of any commercial or financial relationships that could be construed as a potential conflict of interest.
The author(s) declared that they were an editorial board member of Frontiers, at the time of submission. This had no impact on the peer review process and the final decision.
All claims expressed in this article are solely those of the authors and do not necessarily represent those of their affiliated organizations, or those of the publisher, the editors and the reviewers. Any product that may be evaluated in this article, or claim that may be made by its manufacturer, is not guaranteed or endorsed by the publisher.
The Supplementary Material for this article can be found online at: https://www.frontiersin.org/articles/10.3389/fcimb.2024.1408362/full#supplementary-material
Supplementary Figure 1 | Negative control for the Localization of CLas in different tissues of 5th instar nymphs and adults of ACP. (A) midgut of 5th instar nymph; (B) midgut of adult; (C) salivary gland of 5th instar nymph; (D) salivary glands of adult; (E) testes of 5th instar nymph; (F) testes of the adult;(G) ovary of 5th instar nymph; (H) ovary of adult. Nuclear was stained with DAPI (blue); CLas was stained with CLas-Cy3 probe (red).
Ammar, E. D., Achor, D., Levy, A. (2019). Immuno-ultrastructural localization and putative multiplication sites of Huanglongbing bacterium in Asian citrus psyllid Diaphorina citri. Insects 10, 422. doi: 10.3390/insects10120422
Ammar, E., George, J., Sturgeon, K., Stelinski, L. L., Shatters, R. G. (2020). Asian citrus psyllid adults inoculate Huanglongbing bacterium more efficiently than nymphs when this bacterium is acquired by early instar nymphs. Sci. Rep. 10, 18244. doi: 10.1038/s41598–020-75249–5
Ammar, E. D., Ramos, J. E., Hall, D. G., Dawson, W. O., Shatters, R. G. (2016). Acquisition, replication and inoculation of Candidatus Liberibacter asiaticus following various acquisition periods on Huanglongbing-Infected citrus by nymphs and adults of the Asian citrus psyllid. PloS One 11, e0159594. doi: 10.1371/journal.pone.0159594
Ammar, E. D., Shatters, R. G., Hall, D. G. (2011b). Localization of Candidatus Liberibacter asiaticus, associated with citrus Huanglongbing disease, in its psyllid vector using fluorescence in situ hybridization. J. Phytopathol. 159, 726–734. doi: 10.1111/j.1439-0434.2011.01836.x
Ammar, E., Shatters, R. G., Lynch, C., Hall, D. G. (2011a). Detection and relative titer of Candidatus Liberibacter asiaticus in the salivary glands and alimentary canal of Diaphorina citri (Hemiptera: Psyllidae) vector of Citrus Huanglongbing disease. Ann. Entomol. Soc Am. 104, 526–533. doi: 10.1603/AN10134
Bové, J. M. (2006). Huanglongbing: a destructive, newly-emerging, century-old disease of citrus. J. Phytopathol. 88, 7–37. doi: 10.4454/jpp.v88i1.828
Carmo-Sousa, M., Cortés, M. T. B., Lopes, J. R. S. (2020). Understanding psyllid transmission of Candidatus Liberibacter as a basis for managing Huanglongbing. Trop. Plant Pathol. 45, 572–585. doi: 10.1007/s40858-020-00386-1
Coletta, H. D., Daugherty, M. P., Ferreira, C., Lopes, J. R. S. (2014). Temporal progression of ‘Candidatus Liberibacter asiaticus’ infection in citrus and acquisition efficiency by Diaphorina citri. Phytopathol. J. 104, 416–421. doi: 10.1094/PHYTO-06–13-0157-R
Croxton, S. D., Stansly, P. A. (2014). Metalized polyethylene mulch to repel Asian citrus psyllid, slow spread of Huanglongbing and improve growth of new citrus plantings. Pest Manage. Sci. 70, 318–323. doi: 10.1002/ps.3566
Da Graça, J. V., Douhan, G. W., Halbert, S. E., Keremane, M. L., Lee, R. F., Vidalakis, G., et al. (2016). Huanglongbing: an overview of a complex pathosystem ravaging the world’s citrus. J. Integr. Plant Biol. 58, 373–387. doi: 10.1111/jipb.12437
Galdeano, D. M., Breton, M. C., Lopes, J. R. S., Falk, B. W., MaChado, M. A. (2017). Oral delivery of double-stranded RNAs induces mortality in nymphs and adults of the Asian citrus psyllid, Diaphorina citri. PloS One 12, e0171847. doi: 10.1371/journal.pone.0171847
Galdeano, D. M., de Souza Pacheco, I., Alves, G. R., Granato, L. M., Rashidi, M., et al. (2020). Friend or foe? Relationship between ‘Candidatus Liberibacter asiaticus’ and Diaphorina citri. Trop. Plant Pathol. 45, 559–571. doi: 10.1007/s40858-020-00375-4
George, J., Ammar, E., Hall, D. G., Shatters, R. G., Lapointe, S. L. (2018). Prolonged phloem ingestion by Diaphorina citri nymphs compared to adults is correlated with increased acquisition of citrus greening pathogen. Sci. Rep. 8, 10352. doi: 10.1038/s41598–018-28442–6
Ghanim, M., Achor, D., Ghosh, S., Kontsedalov, S., Lebedev, G., Levy, A. (2017). ‘Candidatus Liberibacter asiaticus’ accumulates inside endoplasmic reticulum associated vacuoles in the gut cells of Diaphorina citri. Sci. Rep. 7, 16945. doi: 10.1038/s41598–017-16095-w
Guo, C. F., Ahmed, M. Z., Ou, D., Zhang, L. H., Lu, Z. T., Sang, W., et al. (2021). Parasitoid vectors a plant pathogen, potentially diminishing the benefits it confers as a biological control agent. Commun. Biol. 4, 1331. doi: 10.1038/s42003-021-02851-2
Guo, C. F., Qiu, J. H., Hu, Y. W., Xu, P. P., Deng, Y. Q., Tian, L., et al. (2023). Silencing of V-ATPase-E gene causes midgut apoptosis of Diaphorina citri and affects its acquisition of Huanglongbing pathogen. Insect Sci. 30, 1022–1034. doi: 10.1111/1744-7917.13146
Hall, D. G., Richardson, M. L., Ammar, E. D., Halbert, S. E. (2013). Asian citrus psyllid, Diaphorina citri, vector of citrus Huanglongbing disease. Entomol. Exp. Appl. 146, 207–223. doi: 10.1111/eea.12025
Hogenhout, S. A., Ammar, E. D., Whitfield, A. E., Redinbaugh, M. G. (2008). Insect vector interactions with persistently transmitted viruses. Annu. Rev. Phytopathol. 46, 327–359. doi: 10.1146/annurev.phyto.022508.092135
Hosseinzadeh, S., Ramsey, J., Mann, M., Bennett, L., Hunter, W. B., Shams-Bakhsh, M., et al. (2019a). Color morphology of Diaphorina citri influences interactions with its bacterial endosymbionts and ‘Candidatus Liberibacter asiaticus’. PloS One 14, e0216599. doi: 10.1371/journal.pone.0216599
Hosseinzadeh, S., Shams-Bakhsh, M., Mann, M., Fattah-Hosseini, S., Bagheri, A., Mehrabadi, M., et al. (2019b). Distribution and variation of bacterial endosymbiont and “Candidatus Liberibacter asiaticus” titer in the Huanglongbing insect vector, Diaphorina citri Kuwayama. Microb. Ecol. 78, 206–222. doi: 10.1007/s00248-018-1290-1
Inoue, H., Ohnishi, J., Ito, T., Tomimura, K., Miyata, S., Iwanami, T., et al. (2009). Enhanced proliferation and efficient transmission of Candidatus Liberibacter asiaticus by adult Diaphorina citri after acquisition feeding in the nymphal stage. Ann. Appl. Biol. 155, 29–36. doi: 10.1111/j.1744-7348.2009.00317.x
Killiny, N., Hijaz, F., El-Shesheny, I., Alfaress, S., Jones, S. E., Rogers, M. E. (2017). Metabolomic analyses of the hemolymph of the Asian citrus psyllid Diaphorina citri, the vector of Huanglongbing. Physiol. Entomol. 42, 134–145. doi: 10.1111/phen.12183
Livak, K. J., Schmittgen, T. D. (2001). Analysis of relative gene expression data using real-time quantitative PCR and the 2–ΔΔCT method. Methods 25, 402–408. doi: 10.1006/meth.2001.1262
Mann, M., Fattah-Hosseini, S., Ammar, E., Stange, R., Warrick, E., Sturgeon, K., et al. (2018). Diaphorina citri nymphs are resistant to morphological changes induced by “Candidatus Liberibacter asiaticus” in midgut epithelial cells. Infect. Immun. 4, 86. doi: 10.1128/IAI.00889-17
Mann, R. S., Pelz-Stelinski, K., Hermann, S. L., Tiwari, S., Stelinski, L. L. (2011). Sexual transmission of a plant pathogenic bacterium, Candidatus Liberibacter asiaticus, between conspecific insect vectors during mating. PloS One 6, e29197. doi: 10.1371/journal.pone.0029197
Mercer, J., Schelhaas, M., Helenius, A. (2010). Virus entry by endocytosis. Annu. Rev. Biochem. 79, 803–833. doi: 10.1146/annurev-biochem-060208-104626
Mishra, S., Ghanim, M. (2022). Interactions of Liberibacter species with their psyllid vectors: molecular, biological and behavioral mechanisms. Int. J. Mol. Sci. 23, 4029. doi: 10.3390/ijms23074029
Morgan, J. K., Zhou, L. J., Li, W. B., Shatters, R. G., Keremane, M., Duan, Y. P. (2012). Improved real-time PCR detection of ‘Candidatus Liberibacter asiaticus’ from citrus and psyllid hosts by targeting the intragenic tandem-repeats of its prophage genes. Mol. Cell. Probes 26, 90–98. doi: 10.1016/j.mcp.2011.12.001
Nava, D. E., Torres, M. L. G., Rodrigues, M. D. L., Bento, J. M. S., Parra, J. R. P. (2007). Biology of Diaphorina citri (Hem., Psyllidae) on different hosts and at different temperatures. J. Appl. Entomol. 131, 709–715. doi: 10.1111/j.1439-0418.2007.01230.x
Nian, X. G., Wu, S. J., Li, J. Y., Luo, Y. R., He, J. L., Tan, S. J., et al. (2023). Spatial distribution and temporal dynamics of Candidatus Liberibacter asiaticus in different stages of embryos, nymphs and adults of Diaphorina citri. Int. J. Mol. Sci. 24, 8997. doi: 10.3390/ijms24108997
Pelz-Stelinski, K. S., Brlansky, R. H., Ebert, T. A., Rogers, M. E. (2010). Transmission parameters for Candidatus Liberibacter asiaticus by Asian citrus psyllid (Hemiptera: Psyllidae). J. Econ. Entomol. 103, 1531–1541. doi: 10.1603/EC10123
Perilla-Henao, L. M., Casteel, C. L. (2016). Vector-Borne bacterialp plant pathogens: interactions with hemipteran insects and plants. Front. Plant Sci. 7 1163. doi: 10.3389/fpls.2016.01163
Ramsey, J. S., Chavez, J. D., Johnson, R., Hosseinzadeh, S., Mahoney, J. E., Mohr, J. P., et al. (2017). Protein interaction networks at the host-microbe interface in Diaphorina citri, the insect vector of the citrus greening pathogen. R. Soc Open Sci. 4, 160545. doi: 10.1098/rsos.160545
Ren, S. L., Li, Y. H., Zhou, Y. T., Xu, W. M., Cuthbertson, A. G. S., Guo, Y. J., et al. (2016). Effects of Candidatus Liberibacter asiaticus on the fitness of the vector Diaphorina citri. J. Appl. Microbiol. 121, 1718–1726. doi: 10.1111/jam.2016.121.issue-6
Tiwari, S., Clayson, P. J., Kuhns, E. H., Stelinski, L. L. (2012). Effects of buprofezin and diflubenzuron on various developmental stages of Asian citrus psyllid, Diaphorina citri. Pest Manage. Sci. 68, 1405–1412. doi: 10.1002/ps.3323
Tufail, M., Nagaba, Y., Elgendy, A. M., Takeda, M. (2014). Regulation of vitellogenin genes in insects. Entomol. Sci. 17, 269–282. doi: 10.1111/ens.12086
Wang, H., Chen, Q., Wei, T. Y. (2023). Complex interactions among insect viruses-insect vector-arboviruses. Insect Sci. doi: 10.1111/1744-7917.13285
Weintraub, P. G., Beanland, L. (2005). Insect vectors of phytoplasmas. Annu. Rev. Entomol. 51, 91–111. doi: 10.1146/annurev.ento.51.110104.151039
Keywords: Huanglongbing, CLAS, Diaphorina citri, infection, distribution, dynamic
Citation: Guo C-F, Kong W-Z, Mukangango M, Hu Y-W, Liu Y-T, Sang W and Qiu B-L (2024) Distribution and dynamic changes of Huanglongbing pathogen in its insect vector Diaphorina citri. Front. Cell. Infect. Microbiol. 14:1408362. doi: 10.3389/fcimb.2024.1408362
Received: 28 March 2024; Accepted: 28 May 2024;
Published: 13 June 2024.
Edited by:
Songqing Wu, Fujian Agriculture and Forestry University, ChinaReviewed by:
Chinmay V. Tikhe, Johns Hopkins University, United StatesCopyright © 2024 Guo, Kong, Mukangango, Hu, Liu, Sang and Qiu. This is an open-access article distributed under the terms of the Creative Commons Attribution License (CC BY). The use, distribution or reproduction in other forums is permitted, provided the original author(s) and the copyright owner(s) are credited and that the original publication in this journal is cited, in accordance with accepted academic practice. No use, distribution or reproduction is permitted which does not comply with these terms.
*Correspondence: Bao-Li Qiu, YmFvbGlxaXVAY3FudS5lZHUuY24=
†These authors have contributed equally to this work
Disclaimer: All claims expressed in this article are solely those of the authors and do not necessarily represent those of their affiliated organizations, or those of the publisher, the editors and the reviewers. Any product that may be evaluated in this article or claim that may be made by its manufacturer is not guaranteed or endorsed by the publisher.
Research integrity at Frontiers
Learn more about the work of our research integrity team to safeguard the quality of each article we publish.