- 1Department of Urology, The Affiliated Yantai Yuhuangding Hospital of Qingdao University, Yantai, China
- 2Department of Medical Oncology, The Affiliated Yantai Yuhuangding Hospital of Qingdao University, Yantai, China
The microbiota, also referred to as the microbial community, is a crucial component of the human microenvironment. It is located predominantly in various organs, including the intestines, skin, oral cavity, respiratory tract, and reproductive tract. The microbiota maintains a symbiotic relationship with the human body, influencing physiological and pathological functions to a significant degree. There is increasing evidence linking the microbial flora to human cancers. In contrast to the traditional belief that the urethra and urine of normal individuals are sterile, recent advancements in high-throughput sequencing technology and bacterial cultivation methods have led to the discovery of specific microbial communities in the urethras of healthy individuals. Given the prevalence of bladder cancer (BCa) as a common malignancy of the urinary system, researchers have shifted their focus to exploring the connection between disease development and the unique microbial community within tumors. This shift has led to a deeper investigation into the role of microbiota in the onset, progression, metastasis, prognosis, and potential for early detection of BCa. This article reviews the existing research on the microbiota within BCa tumors and summarizes the findings regarding the roles of different microbes in various aspects of this disease.
1 Introduction
Bladder cancer (BCa) ranks among the top ten most common urinary tract malignancies and is the second-most prevalent malignancy of the urinary system. It accounts for 4.6% of all new cancer diagnoses annually, with approximately 400,000 new cases and 160,000 deaths worldwide each year, and has a five-year mortality rate of 30% (Richters et al., 2020). BCa can be divided into muscle-infiltrating bladder cancer (MIBC) and nonmuscle-infiltrating bladder cancer (NMIBC) based on its invasion into the muscular layer of the bladder wall (Lindskrog et al., 2021). Approximately 70% of BCa cases are NMIBC, while the remaining 30% are MIBC, which has a greater potential for invasion and metastasis (Grayson, 2017). The majority of NMIBC patients undergo transurethral resection of the bladder tumor (TURBT), yet the recurrence rates range between 40% and 80%. Additionally, 25% of NMIBC patients progress to MIBC or distant tumor metastases. MIBC, characterized by few early symptoms, rapid progression, and poor prognosis, remains a significant clinical challenge (Antoni et al., 2017).
The incidence of BCa correlates with age, peaking in individuals aged 75-84 years, who represent 30% of new cases annually (Richters et al., 2020). The disease is 3.7 times more common in men than in women (Sung et al., 2021; Yacouba et al., 2022), a disparity attributed to greater exposure to smoking and chemical carcinogens in men, as well as hormonal differences between the sexes (Madeb and Messing, 2004; Yacouba et al., 2022). Despite an increase in smoking among women, the incidence of BCa in this demographic population remains comparatively low, suggesting additional contributing factors beyond established risk factors (Marcon et al., 2018; Yacouba et al., 2022).
Genetic mutations and alterations in specific pathways have been implicated in BCa. Tumor suppressor genes such as TP53, RB1, and PTEN are frequently mutated in carcinoma in situ (CIS) (Castillo-Martin et al., 2010). Oncogenes such as FGFR3, PIK3CA, and RAS, which promote tumor cell development, are characteristic of NMIBC (Castillo-Martin et al., 2010; Knowles and Hurst, 2015). Diet, particularly meat consumption, has been reviewed for its role in bladder carcinogenesis due to the formation of carcinogenic chemicals during meat cooking and processing (Aveta et al., 2022).
Contrary to previous beliefs that healthy bladders are sterile (Han et al., 2014), advancements in urine collection techniques have revealed a distinct bladder microbiota in healthy individuals (Wolfe et al., 2012; Horwitz et al., 2015; Whiteside et al., 2015a; Thomas-White et al., 2016; Thomas-White et al., 2018). Early research by Hicks RM et al. linked bacteria to schistosomiasis-induced BCa through N-nitrosamine formation (Mansour et al., 2020; Chen et al., 2022). BučevićPopović et al. demonstrated microbial infiltration in 20% of malignant tumor tissues, suggesting bacterial involvement in BCa (Buˇcevi´c Popovi´c et al., 2018). With improvements in detection methods, researchers are increasingly identifying microorganisms in BCa tissues, and exploring their sequencing, functions, and mechanisms. An increasing number of studies indicate that the intratumoral microbiota plays a crucial role in the onset and progression of BCa (Figure 1). This article concentrates on various microbial phyla and reviews the diverse roles played by the intratumoral microbiota in BCa.
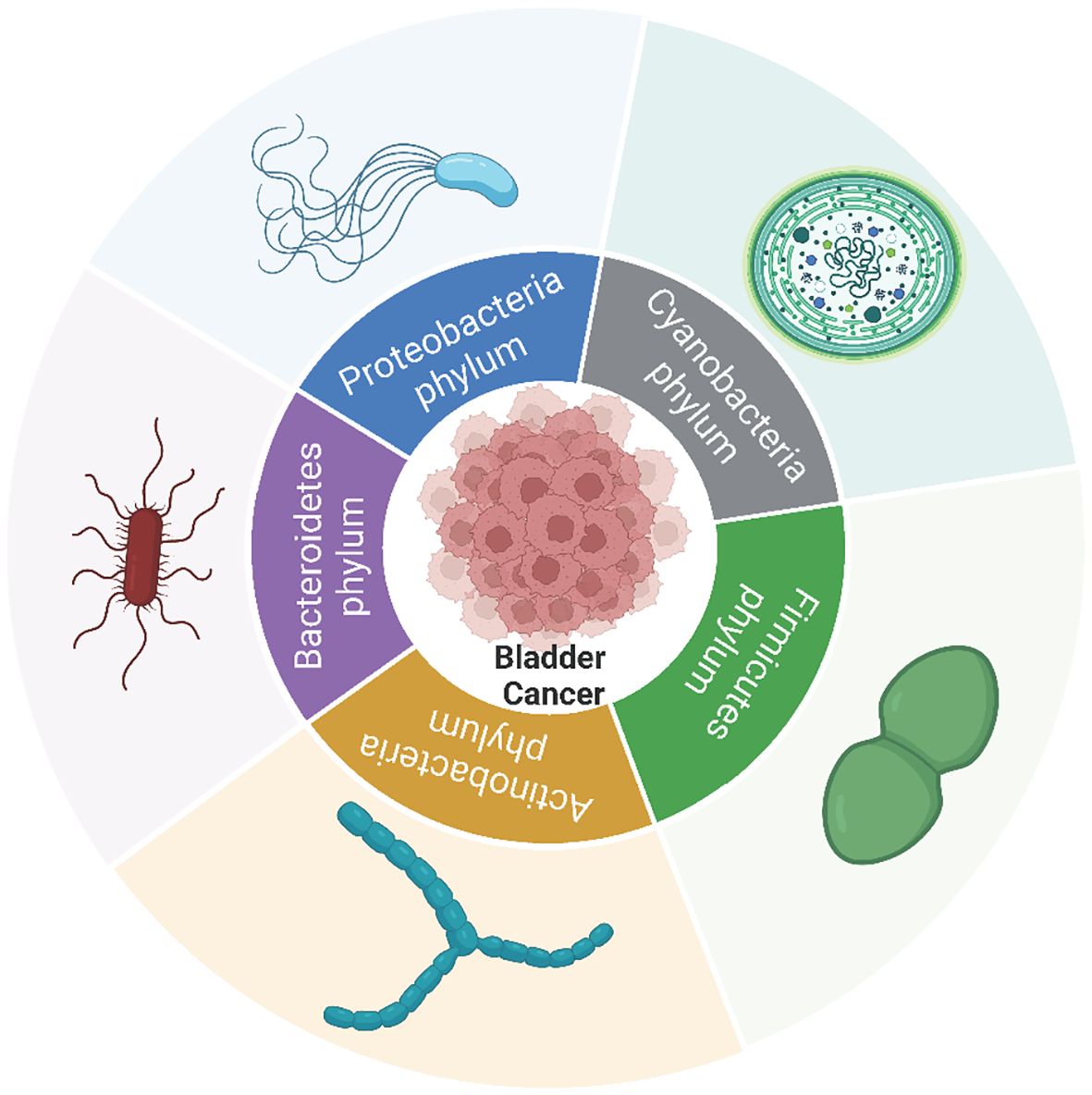
Figure 1 The microbial phyla linked to the onset, progression, metastasis and prognosis of bladder cancer within bladder cancer tumors. Among these, the Proteobacteria, Firmicutes, Bacteroidetes, and Actinobacteria phyla exhibit the highest abundance in bladder cancer as well as adjacent or normal tissues in the majority of studies.
2 Materials and methods
A comprehensive literature search was performed using PubMed, Embase and the China National Knowledge Infrastructure (CNKI) to October 2023. The search terms included ‘bladder cancer tissue’, ‘microbiota’ and ‘microbiome’ (the ‘microbiota’ is defined as the totality of microorganisms living in a specific environment, and the term ‘microbiome’ refers to the totality of microbial genes in a specific biological niche. These terms are often used interchangeably in the literature (Berg et al., 2020). The following criteria were used to include studies in this review: (1) included BCa and control groups (case-control studies), (2) provided information on the presence or abundance of microbial taxa, and (3) provided information on the promotion or inhibition of microorganisms in the BCa and/or control groups. A total of 10 relevant articles were screened, including 8 clinical trial papers and 2 bioinformatics analyses (Table 1).
3 Phylum Proteobacteria
Using data from the TCGA database, Rebecca M. Rodriguez et al. analyzed microbial diversity and species differences in 56 paired tumor and adjacent normal tissue samples from 28 patients. They discovered that Proteobacteria was the most prevalent phylum, comprising 93% of the total reads, with Stenotrophomonas maltophilia being the most common species (accounting for 61% of the total reads) (Rodriguez et al., 2020). Wei Li conducted genomic sequencing of bacterial species in cancer and adjacent tissues from 18 BCa patients without urinary tract infections and with negative urine cultures. This study corroborated the existence of microbial flora in bladder tissue, revealing that the most abundant bacteria in both cancer and adjacent bladder tissues belonged to the phylum Proteobacteria (Li, 2021). Jian-Xuan Sun’s team employed 2bRAD-M microbiome sequencing technology to analyze tumor tissue samples from 22 BCa patients, focusing on the differences in the microbial community between NMIBC and MIBC. Echoing the findings of previous studies, they noted similar microbial compositions in both tumor types, with Ralstonia_sp000620465 being the predominant species (Sun et al., 2023).
Proteobacteria are currently the largest phylum within the domain Bacteria. A common trait of Proteobacteria is the gram-negative staining and, thus, the presence of lipopolysaccharide in the outer membrane. Many common human pathogens are found in the Proteobacteria phylum, such as Brucella genera, Rickettsia genera, Neisseria, Escherichia, Shigella, Salmonella and Helicobacter genera (Human Microbiome Project Consortium, 2012). Proteobacteria, which are typically gut commensals with pathogenic potential, have been studied extensively (Everard et al., 2011; Geurts et al., 2011; Zhang et al., 2012). Recent research highlighted significant variations in the abundance of Proteobacteria in mucosa-associated microbiomes of ileal and rectal biopsies (but not in stool samples) between Crohn’s disease patients and control subjects (Gevers et al., 2014). Similar shifts in gut proteobacterial communities have been observed in patients with colitis-associated colorectal cancer (Bonnet et al., 2014). These findings collectively suggest that an imbalance in Proteobacteria could play a crucial role in bladder carcinogenesis. The increase in the abundance of Proteobacteria might serve as a potential diagnostic indicator for dysbiosis, potentially increasing the risk of BCa.
3.1 Cupriavidus in BCa
Fei Liu et al. examined tissue samples of cancerous bladder mucosa from patients diagnosed with BCa, consisting of 22 carcinoma tissues and 12 adjacent normal tissues. This study confirmed the occurrence of bladder microbiota dysbiosis in BCa patients. The researchers observed that Proteobacteria was the predominant phylum in both cancerous and noncancerous tissues, with a significantly greater abundance in cancerous tissues. In cancerous tissues, the presence of Cupriavidus, Acinetobacter, and Escherichia‐Shigella increases markedly (Wang et al., 2007). Previous research has indicated that certain trivalent pesticide-related chemicals can induce protein carbonylation and oxidative DNA damage in human urothelial cells, potentially leading to BCa (Liu et al., 2019). Interestingly, the enrichment of harmful chemical products, which are subject to metabolic processes, might correlate with the significantly elevated abundance of the genus Cupriavidus. This genus was the most abundant bacteria found in both cancerous and noncancerous bladder tissues, despite notable differences in their levels. Cupriavidus, known from prior studies as an organophosphorus pesticide-degrading microorganism, is thought to break down harmful substances absorbed by the body, subsequently excreting them into the bladder through specific enzymes (Wang et al., 2007).
3.2 Biofilms in BCa development
Naomi Nadler et al. collected tissue samples from ten patients undergoing TURBT and analyzed them for bacterial aggregates using Fluorescence in situ Hybridization (FISH). Dense biofilms were identified in the urothelial cancer tissue of two samples, and spherical bacteria were confirmed in one sample. Notably, both patients had negative preoperative urine cultures. These findings suggest a potential link between biofilm formation and BCa (Nadler et al., 2021).
Bacterial aggregates, commonly referred to as biofilms, often attach to the apical epithelial cells of the bladder and are known as umbrella cells. These cells typically have a protective layer of sulfated polysaccharide aminoglycans. Disruption of this layer may lead to pathological bladder changes and chronic inflammation in the genitourinary system (Garrett, 2015). During biofilm formation, bacteria such as Aeromonas hydrophila and Pseudomonas aeruginosa can augment the production of extracellular proteases, including serine proteases, metalloproteinases, and elastases (Swift et al., 1999). Some pathogens, such as Streptococcus pyogenes, may cause invasive diseases by degrading intercellular junctions in conjunction with the host cysteine protease calpain (Sumitomo et al., 2013).
Studies on bacterial biofilms in colorectal cancer have shown that certain bacteria, such as Escherichia coli, can drive cancer development. Escherichia coli produces the genotoxin colibactin, which causes mutagenic DNA damage through covalent DNA binding to a chemical moiety known to promote tumorigenesis (Garrett, 2015). Further research is required to understand this relationship, particularly whether bacterial dysbiosis and biofilm formation in the bladder exhibit oncogenic features similar to those in colorectal cancer. Establishing a causal link could make microbiome modulation a significant therapeutic area.
3.3 Microbial associations with the epithelial-mesenchymal transition in BCa development
Wei Tse Li et al. identified a range of microbes, including E. coli, the butyrate-producing bacterium SM4/1, and a species of Oscillatoria, that were correlated with the expression of classic EMT-associated genes, such as E-cadherin, vimentin, SNAI2, SNAI3, and TWIST1. Notably, the Escherichia coli str. K-12 substr. showed the most significant correlations with EMT-related gene expression. They also observed significant correlations between microbial abundance and the expression of extracellular matrix (ECM) genes, particularly those related to collagens and elastin. The presence of the Escherichia coli O157:H7 strain exhibited a significant correlation with the expression of ECM proteins (Li et al., 2021).
EMT is a key process in several cancers that enhances metastatic potential by increasing cell mobility and decreasing cell–cell adhesion (Franzen et al., 2015; Ogawa et al., 2020). During EMT, epithelial cells transform into mesenchymal cells, enabling detachment from the basement membrane and invasion into adjacent tissues. These mesenchymal cells can migrate to distant sites and revert back to epithelial cells through mesenchymal–epithelial transition, initiating metastasis. In MIBC, the importance of EMT is highlighted by the upregulation of mesenchymal cell markers, such as N-cadherin and P-cadherin, and the downregulation of epithelial cell markers, such as E-cadherin (Yun and Kim, 2013; Franzen et al., 2015). ECM proteins play a vital role in cancer cell invasion and metastasis, with proteins such as collagens, laminins, and fibronectins associated with survival in urothelial bladder cancer (Brunner and Tzankov, 2007). During metastasis and invasion, ECM proteins degrade and integrins rearrange, facilitating EMT (Brunner and Tzankov, 2007; Jiang et al., 2017). Microbes, known for releasing proteases including collagenases, are thought to influence ECM protein turnover, although this has not yet been confirmed in BCa studies (Alfano et al., 2016).
Interactions between tumor cells and the extracellular microenvironment are critical in cancer development and progression. The interplay between ECM components and bacterial products regulates tissue homeostasis, dysregulation of these processes may create protumorigenic niches, potentially contributing to disease relapse. Understanding the relationship between Escherichia coli and the extracellular microenvironment offers valuable insights into the pathogenesis and progression of BCa.
3.4 Role of Burkholderia in BCa pathobiology
Filippo Pederzoli and his team conducted a study on paired samples from 21 male and 8 female patients, including urine, neoplastic (Npl), and non-neoplastic (non-Npl) tissues. Their findings revealed a greater abundance of the genus Burkholderia in tumor tissues than in non-tumor tissues, regardless of the patient’s gender (Pederzoli et al., 2020). In a parallel study, Bassel Mansour’s research group corroborated these results. They investigated microbiome differences in urine and tissue samples from BCa patients and noted a greater abundance of Burkholderia in cancerous tissues (Mansour et al., 2020). This observation implies a potential role for this genus in the pathobiology of BCa, similar to recent findings in colorectal cancer versus healthy colon mucosa (Alomair et al., 2018). However, the role of this taxon appears to extend beyond merely acting as a trigger for neoplasia. Recent studies have suggested Burkholderiales as a potential “anticancer probiotic.” For instance, in an animal sarcoma model, it was demonstrated that the efficacy of immunotherapy with CTLA-4 antibody was influenced by the composition of the microbiota, particularly by Bacteroides fragilis, Bacteroides thetaiotaomicron, and Burkholderiales (Vétizou et al., 2015). Additionally, the transplantation of these bacteria into antibiotic-conditioned animals showed a protective effect against colitis induced by CTLA-4 blockade.
4 Phylum Cyanobacteria
Cyanobacteria, also known as blue-green algae, are an ancient, highly diverse group of photoautotrophic organisms, that evolve a wide variety of morphologies reaching from unicellular to filamentous organization and thereby represent one of the most diverse prokaryotic phyla. In the past, they were frequently grouped under algae; however, Cyanobacteria exhibited significant distinctions from eukaryotic organisms. Devoid nuclear membranes and organelles and, with their genetic material, DNA that is not organized into chromosomes are characteristic features of bacteria. Consequently, they are now classified within the domain Bacteria (Schirrmeister et al., 2011). Cyanobacteria are considered the inventors of oxygenic photosynthesis, they employ photosynthetic pigments such as carotenoids, phycobilins, and various forms of chlorophyll to capture light energy (Sagan, 1967). Apart from performing oxygenic photosynthesis, many cyanobacteria are able to fix atmospheric nitrogen, adding to their importance in natural ecosystems (Welsh et al., 2008). Cyanobacteria serve as important model organisms with potential biotechnological applications. They are utilized in bioethanol production, edible pigments, human and animal food, nutritional supplements, and raw materials (Tan, 2007; Demay et al., 2019). Cyanobacteria are also known to produce various toxins, termed cyanotoxins, which may pose risks to humans and animals (van Apeldoorn et al., 2007).
4.1 Oscillatoria and EMT-associated gene expression
Wei Tse Li et al. discovered a strong negative correlation between the presence of Oscillatoria, a Cyanobacteria member, and the expression of EMT-promoting genes (Li et al., 2021). Although Cyanobacteria are less characterized in humans, their presence has been detected in the gut (Almeida et al., 2019). Importantly, Oscillatoria species produce butylated hydroxytoluene, a natural antioxidant, which might explain its association with reduced EMT (Babu and Wu, 2008). This connection is significant as oxidative stress and reactive oxygen species are known to regulate ECM proteins and the EMT process (Jiang et al., 2017).
4.2 Cyanobacteria in BCa: microbiota composition and human β-defensins response
Bassel Mansour et al. compared the microbiota composition in cancerous tissues and urine samples from the same patient set. They noted a surprisingly high presence of Cyanobacteria—7% and 8% in urine and tissue samples, respectively—a finding previously undocumented (Mansour et al., 2020).
Mansour, Ádám Monyók, and their team subsequently investigated the tissue microbiome in BCa patients compared to that in benign prostatic hyperplasia patients and healthy volunteers. The authors focused on the mRNA levels of hBDs in tissue and the levels of defensin in urine. Oxyphotobacteria, a Cyanobacterium genus linked to tumor formation (Miao et al., 2016), exhibited a significantly greater abundance in the BCa group, especially in patients with low urinary hBD-1 levels (Mansour et al., 2022).
Defensins, notably hBDs, are critical antimicrobial peptides that play a role in tumor cell dissolution, immune cell attraction, and interactions with complement factors (Adyns et al., 2023). Human β-Defensin-1 (hBD-1) can modify HER2 signal transduction and has been shown to suppress BCa growth (Sun et al., 2019). The ability of hBD-1 to attract cells expressing C-C chemokine receptor 6 (CCR6) suggests its potential for recruiting immune cells, such as Th cells, Tregs, imDCs, and neutrophils (Yang et al., 1999; Biragyn et al., 2001; Wu et al., 2003). The dual antitumor and antibacterial activities of hBD-1, along with the influence of bacterial presence on the production of hBD-2 and hBD-3 (Adyns et al., 2023), highlight the significance of understanding the role of hBDs in antitumoral immune responses and their potential impact on immunotherapy effectiveness.
5 Phylum Firmicutes
Generally, the phylum Firmicutes is recognized for comprising low G + C gram-positive microorganisms characterized by rigid or semi-rigid cell walls containing peptidoglycan. The cells of Firmicutes microorganisms typically take the form of rods or spheres and primarily reproduce through binary fission. Some members can form endospores and exhibit motility facilitated by flagella (Seong et al., 2018). Firmicutes are abundant in soil and aquatic environments and play a crucial role in the decomposition and recycling of organic matter (Baik et al., 2008). Nevertheless, certain genera within this phylum constitute normal flora in the mammalian intestine or act as pathogens for humans, animals, and plants (Lee et al., 2009; Nguyen and Götz, 2016). Additionally, some members of the Firmicutes phylum hold industrial significance for their role in the production of antibiotics, enzymes, and dairy products (Kwak et al., [[NoYear]]; Liu et al., 2012).
5.1 Lactic acid bacteria (LAB) in BCa
LAB, a group of gram-positive bacteria within the phylum Firmicutes, are primarily categorized into the genera Lactobacillus, Streptococcus and Lactococcus (Sharma et al., 2020).
Fei Liu’s research indicated a notable difference in the abundance of Lactobacillus between cancerous and noncancerous tissues, with a greater prevalence in noncancerous tissues (Wang et al., 2007). Lactobacillus, a well-studied probiotic, is known for its health-promoting mechanisms, such as colonizing resistance, acid production, and pathogen exclusion. It has also been linked to cancer, as cell-free supernatants from Lactobacillus can reduce the invasion ability of metastatic tumor cells in vitro (Siddiqui et al., 2012; Pearce et al., 2014). The predominant strain Lactobacillus in women’s bladders and its potential protective role against urothelial bladder cancer have not been determined, although there is evidence suggesting its role in reducing chronic inflammation and enhancing immune responses (Kato et al., 1984; Kato et al., 1988; Takagi et al., 2001; Matsumoto et al., 2009; Shida and Nomoto, 2013).
5.2 Staphylococcus and its relationship with BCa
Wei Li’s study sequenced and analyzed bacterial genomes from cancer and adjacent tissues of 18 BCa patients with no urinary tract infection and negative urine culture. The research has revealed the presence of bacteria in various bladder layers, predominantly in the outer layer of the mucosa. Linear discriminant analysis Effect Size (LEfSe) revealed significant differences in microbial communities, highlighting Staphylococcus at the genus level as significantly more abundant in BCa tissues. This finding aligns with multiple cases of Staphylococcus in the urine cultures of BCa patients (Li, 2021). Similarly, Bassel Mansour’s team noted a greater abundance of Staphylococcus in tumor tissues, correlating with elevated levels of hBD-2 and hBD-3 in urine (Mansour et al., 2022). The inducible production of antibacterial HBD2 and HBD3 is affected by bacteria. Elevated levels of HBD2 were shown to cause treatment failure in anticancer immunotherapy (Adyns et al., 2023). Staphylococcus, particularly saprophytic Staphylococcus, is commonly associated with urinary tract infections. Its role in chronic infections and potential link to the development of BCa warrant further investigation. The impact of saprophytic Staphylococcus on bladder epithelial and cancer cells could be a valuable direction for future research.
6 Phylum Actinobacteria
In terms of the number and variety of identified species, the phylum Actinobacteria represents one of the largest taxonomic units among the 18 major lineages currently recognized within the domain Bacteria (Stackebrandt et al., 1997). Actinobacteria display a wide variety of morphologies, ranging from coccoid or rod-coccoid to fragmenting hyphal forms or permanent and highly differentiated branched mycelia. They also exhibit diverse physiological and metabolic properties, including the production of extracellular enzymes and the formation of a wide variety of secondary metabolites. Many of these secondary metabolites are potent antibiotics (Ventura et al., 2007).
6.1 Actinobacteria: diverse roles in BCa dynamics
Since 1976, BCG (Mycobacterium bovis, Bacillus Calmette–Guérin) has been used as one of the most successful antitumor immunotherapies, particularly for the treatment of BCa (Morales et al., 2017). This specific strain from the Actinobacteria phylum and Mycobacterium genus not only activates the immune system against BCa but also directly induces tumor cell apoptosis (Yu et al., 2015), necrosis (See et al., 2009), or oxidative stress (Shah et al., 2014). The lower incidence of BCa in women might be partially explained by the significantly greater presence of Actinomycetes, including the Mycobacterium genus, in the female urinary microbiome (Lewis et al., 2013).
Mónica Parra-Grande et al. observed a significantly greater abundance of Actinobacteria in nonneoplastic bladder mucosa than in tumor tissues (P = 0.014). Although not statistically significant at the genus level, Propionibacterium was more abundant in the non-tumor mucosa (P = 0.08) (Parra-Grande et al., 2022). Propionibacterium freudenreichii, notable for its probiotic potential and commercial relevance, has been suggested to be a protective agent against colorectal cancer. Research by Casanova et al. indicated that this bacterium could be useful as a probiotic for early-stage colorectal cancer prevention (Casanova et al., 2018). This finding supports the hypothesis that a microbiota rich in Actinomycetes might be linked to the lower incidence of BCa in women (Raoult, 2017), suggesting that a preventive effect similar to that of the BCG vaccine (composed of Actinomycetes), known for its protective role in BCa treatment and relapse prevention (Whiteside et al., 2015b).
Conversely, the research team led by Bassel Mansour and Ádám Monyók reported a greater abundance of another Actinobacteria phylum member, the Corynebacterium genus, in tumor specimens than in non-tumor specimens. This increased presence correlated with elevated levels of hBD-2 and hBD-3 in the urine (Mansour et al., 2022), suggesting a possible role of Corynebacterium in BCa pathogenesis.
6.2 Health-promoting bacterium: Bifidobacterium in BCa
The Bifidobacterium genus is a strictly anaerobic, gram-positive, pleomorphic rod-shaped bacterium that belongs to the Actinobacteria phylum. Owing to their morphological and physiological similarities with Lactobacillus, they were historically classified as members of the Lactobacillus genus for a significant portion of the 20th century. Only recently have they been acknowledged as a distinct genus separate from Lactobacillus (Turroni et al., 2011). Many bifidobacteria are used as active ingredients in a variety of so-called functional foods due to their perceived health-promoting or probiotic properties, such as protection against pathogens mediated through the process of competitive exclusion, bile salt hydrolase activity, immune modulation, and the ability to adhere to mucus or the intestinal epithelium (Liévin et al., 2000; Ouwehand et al., 2002; Stanton et al., 2005).
Mónica Parra-Grande et al. reported an increased abundance of Bifidobacterium in NMIBC tissues (Whiteside et al., 2015b). Bifidobacterium plays a crucial role in microbial homeostasis and anti-inflammatory responses in the mucosa (Di Giacinto et al., 2005; Turroni et al., 2011), and can inhibit IL-6 secretion (Trikha et al., 2003), indicating its possible influence on tumorigenesis. Vyara Matson’s study revealed a link between enriched Bifidobacterium and improved immunotherapy response in melanoma patients (Morales et al., 2017). Parra-Grande’s findings suggest that a lower abundance of Bifidobacterium in MIBC than in NMIBC could indicate bladder mucosal damage and a protective factor against BCa (Parra-Grande et al., 2022).
7 Phylum Bacteroidetes
The phylum Bacteroidetes consists of more than 7000 different gram-negative bacteria, primarily falling within the genera Bacteroides, Prevotella, Parabacteroides, and Porphyromonas (Wexler, 2007). Bacteroidetes, particularly species within the Bacteroides and Prevotella genera, are major degraders of complex carbohydrates, and possess a variety of polysaccharide and glycoside hydrolases capable of breaking down polysaccharides. They play a crucial role in the breakdown of dietary fiber and starch, release energy, and may act as a primary source of propionate (Tuson et al., 2018). Bacteroidetes are actively involved in immunomodulation, with certain members contributing to the suppression of inflammatory activities, while others may promote inflammation, and some are recognized as opportunistic pathogens. Furthermore, the phylum Bacteroidetes plays a role in the regulation of metabolic syndrome and the gut-brain-axis, with intriguing therapeutic implications for mood impairment and neurologic disorders (Gibiino et al., 2018).
7.1 Implications of Prevotella_9 for cancer research
In their study, Fei Liu et al. reported that Prevotella_9 was more prevalent in non-cancerous tissues (Wang et al., 2007). Prevotella, an integral part of the gut microbiome, plays a crucial role in the digestion of fiber and complex carbohydrates. Its abundance is closely linked to dietary habits, particularly when it is high in fiber. Members of the Prevotella genus have been implicated in various cancers, including colorectal, oral, and stomach cancers (Feng et al., 2023). For instance, an increase in Prevotella has been noted in the gut microbiome of colorectal cancer patients (Bonnet et al., 2014). Some Prevotella species may contribute to cancer development by affecting the tumor microenvironment, for example, through modulating local immune responses or producing metabolic byproducts. There is also evidence suggesting that Prevotella may impact the host immune system, potentially playing a role in tumor immune evasion (Chen et al., 2022). While the general research on Prevotella highlights its potential significance in cancer development, additional studies are needed to elucidate the specific role and mechanisms of Prevotella_9 in cancer. The greater abundance of Prevotella_9 in non-cancerous tissues, as found by Fei Liu, might indicate distinct characteristics and roles of this subtype within the Prevotella genus.
8 Others
8.1 Diversity and discrepancies in BCa microbiota studies
Several studies included in this summary have conducted comparative analyses of BCa tissues, adjacent normal tissues, or non-tumor tissue samples. Bassel Mansour’s team highlighted significant differences in microbial composition between tumor and non-tumor tissue samples (Mansour et al., 2022). Fei Liu’s team found that, compared with noncancerous tissues, cancerous tissues had lower species richness and diversity, with notable differences in beta-diversity (Wang et al., 2007). Similarly, Mónica Parra-Grande’s team observed greater microbial diversity in non-tumor bladder mucosa than in tumor tissues, aligning with global indicators of microbiome diversity and richness (Parra-Grande et al., 2022). However, Rebecca M. Rodriguez’s team reported no significant statistical differences in total reads, relative abundance, or positivity ratio between paired tumor and adjacent normal samples (Rodriguez et al., 2020), a finding echoed by Wei Li et al (Li, 2021).
Regarding microbiota variations in BCa tissues of different stages and grades, Mónica Parra-Grande’s team noted significant differences in microbial composition among different tumor grades (Parra-Grande et al., 2022). Jian-Xuan Sun’s team reported similar microbial compositions in MIBC and NMIBC tumor tissues, but with notably greater microbial diversity in NMIBC tissues (Sun et al., 2023). These findings suggest a potential link between the microbiome and tumor biology. Conversely, Bassel Mansour et al. observed no differences in beta-diversity across different tumor grades and stages or in relation to diabetes and hypertension (Mansour et al., 2022). Similarly, Rebecca M. Rodriguez et al. reported no significant differences when paired tumor and normal tissues were stratified according to sex, race, anatomical site, or tumor stage (Rodriguez et al., 2020).
In the context of sex differences, Bassel Mansour et al.’s studies indicated significant diversity variations between male and female tissue samples (Mansour et al., 2020; Mansour et al., 2022), whereas Filippo Pederzoli et al.’s research showed no differences in alpha- and beta-diversity based on sex (Pederzoli et al., 2020). The study of the BCa tumor microbiota is a burgeoning field, with discrepancies among studies highlighting the need for larger sample sizes and further research.
9 Discussion
By reviewing the current research on the intratumoral microbiota of BCa, we identified potential mechanisms through which microorganisms can impact the onset, progression, and prognosis of this disease. Possible mechanisms that may promote Bca include: Cupriavidus, which may lead to the enrichment of metabolizable harmful chemical products within the bladder, inducing protein carbonylation and oxidative DNA damage in human urothelial cells. Bioflms may augment the production of extracellular proteases, degrade intercellular junctions in conjunction and cause mutagenic DNA damage. Escherichia coli may exhibit a significant correlation with the expression of EMT-related genes and ECM proteins. Burkholderi may play a role contrary to other studies where it acts as an “anticancer probiotic” affecting the immune therapeutic effects of CTLA-4 antibodies. Oxyphotobacteria may influence the levels of hBD-1 in urine, affecting its anticancer effect on the HER2 signaling pathway and its antimicrobial effect on recruiting immune cells. Corynebacterium and Staphylococcus may be associated with the elevated levels of hBD-2 in urine, leading to failure of anticancer immunotherapy. Besides, Staphylococcus may play a potential role in chronic urinary tract infections, increasing the risk of bladder cancer.
Moreover, there are potential anticancer mechanisms, for example, Oscillatoria may negatively regulate the EMT and ECM processes by producing a natural antioxidant. Lactobacillus may reduce chronic inflammation, enhance immune responses and reduce the invasion ability of metastatic tumor cells. Bifdobacterium may play a role in microbial homeostasis, anti-inflammatory responses of the mucosa, and may inhibit IL-6 secretion. Propionibacterium may exert a protective role in the treatment and prevention of bladder cancer recurrence, similar to the effects of the BCG vaccine. Prevotella_9 may play a role contrary to what is observed in other cancers, where it influences the host immune system and participates in immune escape in tumor immunity (Figure 2).
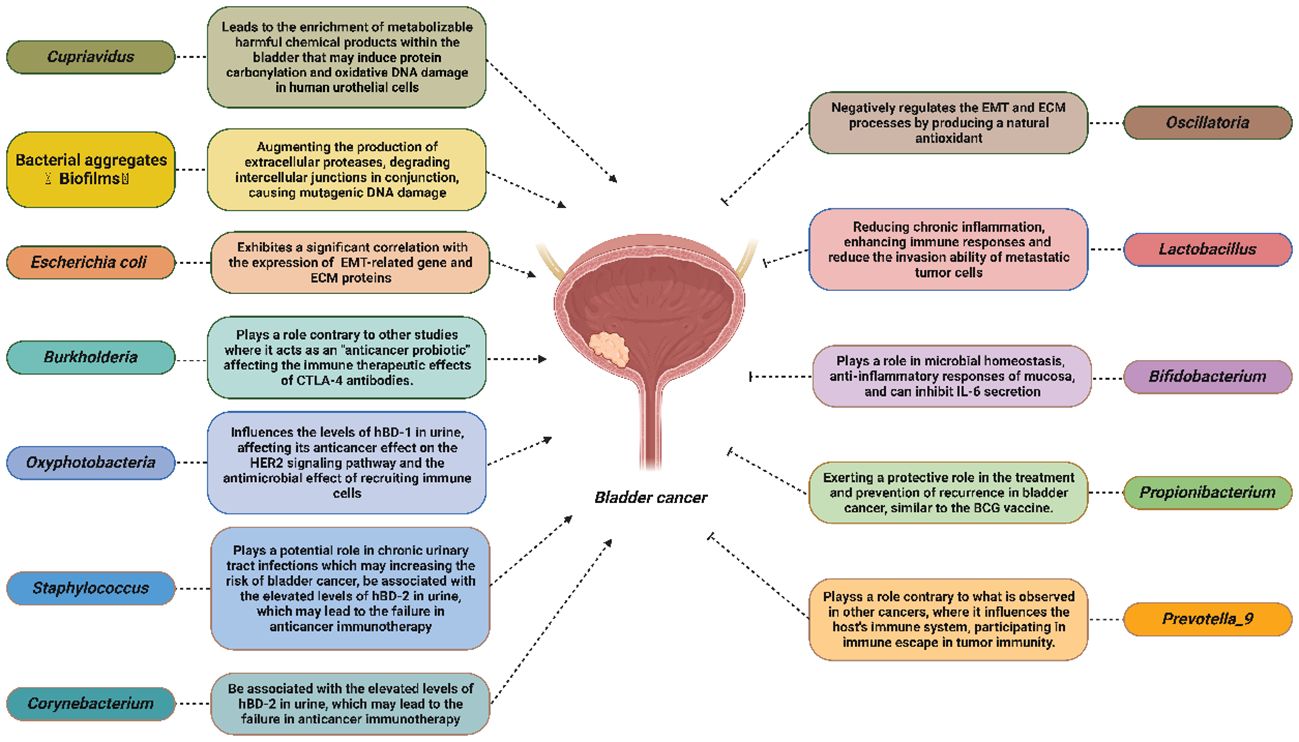
Figure 2 Potential mechanisms of different intratumoral microbiota in the onset, progression, metastasis, prognosis and other aspects of bladder cancer.
Simultaneously, we have contemplated several questions: How do microorganisms enter BCa tumors and in what form? What role does the intratumoral microbiota play in antitumor immunity? Does it have a positive, negative, or surveillance effect, and to what extent is it an instigator, collaborator, or passive observer? Is the intratumoral microbiota detected in BCa a result of purposeful selection by the human body? Does the intratumoral microbiota influence the neural innervation of BCa tumors? What is the extent of the association between the intratumoral microbiota and the recurrence and prognosis of BCa? What kinds of interactions occur among different intratumoral microbiota groups within the tumor? These unresolved questions may lead to new research ideas and directions in the future.
Furthermore, we have identified several limitations that impede research progress. First, ethical considerations prevent the use of healthy samples as controls, thereby hindering comprehensive comparisons. Second, many studies often feature small sample sizes, and lack strong representativeness of the broader population. Third, ensuring complete sample contamination-free processes, such as collection, transportation, storage, and detection, poses a significant challenge, as contamination can potentially impact experimental results. Fourth, different surgical procedures may introduce intergroup differences. Fifth, the small volume of tumor tissue, low bacterial biomass within the tumor, and uncultivable characteristics of some microbial species increase the difficulty of detection. Moreover, tumor samples exhibit a very high host-to-bacterial DNA ratio, potentially causing bias in amplicon-based sequencing results. Additionally, the intrinsic limitations of amplicon-based microbiome methods and incomplete reference databases decrease the extrapolation reliability of these methods. Patient-specific factors such as age, race, sex, breastfeeding status, diet, socioeconomic status, epidemiological factors, genetics, exposure to environmental carcinogens, or disease-specific factors such as pathological TNM stage and focality remain uncontrollable variables. Finally, potential bias in samples introduced by previous BCa diagnostic procedures (e.g., cystoscopy) or prior antibiotic therapies (>4 weeks) cannot be excluded, although the impact of these procedures on the bladder and urinary microbiome is currently unclear.
There are still significant gaps in clinical and laboratory research on the intratumoral microbiota of BCa, and there are several contradictory conclusions among different studies. Addressing these challenges and undertaking innovative or in-depth explorations in this field remains a long journey for researchers. In the future, delving into the molecular mechanisms of the microbiota’s role in BCa tumors, identifying new treatment targets and biomarkers, and exploring how to manipulate the BCa intratumoral bacterial community for cancer patient treatment are crucial. This finding suggests that the biological contribution of the microbiota to BCa is likely to occupy an increasingly prominent position in future BCa research.
10 Conclusions
In conclusion, this article reviews the existing research on the microbiota within BCa tumors, summarizes the findings regarding the roles of different microbes in various aspects of this disease and reflects on the current challenges and future research directions in this field. It is hoped that this study will provide effective assistance for a better understanding of BCa and offer some ideas for further innovative development of the field of intratumoral microbiota in BCa.
Author contributions
KL: Data curation, Formal analysis, Software, Writing – original draft. JC: Methodology, Software, Writing – original draft. JW: Investigation, Methodology, Writing – original draft. JM: Project administration, Resources, Writing – original draft. SL: Supervision, Validation, Writing – original draft. YC: Conceptualization, Data curation, Formal analysis, Funding acquisition, Writing – review & editing.
Funding
The author(s) declare financial support was received for the research, authorship, and/or publication of this article. This work was supported by Joint Fund of Shandong Natural Science Foundation (ZR2021LSW019), the Taishan Scholars Program of Shandong Province (No. tsqn202306403).
Conflict of interest
The authors declare that the research was conducted in the absence of any commercial or financial relationships that could be construed as a potential conflict of interest.
Publisher’s note
All claims expressed in this article are solely those of the authors and do not necessarily represent those of their affiliated organizations, or those of the publisher, the editors and the reviewers. Any product that may be evaluated in this article, or claim that may be made by its manufacturer, is not guaranteed or endorsed by the publisher.
References
Adyns, L., Proost, P., Struyf, S. (2023). Role of defensins in tumor biology. Int. J. Mol. Sci. 24, 5268. doi: 10.3390/ijms24065268
Alfano, M., Canducci, F., Nebuloni, M., Clementi, M., Montorsi, F., Salonia, A. (2016). The interplay of extracellular matrix and microbiome in urothelial bladder cancer. Nat. Rev. Urol. 13, 77–90. doi: 10.1038/nrurol.2015.292
Almeida, A., Mitchell, A. L., Boland, M., Forster, S. C., Gloor, G. B., Tarkowska, A., et al. (2019). A new genomic blueprint of the human gut microbiota. Nature 568, 499–504. doi: 10.1038/s41586-019-0965-1
Alomair, A. O., Masoodi, I., Alyamani, E. J., Allehibi, A. A., Qutub, A. N., Alsayari, K. K., et al. (2018). Colonic mucosal microbiota in colorectal cancer: a single-center metagenomic study in Saudi Arabia. Gastroenterol. Res. Pract. 2018, 5284754. doi: 10.1155/2018/5284754
Antoni, S., Ferlay, J., Soerjomataram, I., Znaor, A., Jemal, A., Bray, F. (2017). Bladder cancer incidence and mortality: A global overview and recent trends. Eur. Urol. 71, 96–108. doi: 10.1016/j.eururo.2016.06.010
Aveta, A., Cacciapuoti, C., Barone, B., Di Zazzo, E., Del Giudice, F., Maggi, M., et al. (2022). The impact of meat intake on bladder cancer incidence: is it really a relevant risk? Cancers (Basel) 14, 4775. doi: 10.3390/cancers14194775
Babu, B., Wu, J. T. (2008). Production of natural butylated hydroxytoluene as an antioxidant by freshwater phytoplankton (1). J. Phycol. 44, 1447–1454. doi: 10.1111/j.1529-8817.2008.00596.x
Baik, K. S., Park, S. C., Kim, E. M., Bae, K. S., Ahn, J. H., Ka, J. O., et al. (2008). Diversity of bacterial community in freshwater of Woopo wetland. J. Microbiol. 46, 647–655. doi: 10.1007/s12275-008-0135-x
Berg, G., Rybakova, D., Fischer, D., Cernava, T., Vergès, M. C. C., Charles, T., et al. (2020). Correction to: Microbiome definition re-visited: old concepts and new challenges. Microbiome 8, 119. doi: 10.1186/s40168-020-00905-x
Biragyn, A., Surenhu, M., Yang, D., Ruffini, P. A., Haines, B. A., Klyushnenkova, E., et al. (2001). Mediators of innate immunity that target immature, but not mature, dendritic cells induce antitumor immunity when genetically fused with nonimmunogenic tumor antigens. J. Immunol. 167, 6644–6653. doi: 10.4049/jimmunol.167.11.6644
Bonnet, M., Buc, E., Sauvanet, P., Darcha, C., Dubois, D., Pereira, B., et al. (2014). Colonization of the human gut by E. coli and colorectal cancer risk. Clin. Cancer Res. 20, 859–867. doi: 10.1158/1078-0432.CCR-13-1343
Brunner, A., Tzankov, A. (2007). The role of structural extracellular matrix proteins in urothelial bladder cancer (review). biomark. Insights 2, 418–427. doi: 10.4137/BMI.S294
Buˇcevi´c Popovi´c, V., Šitum, M., Chow, C. T., Chan, L. S., Roje, B., Terzi´c, J. (2018). The urinary microbiome associated with bladder cancer. Sci. Rep. 8, 12157. doi: 10.1038/s41598-018-29054-w
Casanova, M. R., Azevedo-Silva, J., Rodrigues, L. R., Preto, A. (2018). Colorectal cancer cells increase the production of short chain fatty acids by Propionibacterium freudenreichii impacting on cancer cells survival. Front. Nutr. 5, 44. doi: 10.3389/fnut.2018.00044
Castillo-Martin, M., Domingo-Domenech, J., Karni-Schmidt, O., Matos, T., Cordon-Cardo, C. (2010). Molecular pathways of urothelial development and bladder tumorigenesis. Urol Oncol. 28, 401–408. doi: 10.1016/j.urolonc.2009.04.019
Chen, C., Huang, Z., Huang, P., Li, K., Zeng, J., Wen, Y., et al. (2022). Urogenital microbiota:Potentially important determinant of PD-L1 expression in male patients with non-muscle invasive bladder cancer. BMC Microbiol. 22, 7. doi: 10.1186/s12866-021-02407-8
Chen, Y., Liu, Y., Wang, Y., Chen, X., Wang, C., Chen, X., et al. (2022). Prevotellaceae produces butyrate to alleviate PD-1/PD-L1 inhibitor-related cardiotoxicity via PPARα-CYP4X1 axis in colonic macrophages. J. Exp. Clin. Cancer Res. 41, 1. doi: 10.1186/s13046-021-02201-4
Demay, J., Bernard, C., Reinhardt, A., Marie, B. (2019). Natural products from cyanobacteria: focus on beneficial activities. Mar. Drugs 17, 320. doi: 10.3390/md17060320
Di Giacinto, C., Marinaro, M., Sanchez, M., Strober, W., Boirivant, M. (2005). Probiotics ameliorate recurrent Th1-mediated murine colitis by inducing il-10 and il10-Dependent tgf-Beta-Bearing regulatory cells. J. Immunol. 174, 3237–3246. doi: 10.4049/jimmunol.174.6.3237
Everard, A., Lazarevic, V., Derrien, M., Girard, M., Muccioli, G. G., Neyrinck, A. M., et al. (2011). Responses of gut microbiota and glucose and lipid metabolism to prebiotics in genetic obese and diet-induced leptin-resistant mice. Diabetes 60, 2775–2786. doi: 10.2337/db11-0227
Feng, K., Ren, F., Wang, X. (2023). Association between oral microbiome and seven types of cancers in East Asian population: a two-sample Mendelian randomization analysis. Front. Mol. Biosci. 10, 1327893. doi: 10.3389/fmolb.2023.1327893
Franzen, C. A., Blackwell, R. H., Todorovic, V., Greco, K. A., Foreman, K. E., Flanigan, R. C., et al. (2015). Urothelial cells undergo epithelial-to-mesenchymal transition after exposure to muscle invasive bladder cancer exosomes. Oncogenesis 4, e163. doi: 10.1038/oncsis.2015.21
Geurts, L., Lazarevic, V., Derrien, M., Everard, A, Van Roye, M., Knauf, C., et al. (2011). Altered gut microbiota and endocannabinoid system tone in obese and diabetic leptin-resistant mice: impact on apelin regulation in adipose tissue. Front. Microbiol. 2, 149. doi: 10.3389/fmicb.2011.00149
Gevers, D., Kugathasan, S., Denson, L., Vázquez-Baeza, Y., Van Treuren, W., Ren, B., et al. (2014). The treatment-naive microbiome in new-onset Crohn's disease. Cell Host Microbe 15, 382–392. doi: 10.1016/j.chom.2014.02.005
Gibiino, G., Lopetuso, R. L., Scaldaferri, F., Rizzatti, G., Binda, C., Gasbarrini, A. (2018). Exploring Bacteroidetes: Metabolic key points and immunological tricks of our gut commensals. Dig Liver Dis. 50, 635–639. doi: 10.1016/j.dld.2018.03.016
Han, Q., Wang, R., Sun, C., Jin, X., Liu, D., Zhao, X., et al. (2014). Human beta-defensin-1 suppresses tumor migration and invasion and is an independent predictor for survival of oral squamous cell carcinoma patients. PloS One 9, e91867. doi: 10.1371/journal.pone.0091867
Horwitz, D., McCue, T., Mapes, A. C., Ajami, N. J., Petrosino, J. F., Ramig, R. F., et al. (2015). Decreased microbiota diversity associated with urinary tract infection in a trial of bacterial interference. J. Infect. 71, 358–367. doi: 10.1016/j.jinf.2015.05.014
Human Microbiome Project Consortium(2012). Structure, function and diversity of the healthy human microbiome. Nature 486, 207–214. doi: 10.1038/nature11234
Jiang, J., Wang, K., Chen, Y., Chen, H., Nice, E. C., Huang, C. (2017). Redox regulation in tumor cell epithelial–mesenchymal transition: molecular basis and therapeutic strategy. Signal Transduct Target Ther. 2, 17036. doi: 10.1038/sigtrans.2017.36
Kato, I., Yokokura, T., Mutai, M. (1984). Augmentation of mouse natural killer cell activity by Lactobacillus casei and its surface antigens. Microbiol. Immunol. 28, 209–217. doi: 10.1111/j.1348-0421.1984.tb00672.x
Kato, I., Yokokura, T., Mutai, M. (1988). Correlation between increase in Ia-bearing macrophages and induction of T cell-dependent antitumor activity by Lactobacillus casei in mice. Cancer Immunol. Immunother. 26, 215–221. doi: 10.1007/BF00199932
Knowles, M. A., Hurst, C. D. (2015). Molecular biology of bladder cancer: new insights into pathogenesis and clinical diversity. Nat. Rev. Cancer. 15, 25–41. doi: 10.1038/nrc3817
Kwak, M. K., Liu, R., Kwon, J. O., Kim, M. K., Kim, A. H., Kang, S. O. (2013). Cyclic dipeptides from lactic acid bacteria inhibit proliferation of the influenza A virus. J. Microbiol. 51 (6), 836–843. doi: 10.1007/s12275-013-3521-y
Lee, D. Y., Seo, Y. S., Rayamajhi, N., Kang, M. L., Lee, S. I., Yoo, H. S. (2009). Isolation, characterization, and evaluation of wild isolates of Lactobacillus reuteri from pig feces. J. Microbiol. 47, 663–672. doi: 10.1007/s12275-009-0124-8
Lewis, D. A., Brown, R., Williams, J., White, P., Jacobson, S. K., Marchesi, J. R., et al. (2013). The human urinary microbiome; bacterial DNA in voided urine of asymptomatic adults. Front. Cell Infect. Microbiol. 3, 41. doi: 10.3389/fcimb.2013.00041
Li, W. (2021). Study of the Characteristics and Tissue Distribution of Bladder Cancer-related Urinary System Microbiota and the Effect of Differential Bacteria on Bladder Cancer Cells (Shandong University, PhD dissertation). doi: 10.27272/d.cnki.gshdu.2021.000673
Li, W. T., Iyangar, A. S., Reddy, R., Chakladar, J., Bhargava, V., Sakamoto, K., et al. (2021). The bladder microbiome is associated with epithelial–mesenchymal transition in muscle invasive urothelial bladder carcinoma. Cancers (Basel). 13, 3649. doi: 10.3390/cancers13153649
Liévin, V., Peiffer, I., Hudault, S., Rochat, F., Brassart, D., Neeser, J. R., et al. (2000). Bifidobacterium strains from resident infant human gastrointestinal microflora exert antimicrobial activity. Gut 47, 646–652. doi: 10.1136/gut.47.5.646
Lindskrog, S. V., Prip, F., Lamy, P., Taber, A., Groeneveld, C. S., Birkenkamp-Demtroder, K., et al. (2021). An integrated multi-omics analysis identifies prognostic molecular subtypes of non-muscle-invasive bladder cancer. Nat. Commun. 12, 2301. doi: 10.1038/s41467-021-22465-w
Liu, F., Liu, A., Lu, X., Zhang, Z., Xue, Y., Xu, J., et al. (2019). Dysbiosis signatures of the microbial profile in tissue from bladder cancer. Cancer Med. 8, 6904–6914. doi: 10.1002/cam4.2419
Liu, Q., Roh, J. Y., Wang, Y., Choi, J. Y., Tao, X. Y., Kim, J. S., et al. (2012). Construction and characterisation of an antifungal recombinant Bacillus thuringiensis with an expanded host spectrum. J. Microbiol. 50, 874–877. doi: 10.1007/s12275-012-2201-7
Madeb, R., Messing, E. M. (2004). Gender, racial and age differences in bladder cancer incidence and mortality. Urol Oncol. 22, 86–92. doi: 10.1016/S1078-1439(03)00139-X
Mansour, B., Monyók, Á., Gajdács, M., Stercz, B., Makra, N., Pénzes, K., et al. (2022). B bladder tissue microbiome composition in patients of bladder cancer or benign prostatic hyperplasia and related human beta defensin levels. Biomedicines 10, 1758. doi: 10.3390/biomedicines10071758
Mansour, B., Monyók, Á., Makra, N., Gajdács, M., Vadnay, I., Ligeti, B., et al. (2020). Bladder cancer-related microbiota: examining differences in urine and tissue samples. Sci. Rep. 10, 11042. doi: 10.1038/s41598-020-67443-2
Marcon, A., Pesce, G., Calciano, L., Bellisario, V., Dharmage, S. C., Garcia-Aymerich, J., et al. (2018). Trends in smoking initiation in Europe over 40 years: A retrospective cohort study. PloS One 13, e0201881. doi: 10.1371/journal.pone.0201881
Matsumoto, S., Hara, T., Nagaoka, M., Mike, A., Mitsuyama, K., Sako, T., et al. (2009). component of polysaccharide peptidoglycan complex on Lactobacillus induced an improvement of murine model of inflammatory bowel disease and colitis-associated cancer. Immunology 128, e170–e180. doi: 10.1111/j.1365-2567.2008.02942.x
Miao, C., Ren, Y., Chen, M., Wang, Z., Wang, T. (2016). Microcystin-LR promotes migration and invasion of colorectal cancer through matrix metalloproteinase-13 up-regulation. Mol. Carcinog. 55, 514–524. doi: 10.1002/mc.22298
Morales, A., Eidinger, D., Bruce, A. W. (2017). Intracavitary Bacillus Calmette-Guerin in the treatment of superficial bladder tumors. J. Urol. 197, S142–S145. doi: 10.1016/j.juro.2016.10.101
Nadler, N., Kvich, L., Bjarnsholt, T., Jensen, J. B., Gögenur, I., Azawi, N. (2021). The discovery of bacterial biofilm in patients with muscle invasive bladder cancer. APMIS 129, 265–270. doi: 10.1111/apm.13097
Nguyen, M. T., Götz, F. (2016). Lipoproteins of gram-positive bacteria: key players in the immune response and virulence. Microbiol. Mol. Biol. Rev. 80, 891–903. doi: 10.1128/MMBR.00028-16
Ogawa, K., Shimizu, Y., Uketa, S., Utsunomiya, N., Kanamaru, S. (2020). Prognosis of patients with muscle invasive bladder cancer who are intolerable to receive any anti-cancer treatment. Cancer Treat. Res. Cancer Treat Res. Commun. 24, 100195. doi: 10.1016/j.ctarc.2020.100195
Ouwehand, A. C., Salminen, S., Isolauri, E. (2002). Probiotics: an overview of beneficial effects. Antonie Van Leeuwenhoek. 82, 279–289. doi: 10.1023/A:1020620607611
Parra-Grande, M., Oré-Arce, M., Martínez-Priego, L., D’Auria, G., Rosselló-Mora, R., Lillo, M., et al. (2022). Profiling the bladder microbiota in patients with bladder cancer. Front. Microbiol. 12, 718776. doi: 10.3389/fmicb.2021.718776
Pearce, M. M., Hilt, E. E., Rosenfeld, A. B., Zilliox, M. J., Thomas-White, K., Fok, C., et al. (2014). The female urinary microbiome: a comparison of women with and without urgency urinary incontinence. mBio 5, e01283–e01214. doi: 10.1128/mBio.01283-14
Pederzoli, F., Ferrarese, R., Amato, V., Locatelli, I., Alchera, E., Lucianò, R., et al. (2020). Sex-specific alterations in the urinary and tissue microbiome in therapy-naïve urothelial bladder cancer patients. Eur. Urol Oncol. 3, 784–788. doi: 10.1016/j.euo.2020.04.002
Raoult, D. (2017). Is there a link between urinary microbiota and bladder cancer? Eur. J. Epidemiol. 32 (3), 255. doi: 10.1007/s10654-016-0213-z
Richters, A., Aben, K. K. H., Kiemeney, L. A. L. M. (2020). The global burden of urinary bladder cancer: an update. World J. Urol. 38, 1895–1904. doi: 10.1007/s00345-019-02984-4
Rodriguez, R. M., Hernandez, B. Y., Menor, M., Deng, Y., Khadka, V. S. (2020). The landscape of bacterial presence in tumor and adjacent normal tissue across 9 major cancer types using TCGA exome sequencing. Comput. Struct. Biotechnol. J. 18, 631–641. doi: 10.1016/j.csbj.2020.03.003
Sagan, L. (1967). On the origin of mitosing cells. J. NIH Res 5, 65–72. doi: 10.1016/0022-5193(67)90079-3
Schirrmeister, B. E., Anisimova, M., Antonelli, A., Bagheri, H. C. (2011). Evolution of cyanobacterial morphotypes: Taxa required for improved phylogenomic approaches. Commun. Integr. Biol. 4, 424–427. doi: 10.4161/cib.16183
See, W. A., Zhang, G., Chen, F., Cao, Y., Langenstroer, P., Sandlow, J. (2009). Bacille-Calmette Guerin induces caspase-independent cell death in urothelial carcinoma cells together with release of the necrosis-associated chemokine high molecular group box protein 1. BJU Int. 103, 1714–1720. doi: 10.1111/j.1464-410X.2008.08274.x
Seong, C. N., Kang, J. W., Lee, J. H., Seo, S. Y., Woo, J. J., Park, C., et al. (2018). Taxonomic hierarchy of the phylum Firmicutes and novel Firmicutes species originated from various environments in Korea. J. Microbiol. 56, 1–10. doi: 10.1007/s12275-018-7318-x
Shah, G., Zielonka, J., Chen, F., Zhang, G., Cao, Y., Kalyanaraman, B., et al. (2014). H2O2 generation by bacillus Calmette-Guérin induces the cellular oxidative stress response required for bacillus Calmette-Guérin direct effects on urothelial carcinoma biology. J. Urol. 192, 1238–1248. doi: 10.1016/j.juro.2014.05.115
Sharma, A., Lee, S., Park, Y.-S. (2020). Molecular typing tools for identifying and characterizing lactic acid bacteria: a review. Food Sci. Biotechnol. 29, 1301–1318. doi: 10.1007/s10068-020-00802-x
Shida, K., Nomoto, K. (2013). Probiotics as efficient immunopotentiators: translational role in cancer prevention. Indian J. Med. Res. 138, 808–814.
Siddiqui, H., Lagesen, K., Nederbragt, A. J., Jeansson, S. L., Jakobsen, K. S. (2012). Alterations of microbiota in urine from women with interstitial cystitis. BMC Microbiol. 12, 205. doi: 10.1186/1471-2180-12-205
Stackebrandt, E., Sproer, C., Rainey, F. A., Burghardt, J., Päuker, O., Hippe, H. (1997). Phylogenetic analysis of the genus Desulfotomaculum: evidence for the misclassification of Desulfotomaculum guttoideum and description of Desulfotomaculum orientis as Desulfosporosinus orientis gen. nov., comb. nov. Int. J. Syst. Bacteriol. 47, 1134–1139. doi: 10.1099/00207713-47-4-1134
Stanton, C., Ross, R. P., Fitzgerald, G. F., Van Sinderen, D. (2005). Fermented functional foods based on probiotics and their biogenic metabolites. Curr. Opin. Biotechnol. 16, 198–203. doi: 10.1016/j.copbio.2005.02.008
Sumitomo, T., Nakata, M., Higashino, M., Terao, Y., Kawabata, S. (2013). Group A streptococcal cysteine protease cleaves epithelial junctions and contributes to bacterial translocation. J. Biol. Chem. 288, 13317–13324. doi: 10.1074/jbc.M113.459875
Sun, C. Q., Arnold, R. S., Hsieh, C. L., Dorin, J. R., Lian, F., Li, Z., et al. (2019). Discovery and mechanisms of host defense to oncogenesis: targeting the β-defensin-1 peptide as a natural tumor inhibitor. Cancer Biol. Ther. 20, 774–786. doi: 10.1080/15384047.2018.1564564
Sun, J. X., Xia, Q. D., Zhong, X. Y., Liu, Z., Wang, S. G. (2023). The bladder microbiome of NMIBC and MIBC patients revealed by 2bRAD-M. Front. Cell Infect. Microbiol. 13, 1182322. doi: 10.3389/fcimb.2023.1182322
Sung, H., Ferlay, J., Siegel, R. L., Laversanne, M., Soerjomataram, I., Jemal, A., et al. (2021). Global cancer statistics 2020: GLOBOCAN estimates of incidence and mortality worldwide for 36 cancers in 185 countries. CA Cancer J. Clin. 71, 209–249. doi: 10.3322/caac.21660
Swift, S., Lynch, M. J., Fish, L., Kirke, D. F., Tomás, J. M., Stewart, G. S., et al. (1999). Quorum sensing-dependent regulation and blockade of exoprotease production in Aeromonas hydrophila. Infect. Immun. 67, 5192–5199. doi: 10.1128/IAI.67.10.5192-5199.1999
Takagi, A., Matsuzaki, T., Sato, M., Nomoto, K., Morotomi, M., Yokokura (2001). Enhancement of natural killer cytotoxicity delayed murine carcinogenesis by a probiotic microorganism. Carcinogenesis 22, 599–605. doi: 10.1093/carcin/22.4.599
Tan, L. T. (2007). Bioactive natural products from marine cyanobacteria for drug discovery. Phytochemistry 68, 954–979. doi: 10.1016/j.phytochem.2007.01.012
Thomas-White, K., Brady, M., Wolfe, A. J., Mueller, E. R. (2016). The bladder is not sterile: history and current discoveries on the urinary microbiome. Curr. Bladder Dysfunct Rep. 11, 18–24. doi: 10.1007/s11884-016-0345-8
Thomas-White, K., Forster, S. C., Kumar, N., Van Kuiken, M., Putonti, C., Stares, M. D., et al. (2018). Culturing of female bladder bacteria reveals an interconnected urogenital microbiota. Nat. Commun. 9, 1557. doi: 10.1038/s41467-018-03968-5
Trikha, M., Corringham, R., Klein, B., Rossi, J. F. (2003). Targeted antiInterleukin-6 monoclonal antibody therapy for cancer: a review of the rationale and clinical evidence. Clin. Cancer Res. 9, 4653–4665.
Turroni, F., van Sinderen, D., Ventura, M. (2011). Genomics and ecological overview of the genus Bifidobacterium. Int. J. Food Microbiol. 149, 37–44. doi: 10.1016/j.ijfoodmicro.2010.12.010
Tuson, H. H., Foley, M. H., Koropatkin, N. M., Biteen, J. S. (2018). The starch utilization system assembles around stationary starch-binding proteins. Biophys. J. 115, 242–250. doi: 10.1016/j.bpj.2017.12.015
van Apeldoorn, M. E., van Egmond, H. P., Speijers, G. J. A., Bakker, G. J. I. (2007). Toxins of cyanobacteria. Mol. Nutr. Food Res. 51, 7–60. doi: 10.1002/mnfr.200600185
Ventura, M., Canchaya, C., Tauch, A., Chandra, G., Fitzgerald, G. F., Chater, K. F., et al. (2007). Genomics of Actinobacteria: tracing the evolutionary history of an ancient phylum. Microbiol. Mol. Biol. Rev. 71, 495–548. doi: 10.1128/MMBR.00005-07
Vétizou, M., Pitt, J. M., Daillère, R., Lepage, P., Waldschmitt, N., Flament, C., et al. (2015). Anticancer immunotherapy by CTLA-4 blockade relies on the gut microbiota. Science 350, 1079–1084. doi: 10.1126/science.aad1329
Wang, T. C., Jan, K. Y., Wang, A. S. S., Gurr, J. R. (2007). Trivalent arsenicals induce lipid peroxidation, protein carbonylation, and oxidative DNA damage in human urothelial cells. Mutat. Res. 615, 75–86. doi: 10.1016/j.mrfmmm.2006.10.003
Welsh, E. A., Liberton, M., Stöckel, J., Loh, T., Elvitigala, T., Wang, C., et al. (2008). The genome of Cyanothece 51142, a unicellular diazotrophic cyanobacterium important in the marine nitrogen cycle. Proc. Natl. Acad. Sci. U S A. 105, 15094–15099. doi: 10.1073/pnas.0805418105
Wexler, M. H. (2007). Bacteroides: the good, the bad, and the nitty-gritty. Clin. Microbiol. Rev. 20, 593–621. doi: 10.1128/CMR.00008-07
Whiteside, S. A., Razvi, H., Dave, S., Reid, G., Burton, J. P. (2015a). The microbiome of the urinary tract—a role beyond infection. Nat. Rev. Urol. 12, 81–90. doi: 10.1038/nrurol.2014.361
Whiteside, S. A., Razvi, H., Dave, S., Reid, G., Burton, J. P. (2015b). The microbiome of the urinary tract–a role beyond infection. Nat. Rev. Urol. 12, 81–90. doi: 10.1038/nrurol.2014.361
Wolfe, A. J., Toh, E., Shibata, N., Rong, R., Kenton, K., Fitzgerald, M., et al. (2012). Evidence of uncultivated bacteria in the adult female bladder. J. Clin. Microbiol. 50, 1376–1383. doi: 10.1128/JCM.05852-11
Wu, Z., Hoover, D. M., Yang, D., Boulègue, C., Santamaria, F., Oppenheim, J. J., et al. (2003). Engineering disulfide bridges to dissect antimicrobial and chemotactic activities of human beta-defensin 3. Proc. Natl. Acad. Sci. U S A. 100, 8880–8885. doi: 10.1073/pnas.1533186100
Yacouba, A., Tidjani Alou, M., Lagier, J. C., Dubourg, G., Raoult, D. (2022). Urinary microbiota and bladder cancer: A systematic review and a focus on uropathogens. Semin. Cancer Biol. 86, 875–884. doi: 10.1016/j.semcancer.2021.12.010
Yang, D., Chertov, O., Bykovskaia, S. N., Chen, Q., Buffo, M. J., Shogan, J., et al. (1999). Beta-defensins: linking innate and adaptive immunity through dendritic and T cell CCR6. Science 286, 525–528. doi: 10.1126/science.286.5439.525
Yu, D. S., Wu, C. L., Ping, S. Y., Keng, C., Shen, K. H. (2015). Bacille Calmette-Guerin can induce cellular apoptosis of urothelial cancer directly through toll-like receptor 7 activation. Kaohsiung J. Med. Sci. 31, 391–397. doi: 10.1016/j.kjms.2015.05.005
Yun, S. J., Kim, W. J. (2013). Role of the epithelial-mesenchymal transition in bladder cancer: from prognosis to therapeutic target. Korean J. Urol. 54, 645–650. doi: 10.4111/kju.2013.54.10.645
Keywords: bladder cancer, microbiota, review, prognosis, mechanism
Citation: Lou K, Chi J, Wu J, Ma J, Liu S and Cui Y (2024) Research progress on the microbiota in bladder cancer tumors. Front. Cell. Infect. Microbiol. 14:1374944. doi: 10.3389/fcimb.2024.1374944
Received: 23 January 2024; Accepted: 26 March 2024;
Published: 08 April 2024.
Edited by:
Suhana Chattopadhyay, University of Maryland, College Park, United StatesReviewed by:
Muhammad Akbar Shahid, Bahauddin Zakariya University, PakistanFrancesca Pirini, Scientific Institute of Romagna for the Study and Treatment of Tumors (IRCCS), Italy
Copyright © 2024 Lou, Chi, Wu, Ma, Liu and Cui. This is an open-access article distributed under the terms of the Creative Commons Attribution License (CC BY). The use, distribution or reproduction in other forums is permitted, provided the original author(s) and the copyright owner(s) are credited and that the original publication in this journal is cited, in accordance with accepted academic practice. No use, distribution or reproduction is permitted which does not comply with these terms.
*Correspondence: Yuanshan Cui, ZG9jdG9yY3VpeXNAMTYzLmNvbQ==; Shu Liu, emhvbmcyMDEwc2hhbkAxNjMuY29t
†These authors have contributed equally to this work and share first authorship