- 1Division of Thoracic and Cardiovascular Surgery, Chiayi Chang Gung Memorial Hospital, Chiayi, Taiwan
- 2Division of Infectious Diseases, Department of Internal Medicine, Chiayi Chang Gung Memorial Hospital, Chiayi, Taiwan
- 3College of Medicine, Chang Gung University, Taoyuan, Taiwan
- 4Division of Cardiovascular Surgery, New Taipei Municipal TuCheng Hospital, New Taipei, Taiwan
- 5Division of Thoracic and Cardiovascular Surgery, Chiayi Hospital, Ministry of Health and Welfare, Chiayi, Taiwan
Introduction: Staphylococcus aureus, is a pathogen commonly encountered in both community and hospital settings. Patients receiving hemodialysis treatment face an elevated risk of vascular access infections (VAIs) particularly Staphylococcus aureus, infection. This heightened risk is attributed to the characteristics of Staphylococcus aureus, , enabling it to adhere to suitable surfaces and form biofilms, thereby rendering it resistant to external interventions and complicating treatment efforts.
Methods: Therefore this study utilized PCR and microtiter dish biofilm formation assay to determine the difference in the virulence genes and biofilm formation among in our study collected of 103 Staphylococcus aureus, isolates from hemodialysis patients utilizing arteriovenous grafts (AVGs), tunneled cuffed catheters (TCCs), and arteriovenous fistulas (AVFs) during November 2013 to December 2021.
Results: Our findings revealed that both MRSA and MSSA isolates exhibited strong biofilm production capabilities. Additionally, we confirmed the presence of agr types and virulence genes through PCR analysis. The majority of the collected isolates were identified as agr type I. However, agr type II isolates displayed a higher average number of virulence genes, with MRSA isolates exhibiting a variety of virulence genes. Notably, combinations of biofilm-associated genes, such as eno−clfA−clfB−fib−icaA−icaD and eno−clfA−clfB−fib−fnbB−icaA−icaD, were prevalent among Staphylococcus aureus, isolates obtained from vascular access infections.
Discussion: These insights contribute to a better understanding of the molecular characteristics associated with Staphylococcus aureus, infections in hemodialysis patients and provided more targeted and effective treatment approaches.
1 Introduction
Staphylococcus aureus, a gram-positive bacterium, represents a significant source of infection in both communities and medical institutions. It has developed resistance to a diverse range of antibacterial drugs, giving rise to multi-drug strains such as MRSA (Methicillin-Resistant Staphylococcus aureus), presenting considerable challenges in treatment (Lowy, 1998; Taylor and Unakal, 2022). S. aureus can colonize various parts of the human body, including the skin, nasal cavity, and more. Upon the onset of wounds, the bacteria invade, leading to skin and soft tissue infections, osteomyelitis, pneumonia, septic arthritis, bacteremia, and endocarditis. Notably, Staphylococcus aureus stands as a primary cause of vascular access infection and bacteremia in dialysis patients (Winstel et al., 2019).
Hemodialysis (HD), a life-supporting treatment for individuals with compromised kidney function (Sockrider and Shanawani, 2017), involves three types of vascular access: arteriovenous fistulas (AVFs), arteriovenous grafts (AVGs), and central venous catheters (CVCs) (Maya and Allon, 2008). Despite its life-saving nature, hemodialysis is associated with a heightened risk of morbidity and mortality (LaFrance et al., 2008). During dialysis, patients face an increased risk of intermittent or persistent carriage of S. aureus. Moreover, hemodialysis patients with S. aureus exhibit a 1.8−4.7 fold higher risk of vascular access infections and bacteremia compared to non-S. aureus carriers (Vandecasteele et al., 2009).
Bacterial biofilms, intricate aggregations of bacteria embedded in an extracellular matrix (ECM), pose formidable challenges due to their resistance to mechanical interference, innate and acquired host defenses, and antibiotic treatments. These biofilms contribute significantly to chronic infections, particularly in hospital settings (Costerton et al., 1999). Staphylococcus aureus, known for its biofilm-forming capabilities, is notorious for causing chronic infections by resisting therapeutic interventions, especially on indwelling medical devices such as implanted artificial heart valves, catheters, and joint prosthetics (Ribeiro et al., 2012; Moormeier and Bayles, 2017).
Pathogenic S. aureus actively engages in the host-pathogen interaction by expressing various virulence genes, which facilitate colonization and infection. These virulence factors, regulated by the accessory gene regulator (agr) locus, categorize S. aureus into four groups: type I−IV. These factors not only enable pathogens to enter host tissues, evade immune responses, and attach to host cells but also induce tissue damage through the secretion of exoenzymes and toxins (Kadkhoda et al., 2020; Derakhshan et al., 2021). Microbial surface components recognizing adhesive matrix molecules (MSCRAMMs) on the surface of S. aureus play a crucial role in mediating adhesion between bacteria and the host, facilitating essential steps in infection, including clumping factors A, B; fibronectin binding proteins A, B; fibrinogen binding protein; laminin binding protein; collagen binding protein; elastin binding protein; bone sialo-protein binding protein, and ica (intercellular adhesion) operon, mediating cell adhesion and biofilm formation. Additionally, other virulence factors, such as Panton-Valentine leucocidin (PVL), staphylococcal enterotoxins (SEs; SEA to SEE), exfoliative toxins (ETs: ETA and ETB), or toxic shock syndrome toxin-1, are regulated by corresponding genes and contribute to infectious diseases (Mehrotra et al., 2000; Ghasemian et al., 2015; Pakbaz et al., 2017; Idrees et al., 2021; Koosha et al., 2014).
In summary, this study aims to discern the profile of virulence−associated genes, agr types, and biofilm formation ability in hemodialysis patients with different dialysis vascular access.
2 Methods and materials
2.1 Bacterial isolation, collection, and identification
This study was conducted at Chiayi Chang Gung Memorial Hospital in Chiayi, Taiwan. A total of 103 Staphylococcus aureus isolates were collected from hemodialysis patients experiencing vascular access infections, including arteriovenous fistulas (AVFs), prosthetic arteriovenous grafts (AVGs), and tunneled–cuffed catheters (TCCs), spanning the period from November 2013 to December 2021. The bacterial isolates were obtained from abscesses, blood, Hickman catheter tips, pus, tissue, and wounds, and cultured on blood agar plates (BAP). Initial identification was accomplished through standard biochemical tests, including catalase and coagulase tests until 2019, with a transition to matrix–assisted laser desorption/ionization time–of–flight mass spectrometry (MALDI–TOF) thereafter. Routine cultivation adhered to laboratory standards on tryptic soy agar and tryptic soy broth, and all isolates were preserved in a 15% glycerol stock at −80°C.
2.2 Genomic DNA extraction
A single colony of S. aureus was inoculated in Tryptone Soy Broth (TSB) for 16 hours. The overnight culture was centrifuged, and the pellet was resuspended in 1 ml of ultrapure water, heated at 100°C for 15 min, and the supernatant containing DNA was stored at 4°C for subsequent use.
2.3 Polymerase chain reaction
PCR amplification, performed in a 25μl reaction mixture, included 1μl of each primer, 2 μl of DNA template, and 12μl of 2x KAPA2G Fast HotStart ReadyMix with dye (Roche, USA). After amplification, the samples were analyzed on a 1.5% agarose gel via electrophoresis, and DNA fragments were visualized using UV light.
2.4 Identification of methicillin−resistant S. aureus
Isolates were identified as MRSA based on oxacillin resistance and the presence of the mecA gene, detected using PCR with previously described primers (Pournajaf et al., 2014). It’s important to note that we classified oxacillin–sensitive mecA–positive isolates in this study as MRSA base on a previous study (Hososaka et al., 2007).
2.5 Detection of agr types and virulence−associated genes
Amplified agr genes were categorized into agr types (I−IV) using multiplex PCR (Bibalan et al., 2014). For virulence gene identification, two sets of primers were utilized for multiplex PCR, PCR1 to amplify bbp, cna, ebpS, and eno and PCR2 to amplify fnbA, fnbB, fib, clfA, and clfB (Tristan et al., 2003)., with an additional set designed to amplify sea, seb, sec, sed, see, eta, etb, tst, and PVL (Mehrotra et al., 2000; Mcclure et al., 2006). PCR methods for icaA and icaD genes were also employed (Vasudevan et al., 2003).
2.6 Biofilm production ability
The biofilm formation assay followed a previously established protocol (Stepanović et al., 2000). A colony of S. aureus was isolated from a tryptone soy agar (TSA) plate and inoculated into tryptone soy broth, then incubated for 12–16 hours at 37°C. The culture was diluted in tryptone soy broth with 0.25% glucose and added to 96 flat–bottom polystyrene wells, followed by incubation for 24 hours at 37°C.
Subsequently, the planktonic cells were aspirated, and the plate was washed twice with sterile saline water to remove non–adherent bacterial cells. The attached bacteria were fixed with 99% methanol for 15 minutes, then the plates were emptied and air–dried. Next, 0.1% crystal violet was added to each well, and after 15 minutes, the excess crystal violet was removed by washing the plate twice with water and air–drying.
Finally, the cell–bound crystal violet was dissolved in 99% ethanol and allowed to stand for 15 minutes. Optical density (OD) of the isolates was monitored by measuring absorbance at 570 nm using a microplate reader (Perkin Elmer Enspire 2300, Perkin Elmer, USA). The negative control consisted of only broth, while the biofilm‐forming S. aureus reference strain, ATCC 29213, was used as the positive control.
The adherence capabilities of the tested isolates were classified into four categories following the method described by previous research (Christensen et al., 1985). The cut–off value (ODc) was established as three standard deviations (SD) above the mean OD of the negative control: ODc = average OD of negative control + (3 × SD of negative control).
Strains were classified into the following categories:
OD ≤ ODc = no biofilm producer,
ODc < OD ≤ 2 × ODc = weak biofilm producer,
2 × ODc < OD ≤ 4 × ODc = moderate biofilm producer,
4 × ODc < OD = strong biofilm producer.
3 Results
3.1 The ratio of methicillin−resistant S. aureus and methicillin−sensitive S. aureus from different vascular access infections
A total of 103 Staphylococcus aureus isolates were collected from three types of vascular access infections (Figure 1). The majority of isolates were TCC–MRSA and AVG–MRSA, constituting 31.1% (32/103) and 27.2% (28/103), respectively. Following were 24.3% (25/103) AVG–MSSA and 15.5% (16/103) TCC–MSSA, with AVF–MRSA being less prevalent at only 1.9% (2/103). Some of the isolates, collected from different sites among the 103 S. aureus isolates, belong to the same patients, therefore, they were be considered as individual isolates. Furthermore, the age range of patients was predominantly between 40 and 90 years old. Females more than males, and AVG−S. aureus isolates were predominantly from female patients. Regarding specimens, the majority of AVF and AVG−S. aureus isolates were found in blood, pus, and wound samples, whereas TCC−S. aureus isolates were primarily from tip. This disparity could be associated with the pattern of dialysis access (Table 1).
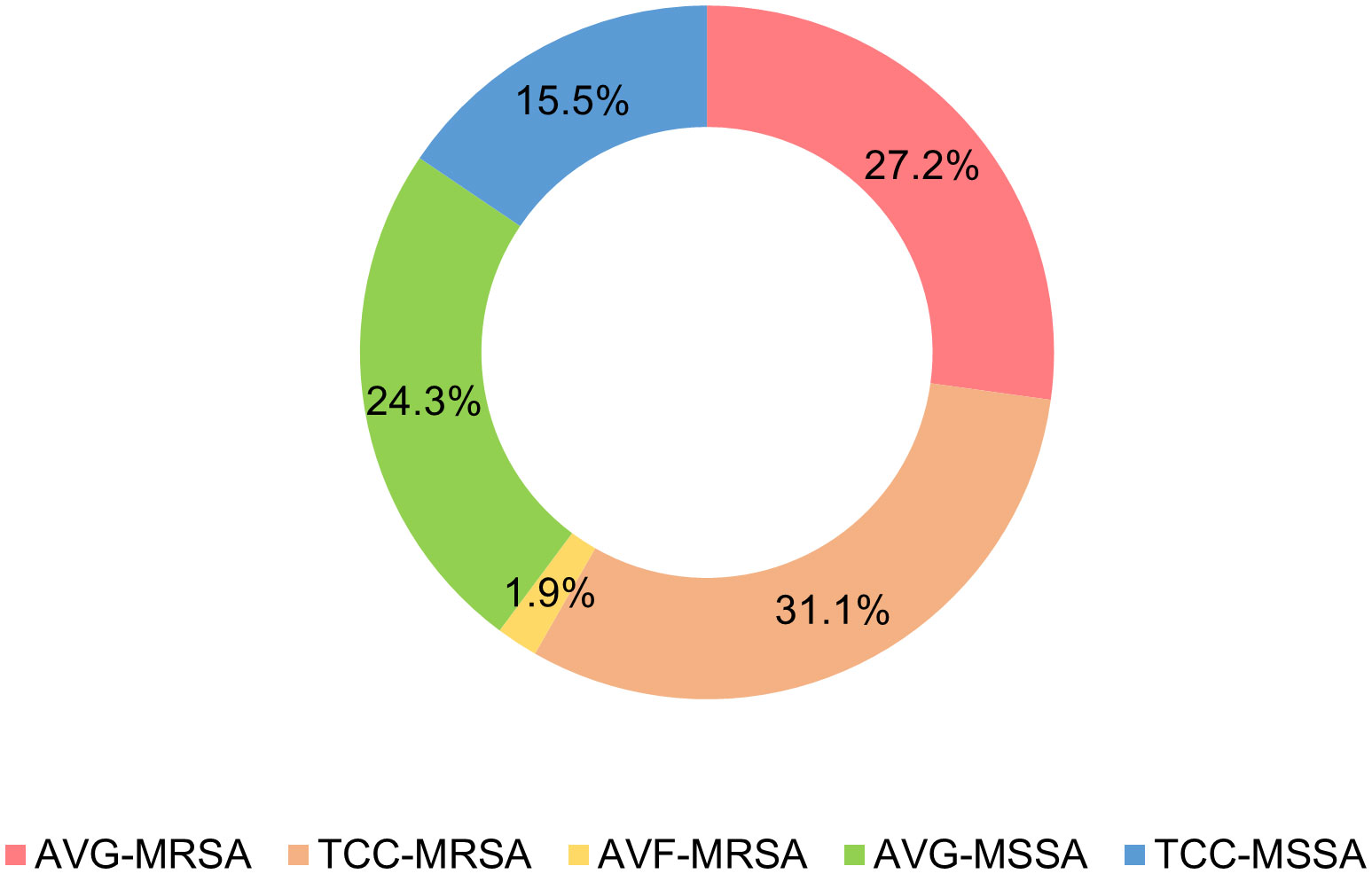
Figure 1 Distribution of Staphylococcus aureus isolates from vascular access infections. AVF, arteriovenous fistulas, AVG, prosthetic arteriovenous grafts, TCC, tunneled–cuffed catheters, MRSA, methicillin–resistant S. aureus, MSSA, methicillin–sensitive S. aureus.
3.2 Distribution of agr types of S. aureus isolates from vascular access infections
Through multiplex PCR, four agr types were investigated among the 103 S. aureus isolates (Figure 2). A small number of MRSA isolates, specifically 7.1% (2/28) AVG–MRSA and 9.4% (3/32) TCC–MRSA, were non–typeable for the agr locus, labeled as agr–negative isolates. Agr I was prevalent in AVG–MRSA, TCC–MRSA, AVF–MRSA, AVG–MSSA, and TCC–MSSA, constituting 78.6% (22/28), 71.9% (23/32), 100% (2/2), 48% (12/25), and 62.5% (10/16), respectively. Agr II was the secondary prevalent type in MSSA isolates, with AVG–MSSA harboring 44.0% (11/25) and TCC–MSSA harboring 31.3% (5/16). Agr III was the following prevalent type in MRSA isolates, constituting 10.7% (3/28) AVG–MRSA and 9.4% (3/32) TCC–MRSA. Agr IV was only detected in TCC–MRSA at 3.1% (1/32).
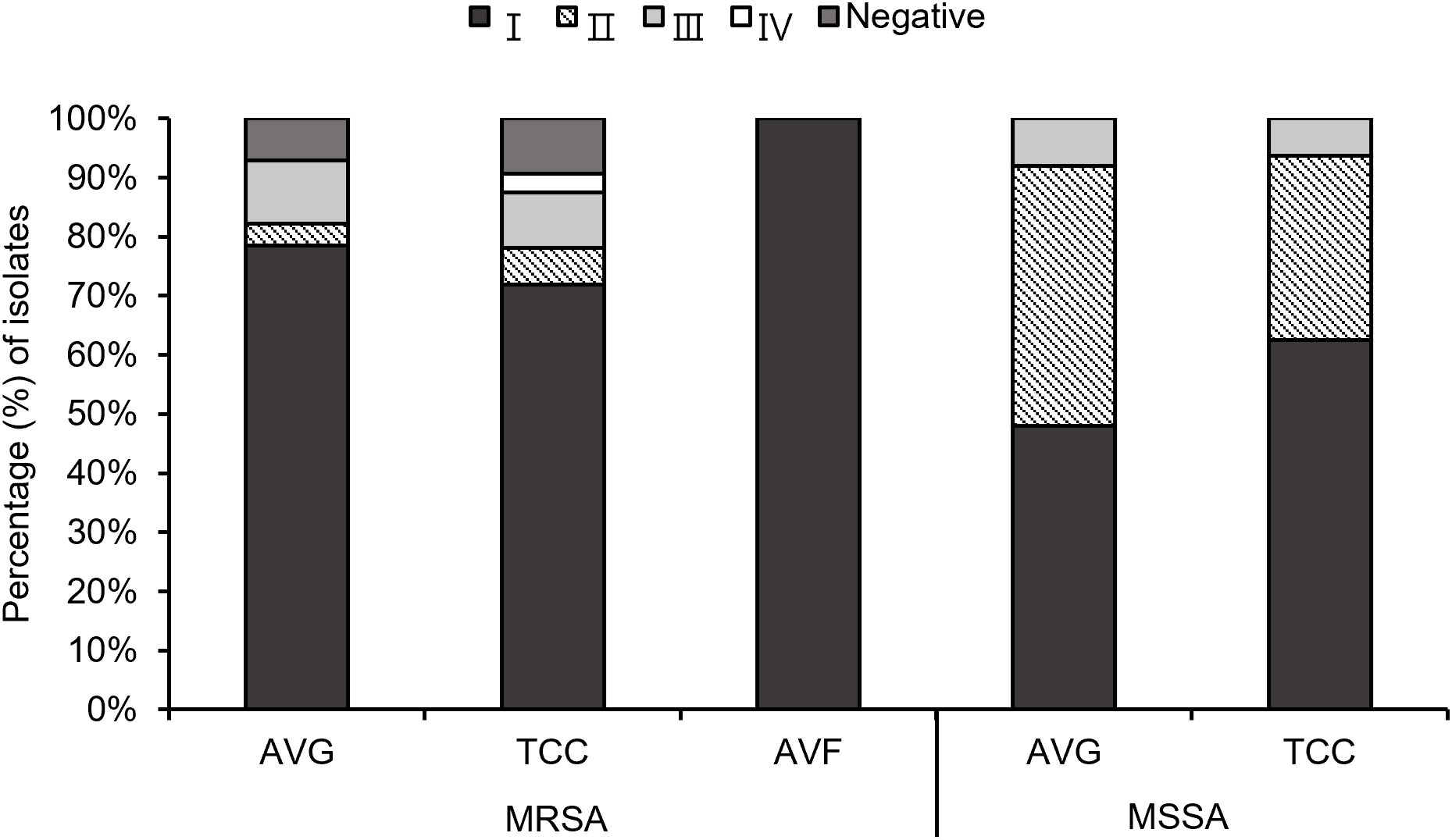
Figure 2 The profile of agr types among methicillin–resistant S. aureus (MRSA) and methicillin–sensitive S. aureus (MSSA) isolates from different vascular access infections.
3.3 The prevalence of virulence−associated genes among S. aureus isolates from different vascular access infections
Using PCR to detect 20 virulence−associated genes, eno (100%), clfA (100%), clfB (100%), and icaD (100%) genes were prevalent in all S. aureus isolates from different vascular access infections. Following were fib (68.9%), and icaA (81.5%) genes. fnbA, sed, see, and etb genes were not observed in S. aureus isolates (Figure 3). The variety of virulence genes was more pronounced among AVG–MRSA and TCC–MRSA than MSSA isolates. Furthermore, it is evident that eno, clfA, and clfB genes were prevalent in S. aureus isolates from blood and other sites, followed by fib genes. However, except for the bbp genes, which were only detected in S. aureus isolates from other sites, the quantity of virulence genes in S. aureus isolates showed minimal differences between blood sites and other sites (Table 2).
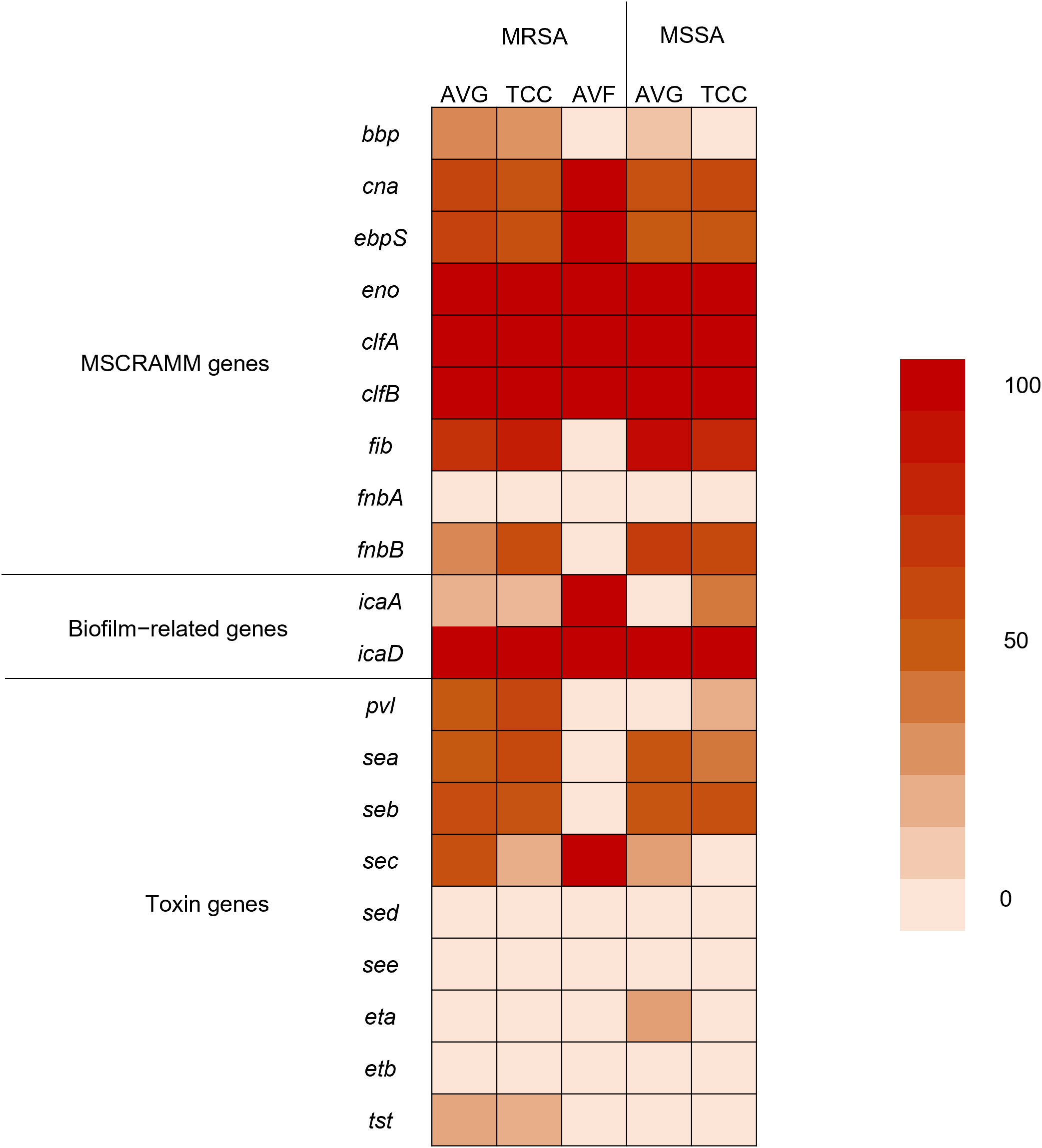
Figure 3 Heatmap indicating the prevalence of virulence genes among methicillin–resistant S. aureus (MRSA) and methicillin–sensitive S. aureus (MSSA) isolates from different vascular access infections.
3.4 Distribution of the average number of virulence−associated genes among S. aureus isolates from vascular access infections in different agr types
The average number of virulence−associated genes was investigated according to agr genotype, calculated as the total number of genes in each agr type with different vascular access infections divided by the total number of isolates in each agr type with different vascular access infections. The average number of virulence genes was 7.23 in all S. aureus isolates. Among MRSA isolates, agr I and II was harbored most average number of virulence gene (I: 7.45 for AVG−MRSA, 8 for AVF−MRSA, II: 8.5 for TCC−MRSA), in MSSA isolates, agr II and III had most average number of virulence gene (II: 7.2 for TCC−MSSA and III: 8 for AVG−MSSA). A lower average number of virulence genes was detected in agr−negative isolates compared to agr−positive isolates among TCC–MRSA (Figure 4A). In all S. aureus isolates, the content of virulence−associated genes, agr II isolates had the highest average number of virulence genes (averaging 7.37), whereas agr−negative isolates had the lowest average number of virulence genes (averaging 6.8) (Figure 4B).
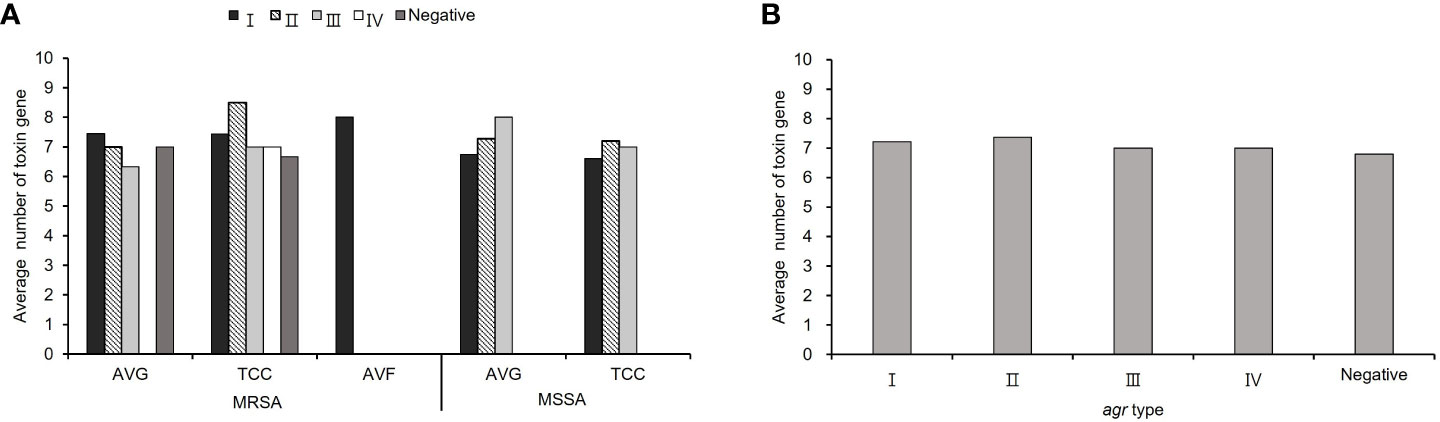
Figure 4 The virulence−associated gene content of methicillin–resistant S. aureus (MRSA) and methicillin–sensitive S. aureus (MSSA) isolates in different agr types. (A) The average number of virulence genes from S. aureus with different vascular access infections. (B) The average number of virulence genes in the agr− positive and negative isolates among all S. aureus isolates.
3.5 Biofilm production ability of S. aureus isolates from vascular access infections
Biofilm production ability was analyzed, and all S. aureus isolates from vascular access infections were able to produce biofilm (Figure 5). Over 75% of MRSA and MSSA isolates exhibited a strong biofilm production ability after 24 hours of incubation, including 78.6% (22/28) AVG–MRSA, 90.6% (29/32) TCC–MRSA, 88.0% (22/25) AVG–MSSA, and 87.5% (14/16) TCC–MSSA. All AVF–MRSA isolates produced a moderate biofilm ability. The results indicated no significant difference in biofilm production ability between MRSA and MSSA, with most MRSA and MSSA demonstrating strong biofilm–forming capabilities.
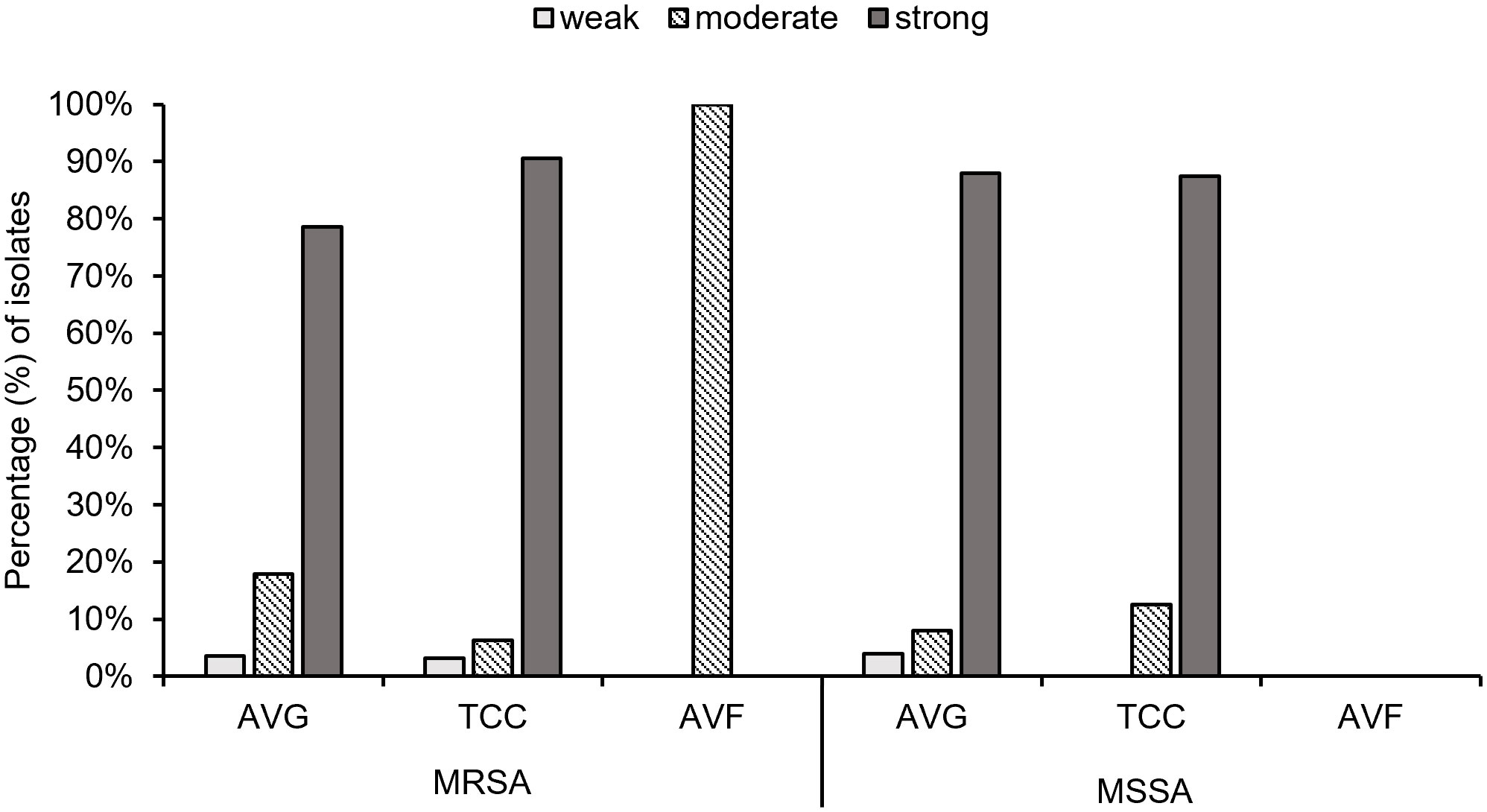
Figure 5 The biofilm production ability of methicillin–resistant S. aureus (MRSA) and methicillin–sensitive S. aureus (MSSA) isolates from different vascular access infections.
3.6 The correlation of agr types and biofilm production ability
Both agr–positive and agr–negative isolates exhibited strong biofilm formation ability. Agr type II isolates collected were all strong biofilm producers, while agr type III isolates collected, except AVG–MRSA, were all strong biofilm producers. Agr type I isolates with strong biofilm production ability also demonstrated moderate and weak biofilm production ability among all S. aureus isolates (Table 3).
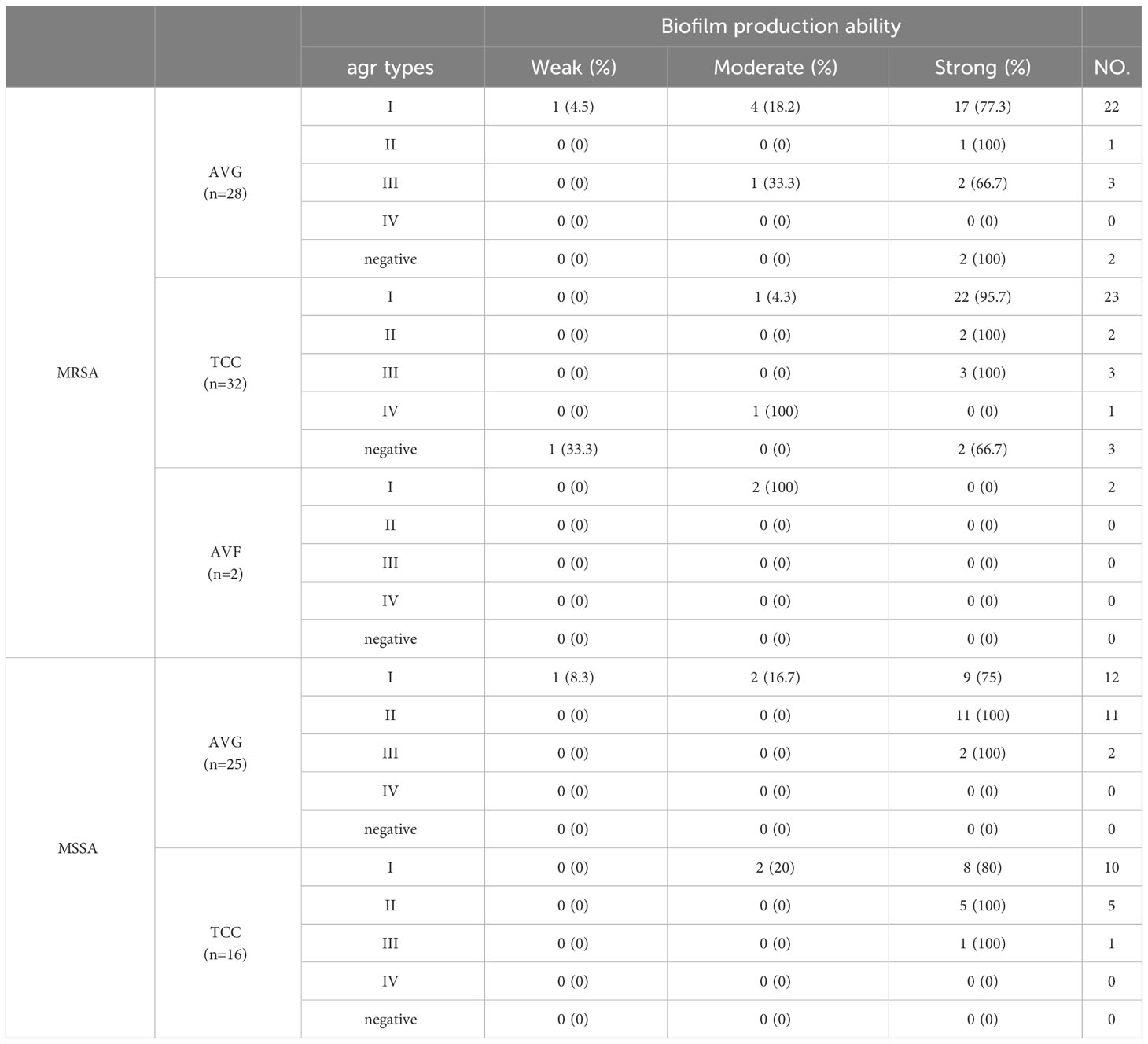
Table 3 The relationship between different types of agr and biofilm formation ability among methicillin–resistant S. aureus (MRSA) and methicillin–sensitive S. aureus (MSSA) isolates from vascular access infections.
3.7 The correlation between biofilm–related gene combinations and biofilm production ability among S. aureus isolates
A comprehensive analysis was conducted to examine the relationship between 18 combinations of biofilm−related genes and their association with biofilm production capacity among S. aureus isolates (Table 4). The most prevalent gene patterns contributing to strong biofilm production were eno−clfA−clfB−fib−icaA−icaD (18.4%, 19/103) and eno−clfA−clfB−fib−fnbB−icaA−icaD (18.4%, 19/103). These patterns were observed in isolates encompassing AVG–MRSA, TCC–MRSA, AVG–MSSA, and TCC–MSSA. Secondary patterns of virulence genes, such as cna−ebpS−eno−clfA−clfB−icaA−icaD (11.6%, 12/103), were primarily found in MRSA isolates, correlating with both moderate and weak biofilm production capabilities.
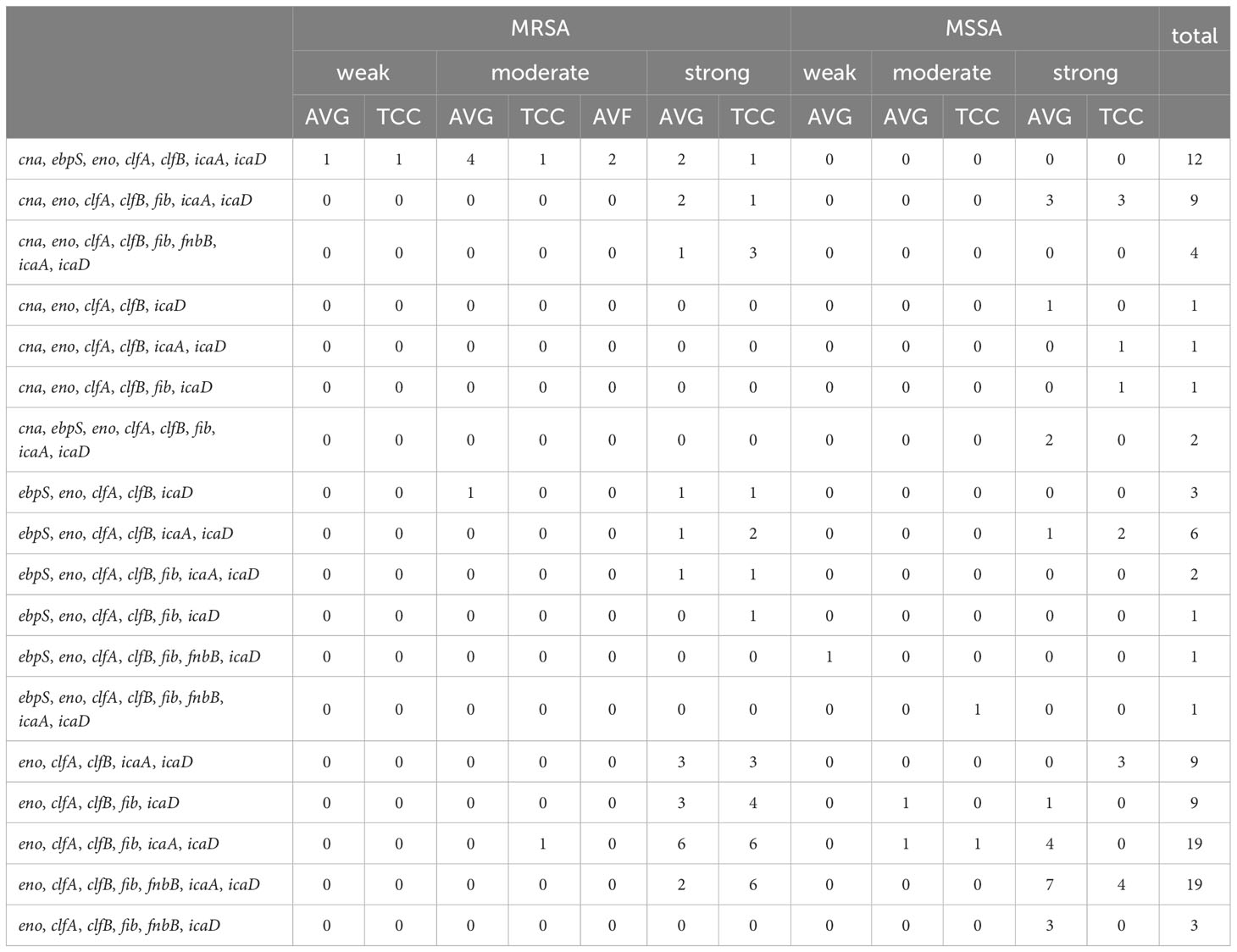
Table 4 The relationship between biofilm−related gene combinations and biofilm production capacity of methicillin–resistant S. aureus (MRSA) and methicillin–sensitive S. aureus (MSSA) isolates in vascular access infections.
4 Discussion
Staphylococcus aureus carriage is a pivotal factor in Staphylococcus aureus infections among dialysis patients, contributing to increased morbidity and mortality in both hemodialysis and peritoneal dialysis settings. Carriage of Staphylococcus aureus in the anterior nasal cavity emerges as a significant risk factor for infections. Prior investigations have revealed that 40%−60% of hemodialysis patients harbor Staphylococcus aureus in the anterior nasal cavity, with a carriage rate and infection risk double that of healthy controls. Molecular typing of strains carried by individuals has shown that strains from the nasal cavity, skin, and infection sites match, indicating bacterial transmission from the nose to hands and subsequently to the skin, causing infections through foreign bodies such as grafts (introduced through venipuncture) or dialysis catheters (HD or PD). The entry of catheters may result in contamination during catheter insertion or through the tunnel at the exit site, leading to access site infections (Kirmani, 1978; Piraino, 2000; Balaban et al., 2003). In this study, we collected 145 Staphylococcus aureus isolates, of which 71% (103/145) were sourced from vascular access infections. The ratio of infections from arteriovenous grafts (AVG) to tunneled–cuffed catheters (TCC) infections was approximately 1:1, with sporadic isolates from arteriovenous fistula (AVF) infections.
Biofilm formation is a critical adaptive and survival strategy employed by bacteria, occurring on both biotic and abiotic surfaces in various environments, including healthcare settings. Extracellular polymeric substances (EPS) produced during biofilm formation protect bacteria from adverse environmental factors and immune responses (Kostakioti et al., 2013; Tan et al., 2014). Bacteria enclosed in biofilms can adhere to wounds, hindering the healing process of chronic wounds and producing toxins that impede wound healing or confer resistance to antibiotics (Rajpaul, 2015). Previous studies have implicated biofilm formation as a risk factor for bacterial infections in hemodialysis patients, with Staphylococcus aureus identified as a great biofilm producer (Marques et al., 2017; Kwiecinski et al., 2019). Our study confirms that all Staphylococcus aureus isolates from vascular access infections demonstrated biofilm formation ability, with 84.5% (87/103) classified as strong biofilm producers. Among these, MRSA isolates (58.6%, 51/87) exhibited a higher prevalence of strong biofilm production compared to MSSA isolates (41.4%, 36/87), particularly TCC–MRSA, with 33.3% (29/87) of strong biofilm isolates.
Biofilm formation involves microbial cell attachment to surfaces and subsequent accumulation, a key factor in infection development. Adhesion formation, a major virulence factor of Staphylococcus aureus, is correlated with various genes, including bbp, cna, ebpS, eno, clfA, clfB, fib, fnbA, fnbB, and the ica group genes. Previous studies have identified clfA, clfB, fib, eno, icaA, and icaD genes as prevalent in Staphylococcus aureus isolates (Atshan et al., 2012; Ghasemian et al., 2015; Xu et al., 2021). Our study yielded similar results, with all MRSA and MSSA isolates harboring eno, clfA, clfB, and icaD genes, followed by fib and icaA genes. However, fnbA genes were not detected in any isolates, diverging from previous research. Staphylococcus aureus infections are also associated with various virulence genes, including Panton–Valentine leukocidin (PVL), toxic shock syndrome toxin–1 (TSST–1), exfoliative toxins (ETs), and staphylococcal enterotoxins (SEs). Although PVL is linked to leukocyte cytolysis, and SEs and TSST–1 primarily cause food poisoning and toxic shock syndrome, our study identified a low frequency of pvl, tst, eta, etb, sea, seb, sec, sed, and see genes in all Staphylococcus aureus isolates, with eta, etb, tst, sed, and see genes nearly absent, aligning with previous reports (Wang et al., 2021).
Patients infected with Staphylococcus aureus may experience various illnesses, including sepsis, pneumonia, septic arthritis, osteomyelitis, toxic shock syndrome following surgery, folliculitis, endocarditis, and urinary tract infections. The accessory gene regulator (agr) system plays a crucial role in controlling and regulating the expression of virulence genes in Staphylococcus aureus. This system classifies S. aureus isolates into four groups (type I, II, III, IV) based on amino acid polymorphisms of AgrB, AgrD, and AgrC. Several studies have associated specific diseases with different agr groups, such as invasive infections linked to agr group I strains and non−invasive infections to agr group III strains. Notably, agr group I predominates among S. aureus isolates (Bibalan et al., 2014; Javdan et al., 2019; Tan et al., 2022). Our study observed a prevalence of agr type I (67%, 69/103) in S. aureus isolates from vascular access infections, particularly in MRSA isolates. Calculations of the average number of virulence genes in each agr type revealed that agr II isolates had the highest average number of virulence genes, consistent with previous studies (Zhang et al., 2018). Additionally, MRSA isolates exhibited a slightly higher average number of virulence genes (8.1, 502/62) than MSSA isolates (7.9, 324/41).
Correlations between agr group and biofilm formation, as well as between virulence genes and biofilm formation, were explored. Previous studies have identified agr groups II and III as the primary biofilm producers among the four types, with agr type II in MRSA exhibiting superior biofilm formation ability (Tan et al., 2018). Our study corroborates these findings, revealing that agr group II constituted strong biofilm producers among collected S. aureus isolates from various vascular infections. The prevalence of biofilm−associated genes, specifically the combination of fib−eno−clfA−clfB−ebpS−icaA−icaD (Kaźmierczak et al., 2021), aligns with previous reports, with eno−clfA−clfB−fib−icaA−icaD and eno−clfA−clfB−fib−fnbB−icaA−icaD being prevalent gene patterns in our study. The following pattern, cna−ebpS−eno−clfA−clfB−icaA−icaD, was also observed. Thus, eno, clfA, clfB, icaA, and icaD genes appear more frequently in biofilm–producing isolates of Staphylococcus aureus.
While this study investigated the molecular, phenotypic, and genotypic characteristics of S. aureus isolates from different vascular access infections, it has limitations. Conducted over an 8–year period at a single institution, the varying frequencies of isolates collected each year and the small sample size may introduce bias.
5 Conclusion
All the MRSA and MSSA isolates obtained from vascular access infections (VAIs) in our study exhibited biofilm–forming capabilities, particularly demonstrating strong biofilm capacity. Subsequent PCR identification confirmed that the majority of Staphylococcus aureus isolates belonged to agr type I. Additionally, the analysis of virulence genes revealed that AVG−MRSA and TCC−MRSA isolates displayed the most diverse array of virulence genes. Molecular testing of Staphylococcus aureus in the context of vascular access infections is crucial for advancing infection management and developing effective treatment strategies.
Data availability statement
All experimental data during this study are included in this published article.
Ethics statement
The studies were approved by Institutional Review Board (IRB) of Chang Gung Memorial Hospital (IRB201508482B0 and IRB201901354B0). The studies were conducted in accordance with the local legislation and institutional requirements. The bacteria samples used in this study were acquired from Department of Laboratory Medicine from the Chang Gung Memorial Hospital, Chiayi, Taiwan. Written informed consent for participation was obtained from the participants or the participants’ legal guardians/next of kin in accordance with the national legislation and institutional requirements.
Author contributions
Y–KH: Conceptualization, Funding acquisition, Methodology, Project administration, Resources, Supervision, Writing – original draft, Writing – review & editing. C–HLa: Data curation, Formal analysis, Investigation, Writing – original draft, Writing – review & editing. MW: Data curation, Formal analysis, Investigation, Writing – original draft, Writing – review & editing. T–YH: Funding acquisition, Methodology, Resources, Writing – review & editing. C–CK: Conceptualization, Funding acquisition, Project administration, Writing – review & editing. Y–HL: Investigation, Validation, Writing – review & editing. C–HLu: Investigation, Validation, Writing – review & editing.
Funding
The author(s) declare financial support was received for the research, authorship, and/or publication of this article. This research was funded by grants from the Chang Gung Memorial Hospital, Chiayi, Taiwan (Grant numbers: CMRPG6M0101–3, CMRPG6L0071 and CMRPG6J0403).
Acknowledgments
We thank Department of Laboratory Medicine from the Chang Gung Memorial Hospital, Chiayi, Taiwan. During the preparation of this work the authors used ChatGPT to improve language and readability. After using this tool/service, the authors reviewed and edited the content as needed and take full responsibility for the content of the publication.
Conflict of interest
The authors declare that the research was conducted in the absence of any commercial or financial relationships that could be construed as a potential conflict of interest.
Publisher’s note
All claims expressed in this article are solely those of the authors and do not necessarily represent those of their affiliated organizations, or those of the publisher, the editors and the reviewers. Any product that may be evaluated in this article, or claim that may be made by its manufacturer, is not guaranteed or endorsed by the publisher.
References
Atshan, S. S., Nor Shamsudin, M., Sekawi, Z., Lung, L. T. T., Hamat, R. A., Karunanidhi, A., et al. (2012). Prevalence of adhesion and regulation of biofilm–related genes in different clones of Staphylococcus aureus. J. Biomed. Biotechnol. 2012, 976972. doi: 10.1155/2012/976972
Balaban, N., Gov, Y., Bitler, A., Boelaert, J. R. (2003). Prevention of Staphylococcus aureus biofilm on dialysis catheters and adherence to human cells. Kidney Int. 63, 340–345. doi: 10.1046/j.1523–1755.2003.00733.x
Bibalan, M. H., Shakeri, F., Javid, N., Ghaemi, A., Ghaemi, E. A. (2014). Accessory gene regulator types of Staphylococcus aureus isolated in Gorgan, North of Iran. J. Clin. Diagn. Res. 8, DC07–DC09. doi: 10.7860/JCDR/2014/6971.4219
Christensen, G. D., Simpson, W. A., Younger, J. J., Baddour, L. M., Barrett, F. F., Melton, D. M., et al. (1985). Adherence of coagulase–negative staphylococci to plastic tissue culture plates: a quantitative model for the adherence of staphylococci to medical devices. J. Clin. Microbiol. 22, 996–1006. doi: 10.1128/jcm.22.6.996–1006.1985
Costerton, J. W., Stewart, P. S., Greenberg, E. P. (1999). Bacterial biofilms: a common cause of persistent infections. Science 284, 1318–1322. doi: 10.1126/science.284.5418.1318
Derakhshan, S., Navidinia, M., Haghi, F. (2021). ). Antibiotic susceptibility of human–associated Staphylococcus aureus and its relation to agr typing, virulence genes, and biofilm formation. BMC Infect. Dis. 21, 627. doi: 10.1186/s12879–021–06307–0
Ghasemian, A., Najar Peerayeh, S., Bakhshi, B., Mirzaee, M. (2015). The Microbial Surface Components Recognizing Adhesive Matrix Molecules (MSCRAMMs) genes among clinical isolates of Staphylococcus aureus from hospitalized children. Iran. J. Pathol. 10, 258–264. doi: 10.7508/ijp.2015.04.002
Hososaka, Y., Hanaki, H., Endo, H., Suzuki, Y., Nakae, T., Nagasawa, Z., et al. (2007). Characterization of oxacillin–susceptible mecA–positive Staphylococcus aureus: a new type of MRSA. J. Infect. Chemother. 13, 79–86. doi: 10.1007/s10156–006–0502–7
Idrees, M., Sawant, S., Karodia, N., Rahman, A. (2021). Staphylococcus aureus biofilm: Morphology, genetics, pathogenesis and treatment strategies. Int. J. Environ. Res. Public Health 18, 7602. doi: 10.3390/ijerph18147602
Javdan, S., Narimani, T., Shahini Shams Abadi, M., Gholipour, A. (2019). Agr typing of Staphylococcus aureus species isolated from clinical samples in training hospitals of Isfahan and Shahrekord. BMC Res. Notes 12, 363. doi: 10.1186/s13104–019–4396–8
Kadkhoda, H., Ghalavand, Z., Nikmanesh, B., Kodori, M., Houri, H., Taghizadeh Maleki, D., et al. (2020). Characterization of biofilm formation and virulence factors of Staphylococcus aureus isolates from paediatric patients in Tehran, Iran. Iran. J. Basic Med. Sci. 23, 691–698. doi: 10.22038/ijbms.2020.36299.8644
Kaźmierczak, N., Grygorcewicz, B., Piechowicz, L. (2021). Biofilm formation and prevalence of biofilm–related genes among clinical strains of multidrug–resistant Staphylococcus aureus. Microb. Drug Resist. 27, 956–964. doi: 10.1089/mdr.2020.0399
Kirmani, N. (1978). Staphylococcus aureus carriage rate of patients receiving long–term hemodialysis. Arch. Intern. Med. 138, 1657–1659. doi: 10.1001/archinte.138.11.1657
Koosha, R. Z., Fooladi, A. A. I., Hosseini, H. M., Aghdam, E. M. (2014). Prevalence of exfoliative toxin A and B genes in Staphylococcus aureus isolated from clinical specimens. J. Infect. Public Health 7, 177–185. doi: 10.1016/j.jiph.2013.11.003
Kostakioti, M., Hadjifrangiskou, M., Hultgren, S. J. (2013). Bacterial biofilms: development, dispersal, and therapeutic strategies in the dawn of the postantibiotic era. Cold Spring Harb. Perspect. Med. 3, a010306. doi: 10.1101/cshperspect.a010306
Kwiecinski, J. M., Jacobsson, G., Horswill, A. R., Josefsson, E., Jin, T. (2019). Biofilm formation by Staphylococcus aureus clinical isolates correlates with the infection type. Infect. Dis. (Lond.) 51, 446–451. doi: 10.1080/23744235.2019.1593499
LaFrance, J-P., Rahme, E., Lelorier, J., Iqbal, S. (2008). Vascular access–related infections: definitions, incidence rates, and risk factors. Am. J. Kidney Dis. 52, 982–993. doi: 10.1053/j.ajkd.2008.06.014
Lowy, F. D. (1998). Staphylococcus aureus infections. N. Engl. J. Med. 339, 520–532. doi: 10.1056/NEJM199808203390806
Marques, V. F., Motta, C. C., Soares, B. D., Melo, D. A., Coelho, S. M., Coelho, I. D., et al. (2017). Biofilm production and beta–lactamic resistance in Brazilian Staphylococcus aureus isolates from bovine mastitis. Braz. J. Microbiol. 48, 118–124. doi: 10.1016/j.bjm.2016.10.001
Maya, I. D., Allon, M. (2008). CORE CURRICULUM IN NEPHROLOGY–Vascular access: core curriculum 2008. Am. J. Kidney Dis. 51, 702–708. doi: 10.1053/j.ajkd.2007.10.046
Mcclure, J. A., Conly, J. M., Lau, V., Elsayed, S., Louie, T., Hutchins, W., et al. (2006). Novel multiplex PCR assay for detection of the staphylococcal virulence marker Panton–Valentine leukocidin genes and simultaneous discrimination of methicillin–susceptible from–resistant staphylococci. J. Clin. Microbiol. 44, 1141–1144. doi: 10.1128/jcm.44.3.1141–1144.2006
Mehrotra, M., Wang, G., Johnson, W. M. (2000). Multiplex PCR for detection of genes for Staphylococcus aureus enterotoxins, exfoliative toxins, toxic shock syndrome toxin 1, and methicillin resistance. J. Clin. Microbiol. 38, 1032–1035. doi: 10.1128/JCM.38.3.1032–1035.2000
Moormeier, D. E., Bayles, K. W. (2017). Staphylococcus aureus biofilm: a complex developmental organism. Mol. Microbiol. 104, 365–376. doi: 10.1111/mmi.13634
Pakbaz, Z., Sahraian, M. A., Sabzi, S., Mahmoodi, M., Pourmand, M. R. (2017). Prevalence of sea, seb, sec, sed, and tsst–1 genes of Staphylococcus aureus in nasal carriage and their association with multiple sclerosis. Germs 7, 171–177. doi: 10.18683/germs.2017.1123
Piraino, B. (2000). Staphylococcus aureus infections in dialysis patients: Focus on prevention. ASAIO J. 46, S13–S17. doi: 10.1097/00002480–200011000–00031
Pournajaf, A., Ardebili, A., Goudarzi, L., Khodabandeh, M., Narimani, T., Abbaszadeh, H. (2014). PCR–based identification of methicillin–resistant Staphylococcus aureus strains and their antibiotic resistance profiles. Asian Pac. J. Trop. Biomed. 4, S293–S297. doi: 10.12980/APJTB.4.2014C423
Rajpaul, K. (2015). Biofilm in wound care. Br. J. Community Nurs. Suppl. Wound Care S6 S8, S10–S11. doi: 10.12968/bjcn.2015.20.Sup3.S6
Ribeiro, M., Monteiro, F. J., Ferraz, M. P. (2012). Infection of orthopedic implants with emphasis on bacterial adhesion process and techniques used in studying bacterial–material interactions. Biomatter 2, 176–194. doi: 10.4161/biom.22905
Sockrider, M., Shanawani, H. (2017). What is hemodialysis? Am. J. Respir. Crit. Care Med. 195, P3–P4. doi: 10.1164/rccm.1952P3
Stepanović, S., Vuković, D., Dakić, I., Savić, B., Švabić–Vlahović, M. (2000). A modified microtiter–plate test for quantification of staphylococcal biofilm formation. J. Microbiol. Methods 40, 175–179. doi: 10.1016/s0167–7012(00)00122–6
Tan, S.Y-E., Chew, S. C., Tan, S.Y-Y., Givskov, M., Yang, L. (2014). Emerging frontiers in detection and control of bacterial biofilms. Curr. Opin. Biotechnol. 26, 1–6. doi: 10.1016/j.copbio.2013.08.002
Tan, L., Huang, Y., Shang, W., Yang, Y., Peng, H., Hu, Z., et al. (2022). Accessory gene regulator (agr) Allelic variants in cognate Staphylococcus aureus strain display similar phenotypes. Front. Microbiol. 13. doi: 10.3389/fmicb.2022.700894
Tan, L., Li, S. R., Jiang, B., Hu, X. M., Li, S. (2018). Therapeutic targeting of the staphylococcus aureus accessory gene regulator (agr) system. Front. Microbiol. 9. doi: 10.3389/fmicb.2018.00055
Taylor, T. A., Unakal, C. G. (2022). “Staphylococcus Aureus,” in StatPearls (Treasure Island: StatPearls Publishing).
Tristan, A., Ying, L., Bes, M., Etienne, J., Vandenesch, F., Lina, G. (2003). Use of multiplex PCR to identify Staphylococcus aureus adhesins involved in human hematogenous infections. J. Clin. Microbiol. 41, 4465–4467. doi: 10.1128/JCM.41.9.4465–4467.2003
Vandecasteele, S. J., Boelaert, J. R., De Vriese, A. S. (2009). Staphylococcus aureus infections in hemodialysis: what a nephrologist should know: What a nephrologist should know. Clin. J. Am. Soc. Nephrology: CJASN 4, 1388–1400. doi: 10.2215/CJN.01590309
Vasudevan, P., Nair, M. K. M., Annamalai, T., Venkitanarayanan, K. S. (2003). Phenotypic and genotypic characterization of bovine mastitis isolates of Staphylococcus aureus for biofilm formation. Vet. Microbiol. 92, 179–185. doi: 10.1016/s0378–1135(02)00360–7
Wang, X., Lin, D., Huang, Z., Zhang, J., Xie, W., Liu, P., et al. (2021). Clonality, virulence genes, and antibiotic resistance of Staphylococcus aureus isolated from blood in Shandong, China. BMC Microbiol. 21. doi: 10.1186/s12866–021–02344–6
Winstel, V., Schneewind, O., Missiakas, D. (2019). Staphylococcus aureus Exploits the Host Apoptotic Pathway To Persist during Infection. MBio 10. doi: 10.1128/mBio.02270–19
Xu, Y., Qian, S-Y., Yao, K-H., Dong, F., Song, W-Q., Sun, C., et al. (2021). Clinical and molecular characteristics of Staphylococcus aureus isolated from Chinese children: association among the agr groups and genotypes, virulence genes and disease types. World J. Pediatr. 17, 180–188. doi: 10.1007/s12519–021–00421–4
Keywords: vascular access infections (VAIs), Staphylococcus aureus, Agr typing, virulence genes, biofilm formation ability, molecular characterization
Citation: Lai C-H, Wong MY, Huang T-Y, Kao C-C, Lin Y-H, Lu C-H and Huang Y-K (2024) Exploration of agr types, virulence−associated genes, and biofilm formation ability in Staphylococcus aureus isolates from hemodialysis patients with vascular access infections. Front. Cell. Infect. Microbiol. 14:1367016. doi: 10.3389/fcimb.2024.1367016
Received: 08 January 2024; Accepted: 21 March 2024;
Published: 12 April 2024.
Edited by:
Reham Wasfi, MSA University, EgyptReviewed by:
Rasha El-Mahdy, Mansoura University, EgyptMadhavi Annamanedi, West Virginia University, United States
Copyright © 2024 Lai, Wong, Huang, Kao, Lin, Lu and Huang. This is an open-access article distributed under the terms of the Creative Commons Attribution License (CC BY). The use, distribution or reproduction in other forums is permitted, provided the original author(s) and the copyright owner(s) are credited and that the original publication in this journal is cited, in accordance with accepted academic practice. No use, distribution or reproduction is permitted which does not comply with these terms.
*Correspondence: Yao-Kuang Huang, aHVhbmcxMzdAaWNsb3VkLmNvbQ==
†These authors have contributed equally to this work and share first authorship