- 1Department of Stomatology, The Fourth Affiliated Hospital of Soochow University, Suzhou Dushu Lake Hospital, Medical Center of Soochow University, Suzhou, China
- 2Fujian Key Laboratory of Oral Diseases and Stomatological Key lab of Fujian College and University, School and Hospital of Stomatology, Fujian Medical University, Fuzhou, China
Background: Temporomandibular joint disorders (TMD) are highly prevalent among people. Numerous investigations have revealed the impact of gut microbiota in many diseases. However, the causal relationship between Temporomandibular joint disorders and gut microbiota remains unclear.
Methods: Genome-Wide Association Studies (GWAS) refer to the identification of sequence variations, namely single nucleotide polymorphisms (SNPs), existing across the entire human genome. GWAS data were collected on gut microbiota and TMD. Then, instrumental variables were screened through F-values and removal of linkage disequilibrium. These SNPs underwent mendelian analysis using five mathematical models. Sensitivity analysis was conducted to further verify the stability of the results. Pathogenic factors of TMD mediate the causal relationship between gut microbiota and TMD were explored through a two-step Mendelian randomization analysis. Finally, reverse mendelian analysis was conducted to account for potential reverse effects.
Results: The analysis of the data in this article suggests that some gut microbiota, including Coprobacter, Ruminococcus torques group, Catenibacterium, Lachnospiraceae, Turicibacter, Victivallis, MollicutesRF9, Methanobacteriales, Methanobacteriaceae, FamilyXI, Methanobacteria were identified as risk factors, while Peptococcaceae provides protection for TMD.
Conclusion: The research reveals the relation of gut microbiota in TMD. These findings provide insights into the underlying mechanisms and suggest potential therapeutic strategy.
1 Introduction
Temporomandibular joint disorders (TMD) are a collective term for a group of conditions affecting the temporomandibular joint and/or surrounding muscles, characterized by pain and dysfunction (Scrivani et al., 2008; Andre et al., 2022; Thomas et al., 2023). TMD are highly prevalent among people, with children and adolescents also being susceptible to the condition (Kopp et al., 2023). Approximately 5% to 12% of Americans are affected by TMD, with annual total costs for treating TMD reaching around $4 billion (Schiffman et al., 2014; Manrriquez et al., 2021). Women have a higher risk of developing TMD, with prevalence rates ranging from 25% to 40% in population (Murphy et al., 2013; Calixtre et al., 2014; Valesan et al., 2021). Nearly half of individuals diagnosed with TMD experience persistent or recurring symptoms, often leading to a diminished quality of life (List and Axelsson, 2010; Maixner et al., 2011). Treating TMD poses many challenges. Comorbidities are highly prevalent among TMD patients, including headaches, widespread pain, fibromyalgia, neck and back pain, and psychosocial disorders like stress, anxiety, depression (Aggarwal et al., 2006). Prospective and risk assessment studies on oral and facial pain support the biological psychological social model of TMD, emphasizing the role of comprehensive treatment (Fillingim et al., 2018). Traditionally, treatment methods for TMD include cognitive–behavioral therapy, anti-inflammatory drug therapy, splint therapy, minimally invasive, arthroscopic, or open surgery (Manrriquez et al., 2021; Valesan et al., 2021). Despite the availability of numerous treatments for TMD, they often fail to prevent the occurrence or recurrence of the condition. The etiological factors of TMD are still unclear.
The human gut harbors thousands of microbial species, forming a complex ecological community, called the gut microbiome (Lagier and Raoult, 2016). These microorganisms are major mediators of body homeostasis, influencing various physiological activities such as metabolism, barrier homeostasis, inflammation, and hematopoiesis through intestinal and parenteral actions. Recently, the gut microbiota has recently been classified as a “vital organ” due to its establishment of multidirectional and communicative links or axes with other organs through neural, endocrine, humoral, immune, and metabolic pathways. Changes in the microbiome can lead to gut-related problems, but also affects other organ-related diseases, although the actual mechanisms of gut-organ interaction are not fully understood. A recent study had revealed a correlation between the occurrence of TMD and the deregulation of microbial metabolites (Ma et al., 2020). Extensive research on the brain-gut axis underscores the pivotal role of gut microbiota in adjusting the secretion of inflammatory neurotransmitters, which could lead to the release of diverse proinflammatory mediators in the process of TMD (Shrivastava et al., 2021; Aburto and Cryan, 2024).
Widespread debate still exists regarding whether there is a causal relationship between abnormal microbial communities associated with diseases. The substitution of a nucleotide in the genome can significantly alter the function of an organism. Single nucleotide polymorphisms (SNPs) are commonly present in microorganisms and can endow bacteria with antibiotic resistance or the ability to infect new host species. The diversity of SNPs may reflect the correlation between host microbial interactions. Current research lacks comprehensive evidence to confirm between gut bacteria and TMD. Mendelian randomization (MR) is a crucial instrument for exploring the potential causal relationship between exposure and outcomes using instrumental variables (IV) (Davey and Hemani, 2014; Swerdlow et al., 2016; Bowden and Holmes, 2019). This approach is based on the fair and random allocation of alleles to the next generation, and has emerged as a powerful tool for exploring the intricate connections between complex traits and diseases (Bowden and Holmes, 2019). MR is currently widely used in oncology, neuroscience, cardiovascular and genetics. Its advantage in exploring causal relationships makes it have broad prospects (Swerdlow et al., 2016; Bowden and Holmes, 2019; Ouyang et al., 2022). With the continuous advancement of technology and the ongoing refinement of research methods, such as Genome-wide association studies, MR becomes an important method in the biomedical fields. In this research, we aimed to explore the causality between the intestinal microbiome and TMD by Mendelian randomization.
2 Methods
2.1 Data sources
A summary of the GWAS data was originated from MiBioGen (https://mibiogen.gcc.rug.nl/), which comprised 16S rRNA sequencing and genotyped their participants with full-genome SNP arrays. The sequencing of gut microbiota in the Mibiogen database offered a comprehensive catalog of gene variations associated with gut microbiota (Kurilshikov et al., 2021). These variations were derived from 18,340 participants representing 24 countries, individuals from Asian, American, and African populations. Currently, this database is recognized as the most extensive and comprehensive among comparable database. The database comprises an analysis of the variable regions V3, V4-V1, and V2-V16 of the 16S rRNA gene from microorganisms. The results are categorized into five levels: genus, family, order, class, and phyla, encompassing a total of 211 species. Our outcomes were sourced from the IEU Open GWAS database (https://gwas.mrcieu.ac.uk/datasets), a comprehensive database (Zheng et al., 2022b). This dataset includes a European population with a comprehensive sample size of 134,280 individuals, including 2,730 diagnosed with TMD and 131,550 control individuals, providing a total of 16,379,953 SNPs. All data in this study were derived from public publications, thus ethical approval or patient consent is not required for analysis.
2.2 Experimental design
GWAS refer to the identification of sequence variations, namely SNPs, existing across the entire human genome. In order to investigate the causal connection between gut microbiota and TMD, we conducted a bidirectional dual-sample MR study utilizing this GWAS dataset (Figure 1). Individuals were grouped into different genotype groups based on SNPs strongly associated with TMD. By simulating random distribution of genetic information from parental genomes to offspring, occurrence rates of TMD among different genotype groups were compared. The IVs need to fit three main assumptions (1): SNPs are closely related to the exposure (2); SNPs are unrelated to the outcome (3); SNPs are unrelated to confounding factors. For exposure SNPs, the pooling process is conducted according to SNP loci with P < 1 × 10-5, while ensuring R2 < 0.001 and genetic distance of 10000 to eliminate linkage disequilibrium (LD). After eliminating the SNPs of echo sequence, the remaining SNPs were chosen. This step ensured that the IVs fits assumption. Weak instrumental variables are genetic variations with lower explanatory power for exposure. They are associated with exposure, but the strength of this association is limited (Davey and Hemani, 2014). According to literature reports, F > 10 was considered to be the standard for excluding weak instrumental variable bias (Burgess and Thompson, 2011; Zheng et al., 2022a). Next, the screened SNPs were used as IVs to evaluate the relationship between gut microbiota and TMD.
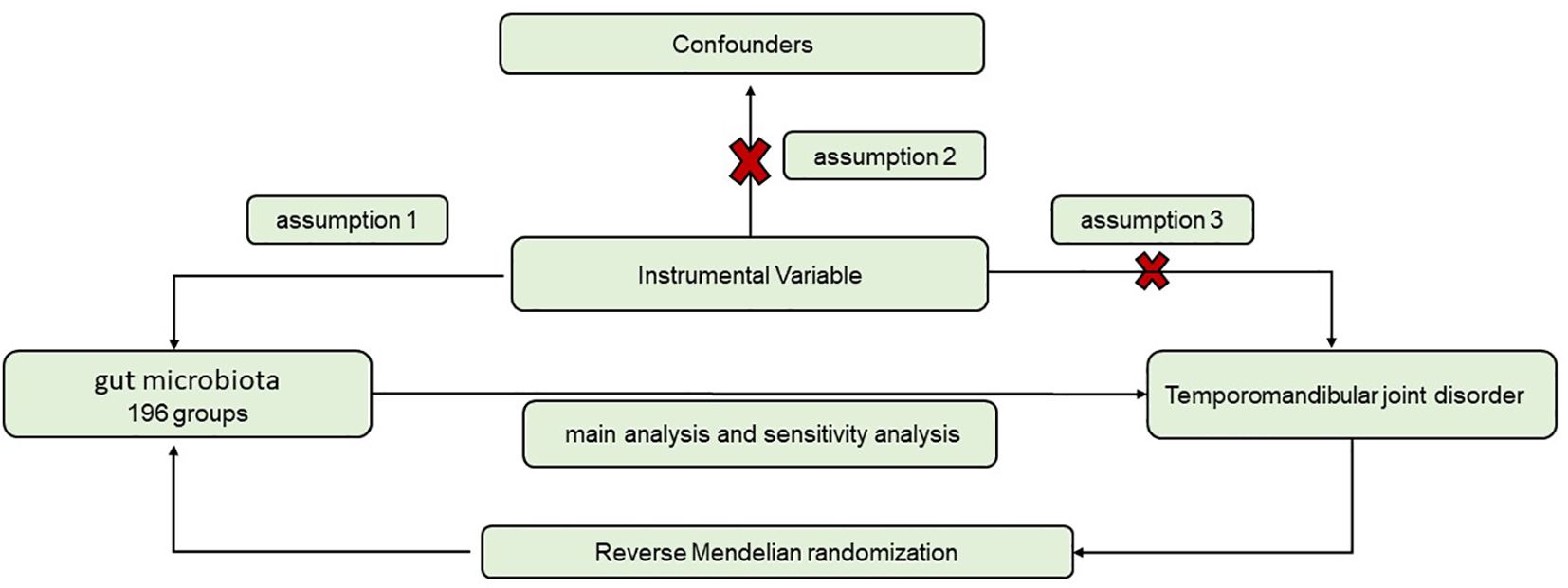
Figure 1. Flowchart for the study of the association between gut microbiota and TMD. (1) SNPs are closely related to the exposure; (2) SNPs are unrelated to the outcome; (3) SNPs are unrelated to confounding factors.
2.3 Statistical analysis
In this investigation, diverse statistical approaches were utilized to assess the causal link between gut microbiota and TMD. These methods encompassed inverse variance weighted (IVW), simple mode, MR- Egger regression, weighted median (WM), and weighted model (WME) (Hemani et al., 2018). The characteristic of IVW method is that it does not consider the presence of intercept terms during regression, and it uses the inverse of the outcome variance (the square of the standard error) as weights for fitting. IVW was seemed as the primary method, and the other methods as Supplementary Methods to evaluate the relationship between exposure and outcomes under various conditions (Carter et al., 2021). If P < 0.05, the outcome was deemed statistically significant and expressed in terms of odds ratio (OR) along with the corresponding 95% confidence interval (95% CI).
2.4 Sensitivity analysis
Sensitivity analysis was performed to validate the stability of this findings and to assess potential biases and heterogeneity in each IVs. Egger regression analysis is a type of weighted linear regression where the standard normal deviation of the effect value serves as the dependent variable, while its accuracy serves as the independent variable. The MR-Egger method was employed to assess the presence of horizontal pleiotropy. Simultaneously, the Cochran Q test was applied to investigate potential heterogeneity, with significance set at P<0.05. And leave-one-out analysis was conducted, systematically removing each exposure to evaluate results stability. Additionally, the MR-PRESSO test was used to identify outliers and recompute the results. The analyses were performed by R version 4.2.3, with the “TwoSampleMR” and “MRPRESSO” software packages (Burgess and Thompson, 2015).
2.5 Two-step mendelian randomization
The overall impact of exposure on the outcome can be decomposed into direct effects and synergistic effects. In order to detect the synergistic effect on TMD, two-step MR was used to examine the mediating effects of other factors (Carter et al., 2021; Zhao et al., 2022). Dentofacial anomalies was considered as TMD influencing factors, serving as an intermediary factor of intestinal flora affecting TMD. Maxillofacial deformities underwent univariate Mendelian randomization, and significant intestinal flora results were tested using the coefficient product method.
2.6 Reverse Mendelian randomization
Reverse Mendelian randomization also was conducted to assess the stability of results.
3 Results
3.1 The screen of IVs
15 groups of unknown data from the gut microbiota were excluded, while 196 data were entered statistical analysis. By computing P values, LD, and conducting F-value tests, we conducted screening on multiple SNPs within 196 genotypes. The SNPs that passed the screening were considered to eliminate instrumental bias and F>10 (Supplementary Table 1). All SNPs which were screened would seem as instrumental variables for the subsequent experiments.
3.2 Effect of IV in TMD
2601 SNPs were screened from 196 microbial samples. 11 microbial group that showed significant effect on the occurrence of TMD, and the IVW outcomes consistently demonstrated significant effects. The IVW results indicated that the following microbial groups were associated with an increased risk of TMD: genus Coprobacter (OR = 1.28, 95% CI (1.04-1.59), P = 0.035), genus Ruminococcus torques group (OR = 1.49, 95% CI (1.04-2.15), P = 0.031), genus Catenibacterium (OR = 1.28, 95% CI (1.04-1.59), P = 0.023), genus LachnospiraceaeUCG010 (OR = 1.39, 95% CI (1.02-1.90), P = 0.035), genus Turicibacter (OR = 1.42, 95% CI (1.12-1.80), P = 0.004), genus Victivallis (OR = 1.20, 95% CI (1.02-1.40), P = 0.016), order MollicutesRF9 (OR = 1.31, 95% CI (1.31-1.67), P = 0.026), order Methanobacteriales (OR = 1.25, 95% CI (1.05-1.49), P = 0.014), family Methanobacteriaceae (OR = 1.25, 95% CI (1.05-1.49), P = 0.014), family FamilyXI (OR = 1.24, 95% CI (1.06-1.46), P = 0.008), class Methanobacteria (OR = 1.25, 95% CI (1.05-1.49), P = 0.014). And one microbial group was demonstrated a significant effect prevent the progression of TMD: family Peptococcaceae (OR = 0.75, 95% CI (0.58-0.98), P = 0.036). (Figure 2; Supplementary Table 2).
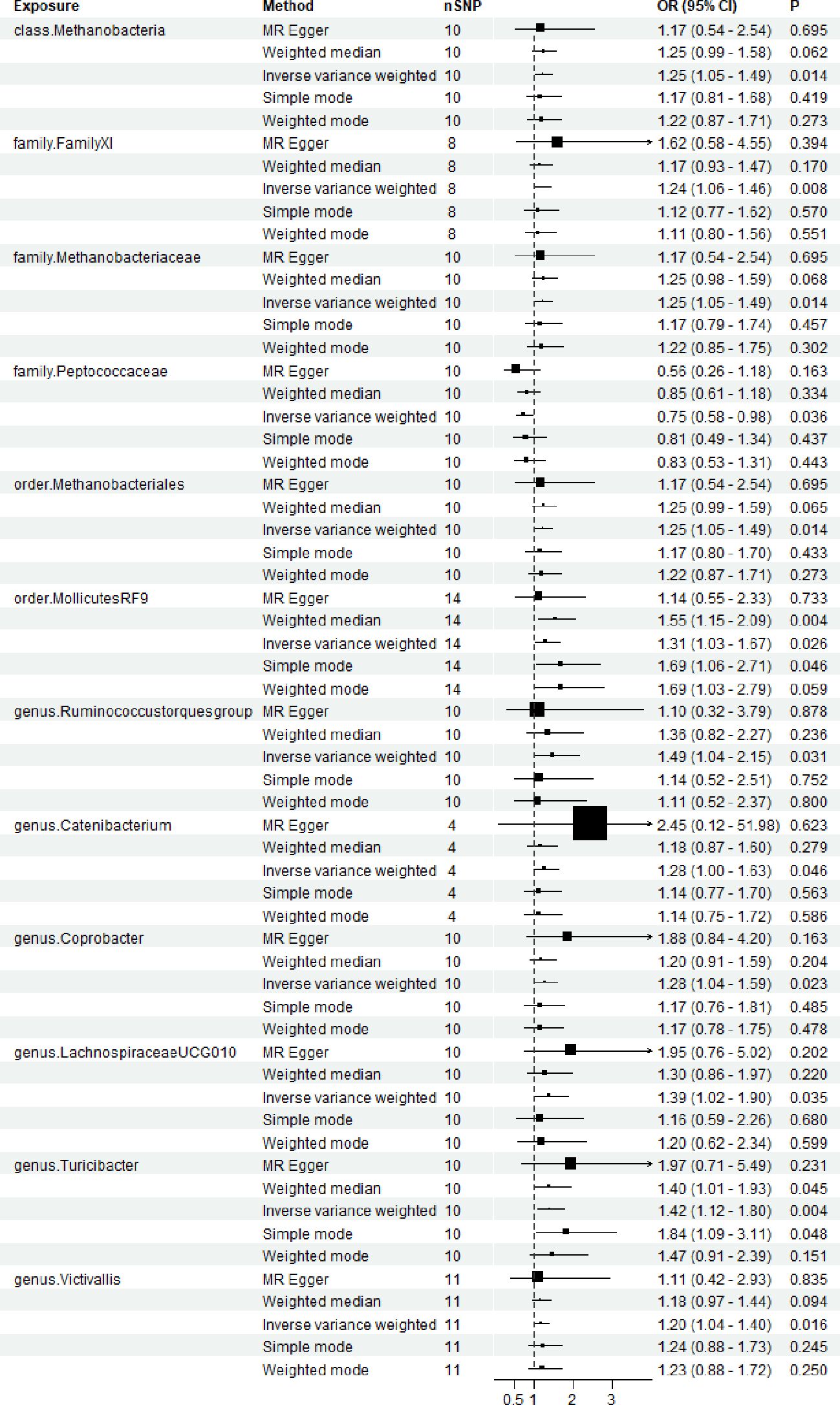
Figure 2. Forest plots for the association of gut microbiota and Temporomandibular joint disorder. OR, odds ratio; CI, confidence interval. P < 0.05.
3.3 Sensitivity analyses
According to the results of the Cochran Q test, including both the IVW and Egger methods, P values were not significant. Therefore, the results seemed to be stable, indicating no apparent heterogeneity. Additionally, tests for Leave-one-out demonstrated stable conclusions (Figure 3). MR-Egger regression testing further confirmed the stability of the results (Figure 4; Supplementary Table 3).
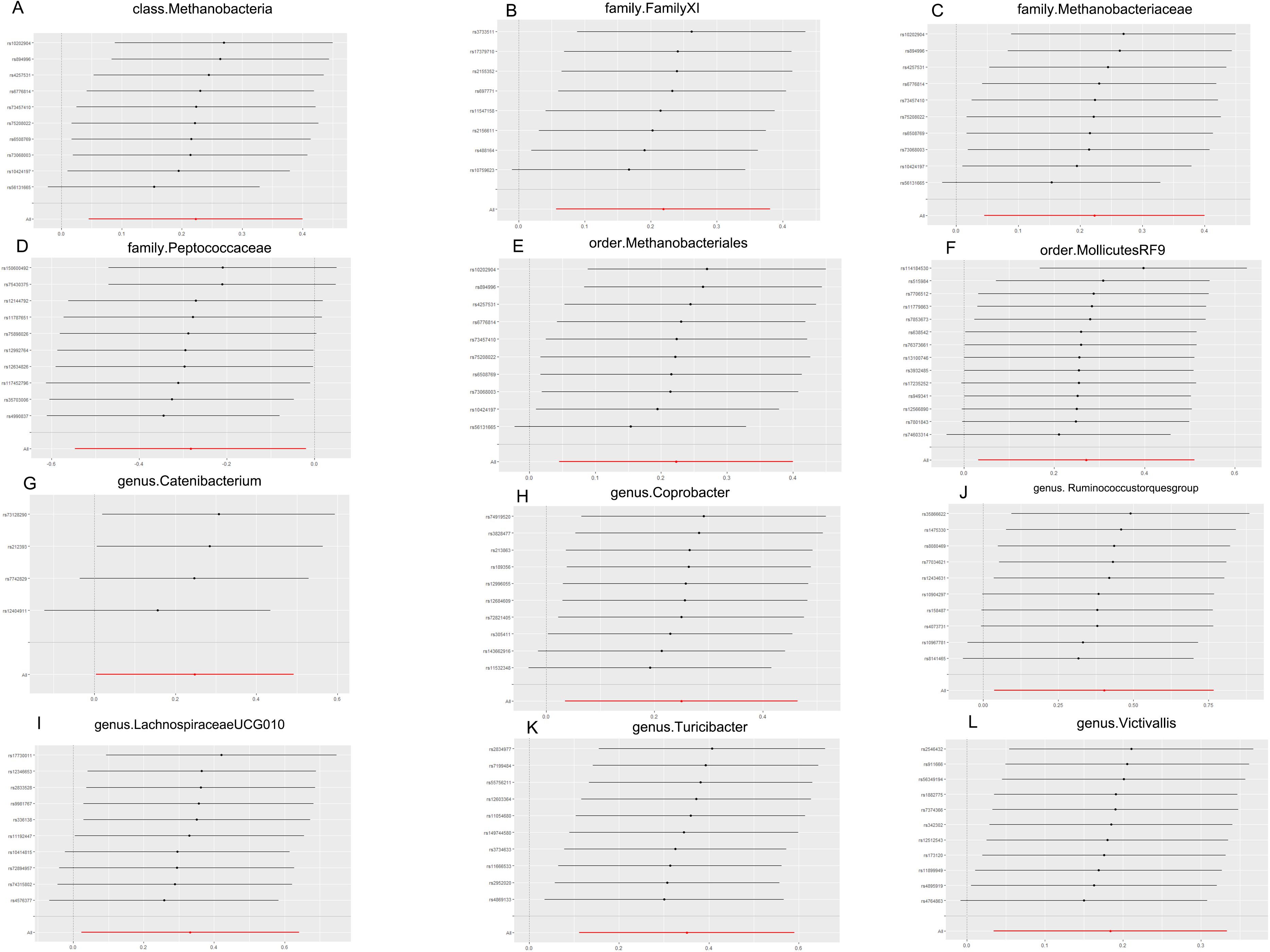
Figure 3. MR leave-one-out sensitivity analysis. (A) Leave-one-out sensitivity analysis of the effect of class. Methanobacteria on TMD; (B) Leave-one-out sensitivity analysis of the effect of family. FamilyXI on TMD; (C) Leave-one-out sensitivity analysis of the effect of family. Methanobacteriaceae on TMD; (D) Leave-one-out sensitivity analysis of the effect of family. Peptococcaceae on TMD; (E) Leave-one-out sensitivity analysis of the effect of order. Methanobacteriales on TMD; (F) Leave-one-out sensitivity analysis of the effect of order.MollicutesRF9 on TMD; (G) Leave-one-out sensitivity analysis of the effect of genus. Catenibacterium on TMD; (H) Leave-one-out sensitivity analysis of the effect of genus. Coprobacter on TMD; (I) Leave-one-out sensitivity analysis of the effect of genus.Lachnospiraceaeucg010 on TMD; (J) Leave-one-out sensitivity analysis of the effect of genus. Ruminococcus torques group on TMD; (K) Leave-one-out sensitivity analysis of the effect of genus. Turicibacter on TMD; (L) Leave-one-out sensitivity analysis of the effect of genus. Victivallis on TMD. TMD, Temporomandibular joint disorder; MR, Mendelian randomization.
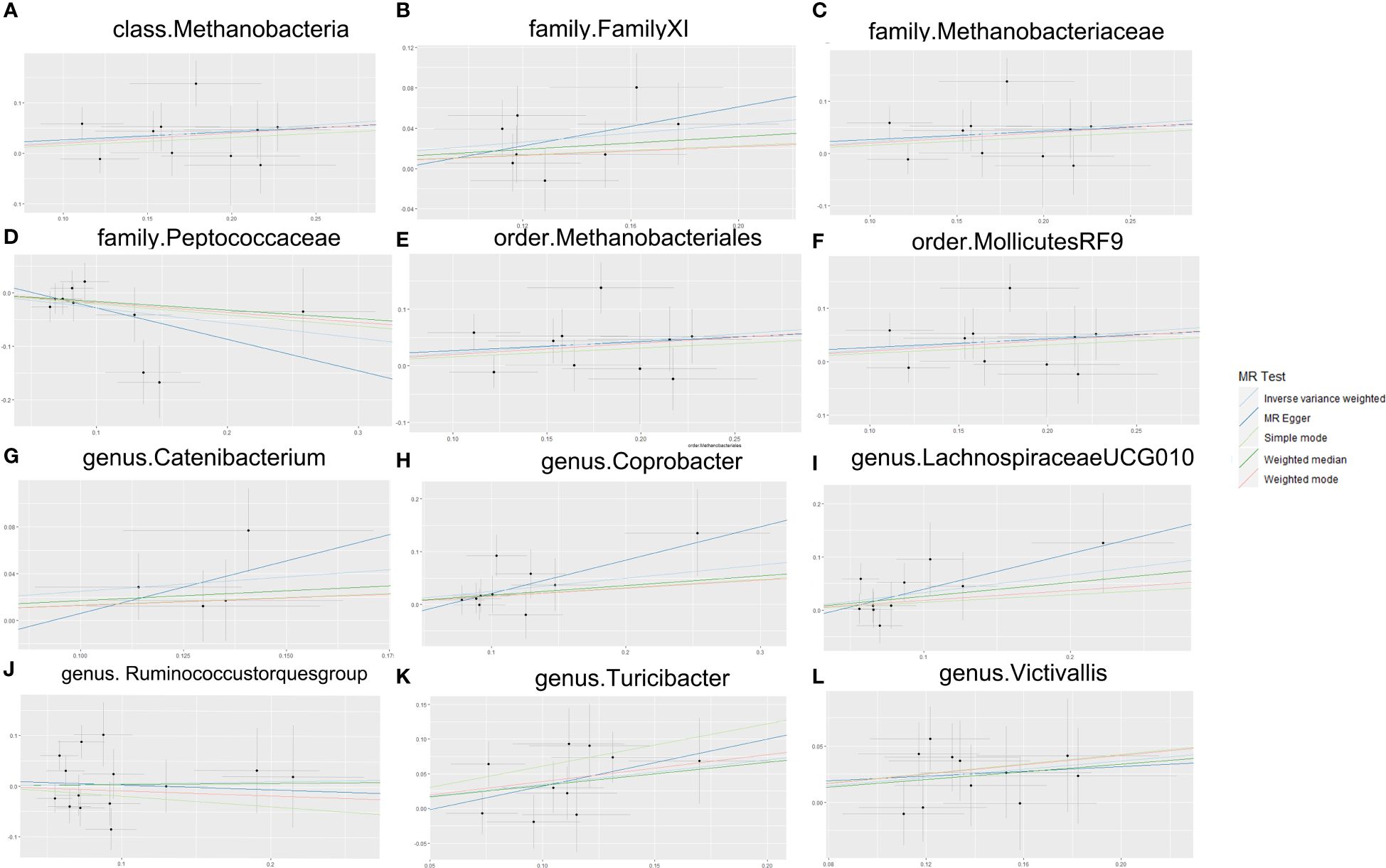
Figure 4. MR Egger sensitivity analysis. (A) Egger sensitivity analysis of the effect of class.Methanobacteria on TMD; (B) Egger sensitivity analysis of the effect of family.FamilyXI on TMD; (C) Egger sensitivity analysis of the effect of family. Methanobacteriaceae on TMD; (D) Egger sensitivity analysis of the effect of family. Peptococcaceae on TMD; (E) Egger sensitivity analysis of the effect of order. Methanobacteriales on TMD; (F) Egger sensitivity analysis of the effect of order.MollicutesRF9 on TMD; (G) Egger sensitivity analysis of the effect of genus. Catenibacterium on TMD; (H) Egger sensitivity analysis of the effect of genus. Coprobacter on TMD; (I) Egger sensitivity analysis of the effect of genus.Lachnospiraceaeucg010 on TMD; (J) Egger sensitivity analysis of the effect of genus. Ruminococcus torques group on TMD; (K) Egger sensitivity analysis of the effect of genus. Turicibacter on TMD; (L) Egger sensitivity analysis of the effect of genus. Victivallis on TMD. TMD, Temporomandibular joint disorder; MR, Mendelian randomization.
3.4 Two-step mendelian randomization
Malocclusion exhibited significant relationships with TMD (Supplementary Figure 3). The results suggest that that the causal relationship between the three bacteria (Turicibacter, Victivallis, Peptococcaceae) (Supplementary Figure 4) and TMD may be partly mediated by maxillofacial deformities.
3.5 Reverse MR analysis
In order to test potential reverse causality influencing the previous results, reverse MR analysis was performed to treating significant gut microbiota as the outcome and TMD as the exposure variables. Notably, the analysis of the data did not yield any evidence supporting a reverse causal association between TMD and the identified gut microbiome (Supplementary Figure 5).
4 Discussion
According to recent epidemiological, cellular biology, and genomics research, a significant portion of the external influences on the human body appears to mediated by the gut microbiota. The gut microbiota, being the largest microbial community, exerts a substantial influence on training host immunity, regulating intestinal endocrine function, promoting neurotransmitter release, modulating drug action and metabolism, eliminating toxins, and producing numerous compounds that affect the host. Extensive debate exists regarding whether abnormal microbial communities associated with diseases are causally related to the diseases (i.e., susceptibility, initiation, or progression) (Davies et al., 2018; Fan and Pedersen, 2021). An important finding is that the abundance of Bacteroidetes and Lachnospiraceae in the gut of TMJ mice significantly decreased (Ma et al., 2020). In another study, 16S rRNA sequencing of TMJ mice revealed significant changes in Bacteroidetes, TM7, Actinobacteria, Tenericutes, Verrucomicrobia, Cyanobacteria, Spirochaetes, and Elusimicrobia (Shen et al., 2023). Additionally, arthritis patients displayed an enrichment of genera such as Anaerostipes, Bifidobacterium, Brachyspira, and Eggerthella, while healthy controls had higher levels of genera such as Faecalibacterium, Lachnoclostridium, Phascolarctobacterium, and Paraprevotella (Jeyaraman et al., 2023). Using IVW estimation, a statistical method that uses the reciprocal of the variance of the results (square of standard error) as a weight for fitting is used to evaluate the causal impact of 211 gut microbiota on TMD. The analysis of the data in this article suggests that the abundance of 12 bacteria were found to have causal relationships with the progression of TMD. Among them, 11 species of bacteria were found to exacerbate TMD, while Peptococcaceae seemed to had a protective effect. In subsequent stability tests, removing SNP loci that influenced the results did not alter the stability of the outcomes. Therefore, the relationship of the above microbial communities on TMD to be stable and reliable. Reverse MR revealed no evidence of causal effect of TMD on the on the identified gut microbiome.
The relationship between gut microbiota and TMD may be based on the susceptibility to TMD caused by dysbiosis of gut microbiota, or the microbiota and its metabolites acting as promoting factors for TMD. Increasing evidence suggested an association between the family Lachnospiraceae and the genus Ruminococcus with inflammatory diseases. Ruminococcus is a Gram-positive anaerobic bacterium belonging to the phylum Firmicutes, and it is one of the 57 species present in 90% of individuals at a median abundance of around 0.1% (Aguilera et al., 2012; Crost et al., 2023). An increase in the abundance of Ruminococcus has been observed in patients with ankylosing spondylitis (Vereecke and Elewaut, 2017). Additionally, a correlation exists between the prevalence of musculoskeletal pain (MSKP) in elderly community members and Ruminococcus (Shmagel et al., 2018).
Costello et al. observed alterations in the composition of gut microbiota from patients with ankylosing spondylitis (AS), where the increased abundance of bacteria from the Lachnospiraceae, Ruminococceae, Rikenellaceae, Porphyromonadae, and Bacteroideae appeared at AS (Costello et al., 2015). This study similarly suggested that Lachnospiraceae ucg-010 and the Ruminococcus may play a promoting role in TMD.
In this study, Peptococcaceae may have a mitigating or protective effect on the occurrence of TMD. This effect may be associated with their impact on metabolic products. Wen et al. confirmed the correlation of Peptococcaceae with various metabolic pathways, including tryptophan and tyrosine, derived from metabolomic and 16S rRNA gene sequencing analyses (Wen et al., 2019).
The analysis of the data in this article also provides some new insights into the effects of drugs in TMD treatment. TMD treatment targets both pain relief and functional improvement. The reports described that a large number of TMD patients had used medication, including anti-inflammatory drugs, over-the-counter painkillers, and even antidepressants, anti-anxiety drugs, and muscle relaxants (Gauer and Semidey, 2015). Although the standard of drug treatment for TMD still lacks evidence-based support, nonsteroidal anti-inflammatory drugs are considered the first choice, and other drugs mentioned above have also been used in combination (Ta and Dionne, 2004). The differences in the abundance of intestinal microorganisms among different populations may lead to different responses to TMD drug treatment. Lachnospiracea, a diverse obligate anaerobic bacteria, is abundant in the human gut. In rats administered with low-dose aspirin, alterations in the Lachnospiracea family and its interactions with the Ruminococcaceae family were observed (Chi et al., 2021). When using medication to treat TMD, changes in the aforementioned bacteria may be potential evaluation indication. Some microbial communities may synergize with malocclusion. Malocclusion is an important cause of TMD, resulting from multiple factors such as genes, occlusal training, and nutritional factors that affect growth and development (Masucci et al., 2020). The synthesis of butyrate by human gut microbiota has significantly improve skeletal muscle mass (Lv et al., 2021). Skeletal muscles, especially chewing muscles and some head and neck muscle groups, have a promoting or inhibiting effect on the development of maxillofacial bones, which may lead to excessive or insufficient development of the jawbone, further leading to malocclusion (Rot et al., 2014). Peptococcaceae has been demonstrated to enhance muscle strength through the synthesis of butyric acid, and occlusal muscle strength is an important factor affecting maxillofacial development (Joshi et al., 2014; Parada et al., 2019; Leszczyszyn et al., 2021). The increase in the abundance of Turicibacter may reduce the content of bile acids such as lithocholic acid, which in turn leads to a decrease in the intestinal absorption of vitamin D (Guida et al., 2020; Hao et al., 2022). Skeletal development is another factor that affects malocclusion. This article suggests that malocclusion played a partial mediating role in the effects of Turcicactor, Victivalis, and Peptococcaceae on TMD. Regulating the gut microbiota is expected to become a part of the precise therapy of TMD, relying on reducing the inflammatory response around the joints, and providing a suitable microenvironment for the regeneration of surrounding chondrocytes.
This study focuses on exploring the causal relationship between gut microbiota and TMD without delving into the specific regulatory mechanisms. Given the complex interplay among gut microbiota and the broad spectrum of pathogenic factors associated with TMD, there may be intricate cross-interactions among these factors. This underscores the necessity for further comprehensive research to achieve precision in treating TMD. Additionally, the study relies on GWAS data grouped based on epidemiological surveys rather than specific TMD pathology group, which hinders further research on the pathogenic factors of different pathological states of TMD. Therefore, the interpretation of the result still needs to be cautious. Moreover, given that the majority of participant are European descent, extending these research conclusions to other populations requires broader data support.
5 Conclusions
In conclusion, this study represents a view in understanding the connections between temporomandibular joint disorders and gut microbiota. These identified microbial signatures associated with TMD contribute to reveal the mechanistic and explore novel therapeutic avenues for individuals precise therapy.
Data availability statement
Publicly available datasets were analyzed in this study. This data can be found here: https://mibiogen.gcc.rug.nl/; https://gwas.mrcieu.ac.uk/datasets/finn-b-TEMPOROMANDIB/.
Author contributions
KZ: Writing – original draft, Writing – review & editing, Conceptualization, Data curation, Formal analysis. SJ: Writing – original draft, Writing – review & editing. HJ: Writing – original draft, Writing – review & editing. YQ: Writing – original draft, Writing – review & editing, Data curation, Formal analysis. WZ: Conceptualization, Data curation, Methodology, Writing – original draft, Writing – review & editing.
Funding
The author(s) declare that no financial support was received for the research, authorship, and/or publication of this article.
Conflict of interest
The authors declare that the research was conducted in the absence of any commercial or financial relationships that could be construed as a potential conflict of interest.
Publisher’s note
All claims expressed in this article are solely those of the authors and do not necessarily represent those of their affiliated organizations, or those of the publisher, the editors and the reviewers. Any product that may be evaluated in this article, or claim that may be made by its manufacturer, is not guaranteed or endorsed by the publisher.
Supplementary material
The Supplementary Material for this article can be found online at: https://www.frontiersin.org/articles/10.3389/fcimb.2024.1361373/full#supplementary-material
References
Aburto, M. R., Cryan, J. F. (2024). Gastrointestinal and brain barriers: unlocking gates of communication across the microbiota-gut-brain axis. Nat. Rev. Gastroenterol. Hepatol. 21, 222–247. doi: 10.1038/s41575-023-00890-0
Aggarwal, V. R., Mcbeth, J., Zakrzewska, J. M., Lunt, M., Macfarlane, G. J. (2006). The epidemiology of chronic syndromes that are frequently unexplained: do they have common associated factors? Int. J. Epidemiol. 35, 468–476. doi: 10.1093/ije/dyi265
Aguilera, M., Rakotoarivonina, H., Brutus, A., Giardina, T., Simon, G., Fons, M. (2012). Aga1, the first alpha-galactosidase from the human bacteria ruminococcus gnavus e1, efficiently transcribed in gut conditions. Res. Microbiol. 163, 14–21. doi: 10.1016/j.resmic.2011.10.005
Andre, A., Kang, J., Dym, H. (2022). Pharmacologic treatment for temporomandibular and temporomandibular joint disorders. Oral. Maxillofac. Surg. Clin. North Am. 34, 49–59. doi: 10.1016/j.coms.2021.08.001
Bowden, J., Holmes, M. V. (2019). Meta-analysis and mendelian randomization: a review. Res. Synth Methods 10, 486–496. doi: 10.1002/jrsm.1346
Burgess, S., Thompson, S. G. (2011). Avoiding bias from weak instruments in mendelian randomization studies. Int. J. Epidemiol. 40, 755–764. doi: 10.1093/ije/dyr036
Burgess, S., Thompson, S. G. (2015). Multivariable mendelian randomization: the use of pleiotropic genetic variants to estimate causal effects. Am. J. Epidemiol. 181, 251–260. doi: 10.1093/aje/kwu283
Calixtre, L. B., Gruninger, B. L., Chaves, T. C., Oliveira, A. B. (2014). Is there an association between anxiety/depression and temporomandibular disorders in college students? J. Appl. Oral. Sci. 22, 15–21. doi: 10.1590/1678-775720130054
Carter, A. R., Sanderson, E., Hammerton, G., Richmond, R. C., Davey, S. G., Heron, J., et al. (2021). Mendelian randomisation for mediation analysis: current methods and challenges for implementation. Eur. J. Epidemiol. 36, 465–478. doi: 10.1007/s10654-021-00757-1
Chi, T., Zhao, Q., Wang, P. (2021). Fecal 16s rrna gene sequencing analysis of changes in the gut microbiota of rats with low-dose aspirin-related intestinal injury. BioMed. Res. Int. 2021, 8848686. doi: 10.1155/2021/8848686
Costello, M. E., Ciccia, F., Willner, D., Warrington, N., Robinson, P. C., Gardiner, B., et al. (2015). Brief report: intestinal dysbiosis in ankylosing spondylitis. Arthritis Rheumatol. 67, 686–691. doi: 10.1002/art.38967
Crost, E. H., Coletto, E., Bell, A., Juge, N. (2023). Ruminococcus gnavus: friend or foe for human health. FEMS Microbiol. Rev. 47, 14. doi: 10.1093/femsre/fuad014
Davey, S. G., Hemani, G. (2014). Mendelian randomization: genetic anchors for causal inference in epidemiological studies. Hum. Mol. Genet. 23, R89–R98. doi: 10.1093/hmg/ddu328
Davies, N. M., Holmes, M. V., Davey, S. G. (2018). Reading mendelian randomisation studies: a guide, glossary, and checklist for clinicians. BMJ 362, k601. doi: 10.1136/bmj.k601
Fan, Y., Pedersen, O. (2021). Gut microbiota in human metabolic health and disease. Nat. Rev. Microbiol. 19, 55–71. doi: 10.1038/s41579-020-0433-9
Fillingim, R. B., Slade, G. D., Greenspan, J. D., Dubner, R., Maixner, W., Bair, E., et al. (2018). Long-term changes in biopsychosocial characteristics related to temporomandibular disorder: findings from the oppera study. Pain. 159, 2403–2413. doi: 10.1097/j.pain.0000000000001348
Gauer, R. L., Semidey, M. J. (2015). Diagnosis and treatment of temporomandibular disorders. Am. Fam Physician 91, 378–386.
Guida, F., Boccella, S., Belardo, C., Iannotta, M., Piscitelli, F., De Filippis, F., et al. (2020). Altered gut microbiota and endocannabinoid system tone in vitamin d deficiency-mediated chronic pain. Brain Behav. Immun. 85, 128–141. doi: 10.1016/j.bbi.2019.04.006
Hao, X., Zhang, J., Shang, X., Sun, K., Zhou, J., Liu, J., et al. (2022). Exercise modifies the disease-relevant gut microbial shifts in post-traumatic osteoarthritis rats. Bone Joint Res. 11, 214–225. doi: 10.1302/2046-3758.114.BJR-2021-0192.R1
Hemani, G., Zheng, J., Elsworth, B., Wade, K. H., Haberland, V., Baird, D., et al. (2018). The mr-base platform supports systematic causal inference across the human phenome. Elife. 7, e34408. doi: 10.7554/eLife.34408
Jeyaraman, M., Ram, P. R., Jeyaraman, N., Yadav, S. (2023). The gut-joint axis in osteoarthritis. Cureus. 15, e48951. doi: 10.7759/cureus.48951
Joshi, N., Hamdan, A. M., Fakhouri, W. D. (2014). Skeletal malocclusion: a developmental disorder with a life-long morbidity. J. Clin. Med. Res. 6, 399–408. doi: 10.14740/jocmr1905w
Kopp, M., Wiesmueller, M., Buchbender, M., Kesting, M., Nagel, A. M., May, M. S., et al. (2023). MRI of temporomandibular joint disorders: a comparative study of 0.55 t and 1.5 t MRI. Invest. Radiol. 59, 223–229. doi: 10.1097/RLI.0000000000001008
Kurilshikov, A., Medina-Gomez, C., Bacigalupe, R., Radjabzadeh, D., Wang, J., Demirkan, A., et al. (2021). Large-scale association analyses identify host factors influencing human gut microbiome composition. Nat. Genet. 53, 156–165. doi: 10.1038/s41588-020-00763-1
Lagier, J. C., Raoult, D. (2016). Fecal microbiota transplantation: indications and perspectives. Med. Sci. (Paris). 32, 991–997. doi: 10.1051/medsci/20163211015
Leszczyszyn, A., Hnitecka, S., Dominiak, M. (2021). Could vitamin d3 deficiency influence malocclusion development? Nutrients. 13, 2122. doi: 10.3390/nu13062122
List, T., Axelsson, S. (2010). Management of tmd: evidence from systematic reviews and meta-analyses. J. Oral. Rehabil. 37, 430–451. doi: 10.1111/jor.2010.37.issue-6
Lv, W. Q., Lin, X., Shen, H., Liu, H. M., Qiu, X., Li, B. Y., et al. (2021). Human gut microbiome impacts skeletal muscle mass via gut microbial synthesis of the short-chain fatty acid butyrate among healthy menopausal women. J. Cachexia Sarcopenia Muscle. 12, 1860–1870. doi: 10.1002/jcsm.12788
Ma, Y., Liu, S., Shu, H., Crawford, J., Xing, Y., Tao, F. (2020). Resveratrol alleviates temporomandibular joint inflammatory pain by recovering disturbed gut microbiota. Brain Behav. Immun. 87, 455–464. doi: 10.1016/j.bbi.2020.01.016
Maixner, W., Diatchenko, L., Dubner, R., Fillingim, R. B., Greenspan, J. D., Knott, C., et al. (2011). Orofacial pain prospective evaluation and risk assessment study–the oppera study. J. Pain 12, T4–11. doi: 10.1016/j.jpain.2011.08.002
Manrriquez, S. L., Robles, K., Pareek, K., Besharati, A., Enciso, R. (2021). Reduction of headache intensity and frequency with maxillary stabilization splint therapy in patients with temporomandibular disorders-headache comorbidity: a systematic review and meta-analysis. J. Dent. Anesth. Pain Med. 21, 183–205. doi: 10.17245/jdapm.2021.21.3.183
Masucci, C., Oueiss, A., Maniere-Ezvan, A., Orthlieb, J. D., Casazza, E. (2020). [What is a malocclusion]? Orthod Fr. 91, 57–67. doi: 10.1684/orthodfr.2020.11
Murphy, M. K., Macbarb, R. F., Wong, M. E., Athanasiou, K. A. (2013). Temporomandibular disorders: a review of etiology, clinical management, and tissue engineering strategies. Int. J. Oral. Maxillofac. Implants. 28, e393–e414. doi: 10.11607/jomi.te20
Ouyang, Y., Chen, Y., Wang, G., Song, Y., Zhao, H., Xiao, B., et al. (2022). Genetically proxied gut microbiota, gut metabolites with risk of epilepsy and the subtypes: a bi-directional mendelian randomization study. Front. Mol. Neurosci. 15. doi: 10.3389/fnmol.2022.994270
Parada, V. D., de la Fuente, M. K., Landskron, G., Gonzalez, M. J., Quera, R., Dijkstra, G., et al. (2019). Short chain fatty acids (scfas)-mediated gut epithelial and immune regulation and its relevance for inflammatory bowel diseases. Front. Immunol. 10. doi: 10.3389/fimmu.2019.00277
Rot, I., Mardesic-Brakus, S., Costain, W. J., Saraga-Babic, M., Kablar, B. (2014). Role of skeletal muscle in mandible development. Histol Histopathol. 29, 1377–1394. doi: 10.14670/HH-29.1377
Schiffman, E., Ohrbach, R., Truelove, E., Look, J., Anderson, G., Goulet, J. P., et al. (2014). Diagnostic criteria for temporomandibular disorders (dc/tmd) for clinical and research applications: recommendations of the international rdc/tmd consortium network and orofacial pain special interest groupdagger. J. Oral. Facial Pain Headache. 28, 6–27. doi: 10.11607/jop.1151
Scrivani, S. J., Keith, D. A., Kaban, L. B. (2008). Temporomandibular disorders. N Engl. J. Med. 359, 2693–2705. doi: 10.1056/NEJMra0802472
Shen, Q., Huang, W., Qiu, Y., Wang, S., Zhang, B., Sun, N., et al. (2023). Bergapten exerts a chondroprotective effect in temporomandibular joint osteoarthritis by combining intestinal flora alteration and reactive oxygen species reduction. BioMed. Pharmacother. 167, 115525. doi: 10.1016/j.biopha.2023.115525
Shmagel, A., Onizuka, N., Langsetmo, L., Vo, T., Foley, R., Ensrud, K., et al. (2018). Low magnesium intake is associated with increased knee pain in subjects with radiographic knee osteoarthritis: data from the osteoarthritis initiative. Osteoarthritis Cartilage. 26, 651–658. doi: 10.1016/j.joca.2018.02.002
Shrivastava, M., Battaglino, R., Ye, L. (2021). A comprehensive review on biomarkers associated with painful temporomandibular disorders. Int. J. Oral. Sci. 13, 23. doi: 10.1038/s41368-021-00129-1
Swerdlow, D. I., Kuchenbaecker, K. B., Shah, S., Sofat, R., Holmes, M. V., White, J., et al. (2016). Selecting instruments for mendelian randomization in the wake of genome-wide association studies. Int. J. Epidemiol. 45, 1600–1616. doi: 10.1093/ije/dyw088
Ta, L. E., Dionne, R. A. (2004). Treatment of painful temporomandibular joints with a cyclooxygenase-2 inhibitor: a randomized placebo-controlled comparison of celecoxib to naproxen. Pain. 111, 13–21. doi: 10.1016/j.pain.2004.04.029
Thomas, D. C., Khan, J., Manfredini, D., Ailani, J. (2023). Temporomandibular joint disorder comorbidities. Dent. Clin. North Am. 67, 379–392. doi: 10.1016/j.cden.2022.10.005
Valesan, L. F., Da-Cas, C. D., Reus, J. C., Denardin, A., Garanhani, R. R., Bonotto, D., et al. (2021). Prevalence of temporomandibular joint disorders: a systematic review and meta-analysis. Clin. Oral. Investig. 25, 441–453. doi: 10.1007/s00784-020-03710-w
Vereecke, L., Elewaut, D. (2017). Spondyloarthropathies: ruminococcus on the horizon in arthritic disease. Nat. Rev. Rheumatol. 13, 574–576. doi: 10.1038/nrrheum.2017.130
Wen, Z., He, M., Peng, C., Rao, Y., Li, J., Li, Z., et al. (2019). Metabolomics and 16s rrna gene sequencing analyses of changes in the intestinal flora and biomarkers induced by gastrodia-uncaria treatment in a rat model of chronic migraine. Front. Pharmacol. 10. doi: 10.3389/fphar.2019.01425
Zhao, S. S., Holmes, M. V., Zheng, J., Sanderson, E., Carter, A. R. (2022). The impact of education inequality on rheumatoid arthritis risk is mediated by smoking and body mass index: mendelian randomization study. Rheumatol. (Oxford). 61, 2167–2175. doi: 10.1093/rheumatology/keab654
Zheng, J., Zhang, Y., Rasheed, H., Walker, V., Sugawara, Y., Li, J., et al. (2022a). Trans-ethnic mendelian-randomization study reveals causal relationships between cardiometabolic factors and chronic kidney disease. Int. J. Epidemiol. 50, 1995–2010. doi: 10.1093/ije/dyab203
Keywords: gut microbiota, temporomandibular joint disorder, Mendelian randomization, causal inference, genetic variation
Citation: Zhao K, JI S, Jiang H, Qian Y and Zhang W (2024) Exploring the gut microbiota’s effect on temporomandibular joint disorder: a two−sample Mendelian randomization analysis. Front. Cell. Infect. Microbiol. 14:1361373. doi: 10.3389/fcimb.2024.1361373
Received: 09 January 2024; Accepted: 19 July 2024;
Published: 12 August 2024.
Edited by:
Feng Tao, Texas A&M University, United StatesReviewed by:
Takahiko Shiba, Tokyo Medical and Dental University, JapanAlbraa Alolayan, Taibah University, Saudi Arabia
Liliana Argueta-Figueroa, Universidad Autónoma Benito Juárez de Oaxaca, Mexico
Copyright © 2024 Zhao, JI, Jiang, Qian and Zhang. This is an open-access article distributed under the terms of the Creative Commons Attribution License (CC BY). The use, distribution or reproduction in other forums is permitted, provided the original author(s) and the copyright owner(s) are credited and that the original publication in this journal is cited, in accordance with accepted academic practice. No use, distribution or reproduction is permitted which does not comply with these terms.
*Correspondence: Kai Zhao, MTE0MjA4Mzc5N0BxcS5jb20=