- Molecular Microbiology Laboratory, Department of Marine Biotechnology, Centro de Investigación Científica y de Educación Superior de Ensenada, Ensenada, Baja California, Mexico
The present study evaluated the capacity of three Bacillus species to improve health status and growth performance of Nile Tilapia fed with high levels of soybean meal and challenged with Aeromonas hydrophila. In vitro experiments showed that β-hemolysin and metalloprotease enzymes were produced by A. hydrophila throughout the exponential growth phase. In vivo experiments showed that 107 colony-forming units (CFUs)/ml of this pathogen killed 50% of control group fishes in 13 days. To evaluate the influence of Bacillus strains on health status and growth performance in Nile Tilapia, 180 fishes (33.44 + 0.05 g) were distributed in 12 tanks of 200 L each, and animals were fed twice per day until satiety. 1) Control group without Bacillus, 2) Bacillus sp1, 3) Bacillus sp2, and 4) Bacillus sp3 groups were formulated containing 106 CFU/g. After 40 days of feeding, the fishes were intraperitoneally injected with 1 ml of A. hydrophila at 2 × 107 CFU/ml, and mortality was recorded. The results showed that cumulative mortality rate was significantly (p< 0.05) lower in the Bacillus sp1 (25%), sp2 (5%), and sp3 (15%) groups, than the control group (50%). Weight gain was also significantly better (p< 0.05) in the Bacillus sp1 (36%), sp2 (67%), and sp3 (55%) groups with respect to the control group (30%). In conclusion, functional diet formulated with high levels of soybean meal and supplemented with Bacillus sp2 could be an alternative to protect Nile tilapia cultures from A. hydrophila infections and improve fish growth performance.
1 Introduction
Fish global demand has been growing in the last decades, and to satisfy present requirements, the aquaculture sector has developed several alternatives to improve its production. Intensive systems are capable of increasing fish production, but also increases fish susceptibility to bacterial infections due to stress induced on animals (Bondad-Reantaso et al., 2005; Makled et al., 2019). Aeromonas hydrophila is one of the most important pathogens in freshwater fish production. This bacterium is responsible for disease outbreaks and economical losses in aquaculture systems (Řehulka, 2002; Kumar, 2005; Abdel-Latif and Khafaga, 2020). A. hydrophila could produce toxins, hemolysins, and proteases, which are responsible for inducing systemic damage and fish death (Beaz-Hidalgo and Figueras, 2013; Tomás, 2012; El-Bahar et al., 2019). There is not enough information about this pathogen behavior; therefore, some questions arise as follows: 1) Which optimum conditions could grow it (growth medium, temperature, pH, aeration rate, etc.)? 2) How do these conditions influence virulence factor (VF) production? 3) When does VF initiate and finish its production? 4) How long (time) does VF reach their maximal concentration? Nowadays, antibiotics and other chemicals are the main compounds used for disease control in aquaculture; however, this strategy has induced pathogen resistance and food safety problems (Angulo et al., 2004; Cabello, 2006). New strategies to replace antibiotics in the aquaculture industry are being investigated to prevent and control disease outbreaks. Functional feeds formulated with probiotics and high levels of vegetable ingredients have been a successful alternative to improve the health status and growth performance of animals (Olmos et al., 2022). The Bacillus species are being used as probiotic bacteria in aquafeed formulations due to their growth capacity in different nutrient sources, tolerance for extreme environmental conditions, secretion of high levels of enzymes, production of antimicrobial compounds, and their being considered as Generally recognized as safe (GRAS) by the Food and Drug Administration (FDA) (Olmos and Paniagua Michel, 2014; Soltani et al., 2019; Kuebutornye et al., 2020a). Recently, the Bacillus species have been used in Nile Tilapia diets to protect it against A. hydrophila infections (Mehisan et al., 2015; Gobi et al., 2018; Naser et al., 2019; Kuebutornye et al., 2020b; Won et al., 2020; Xu et al., 2022). The results show that the addition of Bacillus to those diets improved health status and growth performance of the fish. In this work, Nile Tilapia weight gain and survival percentage were improved with respect to previous reports when Bacillus sp2 strain was included in feed formulated with high levels of soybean meal and challenged with A. hydrophila pathogen strain.
2 Materials and methods
2.1 Aeromonas hydrophila characterization
2.1.1 DNA purification
A. hydrophila CAIM675 strain was isolated from mouth lesions of rainbow trout at the Food and Development Research Center (CIAD, Mazatlán, Mexico). Bacterium was grown at 30°C in brain hart infusion medium (BHI) for 12 h at 200 rpm and centrifuged at 12,000 × g for 3 min. The pellet was suspended in 567 μl of Buffer TE and incubated with 5 μl of lysozyme and 10 μl of RNAasa. Three microliters of proteinase K and 30 μl of Sodium dodecyl sulfate (SDS) were added, and the sample was incubated for 1 h at 37°C. One hundred microliters of NaCl 5 M and 80 μl of Cetyltrimethylammonium bromide (CTAB)/NaCl were added, and the sample was incubated for 10 min at 65°C. To remove CTAB–protein/polysaccharides, isoamyl alcohol/chloroform solution was added and vortex was applied. Isoamyl alcohol/phenol–chloroform solution was added, vortexed, and centrifuged for 5 min. To DNA precipitation, 360 μl of isopropanol was added, and the sample was then mixed and centrifuged. Ethanol at 70% was used to wash and purify DNA; the sample was centrifuged and dried by Vacufuge for 10 min at 30°C. The pellet was suspended in Buffer TE and stored at −20°C (Sambrook et al., 1989).
2.1.2 Virulence gene identification
PCR reactions were carried out in a 25-μl final volume using 12.5 μl of Taq 2× Master mix, 0.5 μl of each primer, 0.5 μl of DNA, and 11 μl of H2O dd. The amplification was performed using 25 cycles in a BioRad iCycler Thermal Cycler with a melting temperature (Tm) of 53°C to all reactions. One cycle consisted of 1 min at 95°C, 1 min at 53°C, and 2 min at 72°C. To identify virulence genes, primers in Table 1 were designed. PCR amplification was analyzed through electrophoresis using 1.2% agarose gel.
2.1.3 Growth conditions and virulence factors production
A. hydrophila was grown at 30°C and 200 rpm for 12 h in Erlenmeyer flasks of 250-ml capacity containing 30 ml of Brain-heart infusion (BHI) medium (MCD LAB, Mexico). New flasks with 27 ml of BHI medium were inoculated with 3 ml of preinoculum mentioned above and grown at the same conditions. Bacterium growth was followed each hour at 600 nm, and samples were collected every 2 h. A supernatant obtained through centrifugation at 12,000 × g for 10 min at 4°C was passed through a sterile 0.2-µm filter (Supor® 200 Membrane Disc Filters, 0.2 µm to 13 mm, plain 100/pkg; Pall Corporation), and 1 ml of the sample was stored at −20°C. Hemolytic activity was evaluated applying 40 μl of filtered sample onto blood–agar plates. Proteolytic activity was evaluated applying 40 μl of filtered sample onto skim milk agar plates. All experiments were performed in triplicate, and plates were incubated at 30°C for 24 h. The degradation zone around colonies was measured in mm2.
2.1.4 Median lethal dose identification (LD50)
LD50 in Nile Tilapia was estimated by following the method of Reed and Muench (1938) with slight modifications: A. hydrophila was grown in BHI medium at 30°C and 200 rpm for 6 h, optical density was measured at 600 nm, and colony-forming units (CFUs) were obtained in agar plates. Subsequently, 10 fishes weighing 60 ± 0.5 g were intraperitoneally injected with 1 ml of A. hydrophila at 2 × 107 CFU/ml. Fishes were monitored, and typical infection symptoms were identified and recorded according to Yardimci and Aydin (2011).
2.2 Bacillus strain characterization
2.2.1 Bacillus strain identification
Bacillus strains used in this research were obtained from our laboratory collection at the Ensenada Center for Scientific Research and Higher Education (CICESE, Ensenada, Baja California, Mexico). Three strains named sp1, sp2, and sp3 were selected for their capacity to grow in soy-based media and inhibit A. hydrophila development in agar plates. For molecular identification, sp1, sp2, and sp3 strains were grown in LB medium for 12 h to DNA extraction. DNA purification was carried out by standard phenol:chloroform:isoamyl alcohol (25:24:1) procedure, and its quality was evaluated by agarose gel (1.2% w/v) electrophoresis. 16S rDNA gene amplification was performed using Bacillus-specific oligonucleotides F (5′-ACAGAGTTTGATCCTGGCTCAG-3′) and R (5′-CCCAGTTTCCAATGACC-3′) previously described (Arellano and Olmos, 2002), and products were sequenced by the Institute of Biotechnology (IBT) of the National Autonomous University of Mexico (UNAM). Strain identity was determined by the NCBI database using 16S rDNA gene and Basic Local Alignment Search Tool (BLAST). Phylogenetic identification analysis was performed using the MEGA X program.
2.2.2 Antimicrobial activity against A. hydrophila
Antimicrobial activity was evaluated making co-cultures of Bacillus sp1, sp2, and sp3 strains with A. hydrophila in BHI agar plates at 30°C for 24 h. Inhibition zone was measured in mm2 and recorded.
2.2.3 Bacillus strain enzymatic activity
Protease, carbohydrase, and lipase activity was measured according to Ochoa-Solano and Olmos-Soto (2006). Protease production was evaluated using skim milk and soy agar plates. Carbohydrase activity was tested using a medium with starch, corn meal, or wheat flour. Lipase production was evaluated using olive oil at 2.5% (w/v) and Rhodamine B solution at 0.001% (w/v). All plates were incubated at 37°C; bacterial growth and degradation zone around colonies were measured after 24 h.
2.2.4 Antibiotic resistance
Following the CSLI (Clinical and Laboratory Standards Institute) and EFSA (European Food Safety Authorities) guidelines, Bacillus strains and A. hydrophila were tested for resistance to ampicillin, chloramphenicol, oxytetracycline, erythromycin, streptomycin, clindamycin, cephalexin, and doxycycline. Plates were incubated for 24 h at 37°C for Bacillus and 30°C for A. hydrophila.
2.2.5 Bacillus strain growth
Bacillus strains were grown in Luria-Bertani (LB) agar plates at 37°C for 12 h, and Erlenmeyer flasks containing 30 ml of LB broth were inoculated and incubated at 37°C and 250 rpm for 12 h. Three milliliters of preinoculum mentioned above were inoculated in 27 ml of Schaffer medium, and growth was permitted at conditions mentioned above. Bacillus strain growth was measured each hour at 600 nm, and samples were taken every 2 h.
2.3 Diet formulation
Table 2 shows the ingredients used in the control and experimental diets formulated in this experiment. Diets were prepared, dried at 60°C, and stored. Bacillus sp1, sp2, and sp3 strains were added to feed formulation at 2 × 106 CFU/g.
2.4 Experimental design
Juveniles (180) of Nile tilapia (33.44 + 0.05 g) were obtained from a local fish farm (Baja California, México) and acclimated for 2 weeks in 2,000-L-water-capacity tanks at 25°C. Fish were randomly distributed in 12 tanks with 200-L water capacity, fed twice a day until satiation with corresponding diet, and parameters were maintained at the following conditions: temperature: 25 ± 2°C, Optical Density (OD): 6.8 ± 0.3 mg/L, pH: 7.4 ± 0.7). After 40 days, growth performance was measured using the next equations:
where FW is the final weight, IW is the initial weight, m is the mean, and t is the time.
2.5 Challenging test with A. hydrophila
After 40 days of growth, all fishes were inoculated intraperitoneally with 1 ml of A. hydrophila solution using 2 × 107 CFU/ml, mortality was recorded, and percentage was calculated using the following equation:
2.6 Statistical analysis
Data obtained from growth performance were analyzed by one-way analysis of variance (ANOVA) to determine significant variations. Differences between groups were calculated with Tukey’s test. Data were expressed as a mean ± standard error (SE). GraphPad Prism 9 Software (LLC, Boston, MA, USA) was used to perform statistical analyses.
3 Results
3.1 A. hydrophila characterization
3.1.1 Virulence gene detection
To evaluate the strain capacity of A. hydrophila to produce virulence factors, six pairs of oligonucleotides were designed (Table 1). PCR results showed that this strain contain hylA (717 bp) and ahpB (520 bp) virulence genes reported with hemolytic and protease activity, respectively (Figure 1).
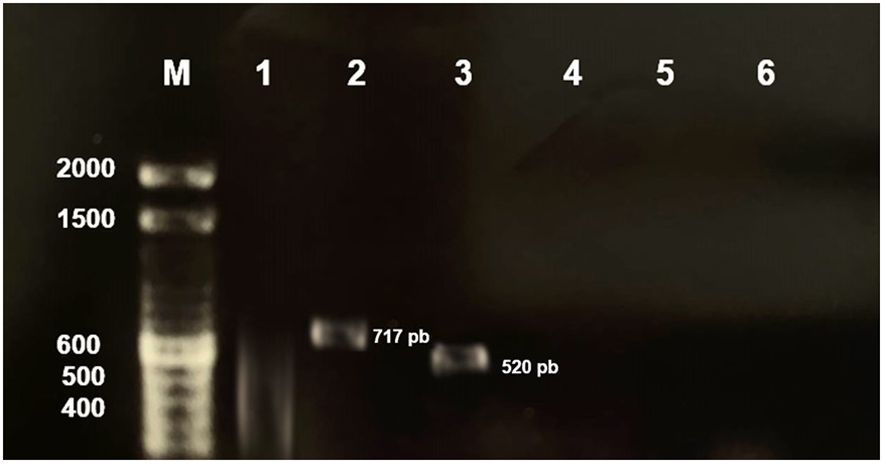
Figure 1 Detection of A. hydrophila CAIM675 virulence genes in 1.2% agarose gel. Electrophoresis of PCR products revealed existence of hylA (717 pb) and ahpB (520 pb) virulence genes. (M) Molecular marker, (1) act, (2) hylA, (3) ahpB, (4) ast, (5) ser, and (6) aerA.
3.1.2 Growth kinetic and virulence factor production
The growth of A. hydrophila in BHI medium is shown in Figure 2A. To evaluate virulence factor production, samples were taken every 2 h throughout the growth curve. Hemolysin activity was observed only at the early exponential growth phase and does not appear again (Figure 2B). Protease activity began just after hemolysin activity ended, and it lasted several hours (Figure 2B) showing its highest proteolytic activity at 6 h of culture initiation (Figure 2C).
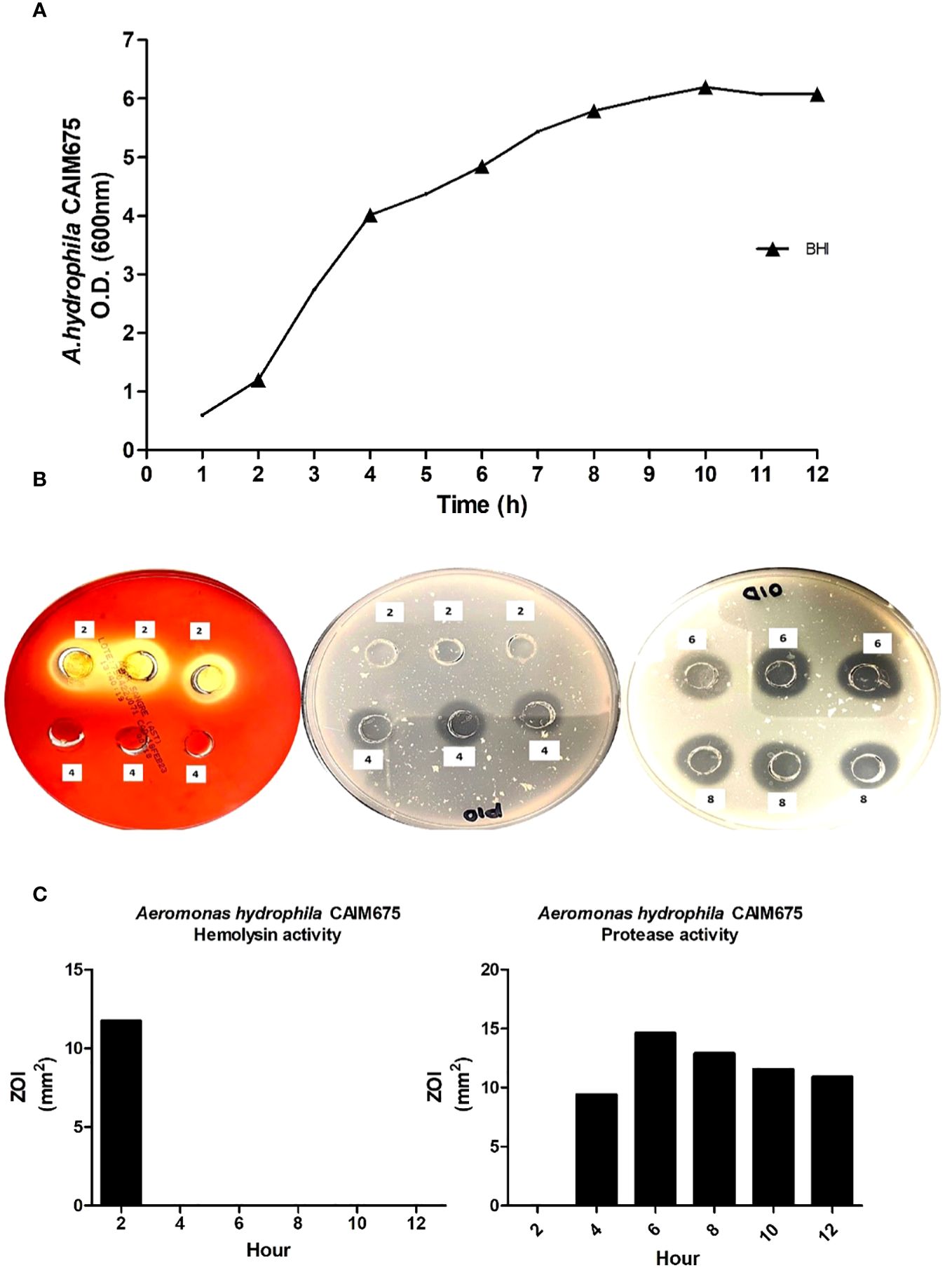
Figure 2 (A) Growth kinetic and VF detection (▴) in A. hydrophila CAIM675 cultured in BHI medium. The bacterium was cultivated at 37°C and 200 rpm. Growth was followed for 12 h, and samples were collected throughout the exponential growth phase. (B) Assessment of hemolytic and proteolytic activities in A. hydrophila supernatants; plates were incubated at 30°C for both 24 h, and assays were performed in triplicate. (C) Hemolytic and proteolytic activities in A. hydrophila CAIM675 EPCs; enzymatic halos were measured in mm2, and corresponding values were recorded.
3.1.3 Median lethal dose identification (LD50)
Seven days after the inoculation of pathogen, the fishes started to show typical symptoms (hemorrhages, exophthalmia, necrosis, ulcers) of A. hydrophila infection (Figure 3). LD50 in the control group was reached after 13 days of infection using 2 × 107 CFU/ml of the pathogen bacterium.
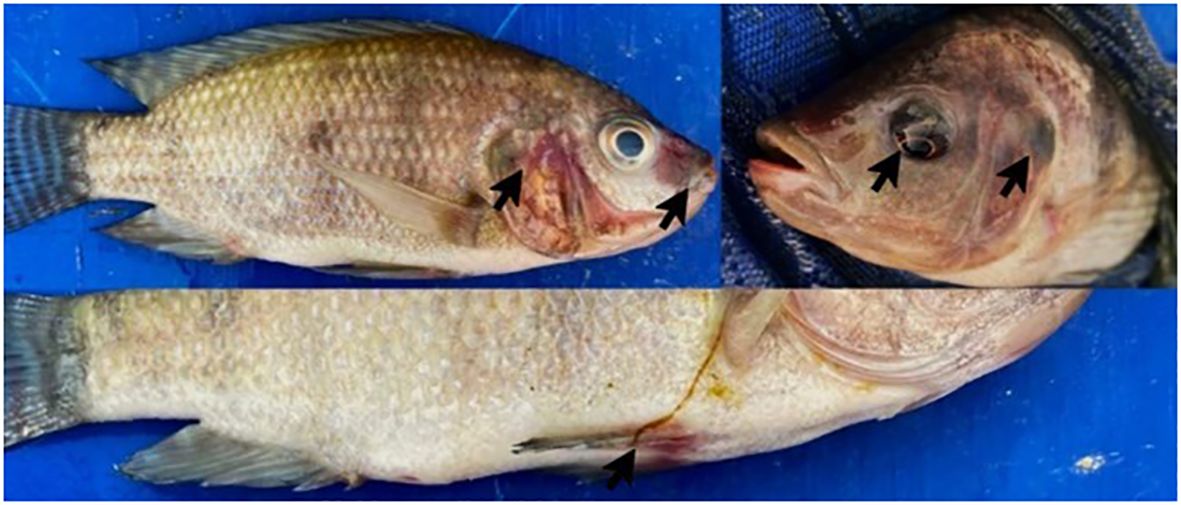
Figure 3 Nile Tilapia infected with A. hydrophila CAIM675. Arrows indicate typical symptoms and signs of A. hydrophila infection, such as necrosis, hemorrhage, and exophthalmia.
3.2 Bacillus strain characterization
3.2.1 Bacillus strain identification
Bacillus sp1, sp2, and sp3 strains were selected from a group of 30 sporulating bacteria due to their capacity to grow in soybean products and inhibit A. hydrophila in agar plates. After the selection, Bacillus strains were grown overnight in LB liquid medium. DNA was extracted, and 16S rDNA genes were amplified. Its sequence was deposited in the PubMed database (sp1 OR504279, sp2 PP229194, and sp3 OR504281). Phylogenetic identification shows that strains sp1, sp2, and sp3 are closely related to Bacillus velezensis and Bacillus amyloliquefaciens; species that share almost 100% identity belong to the GRAS group of Bacillus subtilis (Huynh et al., 2022) (Figure 4).
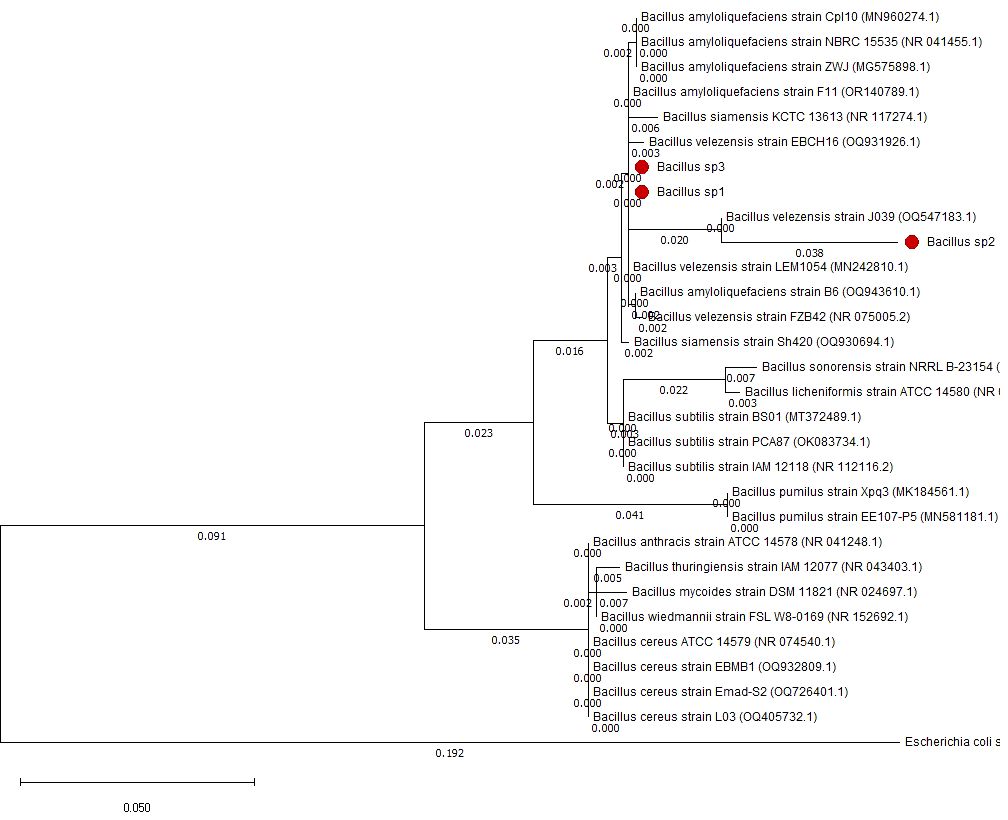
Figure 4 Phylogenetic tree made with 16S rDNA sequences to identify Bacillus sp1, sp2, and sp3 strains.
3.2.2 Bacillus antimicrobial activity
Figure 5 represents the antimicrobial activity produced by Bacillus sp1, sp2, and sp3 strains against A. hydrophila. The results show that the sp2 strain produced the highest inhibition activity against the pathogen bacteria producing three- and twofold more activity than the sp1 and sp3 strains, respectively.
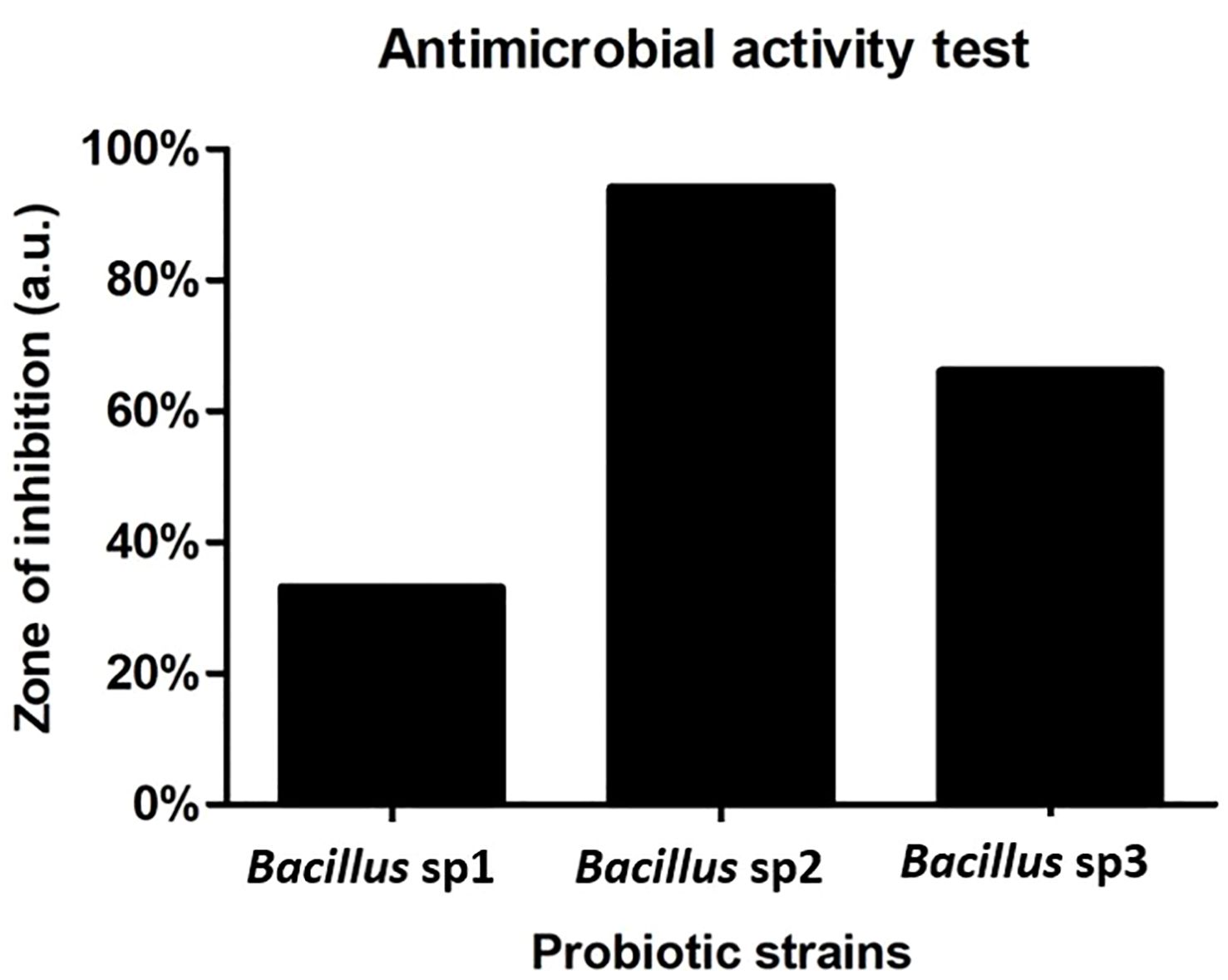
Figure 5 Antimicrobial activity of Bacillus strains against A. hydrophila CAIM675. Bacillus sp2 produced three- and twofold more activity than sp1 and sp3, respectively.
3.2.3 Bacillus enzymatic activity
Table 3 shows the growth and enzymatic activity of Bacillus sp1, sp2, and sp3 strains in substrates commonly used in aquafeed formulation. The three strains produced similar growth in all the assayed substrates; however, degradation activity produced by these strains was slightly different among them.
3.2.4 Bacillus antibiotic resistance
Table 4 shows that only A. hydrophila and Bacillus cereus that was used as the positive control grew in streptomycin and ampicillin, respectively. However, neither sp1, sp2, nor sp3 grew in any of the assayed antibiotics.
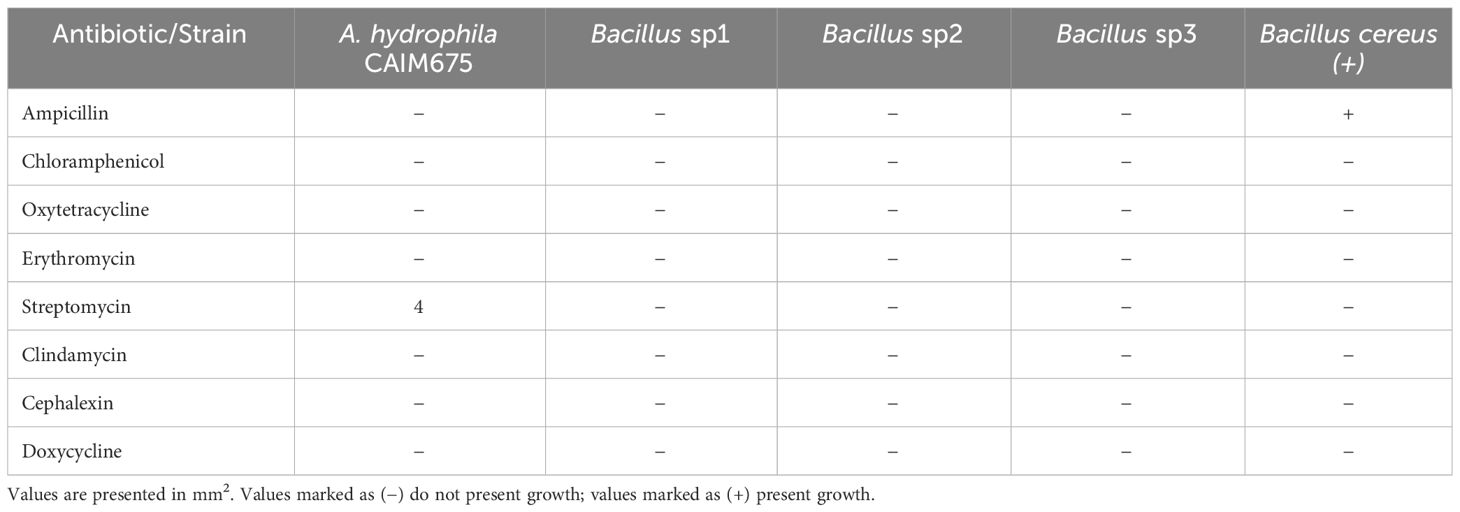
Table 4 Antibiotic resistance test following CSLI and EFSA guidelines of Bacillus and A. hydrophila CAIM675 strains.
3.2.5 Bacillus sp2 strain growth curve
Figure 6 shows the growth curve obtained for sp2 Bacillus strain in Schaeffer medium. At T0, the exponential growth ended, and the sporulation process began. T8 indicates the end of the sporulation process and sampling time for diet preparation. The growth of Bacillus sp1 and sp3 was omitted due to a similar behavior.
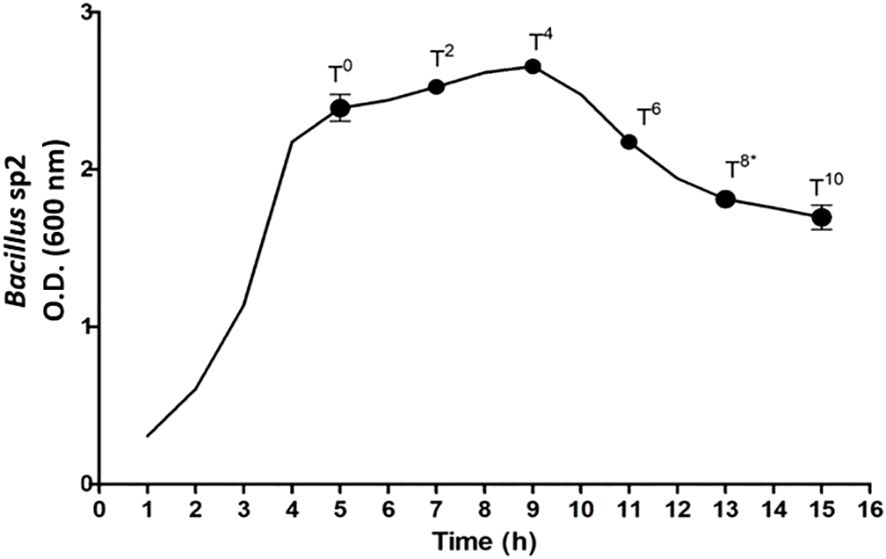
Figure 6 Bacillus sp2 strain growth curve in Schaeffer medium. T0 indicates the end of exponential growth, and sporulation process began. T8 indicates the end of the sporulation process.
3.3 Nile tilapia growth performance
After 40 days of feeding, the fish were measured, and the results were recorded. Table 5 shows the weight gained, specific growth rate, and other growth parameters that were analyzed in the experiment. Figure 7 shows that most of these parameters were significantly improved in the Bacillus sp2 group with respect to the control, and sp1 and sp3 experimental groups (Figure 7).
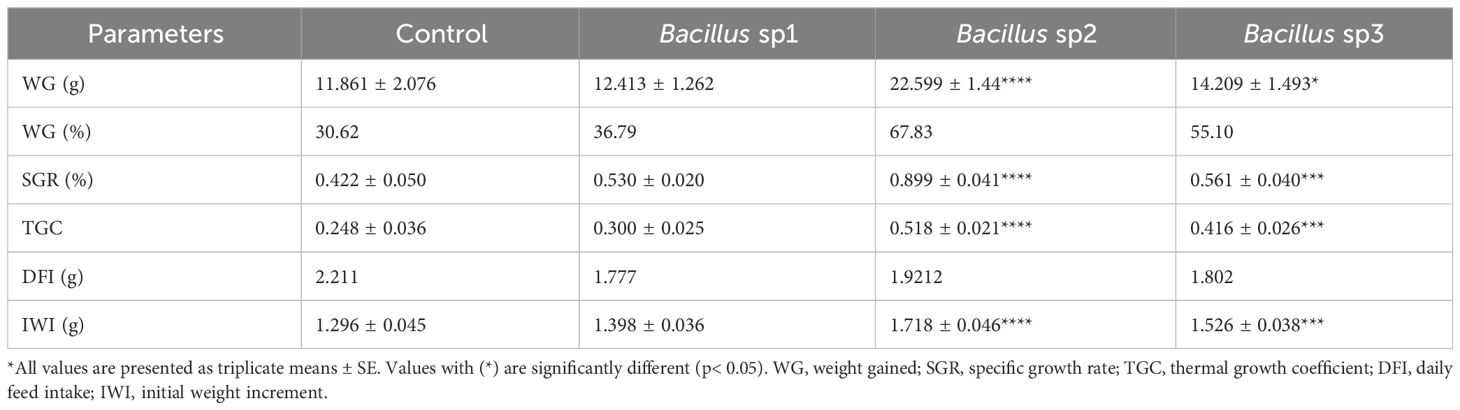
Table 5 Growth performance of Nile Tilapia 40 days after diet supplementation with the Bacillus strains.
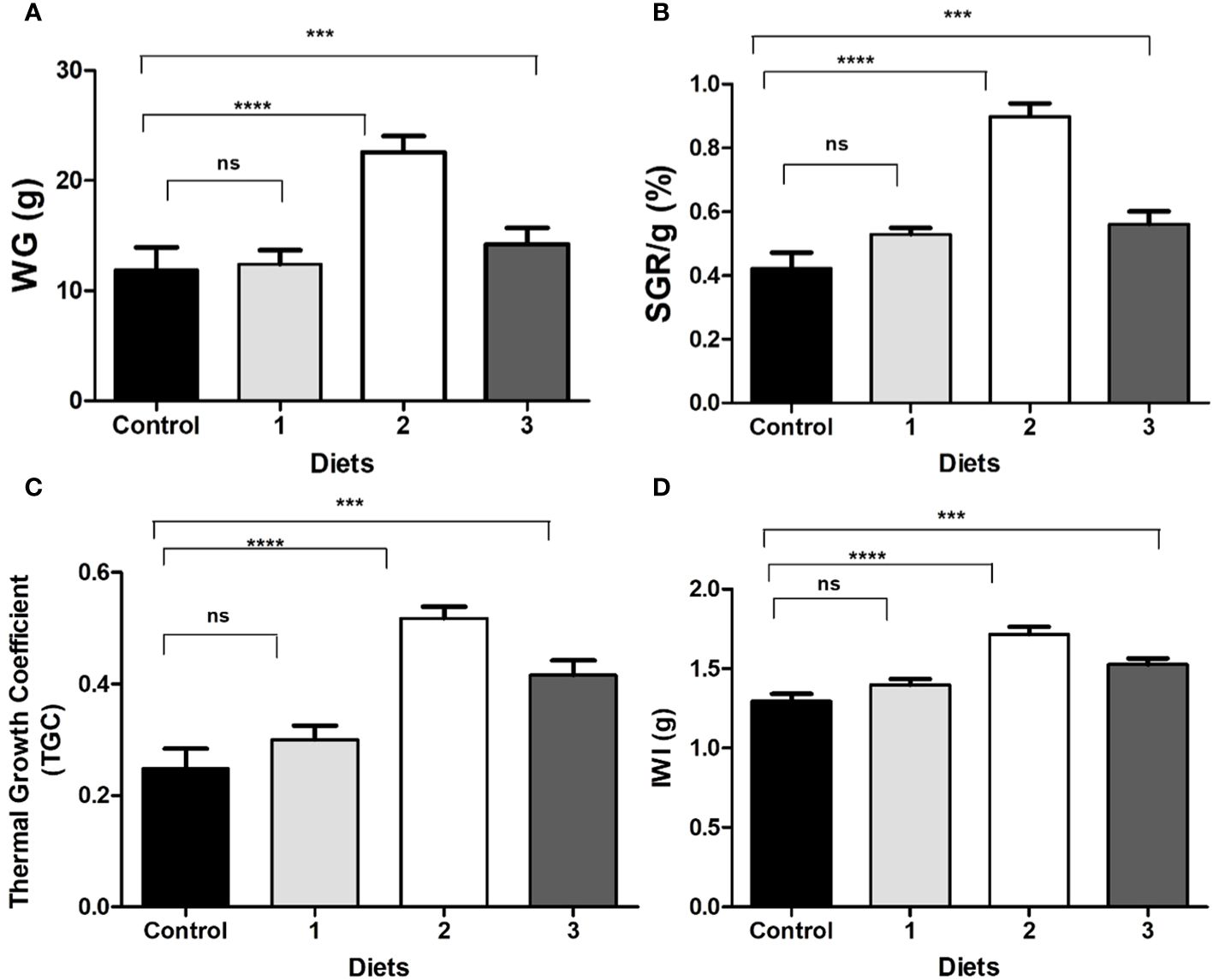
Figure 7 (A) Gained weight (WG); (B) specific growth rate (SGR); (C) thermal growth coefficient (TGC); (D) initial weight increment (IWI) of Nile Tilapia fed with control diet and functional diets for 40 days. (Control) Diet without Bacillus, (1) Bacillus sp1 diet, (2) Bacillus sp2 diet, (3) Bacillus sp3. Values are means ± SE of triplicate. *Groups are statistically different (p< 0.05), and (ns) are not statistically different.
3.4 Challenging test with A. hydrophila
Fishes infected with Aeromonas hydrophila after 40 days of feeding were followed for an additional 15 days (Figure 8). The results show that the control group reached LD50 after 13 days of initial infection. Concurrently, the cumulative mortality of Bacillus sp1, sp2, and sp3 reached 25%, 5%, and 15%, respectively, on the same day (Figure 8).
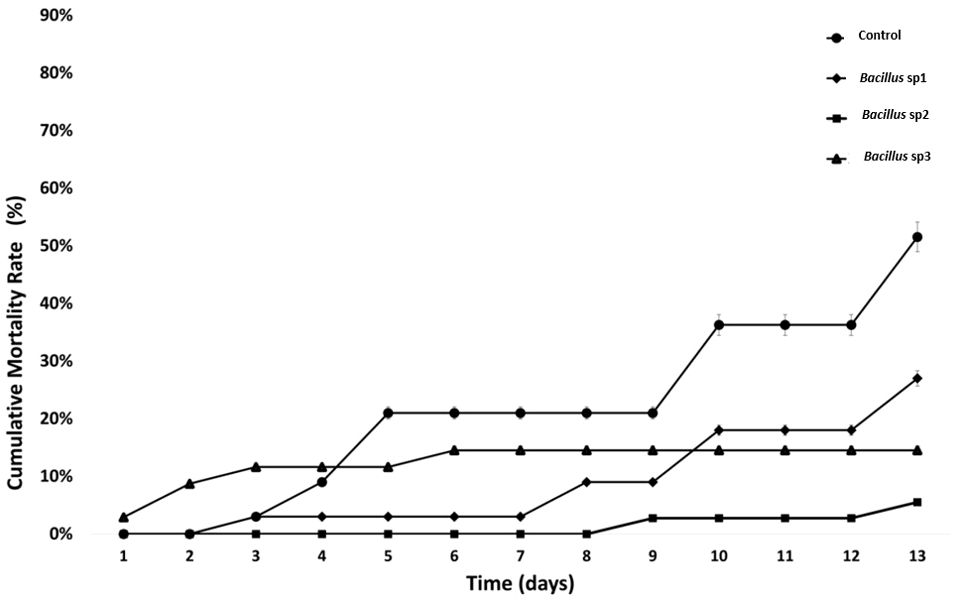
Figure 8 Cumulative mortality rate (%) in Nile Tilapia fed with control and functional diets, and challenged with A. hydrophila CAIM675 for 15 Days. The control group reached LD50 on the 13th day.
4 Discussion
Nile Tilapia is susceptible to pathogen bacteria, such as A. hydrophila, that induce disease outbreaks and economic losses on intensive culture systems (Kayansamruaj et al., 2014). A. hydrophila produce VFs, such as hemolysin, protease, and lipase enzymes, which have been reported as responsible for Nile Tilapia tissue damage and death. Hemolytic and proteolytic activities in tilapia cultures have been directly related to A. hydrophila infections (El-Bahar et al., 2019). It is reported that hemolysin is primarily responsible for tissue damage, enteritis, hemorrhages, and anemia; nevertheless, Kanai and Wakabayashi (1984) demonstrated that A. hydrophila proteases also produce hemorrhage and muscle necrosis in fish, as does hemolysin. In this sense, the A. hydrophila strain used in this work amplified an hylA gene, which has been related to hemolysin activity, and an ahpB gene, which has been associated with metalloprotease activity (Figure 1) (Rasmussen-Ivey et al., 2016). The hylA gene product is a cytolytic pore-forming toxin secreted by Aeromonas that binds to cell membrane receptors and induces hemolysis (Tomás, 2012; Dubey et al., 2022). On the other hand, the ahpB gene product has been related to virulence activity in rainbow trout (Cascón et al., 2000). Both hemolysin and protease enzymes are among the most reported virulence factors in A. hydrophila isolates (Li et al., 2011). It is known that VF production depends on the Aeromonas strain and culture conditions utilized (Tomás, 2012; Beaz-Hidalgo and Figueras, 2013). Therefore, the culture conditions required to produce virulence factors in A. hydrophila CAIM675 strain were investigated in this work (data not shown). BHI medium at 30°C and 200 rpm were identified as the optimum conditions to grow this pathogen (Figure 2A). The A. hydrophila strain grown under these conditions reached higher optical density compared to those of previous reports (Khalil and Mansour, 1997; Sahu et al., 2011). In addition, VF and their specific production time were characterized. In this sense, hemolysin activity was found only at the beginning of the culture, while protease activity was maintained throughout the exponential growth phase (Figure 2B). Some authors have reported that Aeromonas can produce alpha-hemolysin during the entire exponential growth phase and beta-hemolysin only at the first hour (Bernheimer and Avigad, 1974; Ljungh et al., 1981; Asao et al., 1986; Tomás, 2012).
In this work, unlike hemolysin production, proteolytic activity was recorded throughout the exponential growth and early stationary phase (Figure 2B), as was reported by Khalil and Mansour (1997). In addition, the maximum proteolytic activity was obtained 6 h after culture initiation (Figure 2C). Therefore, LD50 assay on Nile Tilapia was performed using this culture sample (Figure 3).
Bacillus species have been used to inhibit the virulence factor effects of A. hydrophila on Nile Tilapia cultures (Mehisan et al., 2015; Gobi et al., 2018; Kuebutornye et al., 2020b; Won et al., 2020). In this study, the antimicrobial activity of Bacillus sp1, sp2, and sp3 strains was phylogenetically identified (Figure 4) and evaluated in vitro against A. hydrophila (Figure 5). This figure shows that Bacillus sp2 was the most effective in inhibiting A. hydrophila development, producing 60% and 30% more antimicrobial activity than sp1 and sp3 strains, respectively. In addition, Nile Tilapia fed with a functional feed supplemented with the Bacillus strains and high levels of soybean meal was infected with A. hydrophila CAIM675 strain after 40 days of feeding. In this experiment, groups fed with Bacillus sp1 (25%), sp2 (5%), or sp3 (15%) strain showed less cumulative mortality compared to the control group (50%) (Figure 8). Therefore, Bacillus sp2 was the most effective strain in protecting Nile Tilapia from A. hydrophila infections. In this sense, in vivo results are in agreement with in vitro results mentioned above (Figure 5). The Bacillus capacity to protect Nile Tilapia cultures against A. hydrophila has shown good results (Mehisan et al., 2015; Gobi et al., 2018); however, the mortality (5%) of the sp2 group has been the lowest rate reported until now (Figure 9).
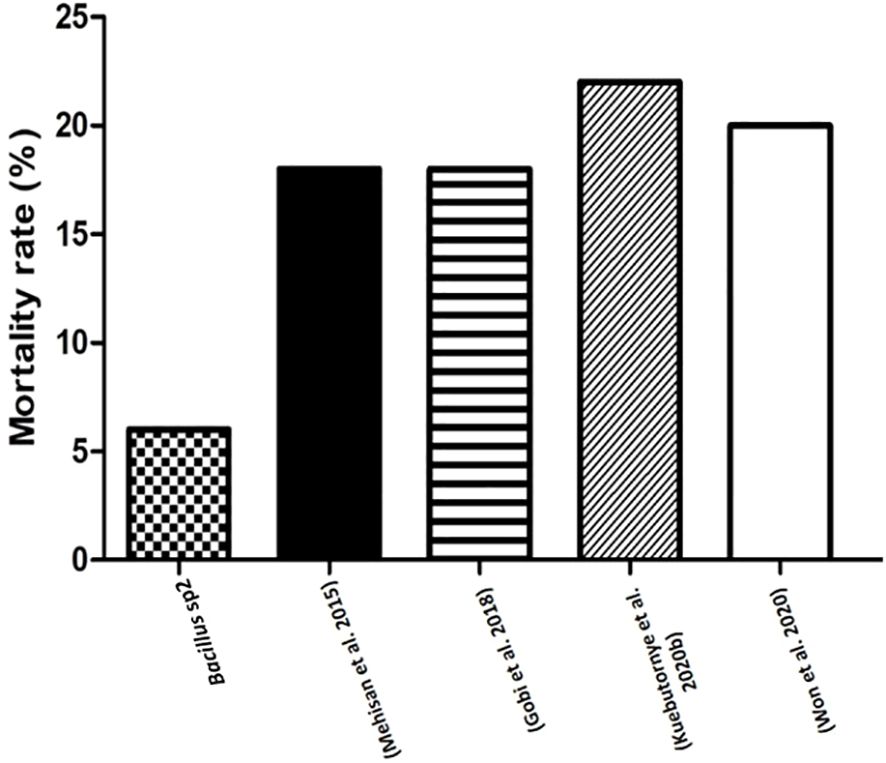
Figure 9 Comparative mortality rates (%) of Nile Tilapia challenged with A. hydrophila. This graph compares mortality rates of Nile Tilapia fed with sp2 diet and other reported diets with Bacillus strains, and challenged with A. hydrophila.
Antimicrobial peptides, lytic enzymes, organic acids, quorum quenching, nutrient competition, and host immunostimulation, are mechanisms used by Bacillus species to control pathogen development . In this sense, some authors have reported the capacity of Bacillus peptides to control A. hydrophila in Nile Tilapia cultures (Banerjee et al., 2017; Feliatra et al., 2018).
Moreover, to demonstrate the capacity of Bacillus to protect Nile Tilapia from A. hydrophila infections, Bacillus strains used in this work also showed the capacity to improve growth performance of these fishes (Table 5). Thus, diets supplemented with Bacillus sp1 (36%), sp2 (67%), and sp3 (55%) strains showed better growth performance than the control group (30%) without Bacillus especially the Bacillus sp2 group, which improved all parameters (Table 5 and Figure 7). Similar findings have been reported on Nile Tilapia when Bacillus coagulans (Mehisan et al., 2015), Bacillus amyloliquefaciens (Naser et al., 2019), and Bacillus licheniformis (Gobi et al., 2018), were supplemented to Nile Tilapia diets. Nevertheless, the Bacillus sp2 group produced better results as it duplicated the weight of the Tilapia in comparison with the control group (Figure 10). It is important to mention that even when sp1, sp2, and sp3 induced different growths in Tilapia and inhibited A. hydrophila with different capacities, they were isolated from the same fermented soybean sample. In this sense, this could be the reason they are so phylogenetically related (Figure 4). Results obtained in this work highlight the properties of the sp2-isolated strain to inhibit in vitro development of A. hydrophila (Figure 5) and reduce pathogen infection capacity in Tilapia (Figure 9). In addition, the sp2 strain induced better growth performance than the other reported Bacillus strains (Figure 10).
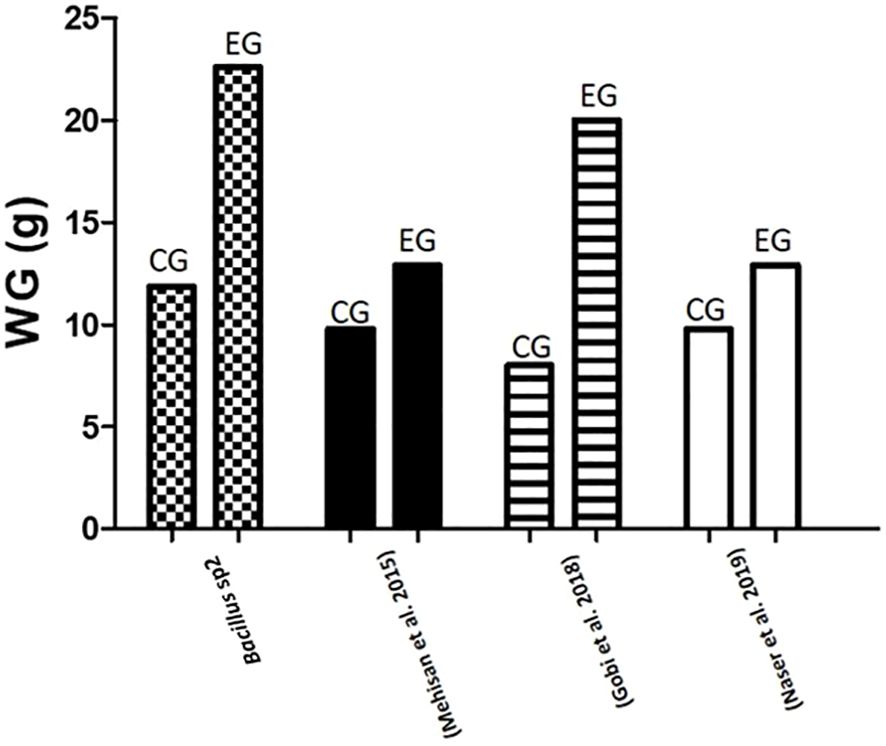
Figure 10 Comparative gained weight (g) in Nile Tilapia experiments. This graph compares results of weight gain in Nile Tilapia cultures fed with control diet (CG) and diet supplemented with sp2 Bacillus strain (EG) against other similar experiments reported in the literature.
FAO (2023) suggested the concentration of macronutrients of Nile Tilapia diet as follows: protein 30%–35%, fat 10%–15%, and carbohydrate 40%–50%. Therefore, diets formulated in this work followed this recommendation (Table 2). The Bacillus species can grow in a great diversity of nutrient sources due to its capacity to produce protease, carbohydrase, and lipase enzymes (Olmos et al., 2020). In this experiment, the Bacillus strains grew well in vegetable sources such as soybean meal (Table 3). Soybean products have been used as fish product replacement to prevent environmental contamination, overfishing, and high prices (Tacon and Metian, 2008; Olmos Soto, 2017). However, soy and other leguminous plants contain antinutritional factors, such as trypsin inhibitors, lectins, and toxic oligosaccharides, with capacity to produce tissue damage and animal death. In this sense, some Bacillus species have been reported with the capacity to break down antinutritional factors contained in soy products (Shi et al., 2017; Giliyaru et al., 2018).
Some authors have reported aquafeed formulation using soy products and Bacillus strains; Oreochromis niloticus (Addo et al., 2017), Pagrus major (Zaineldin et al., 2021), and Totoaba macdonaldi (Olmos et al., 2022) are some successful examples. In this sense, the growth performance of Nile Tilapia obtained in this work suggests that Bacillus strains, especially Bacillus sp2, could alleviate antinutritional factor effects produced by soybean products (Table 5 and Figure 7). Gobi et al. (2018) found a similar growth rate using even more soybean meal; however, the authors used almost three times more fishmeal than was used in this experiment (Figure 11).
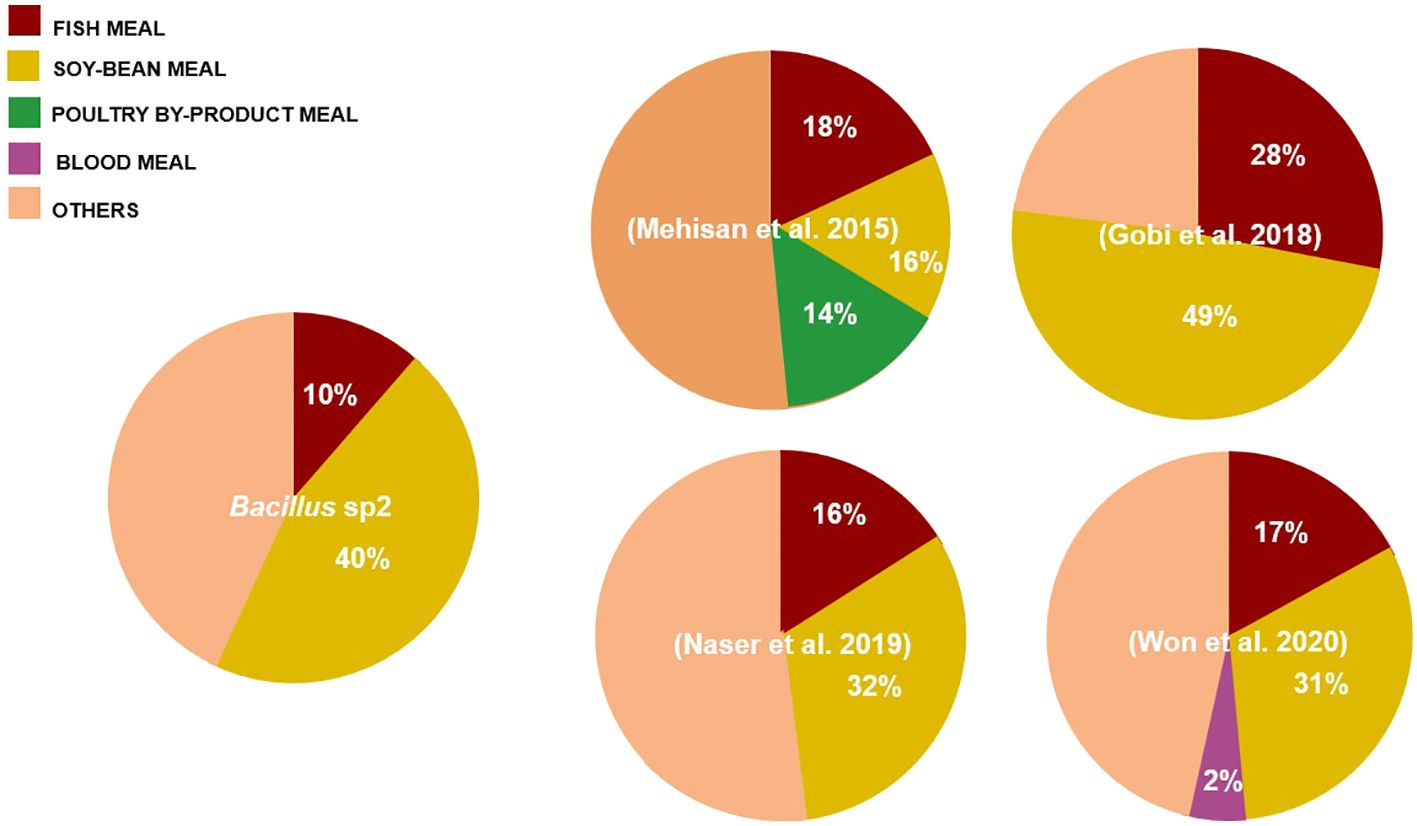
Figure 11 Comparison of diets formulated with Bacillus and soybean meal (%) used in Nile Tilapia cultures. The pie chart compares our formulation with respect to formulations used in other experiments.
5 Conclusion
A. hydrophila produce virulence factors implicated in Nile Tilapia disease outbreaks. Hemolysin and protease enzymes have been identified as responsible for Nile Tilapia tissue damage and death. In this work, hylA and ahpB gene products seem to be the virulence factors responsible for inducing tissue damage and death in cultured fishes. In the present study, a functional feed was formulated with soybean meal and the Bacillus sp2 strain capable of increasing the health status of Nile Tilapia by inhibiting A. hydrophila strain development and VF production. In addition, this feed enhanced growth performance in Nile Tilapia as the Bacillus sp2 strain had the capacity to grow in a great variety of plant-based substrates such as soybean meal and other leguminous plants. Additionally, the sp2 strain was identified as part of the B. subtilis group; therefore, it can be considered GRAS for further commercial feed development.
Data availability statement
The raw data supporting the conclusions of this article will be made available by the authors, without undue reservation.
Ethics statement
The animal study was approved by Comité de Ética CICESE, Centro de Investigación Científica y de Educación Superior de Ensenada, Baja California. The study was conducted in accordance with the local legislation and institutional requirements.
Author contributions
LM: Data curation, Investigation, Methodology, Writing - original draft. VM: Investigation, Methodology, Writing - review & editing. JO: Investigation, Methodology, Supervision, Writing - review & editing.
Funding
The author(s) declare that no financial support was received for the research, authorship, and/or publication of this article.
Conflict of interest
The authors declare that the research was conducted in the absence of any commercial or financial relationships that could be construed as a potential conflict of interest.
Publisher’s note
All claims expressed in this article are solely those of the authors and do not necessarily represent those of their affiliated organizations, or those of the publisher, the editors and the reviewers. Any product that may be evaluated in this article, or claim that may be made by its manufacturer, is not guaranteed or endorsed by the publisher.
References
Abdel-Latif, H. M., Khafaga, A. F. (2020). Natural cotural, N.RE of cultured Nile tilapia Oreochromis niloticus with Aeromonas hydrophila and Gyrodactylus cichlidarum experiencing high mortality during summer. Aquac Res. 51, 1880–1892. doi: 10.1111/are.14538
Addo, S., Carrias, A. A., Williams, M. A., Liles, M. R., Terhune, J. S., Davis, D. A. (2017). Effects of Bacillus subtilis strains on growth, immune parameters, and Streptococcus iniae susceptibility in Nile tilapia, Oreochromis niloticus. J. World Aquac Soc. 48, 257–267. doi: 10.1111/jwas.12380
Angulo, F., Nargund, V., Chiller, T. (2004). Evidence of an association between use of antieentions.1 agents in food animals and antialstions.1 resistance among bacteria isolated from humans and the human health consequences of such resistance. J. Vet. Med. B 51, 374–379. doi: 10.1111/j.1439-0450.2004.00789.x
Arellano, F., Olmos, J. (2002). Thermostable α-1,4- and α-1,6-glucosidase enzymes from Bacillus sp. Isolated from a marine environment. World J. Microbiol. Biotechnol. 18, 791–795. doi: 10.1023/A:1020433210432
Asao, T., Kozaki, S., Kato, K., Kinoshita, Y., Otsu, K., Uemura, T., et al. (1986). Purification and characterization of an Aeromonas hydrophila hemolysin. J. Clin. Microbiol. 24, 228–232. doi: 10.1128/jcm.24.2.228-232.1986
Banerjee, G., Nandi, A., Ray, A. K. (2017). Assessment of hemolytic activity, enzyme production and bacteriocin characterization of Bacillus subtilis LR1 isolated from the gastrointestinal tract of fish. Arch. Microbiol. 199, 115–124. doi: 10.1007/s00203-016-1283-8
Beaz-Hidalgo, R., Figueras, M. (2013). Aeromonas spp. whole genomes and virulence factors implicated in fish disease. J. Fish Dis. 36, 371–388. doi: 10.1111/jfd.12025
Bernheimer, A. W., Avigad, L. S. (1974). Partial characterization of aerolysin, a lytic exotoxin from Aeromonas hydrophila. Infect. Immun. 9, 1016–1021. doi: 10.1128/iai.9.6.1016-1021.1974
Bondad-Reantaso, M. G., Subasinghe, R. P., Arthur, J. R., Ogawa, K., Chinabut, S., Adlard, R., et al. (2005). Disease and health management in Asian aquaculture. Vet. Parasitol. 132, 249–272. doi: 10.1016/j.vetpar.2005.07.005
Cabello, F. C. (2006). Heavy use of prophylactic antibiotics in aquaculture: a growing problem for human and animal health and for the environment. Environ. Microbiol. 8, 1137–1144. doi: 10.1111/j.1462-2920.2006.01054.x
Cascón, A., Yugueros, J., Temprano, A., Sánchez, M., Hernanz, C., Luengo, J. M., et al. (2000). A major secreted elastase is essential for pathogenicity of Aeromonas hydrophila. Infect. Immun. 68, 3233–3241. doi: 10.1128/IAI.68.6.3233-3241.2000
Dubey, S., Ager-Wick, E., Peng, B., Evensen, Ø., Sørum, H., Munang’andu, H. M. (2022). Characterization of virulence and antimicrobial resistance genes of Aeromonas media strain SD/21-15 from marine sediments in comparison with other Aeromonas spp. Front. Microbiol. 13. doi: 10.3389/fmicb.2022.1022639
El-Bahar, H. M., Ali, N. G., Aboyadak, I. M., Khalil, S. A. E. S., Ibrahim, M. S. (2019). Virulence genes contributing to Aeromonas hydrophila pathogenicity in Oreochromis niloticus. Int. Microbiol. 22, 479–490. doi: 10.1007/s10123-019-00075-3
FAO (2023). Oreochromis niloticus. Cultured Aquatic Species Information Programme (Rome: Fisheries and Aquaculture Division).
Feliatra, F., Muchlisin, Z. A., Teruna, H. Y., Utamy, W. R., Nursyirwani, N., Dahliaty, A. (2018). Potential of bacteriocins produced by probiotic bacteria isolated from tiger shrimp and prawns as antibacterial to Vibrio, Pseudomonas, and Aeromonas species on fish. F1000Res 7, 415. doi: 10.12688/f1000research.13958.1
Giliyaru, R., Prakash, M., Gonvindarajalu, V. (2018). Identification and characterization of a calcium dependent bacillopeptidase from Bacillus subtilis CFR5 with novel kunitz trypsin inhibitor degradation activity. Food Res. Int. 103, 263–272. doi: 10.1016/j.foodres.2017.10.049
Gobi, N., Vaseeharan, B., Chen, J.-C., Rekha, R., Vijayakumar, S., Anjugam, M., et al. (2018). Dietary supplementation of probiotic Bacillus licheniformis Dahb1 improves growth performance, mucus and serum immune parameters, antioxidant enzyme activity as well as resistance against Aeromonas hydrophila in tilapia Oreochromis mossambicus. Fish Shellfish Immunol. 74, 501–508. doi: 10.1016/j.fsi.2017.12.066
Huynh, T., Vörös, M., Kedves, O., Turbat, A., Sipos, G., Leitgeb, B., et al. (2022). Discrimination between the two closely related species of the operational group B.amyloliquefaciens based on whole-cell fatty acid profiling. Microorganisms 10, 418. doi: 10.3390/microorganisms10020418
Kanai, K., Wakabayashi, H. (1984). Purification and same properties of protease from Aeromonas hydrophila. Bull. Japanese Soc. Sci. Fisheries 50, 1367–1374. doi: 10.2331/suisan.50.1367
Kayansamruaj, P., Pirarat, N., Katagiri, T., Hirono, I., Rodkhum, C. (2014). Molecular characterization and virulence gene profiling of pathogenic Streptococcus agalactiae populations from tilapia (Oreochromis sp.) farms in Thailand. J. Vet. Diagn. Invest. 26, 488–495. doi: 10.1177/1040638714534237
Khalil, A. H., Mansour, E. H. (1997). Toxicity of crude extracellular products of Aeromonas hydrophila in tilapia, Tilapia nilotica. Lett. Appl. Microbiol. 25, 269–273. doi: 10.1046/j.1472-765X.1997.00220.x
Kuebutornye, F., Abarike, E. D., Lu, Y., Hlordzi, V., Sakyi, M. E., Afriyie, G., et al. (2020a). Mechanisms and the role of probiotic Bacillus in mitigating fish pathogens in aquaculture. Fish Physiol. Biochem. 46, 819–841. doi: 10.1007/s10695-019-00754-y
Kuebutornye, F., Wang, Z., Lu, Y., Abarike, E. D., Sakyi, M. E., Li, Y., et al. (2020b). Effects of three host-associated Bacillus species on mucosal immunity and gut health of Nile tilapia, Oreochromis niloticus and its resistance against Aeromonas hydrophila infection. Fish Shellfish Immunol. 97, 83–95. doi: 10.1016/j.fsi.2019.12.046
Kumar, P. (2005). Abdominal dropsy disease in major carps of Meghalaya: isolation and characterization of Aeromonas hydrophila. Curr. Sci. 88, 1897–1900.
Li, J., Ni, X. D., Liu, Y. J., Lu, C. P. (2011). Detection of three virulence genes alt, ahp and aerA in Aeromonas hydrophila and their relationship with actual virulence to zebrafish. J. Appl. Microbiol. 110, 823–830. doi: 10.1111/jam.2011.110.issue-3
Ljungh, A., Wretlind, B., Möllby, R. (1981). Separation and characterization of enterotoxin and two haemolysins from Aeromonas hydrophila. Acta Pathol. Microbiol. Scand. B 89, 387–397. doi: 10.1111/j.1699-0463.1981.tb00205_89B.x
Makled, S. O., Hamdan, A. M., EldSayed, A. F. M. (2019). Effects of dietary supplementation of a marine thermotolerant bacterium, Bacillus paralicheniformis SOral on growth performance and immune responses of Nile tilapia, Oreochromis niloticus. Aquac Nut 25, 817–827. doi: 10.1111/anu.12899
Mehisan, M., El-moghazy, G., El-Sayed, A. E.-K., Iraqi, M., Soltan, M. (2015). Role of probiotics in improving growth performance, immunity and controlling Aeromonas hydrophila in the Nile tilapia Oreochromis niloticus. Egypt J. Aquat Biol. Fish 19, 55–70. doi: 10.21608/EJABF.2015.2271
Naser, M. E., El-Motaal, A., Sabry, M., El-Sheikh, S. M., Mahmoud, S. H. (2019). Effects of commercial and fish isolated gut Bacillus strains supplementation on the performance of Oreochromis niloticus challenged with Aeromonas hydrophila. Egyptian J. Aquaculture 9, 61–75. doi: 10.21608/eja.2019.33105
Ochoa-Solano, J. L., Olmos-Soto, J. (2006). The functional property of Bacillus for shrimp feeds. Food Microbiol. 23, 519–525. doi: 10.1016/j.fm.2005.10.004
Olmos, J., Acosta, M., Mendoza, G., Pitones, V. (2020). Bacillus subtilis, an ideal probiotic bacterium to shrimp and fish aquaculture that increase feed digestibility, prevent microbial diseases, and avoid water pollution. Arch. Microbiol. 202, 427–435. doi: 10.1007/s00203-019-01757-2
Olmos, J., López, L. M., Gorriño, A., Galaviz, M. A., Mercado, V. (2022). Bacillus subtilis Effects on Growth Performance and Health Status of Totoaba macdonaldi Fed with High Levels of Soy Protein Concentrate. Animals 12, 3422. doi: 10.3390/ani12233422
Olmos, J., Paniagua Michel, J. (2014). Bacillus subtilis a potential probiotic bacterium to formulate functional feeds for aquaculture. J. Microb. Biochem. Technol. 6, 361–365. doi: 10.4172/1948-5948.1000169
Olmos Soto, J. (2017). "Chapter two - Bacillus probiotic enzymes: external auxiliary apparatus to avoid digestive deficiencies, water pollution, diseases, and economic problems in marine cultivated animals," in Advances in food and nutrition research, eds. Kim, S.-K., Toldrá, F.. (Academic Press), 15–35.
Rasmussen-Ivey, C. R., Figueras, M. J., McGarey, D., Liles, M. R. (2016). Virulence factors of Aeromonas hydrophila: in the wake of reclassification. Front. Microbiol. 1337. doi: 10.3389/fmicb.2016.01337
Reed, L. J., Muench, H. (1938). A simple method of estimating fifty per cent endpoints12. Am. J. Epidemiol. 27, 493–497. doi: 10.1093/oxfordjournals.aje.a118408
Řehulka, J. (2002). Aeromonas causes severe skin lesions in rainbow trout (Oncorhynchus mykiss): clinical pathology, haematology, and biochemistry. Acta Veterinaria Brno 71, 351–360. doi: 10.2754/avb200271030351
Sahu, I., Das, B. K., Marhual, N., Samanta, M., Mishra, B. K., Eknath, A. E. (2011). Toxicity of Crude Extracellular Products of Aeromonas hydrophila on Rohu, Labeo rohita (Ham.). Indian J. Microbiol. 51, 515–520. doi: 10.1007/s12088-011-0182-6
Sambrook, J., Fritsch, E. F., Maniatis, T. (1989). Molecular Cloning: A Laboratory Manual (USA: Cold Spring Harbor Laboratory).
Shi, C., Zhang, Y., Lu, Z., Wan, Y. (2017). Solid-state fermentation of corn-soybean meal mixed feed with Bacillus subtilis and Enterococcus faecium for degrading antinutritional factors and enhancing nutritional value. J. Anim. Sci. Biotechnol. 8, 50. doi: 10.1186/s40104-017-0184-2
Soltani, M., Ghosh, K., Hoseinifar, S. H., Kumar, V., Lymbery, A. J., Roy, S., et al. (2019). Genus Bacillus, promising probiotics in aquaculture: aquatic animal origin, bio-active components, bioremediation and efficacy in fish and shellfish. Rev. Fish Sci. Aquac 27, 331–379. doi: 10.1080/23308249.2019.1597010
Tacon, A. G. J., Metian, M. (2008). Global overview on the use of fish meal and fish oil in industrially compounded aquafeeds: Trends and future prospects. Aquaculture 285, 146–158. doi: 10.1016/j.aquaculture.2008.08.015
Tomás, J. (2012). The main Aeromonas pathogenic factors. ISRN Microbiol. 2012, 22. doi: 10.5402/2012/256261
Won, S., Hamidoghli, A., Choi, W., Park, Y., Jang, W. J., Kong, I.-S., et al. (2020). Effects of Bacillus subtilis WB60 and Lactococcus lactis on growth, immune responses, histology and gene expression in Nile tilapia, Oreochromis niloticus. Microorganisms 8, 67. doi: 10.3390/microorganisms8010067
Xu, R., Ding, F.-F., Zhou, N.-N., Wang, T., Wu, H.-X., Qiao, F., et al. (2022). Bacillus amyloliquefaciens protects Nile tilapia against Aeromonas hydrophila infection and alleviates liver inflammation induced by high-carbohydrate diet. Fish Shellfish Immunol. 127, 836–842. doi: 10.1016/j.fsi.2022.07.033
Yardimci, B., Aydin, Y. (2011). Pathological findings of experimental Aeromonas hydrophila infection in Nile tilapia (Oreochromis niloticus). Ankara Üniv Vet. Fak Derg 58, 47–54. doi: 10.1501/Vetfak_0000002448
Zaineldin, A. I., Hegazi, S., Koshio, S., Ishikawa, M., El Basuini, M. F., Dossou, S., et al. (2021). The influences of Bacillus subtilis Cubtil inclusion in the red sea bream diet containing high levels of soybean meal on growth performance, gut morphology, blood health, immune response, digestibility, digestive enzymes, and stress resistance. Aquac Nut 27, 2612–2628. doi: 10.1111/anu.13389
Keywords: Aeromonas hydrophila, Bacillus, Nile tilapia, probiotics, functional foods
Citation: Macias L, Mercado V and Olmos J (2024) Assessment of Bacillus species capacity to protect Nile tilapia from A. hydrophila infection and improve growth performance. Front. Cell. Infect. Microbiol. 14:1354736. doi: 10.3389/fcimb.2024.1354736
Received: 12 December 2023; Accepted: 19 February 2024;
Published: 09 July 2024.
Edited by:
Jose Ramos-Vivas, Universidad Europea del Atlántico, SpainReviewed by:
Rajeev Meora, Inha University, Republic of KoreaRajagopal Kammara, Central Food Technological Research Institute (CSIR), India
Copyright © 2024 Macias, Mercado and Olmos. This is an open-access article distributed under the terms of the Creative Commons Attribution License (CC BY). The use, distribution or reproduction in other forums is permitted, provided the original author(s) and the copyright owner(s) are credited and that the original publication in this journal is cited, in accordance with accepted academic practice. No use, distribution or reproduction is permitted which does not comply with these terms.
*Correspondence: Jorge Olmos, jolmos@cicese.mx
†ORCID: Leslye Macias, orcid.org/0009-0004-3396-3708
Víctor Mercado, orcid.org/0000-0002-7748-4352
Jorge Olmos, orcid.org/0000-0002-6797-5385